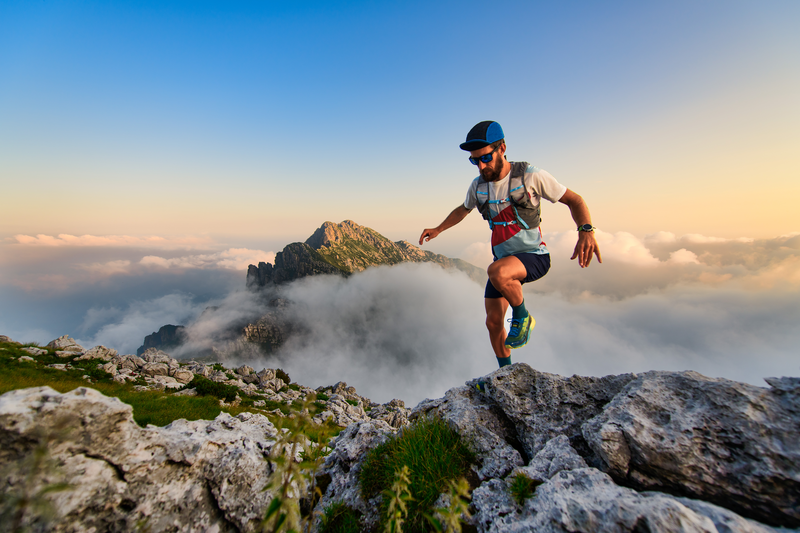
94% of researchers rate our articles as excellent or good
Learn more about the work of our research integrity team to safeguard the quality of each article we publish.
Find out more
REVIEW article
Front. Immunol.
Sec. T Cell Biology
Volume 16 - 2025 | doi: 10.3389/fimmu.2025.1563150
The final, formatted version of the article will be published soon.
You have multiple emails registered with Frontiers:
Please enter your email address:
If you already have an account, please login
You don't have a Frontiers account ? You can register here
Dynamic changes in various forms of RNA modification are critical to the functional homeostasis of the immune system and the pathophysiology of autoimmune diseases. RNA modification-related proteins play an essential role in these processes. At present, the research methods of RNA modification in autoimmune diseases are mainly to detect the expression changes of RNA modification-related proteins in tissues or cells, but there is a lack of explorations of target RNAs and in-depth mechanisms. Considering the important role of CD4+ T cell dysfunction in the pathogenesis and progression of autoimmune diseases, the regulatory effect of abnormal RNA modification on CD4+ T cells deserves attention, which will provide a perspective for further exploring the mechanism of RNA modification in autoimmune diseases. In this Review, we discuss the abnormal RNA modification changes in patients with autoimmune diseases and highlight the effects of these abnormal changes on CD4+ T cells.
Keywords: post-transcriptional modification, RNA modification, Autoimmune Diseases, CD4+ T cells, T cell differentiation
Received: 19 Jan 2025; Accepted: 06 Mar 2025.
Copyright: © 2025 Yu, Ma, Su, Xu and YI. This is an open-access article distributed under the terms of the Creative Commons Attribution License (CC BY). The use, distribution or reproduction in other forums is permitted, provided the original author(s) or licensor are credited and that the original publication in this journal is cited, in accordance with accepted academic practice. No use, distribution or reproduction is permitted which does not comply with these terms.
* Correspondence:
HUANFA YI, Jilin University, Changchun, China
Disclaimer: All claims expressed in this article are solely those of the authors and do not necessarily represent those of their affiliated organizations, or those of the publisher, the editors and the reviewers. Any product that may be evaluated in this article or claim that may be made by its manufacturer is not guaranteed or endorsed by the publisher.
Research integrity at Frontiers
Learn more about the work of our research integrity team to safeguard the quality of each article we publish.