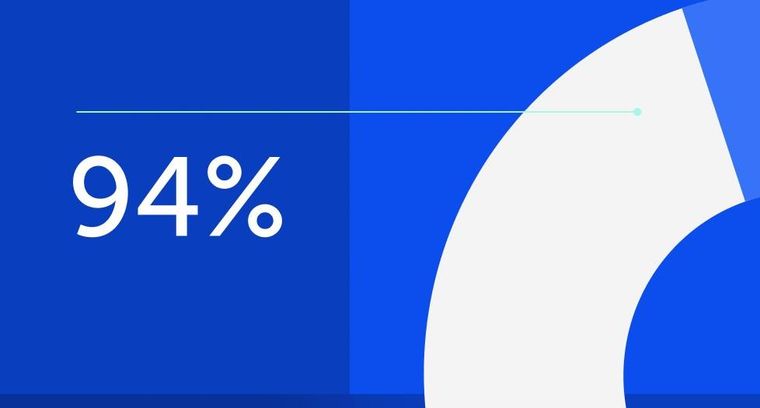
94% of researchers rate our articles as excellent or good
Learn more about the work of our research integrity team to safeguard the quality of each article we publish.
Find out more
REVIEW article
Front. Immunol., 25 March 2025
Sec. Inflammation
Volume 16 - 2025 | https://doi.org/10.3389/fimmu.2025.1562546
More than 10% of adults suffer from chronic rhinosinusitis (CRS), a chronic inflammatory condition that lowers quality of life, reduces productivity, and shortens work hours. Every year, more than 1 million surgeries are performed worldwide as a result of CRS. In recent years, targeted therapy for CRS has become a hotspot of research at home and abroad and has made significant progress, but CRS still has a high recurrence rate. Therefore CRS urgently needs precise targeted therapy. In the pathological process of CRS, the involvement of eosinophils is an important inflammatory mechanism. And excessive aggregation of eosinophils often leads to severe inflammatory responses. Studies have shown that chitinase 3-like protein 1 (CHI3L1) plays a key role in the activation and migration of eosinophils. This review will combine the latest research results to analyse in detail the biological properties of CHI3L1, its expression pattern in CRS, and the possible mechanisms by which it affects eosinophil aggregation by regulating immune responses and inflammatory processes, which will provide insights into the key role of CHI3L1 in the pathological process of CRS and offer a new target for the treatment of CRS.
Chronic sinusitis is a chronic purulent inflammation of the sinuses over time. According to epidemiological studies, 5% ~ 12% of the world’s population suffer from CRS (1–4). Diagnosed by CT or nasal endoscopy, based on symptoms and clinical assessment, with a prevalence ranging from 1.2% to 6.8% (5–8). It is shown that the definitive diagnosis of CRS needs to be diagnosed with definitive tests. Although appropriate pharmacologic or surgical treatments are currently administered to patients with CRS, according to a cross-sectional analysis of patients with CRS, treatment is incomplete in 40 per cent of patients, leading to disease recurrence and affecting the quality of subsequent survival (9). In recent years, darbepoetin, omalizumab, and mepolizumab (10–12) have been used to target IL-4R α, IgE, and IL-5, respectively, in CRS, but the results are still poor. Therefore, precision targeted therapy for CRS is still imminent.
CRS is characterized by persistent inflammation of the nasal mucosa and sinus tissue with at least two symptoms: nasal congestion and/or runny nose with impaired sense of smell or facial pain or pressure lasting at least 12 weeks (4, 13). CRS can be clinically categorized into two types, chronic sinusitis with nasal polyps (CRSwNP) and chronic sinusitis without nasal polyps (CRSsNP) (14). Although the clinical presentation of the two types is very diverse, they frequently display distinct pathological characteristics and inherent pathogenic pathways despite having the same phenotype. Another classification is based on endophenotypes, which are groups characterized by certain disease processes and/or biological markers. Based on the variation in the expression content among the main cytokines in the patient’s endophenotype, we divide CRS into three groups: type 1, which is mediated by the T1 cytokine IFN-γ; type 2, which is mediated by the T2 cytokines IL-5 and eosinophilia; and type 3, which is mediated by the T3 cytokine IL-17A (15, 16). CRS can be further divided into two categories: eosinophilic CRS (ECRS) and non-ECRS (NECRS), depending on the proportion of eosinophils in the nasal mucosal tissue (17). Increased tissue eosinophil levels and a Th2-type inflammatory response, which is frequently linked to allergy illness, are characteristics of ECRS (18). The incidence and prevalence of eosinophilic chronic sinusitis vary widely according to the region and ethnicity. Previous studies have reported that eosinophilic chronic sinusitis is predominantly found in patients with chronic sinusitis in Western countries, accounting for up to 80% of all patients with chronic sinusitis. In Asian countries, such as China and Japan, eosinophilic chronic sinusitis is found in less than 50% of all patients with chronic sinusitis (19). Most of the patients had eosinophilia, and it was recently discovered that the endophenotype of CRSwNP in Asia gradually changed to type 2 inflammation (20, 21). It is hypothesized that the westernization of lifestyles in Asian nations is the cause of this growing tendency. According to Wirach Chitsuthipakorn et al.’s usage of cut-off values and standardized definitions, the percentage of ECRS in Asia may not differ from that in Europe and the US (22). The prevalence of ECRS and the ensuing rise in CRS severity are no longer limited to the West; they are now a global issue. Precision targeted therapy for ECRS is therefore urgent. A growing body of research in recent years has demonstrated the critical role eosinophils play in the pathophysiology of chronic sinusitis. Natural immune granulocytes called eosinophils are important in type 2 inflammation. Numerous active chemicals found in eosinophils can be released from the cell in response to external stimuli, resulting in a range of healthy or pathological reactions. Four granule-associated proteins have been identified in eosinophils, including major basic protein (MBP), eosinophil cationic protein (ECP), eosinophil peroxidase (EPO), and eosinophil-derived neurotoxin (EDN), which have been shown to have the potential to inhibit the growth of bacteria and resist the survival of parasites (23–25). Conversely, these proteins are also cytotoxic, and their excessive release not only elicits an inflammatory response, but also damages local tissues and promotes the formation of nasal polyps (26, 27). In addition, eosinophil activation releases a large number of inflammatory mediators that remodel the vascular epithelium, increase vascular permeability, and allow fluid to leak out, leading to fluid collection in the nasal mucosa further leading to mucosal swelling. In chronic sinusitis with eosinophilia, the eosinophil infiltration environment further leads to the above situation, further aggravating the formation and development of nasal polyps (27). Although the exact cause of ECRS is unknown, it is presently thought that S. aureus and its enterotoxins stimulate the Th2 system in order to encourage the generation of IgE and eosinophil infiltration via a number of different mechanisms (28). The buildup of fibrin webs in nasal polyps results from the simultaneous activation of the coagulation system and inhibition of the fibrinolytic system. The formation of nasal polyps is likely caused by the disruption of the fibrinolytic system, which impedes the fibrinolytic pathway and results in excessive fibrin deposition. This is supported by the fact that the concentration of t-PA in the leptomeningeal tissues of patients with CRSsNP and patients with a simple deviated septum was significantly higher than that in the nasal polyp tissues of patients with CRSwNP. Nasal polyps develop as a result of the excessive fibrin deposition caused by this (29, 30). Therefore, modulation of relevant targets controlling fibrin deposition may be a novel approach for the treatment of ECRS.
CHI3L1, another name for YKL-40, is a glycoprotein implicated in a number of inflammatory conditions and tissue remodeling processes. According to earlier research, patients with atopic dermatitis or asthma have noticeably higher serum levels of CHI3L1, indicating that CHI3L1 is linked to allergic disorders (31, 32). Through its effects on fibrosis, tissue remodeling, and immune cell recruitment, it is also thought to play a role in the pathophysiology of the illness. According to recent research, CHI3L1 is crucial for controlling the fibrinolytic system. In particular, the Th2 inflammatory response is upregulated by CHI3L1 (33). By encouraging macrophage differentiation toward the M2 type, disruption of the Th1/Th2 balance increases fibrin deposition in the ECRS. As a biomarker, elevated levels of CHI3L1 have been linked to eosinophilic respiratory conditions like asthma (31, 34, 35). It is clear that the importance of CHI3L1 in inflammatory diseases cannot be ignored.
This review will delve into the specific mechanism of action of CHI3L1 on eosinophilic inflammation in CRS. We will review the existing research results, analyse the structure, expression and its function of CHI3L1, analyse the role of CHI3L1 in various diseases, especially its expression in CRS and its mechanism of action, and analyse how CHI3L1 affects the pathological process of CRS by regulating the function of eosinophils. In addition, we will discuss the possibility of CHI3L1 as a potential therapeutic target and the direction of future research. Through these discussions, we hope to provide new ideas and strategies for the diagnosis and treatment of CRS.
A highly conserved glycoprotein that belongs to the family of chitinase 3-like proteins, CHI3L1 is a single polypeptide chain consisting of 383 amino acid residues with an isoelectric point of 7.6 (36, 37). It was first identified in mouse breast cancer cells and is referred to as mammary degradation protein 39 (BRP39) in mice (38). It is a 40 kDa secreted mammalian glycoprotein that can bind chitin but does not have the enzyme activity to catalyze chitin. A chitinase-like structural domain, a C-terminal glycosylation site, and an N-terminal signal peptide are all present in its molecular structure (39). It has recently been determined what CHI3L1’s native structure is. Four separate CHI3L1 monomers that interact with one another in a highly comparable way make up the structure (Figure 1). The four monomers contain 117 water molecules and 22–383 residues. One noteworthy feature of the CHI3L1 crystal structure was the lack of a leading sequence from the first 21 N-terminal residues. The ability of CHI3L1 to bind chitin, as previously mentioned, but the absence of enzymatic activity to catalyze chitin is also explained by this structure.The structural domains of barrel glycoside hydrolase family 18 (GH18), which are typical of chitinases and chitinase-like proteins, are also present in the crystal structure of CHI3L1 (40, 41). The conserved cysteine residues seen in the crystal structure of CHI3L1 are also generated via a variety of disulfide bonds. These residues are thought to be a significant factor in the stability and biological function of CHI3L1 and can aid in the crystal structure’s development (42, 43). It is interesting to note that the researchers discovered that two of the three cis-peptide linkages in the crystal structure of CHI3L1 were situated in the sugar-binding motif (44). CHI3L1 is N-glycosylated at Asn60 and has two β(1, 4)-linked Glc NAc residues. After rigorous analysis using the electron densitometry technique, it was discovered that there was a particularly noticeable sequence mismatch at position 311, where an isoleucine residue rather than the predicted threonine was present. The researchers used mass spectrometry to demonstrate that these sequence discrepancies were similar in all four asymmetric CHI3L1 monomers. We have a foundation for understanding the biological role and possible therapeutic benefit of CHI3L1 thanks to the clarification of its crystal structure and the variations in sequence between its four asymmetric monomers.
Figure 1. CHI3L1’s (YKL40) biological and pathological characteristics. Cell division, apoptosis, tissue remodeling, inflammatory reactions, immunological control, vascular healing, neogenesis, and cell proliferation are some of its biological characteristics. Pathological diseases such as chronic sinusitis, inflammatory bowel disease, COPD, non-alcoholic fatty liver disease, and polymyositis have been linked to dysregulation of CHI3L1. (This protein structure is from The UniProt Knowledgebase (UniProtKB), Entry:P36222).
CHI3L1, a candidate gene for type 2 airway inflammation, may increase eosinophils, leading to a subsequent cascade of inflammation. In recent years, with many advances in genetic studies of CHI3L1 (45–47), CHI3L1 has been found to be associated with susceptibility to chronic sinusitis. CHI3L1 is a candidate gene for type 2 airway inflammation, which leads to an increase in eosinophils and triggers a cascade of inflammation. CHI3L1 gene polymorphisms regulate the expression of CHI3L1 in the airways, and the CHI3L1 protein is encoded by the chitinase 3-like 1 gene CHI3L1. Single-nucleotide polymorphisms (SNPs) in the CHI3L1 promoter are associated with elevated serum levels of CHI3L1 (32, 46). Single nucleotide polymorphisms (SNPs) affecting CHI3L1 levels also influence the progression of chronic sinusitis (48).
A comprehensive review of the literature has led to the conclusion that CHI3L1 is produced in a wide variety of cells, such as human synoviocytes (49), osteosarcoma cells (50), fibroblasts (51), endothelial cells (52), chondrocytes (53), smooth muscle cells (36), macrophages (54), neutrophils (55), and colonic epithelial cells (56). It was found that CHI3L1 has very important roles in both physiological and pathological states, which proves that CHI3L1 plays an important role in the study of health and disease (57) (Figure 2). CHI3L1 defends against infections and responds to oxidative induction and antigen presentation-induced damage. It coordinates tissue remodeling and repair, controls the release of inflammatory mediators, and plans the migration and aggregation of inflammatory cells. In particular, CHI3L1 regulates basic biological functions such as oxidative damage, apoptosis, cellular pyroptosis, activation of inflammatory vesicles, differentiation of M2 macrophages, aggregation of dendritic cells (DC), modulation of ECM (ECM), and creation of parenchymal scars (39, 58, 59). Additionally, CHI3L1 chemotactically affects smooth muscle and vascular endothelial cells. Through the promotion of vascular smooth muscle cell migration and the stimulation of endothelial tubule formation, its regulatory actions also extend to the regulation of vascular endothelial cell shape (60, 61).
Figure 2. CHI3L1’s function includes oxidative damage, apoptosis, cellular pyrolysis, activation of inflammatory vesicles, development of M2 macrophages, aggregation of dendritic cells (DC), modification of the ECM (ECM), and the production of parenchymal scars.
CHI3L1 also plays a significant function in the inflammatory response (Figure 3). According to research, CHI3L1 may have a role in maintaining a balance between pro- and anti-inflammatory signaling via regulating anti-inflammatory cytokines in physiological settings. Strong external stressors have the ability to enhance the expression of pro-inflammatory cytokines such tumor necrosis factor-α (TNF-α), interleukin 6 (IL-6), and interleukin 13 (IL-13) (62), which can activate numerous immune cells, including neutrophils, monocytes, and eosinophils, via mediating downstream signaling pathways implicated in inflammatory responses. Numerous inflammatory substances are released when these cells are activated by CHI3L1, disrupting the normal cellular barrier and causing a cascade of immunological responses that are dysregulated (59, 63, 64).
Figure 3. CHI3L1’s function in the inflammatory response. Pro-inflammatory cytokines including interleukin 6 (IL-6), interleukin 13 (IL-13), and tumor necrosis factor-alpha (TNF-alpha) are expressed more when CHI3L1 is present. This causes a number of immune cells, including neutrophils, monocytes, and eosinophils, to become activated.Via PI3K/Akt activation, CHI3L1 influences cell adhesion, migration, and the architecture of ECM proteins like collagen, fibronectin, and proteoglycans among other things. Furthermore, via influencing endothelial cell function and angiogenic signaling pathways, CHI3L1 facilitates vascular healing and neointima development.
In addition to immunomodulation, CHI3L1 has also been implicated in the regulation of ECM components. It was found that CHI3L1, in regulating the extracellular matrix (ECM), may transmit relevant signals through activation of the PI3K/Akt (41, 65) signaling pathway, inducing cells to secrete ECM proteins, including, i.e., collagens, non-collagenous proteins, elastin, proteoglycans, and aminoglycans, and that the cells continue to remodel the ECM through synthesis, degradation, and reorganization mechanisms in development, wound healing, and in certain disease states. The dynamics of the ECM are particularly well characterized (66). Furthermore, CHI3L1 stimulates fibroblast migration, activation, and proliferation inside the organism. The activation, proliferation, and release of active substances like fibrinogen by fibroblasts during CHI3L1 stimulation may stimulate the creation of ECM proteins, hence facilitating tissue remodeling and healing. Additionally, CHI3L1 may regulate the morphology of vascular endothelial cells from vascular repair to angiogenesis by promoting the migration of vascular smooth muscle cells, stimulating the formation of endothelial tubules by endothelial cells, and inducing the production of vascular endothelial growth factor (VEGF) signaling pathway (61). By triggering the particular signaling pathways listed above, CHI3L1 may cause cells to release pro-angiogenic molecules during tissue repair, thus encouraging angiogenesis. High levels of CHI3L1 expression are strongly associated with the severity and course of chronic inflammatory disorders, indicating a critical function for this protein in inflammation regulation.
The pathogenesis of CRS is currently the subject of much debate, but most believe (67, 68) that it is due to immune dysfunction, leading to a series of mechanisms that result in the formation of nasal polyps. Although a great deal of research has been conducted on CHI3L1 in immune disorders (69, 70), In particular, Chun Geun et al. (71)in their study verified the establishment of a novel regulatory role of CHI3L1 in the initiation and effector phases of Th2 inflammation and remodeling by in vitro modeling the production and characterization of CHI3L1/mice, CHI3L1 transgenic mice, and mice that lacked CHI3L1 and produced CHI3L1 only in the lung epithelium and revealed CHI3L1 to be a Th2 and therapeutic targets for macrophage-mediated diseases, and we know that chronic sinusitis is caused by dysregulated Th2 immunity. In the past, a large number of scholars have investigated the role of CHI3L1 in CRS. Wang et al. simulated immunoregulation in real human ECRS by creating an in vitro model of ECRS and verified that CHI3L1 is one of the major genes upregulated in patients with ECRS by single-cell sequencing (38). In addition, in nasal polyp tissue from patients with CRSwNP, CHI3L1 levels were found to be significantly higher than in patients with CRSsNP and with deviated septum alone, and it was revealed that epithelial cells are one of the major cells for CHI3L1 production (72). An in vitro model of CRS with eosinophilia was again created, and after treatment with anti-CHI3L1 antibody, it was found that eosinophils in the nasal mucosa of the in vitro model were greatly reduced, inflammatory mediators were significantly reduced, and there was a significant reduction in the distribution of cup cells as well as a decrease in mucus secretion, leading to a significant improvement in epithelial cell thickening.Moreover, in nasal polyps treated with anti-CHI3L1 antibody, we found that the expression of TNF-α and eotaxin - 1 was also significantly decreased, suggesting that CHI3L1 may activate signaling pathways through both TNF-α and eotaxin - 1 pathways, inducing eosinophil activation, migration, and release of inflammatory mediators, leading to the development of disease (73). Thus, CHI3L1 is highly associated with eosinophilic chronic sinusitis, and whether CHI3L1 also causes eosinophilia through other mechanisms in patients with chronic sinusitis and nasal polyps needs to be further investigated. Ma et al. isolated and cultured human nasal mucosal epithelial cells, stimulated them with different concentrations of IL-4, and found that CHI3L1 was substantially elevated in patients with chronic sinusitis with polyps, in patients with CRSsNP, and in patients with simple septal deviation, especially in those with CRSwNP. The expression of CHI3L1 was inhibited by other pro-inflammatory cytokines, and lipopolysaccharide (LPS) and dexamethasone caused a significant decrease in the expression of CHI3L1 in human nasal mucosal epithelial cells (72). Therefore, CHI3L1 knockout mice can be used to study the role of CHI3L1 in vivo. This will help to elucidate the specific role of CHI3L1 in the pathogenic process involved in ECRSwNP and to design new therapeutic strategies to manage these CRS patients.
In an animal model of chronic sinusitis, the expression pattern of CHI3L1 was consistent with observations in clinical samples. A significant increase in CHI3L1 expression in the nasal mucosa was found by inducing a mouse model of sinusitis. This increase was closely associated with the aggregation of inflammatory cells and the release of inflammatory mediators, further validating the key role of CHI3L1 in the inflammatory response. In addition, reducing CHI3L1 expression by gene knockout or pharmacological intervention significantly reduces inflammatory symptoms, By generating CC10-knockout purebred mice, Wang et al. established a mouse model of ECRS. It turned out that CC10-knockout ECRS mice demonstrated a marked increase in inflammatory cells and inflammatory mediators in sinus tissues in contrast with wild-type mice (38). The expression of CC10 in the nasal mucosa acts through different cytokines that regulate mRNA stability and the expression of certain transcription factors. In addition, CC10 can inhibit the expression and regulation of a variety of cytokines through certain mechanisms and can also inhibit the activity of phospholipase A2, thus attenuating the production and aggregation of inflammatory cells. Lee et al. (74)found by animal modeling that CC10 gene-deficient mice exhibited Th2-type inflammation and IL-13 effector responses by inducing eosinophil chemotaxis and aggregation, increasing IgE production, DC accumulation, and activation, as well as inhibiting inflammatory cell apoptosis and CD95 expression, and inducing PKB/AKT activation due to elevated levels of CHI3L1.It has been found that in human nasal polyps, CC10 gene expression is most significantly down-regulated (75), which leads to an increase in the expression of CHI3L1, inducing eosinophil chemotaxis and the formation of extracellular traps for eosinophils, which cleave and release a large number of cytokines and reactive substances, thus leading to CRS.It is suggested that CHI3L1 may be a potential target for the treatment of chronic sinusitis.
CHI3L1 (also known as YKL-40) is a glycoprotein secreted by a variety of cell types that plays an important role in CRS. Studies have shown that CHI3L1 expression is significantly increased in ECRS (72), suggesting an important role in eosinophil activation and migration. Eosinophils are key inflammatory cells in CRS, and their overactivation is strongly associated with disease severity and persistence of symptoms. CHI3L1 triggers a series of signalling pathways by binding to receptors on the surface of eosinophils, thereby promoting the activation and functional enhancement of these cells.
In He’s study, TNF-α was found to synergistically induce CHI3L1 mRNA expression with IL-13 or IL-4, and this effect was more pronounced when TNF-α was combined with IL-13 (34). CHI3L1 is able to bind to interleukin-13 receptor a2 (IL-13Ra2) on the surface of eosinophils and activate the PI3K/Akt and MAPK signalling pathways (76).Activation of these signalling pathways leads to enhanced degranulation of eosinophils and release of large amounts of inflammatory mediators such as histamine, leukotrienes and cytokines. This activation is particularly evident in eosinophil-associated diseases such as chronic sinusitis, suggesting a key role for CHI3L1 in disease progression. Further studies have shown that inhibition of CHI3L1 expression significantly reduces the activation status of eosinophils, thereby reducing inflammatory symptoms.
CHI3L1 not only affects the activation of eosinophils, but also significantly regulates their chemotaxis. CHI3L1 affects the expression and function of chemokines, a class of small molecule proteins that regulate cell migration, inflammation and immune response, through a variety of mechanisms.CHI3L1 can regulate chemokine signalling by interacting with other proteins in the cell. For example, CHI3L1 can bind to STAT3 (signal transducer and activator of transcription 3) and affect its phosphorylation and activation status, thereby regulating the expression of chemokine-related genes (77). This intracellular regulatory mechanism allows CHI3L1 to regulate chemokine function at a finer level. It has been shown that CHI3L1 is able to promote the release of chemokines from eosinophils by binding to receptors on their surface, such as CD44 and RAGE, thereby enhancing the migration of eosinophils towards inflammatory regions (65). This chemotaxis is particularly evident in eosinophil-associated diseases such as chronic sinusitis, suggesting a key role for CHI3L1 in disease progression. Further studies have shown that inhibition of CHI3L1 expression significantly reduces eosinophil chemotaxis, thereby attenuating inflammatory symptoms.
In recent years, targeted drugs against CHI3L1 have made significant progress in the treatment of various diseases (Table 1). Studies have shown significant achievements in targeted therapy of recurrent brain tumors (78), breast cancer (79), airway inflammatory diseases (80), and glioblastoma (81).
Based on the critical role of CHI3L1 in CRS, drug development targeting CHI3L1 has become a research direction of great interest. Several strategies have been proposed to inhibit the activity of CHI3L1, including monoclonal antibodies, small molecule inhibitors, and gene therapy. Monoclonal antibodies act directly on the CHI3L1 protein to inhibit the inflammatory response by blocking its binding to the receptor. Small molecule inhibitors, on the other hand, achieve therapeutic effects by interfering with the synthesis or secretion of CHI3L1. Gene therapy, on the other hand, aims to radically reduce the role of CHI3L1 in the inflammatory process by modulating its expression level.
Although the development of drugs targeting CHI3L1 is still in the early stages, initial preclinical studies have shown some potential. For example, certain monoclonal antibodies significantly reduced the inflammatory response of the sinus mucosa and improved associated symptoms in animal models. However, the safety and efficacy of these drugs still need to be validated in further clinical trials. In addition, how to choose the appropriate route and dose of administration and how to evaluate the therapeutic effect are also issues that need to be addressed in future studies. Overall, drug development targeting CHI3L1 offers new hope for the treatment of chronic sinusitis. With further research, it is expected that safer and more effective therapeutic regimens will be developed in the future, thus improving the clinical prognosis and quality of life of patients.
CHI3L1 is a protein associated with a variety of diseases, and its use as a biomarker has gradually increased in recent years (Table 2). CHI3L1 expression levels are significantly elevated in a variety of inflammatory diseases, tumors, and neurodegenerative disorders, making it a potential biomarker. Examples include polymyositis/dermatomyositis-associated interstitial lung disease (82), non-alcoholic fatty liver disease (NAFLD) (83), inflammatory bowel disease (IBD) in children (84) and adults (85), endothelial dysfunction and hypertension in patients with obstructive sleep apnea (86), and diabetes (87).
And in the study of CRS, CHI3L1 has also been considered as a possible potential biomarker. CRS is a common upper respiratory disease characterised by chronic inflammation of the sinus mucosa, often accompanied by nasal congestion and purulent discharge. Due to its complex pathological process involving the interaction of multiple immune cells and inflammatory mediators, the search for effective biomarkers is important for the diagnosis and treatment of the disease. Studies have shown that CHI3L1 expression is significantly elevated in nasal secretions and tissues of patients with chronic sinusitis. This high expression was closely related to the severity of the disease and the degree of inflammatory activity.CHI3L1 is not only involved in the regulation of inflammatory responses, but may also affect the structure and function of sinus mucosa by promoting fibrosis and tissue remodelling. In addition, high CHI3L1 expression was associated with eosinophil infiltration, which is an important pathological feature in chronic sinusitis.
Therefore, CHI3L1 not only plays an important role in the pathological process of CRS, but may also be used as a potential biomarker for early diagnosis of the disease, assessment of the condition and monitoring of treatment effects. Future studies should further validate the expression differences of CHI3L1 in different types of CRS and explore its feasibility and accuracy in clinical applications.
This review methodically investigated the various ways that CHI3L1 contributes to eosinophilic inflammation in CRS, highlighting its crucial functions in immune response mediation, eosinophil function regulation, and possible therapeutic uses. In addition to expanding our knowledge of CHI3L1’s role in the pathophysiology of CRS, these investigations offer a crucial theoretical foundation for upcoming clinical research and treatment approaches. Advances in the study of CHI3L1 represent the in-depth analysis of the illness mechanism by modern medicine at the molecular level. There are still some differences and disagreements in the findings from various studies, despite the fact that a significant number of investigations have been carried out to demonstrate the critical role of CHI3L1 in CRS. For instance, some research highlight CHI3L1’s pro-inflammatory function, while others suggest that it may have anti-inflammatory properties. The variety of experimental designs, sample sources, and disease stages may be the cause of these discrepancies. Therefore, using bigger sample numbers and multicenter cooperation, future research must further validate and improve the mechanism of action of CHI3L1. The evolution of treatment approaches connected to CHI3L1 will be influenced by how these various research viewpoints are balanced and integrated. For instance, CHI3L1 expression can be more accurately controlled by combining gene editing technology with bioinformatics analysis, allowing for more individualized therapy of chronic sinusitis. Furthermore, as CHI3L1 is extensively expressed in many inflammatory disorders, its discovery in chronic sinusitis may also lead to novel treatment approaches for other associated conditions. Thus, research on CHI3L1 offers a wide range of opportunities for interdisciplinary collaboration and the creation of novel treatment approaches in addition to its significant clinical relevance.
LG: Writing – original draft, Writing – review & editing. YP: Writing – original draft. CY: Writing – original draft. XL: Writing – original draft. WX: Writing – original draft. WL: Writing – original draft. JF: Supervision, Writing – original draft.
The author(s) declare that financial support was received for the research and/or publication of this article. The National Natural Science Foundation of China(82471181), and the Sichuan Provincial Cadre Health Care Research Project (No.2024-219).
The authors declare that the research was conducted in the absence of any commercial or financial relationships that could be construed as a potential conflict of interest.
The author(s) declare that no Generative AI was used in the creation of this manuscript.
All claims expressed in this article are solely those of the authors and do not necessarily represent those of their affiliated organizations, or those of the publisher, the editors and the reviewers. Any product that may be evaluated in this article, or claim that may be made by its manufacturer, is not guaranteed or endorsed by the publisher.
1. Hastan D, Fokkens W, Bachert C, Tomassen P, Newson R, Jarvis D, et al. Chronic rhinosinusitis in Europe - an underestimated disease. A GA2LEN study. Allergy. (2011) 66:128–8. doi: 10.1111/j.1398-9995.2011.02646.x
2. Jarvis D, Newson R, Lotvall J, Hastan D, Tomassen P, Keil T, et al. Asthma in adults and its association with chronic rhinosinusitis: The GA2LEN survey in Europe. Allergy. (2012) 67:91–8. doi: 10.1111/j.1398-9995.2011.02709.x
3. Kurbacheva O, Kozlov V, Solovkina Y, Ostankova O, Bulatov V. Real-world data on Chronic Rhinosinusitis with Nasal Polyps in Russia from open internet sources assessed by artificial intelligence. Allergy. (2020) 75:494–5.
4. Yang QT, Sun YQ, Wu QW, Shi JB. Interpretation of 2020 European position paper on rhinosinusitis and nasal polyps. Zhonghua er bi yan hou tou jing wai ke za zhi = Chin J Otorhinolaryngol Head Neck Surg. (2020) 55:304–8. doi: 10.3760/cma.j.issn.1673-0860.2020.03.024
5. Kim JH, Cho C, Lee EJ, Suh YS, Choi BI, Kim KS. Prevalence and risk factors of chronic rhinosinusitis in South Korea according to diagnostic criteria. Rhinology. (2016) 54:329–35. doi: 10.4193/Rhino15.157
6. Tomassen P, Newson RB, Hoffmans R, Lötvall J, Cardell LO, Gunnbjörnsdóttir M, et al. Reliability of EP3OS symptom criteria and nasal endoscopy in the assessment of chronic rhinosinusitis - a GA2LEN study. Allergy. (2011) 66:556–61. doi: 10.1111/j.1398-9995.2010.02503.x
7. de Loos DD, Lourijsen ES, Wildeman MAM, Freling NJM, Wolvers MDJ, Reitsma S, et al. Prevalence of chronic rhinosinusitis in the general population based on sinus radiology and symptomatology. J Allergy Clin Immunol. (2019) 143:1207–14. doi: 10.1016/j.jaci.2018.12.986
8. Hirsch AG, Nordberg C, Bandeen-Roche K, Tan BK, Schleimer RP, Kern RC, et al. Radiologic sinus inflammation and symptoms of chronic rhinosinusitis in a population-based sample. Allergy. (2020) 75:911–20. doi: 10.1111/all.14106
9. Khan A, Huynh TMT, Vandeplas G, Joish VN, Mannent LP, Tomassen P, et al. The GALEN rhinosinusitis cohort: chronic rhinosinusitis with nasal polyps affects health-related quality of life. Rhinology. (2019) 57:343–51. doi: 10.4193/Rhin19.158
10. Munari S, Ciotti G, Cestaro W, Corsi L, Tonin S, Ballarin A, et al. Severe hypereosinophilia in a patient treated with dupilumab and shift to mepolizumab: the importance of multidisciplinary management. A case report and literature review. Drugs Context. (2024) 13. doi: 10.7573/dic.2024-3-5
11. Han JK, Bachert C, Fokkens W, Desrosiers M, Wagenmann M, Lee SE, et al. Mepolizumab for chronic rhinosinusitis with nasal polyps (SYNAPSE): a randomised, double-blind, placebo-controlled, phase 3 trial. Lancet Respir Med. (2021) 9:1141–53. doi: 10.1016/S2213-2600(21)00097-7
12. Gevaert P, Saenz R, Corren J, Han JK, Mullol J, Lee SE, et al. Long-term efficacy and safety of omalizumab for nasal polyposis in an open-label extension study. J Allergy Clin Immunol. (2022) 149:957–+. doi: 10.1016/j.jaci.2021.07.045
13. Kohli P, Naik AN, Harruff EE, Nguyen SA, Schlosser RJ, Soler ZM. The prevalence of olfactory dysfunction in chronic rhinosinusitis. Laryngoscope. (2017) 127:309–20. doi: 10.1002/lary.v127.2
14. Soler ZM, Schlosser RJ, Bodner TE, Alt JA, Ramakrishnan VR, Mattos JL, et al. Endotyping chronic rhinosinusitis based on olfactory cleft mucus biomarkers. J Allergy Clin Immunol. (2021) 147:1732–+. doi: 10.1016/j.jaci.2021.01.021
15. Stevens WW, Peters AT, Tan BK, Klingler AI, Poposki JA, Hulse KE, et al. Associations between inflammatory endotypes and clinical presentations in chronic rhinosinusitis. J Allergy Clin Immunol-in Pract. (2019) 7:2812–+. doi: 10.1016/j.jaip.2019.05.009
16. Klingler AI, Stevens WW, Tan BK, Peters AT, Poposki JA, Grammer LC, et al. Mechanisms and biomarkers of inflammatory endotypes in chronic rhinosinusitis without nasal polyps. J Allergy Clin Immunol. (2021) 147:1306–17. doi: 10.1016/j.jaci.2020.11.037
17. Lin YT, Lin CF, Liao CK, Chiang BL, Yeh TH. Clinical characteristics and cytokine profiles of central-compartment-type chronic rhinosinusitis. Int Forum Allergy Rhinol. (2021) 11:1064–73. doi: 10.1002/alr.22759
18. Bachert C, Gevaert P, Hellings P. Biotherapeutics in chronic rhinosinusitis with and without nasal polyps. J Allergy Clin Immunol-in Pract. (2017) 5:1512–6. doi: 10.1016/j.jaip.2017.04.024
19. Tokunaga T, Sakashita M, Haruna T, Asaka D, Takeno S, Ikeda H, et al. Novel scoring system and algorithm for classifying chronic rhinosinusitis: the JESREC Study. Allergy. (2015) 70:995–1003. doi: 10.1111/all.2015.70.issue-8
20. Katotomichelakis M, Tantilipikorn P, Holtappels G, De Ruyck N, Feng L, Van Zele T, et al. Inflammatory patterns in upper airway disease in the same geographical area may change over time. Am J Rhinol Allergy. (2013) 27:354–60. doi: 10.2500/ajra.2013.27.3922
21. Min HK, Lee S, Kim S, Son Y, Park J, Kim HJ, et al. Global incidence and prevalence of chronic rhinosinusitis: A systematic review. Clin Exp Allergy. (2024) 55(1):52–66. doi: 10.1111/cea.14592
22. Chitsuthipakorn W, Seresirikachorn K, Sommer DD, McHugh T, Snidvongs K. Endotypes of chronic rhinosinusitis across ancestry and geographic regions. Curr Allergy Asthma Rep. (2018) 18:15. doi: 10.1007/s11882-018-0800-z
23. Tsuda T, Maeda Y, Nishide M, Koyama S, Hayama Y, Nojima S, et al. Eosinophil-derived neurotoxin enhances airway remodeling in eosinophilic chronic rhinosinusitis and correlates with disease severity. Int Immunol. (2019) 31:33–40. doi: 10.1093/intimm/dxy061
24. Salter B, Zhao N, Gheva-Dvorkin A, Radford K, Lavigne N, Huang C, et al. Clinical indices of autoimmunity do not predict sputum autoantibodies in patients with severe eosinophilic asthma: A prospective study. Am J Respir Crit Care Med. (2021) 203:2. doi: 10.1164/ajrccm-conference.2021.TP6
25. Liang ZY, Wang FY, Zhang DY, Long F, Yang YQ, Gu WL, et al. Sputum and serum autoantibody profiles and their clinical correlation patterns in COPD patients with and without eosinophilic airway inflammation. J Thorac Dis. (2020) 12:3085–100. doi: 10.21037/jtd-20-545
26. Siddiqui S, Bachert C, Bjermer L, Buchheit KM, Castro M, Qin YM, et al. Eosinophils and tissue remodeling: Relevance to airway disease. J Allergy Clin Immunol. (2023) 152:841–57. doi: 10.1016/j.jaci.2023.06.005
27. Vukadinovic T, Durdevic BV, Peric A. Expression of major basic protein and endothelial adhesion molecules in chronically inflamed mucosa of the ethmoid labyrinth. Immun Inflammation Dis. (2024) 12:7. doi: 10.1002/iid3.v12.12
28. Xu XD, Tao L, Liu JM, Jing Z, Bing Z, Du JT, et al. Crocin inhibits the type 2 inflammatory response produced by ILC2s in eosinophilic nasal polyps. Am J Rhinol Allergy. (2023) 37:656–69. doi: 10.1177/19458924231185296
29. Takabayashi T, Kato A, Peters AT, Hulse KE, Suh LA, Carter R, et al. Excessive fibrin deposition in nasal polyps caused by fibrinolytic impairment through reduction of tissue plasminogen activator expression. Am J Respir Crit Care Med. (2013) 187:49–57. doi: 10.1164/rccm.201207-1292OC
30. Takabayashi T, Schleimer RP. Formation of nasal polyps: The roles of innate type 2 inflammation and deposition of fibrin. J Allergy Clin Immunol. (2020) 145:740–50. doi: 10.1016/j.jaci.2020.01.027
31. Chupp GL, Lee CG, Jarjour N, Shim YM, Holm CT, He S, et al. A chitinase-like protein in the lung and circulation of patients with severe asthma. New Engl J Med. (2007) 357:2016–27. doi: 10.1056/NEJMoa073600
32. Ober C, Tan Z, Sun Y, Possick JD, Pan L, Nicolae R, et al. Effect of variation in CHI3L1 on serum YKL-40 level, risk of asthma, and lung function. New Engl J Med. (2008) 358:1682–91. doi: 10.1056/NEJMoa0708801
33. Zhu Z, Zheng T, Homer RJ, Kim YK, Chen NY, Cohn L, et al. Acidic mammalian chitinase in asthmatic Th2 inflammation and IL-13 pathway activation. Science. (2004) 304:1678–82. doi: 10.1126/science.1095336
34. He CH, Lee CG, Dela Cruz CS, Lee CM, Zhou Y, Ahangari F, et al. Chitinase 3-like 1 Regulates Cellular and Tissue Responses via IL-13 Receptor α2 (vol 4, pg 830, 2013). Cell Rep. (2015) 10:1433–3. doi: 10.1016/j.celrep.2015.02.017
35. Imoto Y, Kato A, Takabayashi T, Stevens W, Norton JE, Suh LA, et al. Increased thrombin-activatable fibrinolysis inhibitor levels in patients with chronic rhinosinusitis with nasal polyps. J Allergy Clin Immunol. (2019) 144:1566–+. doi: 10.1016/j.jaci.2019.08.040
36. Shackelton LM, Mann DM, Millis AJT. Identification of a 38-KDA heparin-binding glycoprotein (gp38k) in differentiating vascular smooth-muscle cells as a member of a group of proteins associated with tissue remodeling. J Biol Chem. (1995) 270:13076–83. doi: 10.1074/jbc.270.22.13076
37. Kanneganti M, Kamba A, Mizoguchi E. Role of chitotriosidase (chitinase 1) under normal and disease conditions. J Epithelial Biol Pharmacol. (2012) 5:1–9. doi: 10.2174/1875044301205010001
38. Wang H, Long XB, Cao PP, Wang N, Liu Y, Cui YH, et al. Clara cell 10-kD protein suppresses chitinase 3-like 1 expression associated with eosinophilic chronic rhinosinusitis. Am J Respir Crit Care Med. (2010) 181:908–16. doi: 10.1164/rccm.200904-0597OC
39. Coffman F. Chitinase 3-like-1 (CHI3L1): A putative disease marker at the interface of proteomics and glycomics. Crit Rev Clin Lab Sci. (2008) 45:531–62. doi: 10.1080/10408360802334743
40. Jiang L, Xu D, Zhang WJ, Tang Y, Peng Y. Astrocytes induce proliferation of oligodendrocyte progenitor cells via connexin 47-mediated activation of Chi3l1 expression. Eur Rev Med Pharmacol Sci. (2019) 23:3012–20. doi: 10.26355/eurrev_201904_17583
41. Lee CM, He CH, Nour AM, Zhou Y, Ma B, Park JW, et al. IL-13Rα2 uses TMEM219 in chitinase 3-like-1-induced signalling and effector responses (vol 7, 12752, 2016). Nat Commun. (2016) 7:13541 doi: 10.1038/ncomms13541
42. Mohanty AK, Choudhary S, Kaushik JK, Fisher AJ. Crystal structure of breast regression protein 39 (BRP39), a signaling glycoprotein expressed during mammary gland apoptosis, at 2.6 Å resolution. J Struct Biol. (2021) 213:10. doi: 10.1016/j.jsb.2021.107737
43. Fusetti F, Pijning T, Kalk KH, Bos E, Dijkstra BW. Crystal structure and carbohydrate-binding properties of the human cartilage glycoprotein-39. J Biol Chem. (2003) 278:37753–60. doi: 10.1074/jbc.M303137200
44. Suzuki K, Okawa K, Ohkura M, Kanaizumi T, Kobayashi T, Takahashi K, et al. Evolutionary insights into sequence modifications governing chitin recognition and chitinase inactivity in YKL-40 (HC-gp39, CHI3L1). J Biol Chem. (2024) 300:12. doi: 10.1016/j.jbc.2024.107365
45. Huang QP, Xie ZF, Huang J. Assessment of the association between genetic polymorphisms in the CHI3L1 gene and asthma risk. Int Arch Allergy Immunol. (2022) 183:907–18. doi: 10.1159/000522393
46. Kruit A, Grutters JC, Ruven HJT, van Moorse C.CM, van den Bosch JMM. A CHI3L1 gene polymorphism is associated with serum levels of YKL-40, a novel sarcoidosis marker. Respir Med. (2007) 101:1563–71. doi: 10.1016/j.rmed.2006.12.006
47. Zhu YT, Yan X, Zhai C, Yang L, Li MX. Association between risk of asthma and gene polymorphisms in CHI3L1 and CHIA: a systematic meta-analysis. BMC Pulmonary Med. (2017) 17:10. doi: 10.1186/s12890-017-0515-2
48. Zhao XZ, Tang RQ, Gao B, Shi Y. Y, Zhou J, Guo SZ, et al. Functional variants in the promoter region of chitinase 3-like 1 (CHI3L1) and susceptibility to schizophrenia. Am J Hum Genet. (2007) 80:12–8. doi: 10.1086/510438
49. Nyirkos P, Golds EE. Human synovial cells secrete a 39 kDa protein similar to a bovine mammary protein expressed during the non-lactating period. Biochem J. (1990) 269:265–8. doi: 10.1042/bj2690265
50. Johansen JS, Williamson MK, Rice JS, Price PA. Identification of proteins secreted by human osteoblastic cells in culture. J Bone Mineral Res. (1992) 7:501–12. doi: 10.1002/jbmr.5650070506
51. Wang X, Ye T, Huang J, Hu F, Huang C, Gu B, et al. Aberrant chitinase 3-like 1 expression in basal cells contributes to systemic sclerosis fibrosis. Advanced Sci. (2024) 12(6):e2310169. doi: 10.1002/advs.202310169
52. Sun XN, Nakajima E, Norbrun C, Sorkhdini P, Yang AX, Yang DQ, et al. Chitinase 3 like 1 contributes to the development of pulmonary vascular remodeling in pulmonary hypertension. JCI Insight. (2022) 7:22. doi: 10.1172/jci.insight.159578
53. Hakala BE, White C, Recklies AD. Human cartilage gp-39, a major secretory product of articular chondrocytes and synovial-cells, is a mammalian member of a chitinase protein family. J Biol Chem. (1993) 268:25803–10. doi: 10.1016/S0021-9258(19)74461-5
54. Renkema GH, Boot RG, Au FL, Donker-Koopman WE, Strijland A, Muijsers AO, et al. Chitotriosidase, a chitinase, and the 39-kDa human cartilage glycoprotein, a chitin-binding lectin, are homologues of family 18 glycosyl hydrolases secreted by human macrophages. Eur J Biochem. (1998) 251:504–9. doi: 10.1046/j.1432-1327.1998.2510504.x
55. Volck B, Price PA, Johansen JS, Sorensen O, Benfield TL, Nielsen HJ, et al. YKL-40, a mammalian member of the chitinase family, is a matrix protein of specific granules in human neutrophils. Proc Assoc Am Physicians. (1998) 110:351–60.
56. Hachiya Y, Sugimoto K, Mizoguchi E. Chitinase 3-like-1 (Chi3L1/YKL-40/HC gp-39) exacerbates intestinal inflammation by enhancing bacterial adhesion and internalization in colonic epithelial cells. Gastroenterology. (2005) 128:A5.
57. Nishikawa KC, Millis AJT. gp38k (CHI3L1) is a novel adhesion and migration factor for vascular cells. Exp Cell Res. (2003) 287:79–87. doi: 10.1016/S0014-4827(03)00069-7
58. Zimmerman SG, Merrihew GE, MacCoss MJ, Berg CA. Proteomics analysis identifies orthologs of human chitinase-like proteins as inducers of tube morphogenesis defects in Drosophila melanogaster. Genetics. (2017) 206:973–84. doi: 10.1534/genetics.116.199323
59. Johansen JS. Studies on serum YKL-40 as a biomarker in diseases with inflammation, tissue remodelling, fibroses and cancer. Danish Med Bull. (2006) 53:172–209.
60. Francescone R, Ngernyuang N, Yan W, Bentley B, Shao R. Tumor-derived mural-like cells coordinate with endothelial cells: role of YKL-40 in mural cell-mediated angiogenesis. Oncogene. (2014) 33:2110–22. doi: 10.1038/onc.2013.160
61. Norbrun C, Sun X, Nakajima E, Sorkhdini P, Yang A, Yang D, et al. Chitinase 3-like-1 protein contributes to the development of pulmonary vascular remodeling in pulmonary hypertension. Am J Respir Crit Care Med. (2023) 207:2. doi: 10.1164/ajrccm-conference.2023.C26
62. Ge LL, Wang YX, Liu Z, Du H, Zhao DC. Chitinase 3-like 1 plays a pivotal role in airway response of RSV infection via regulating DC functional transition. Int Immunopharmacol. (2023) 124:11. doi: 10.1016/j.intimp.2023.110819
63. Rathcke CN, Vestergaard H. YKL-40-an emerging biomarker in cardiovascular disease and diabetes. Cardiovasc Diabetol. (2009) 8:7. doi: 10.1186/1475-2840-8-61
64. Wang YG, Lin C, Huang M, Fang XL, Chen GH, Ye SN. Overexpression of YKL40,IL-6, IL-8, TNF-α in tonsils and the role of YKL40 in childhood with obstructive sleep apnea syndrome. Sci Rep. (2024) 14:11. doi: 10.1038/s41598-024-74402-8
65. Geng B, Pan JS, Zhao T, Ji J, Zhang C, Che Y, et al. Chitinase 3-like 1-CD44 interaction promotes metastasis and epithelial-to-mesenchymal transition through β-catenin/Erk/Akt signaling in gastric cancer. J Exp Clin Cancer Res. (2018) 37:20. doi: 10.1186/s13046-018-0876-2
66. Choi D, Bakhtiari M, Pilcher W, Huang C, Thomas BE, Mumme H, et al. Single-cell analysis of debrided diabetic foot ulcers reveals dysregulated wound healing environment in non-hispanic blacks. J Invest Dermatol. (2024) 145(3):678–90. doi: 10.1016/j.jid.2024.07.017
67. Stevens WW, Staudacher AG, Hulse KE, Poposki JA, Kato A, Carter RG, et al. Studies of the role of basophils in aspirin-exacerbated respiratory disease pathogenesis. J Allergy Clin Immunol. (2021) 148:439–+. doi: 10.1016/j.jaci.2021.02.045
68. Pezato R, Swierczynska-Krepa M, Niankowska-Mogilnicka E, Holtappels G, De Ruyck N, Sanak M, et al. Systemic expression of inflammatory mediators in patients with chronic rhinosinusitis and nasal polyps with and without Aspirin Exacerbated Respiratory Disease. Cytokine. (2016) 77:157–67. doi: 10.1016/j.cyto.2015.10.011
69. Blok KM, Kranenbarg R, Ananth K, Engelenburg H.J, van den Bosch A, Giannini LAA, et al. Multifaceted biomarkers suggest a similar profile of CNS pathology in relapsing and progressive MS. Eur J Neurol. (2025) 32:11. doi: 10.1111/ene.70052
70. Mwale PF, Hsieh CT, Yen TL, Jan JS, Taliyan R, Yang CH, et al. Chitinase-3-like-1: a multifaceted player in neuroinflammation and degenerative pathologies with therapeutic implications. Mol Neurodegeneration. (2025) 20:26. doi: 10.1186/s13024-025-00801-8
71. Lee CG, Hartl D, Lee GR, Koller B, Matsuura H, Da Silva CA, et al. Role of breast regression protein 39 (BRP-39)/chitinase 3-like-1 in Th2 and IL-13-induced tissue responses and apoptosis. J Exp Med. (2009) 206:1149–66. doi: 10.1084/jem.20081271
72. Ma Y, Zheng CQ, Shi L. The role of YKL40 in the pathogenesis of CRS with nasal polyps. Eur Arch Oto-Rhino-Laryngol. (2018) 275:431–8. doi: 10.1007/s00405-017-4859-2
73. Ye MX, Ji F, Huang C, Li F, Zhang CL, Zhang Y, et al. A novel probiotic formula, BLLL, ameliorates chronic stress-induced depression-like behaviors in mice by reducing neuroinflammation and increasing neurotrophic factors. Front Pharmacol. (2024) 15:15. doi: 10.3389/fphar.2024.1398292
74. Lee CG, Da Silva CA, Dela Cruz CS, Ahangari F, Ma B, Kang MJ, et al. Role of chitin and chitinase/chitinase-like proteins in inflammation, tissue remodeling, and injury. In: Julius D, Clapham DE, editors. Annual Review of Physiology, vol. 73. Palo Alto: Annual Reviews (2011). p. 479–501.
75. Liu Z, Kim J, Sypek JP, Wang IM, Horton H, Oppenheim FG, et al. Gene expression profiles in human nasal polyp tissues studied by means of DNA microarray. J Allergy Clin Immunol. (2004) 114:783–90. doi: 10.1016/j.jaci.2004.04.052
76. Mi L, Jin J, Zhang YY, Chen M, Cui JL, Chen R, et al. Chitinase 3-like 1 overexpression aggravates hypoxia-reoxygenation injury in IEC-6 cells by inhibiting the PI3K/AKT signalling pathway. Exp Physiol. (2024) 109:2073–87. doi: 10.1113/eph.v109.12
77. Jones LM, Broz ML, Ranger JJ, Ozcelik J, Ahn R, Zuo DM, et al. STAT3 establishes an immunosuppressive microenvironment during the early stages of breast carcinogenesis to promote tumor growth and metastasis. Cancer Res. (2016) 76:1416–28. doi: 10.1158/0008-5472.CAN-15-2770
78. Miyata H, Ashizawa T, Iizuka A, Kondou R, Nonomura C, Sugino T, et al. Combination of a STAT3 inhibitor and an mTOR inhibitor against a temozolomide-resistant glioblastoma cell line. Cancer Genomics Proteomics. (2017) 14:83–91. doi: 10.21873/cgp.20021
79. Ngernyuang N, Yan W, Schwartz LM, Oh D, Liu YB, Chen HZ, et al. A heparin binding motif rich in arginine and lysine is the functional domain of YKL-40. Neoplasia. (2018) 20:182–92. doi: 10.1016/j.neo.2017.11.011
80. Kim MJ, Shim DH, Cha HR, Moon KY, Yang CM, Hwang SJ, et al. Chitinase 3-like 1 protein plays a critical role in respiratory syncytial virus-induced airway inflammation. Allergy. (2019) 74:685–97. doi: 10.1111/all.2019.74.issue-4
81. Francescone R, Faibish M, Bentley B, Yan W, Shao R. A YKL-40-neutralizing antibody blocks tumor angiogenesis and progression: A potential therapeutic agent in cancers. Cancer Res. (2012) 72:1. doi: 10.1158/1538-7445.AM2012-4620
82. Hozumi H, Fujisawa T, Enomoto N, Nakashima R, Enomoto Y, Suzuki Y, et al. Clinical utility of YKL-40 in polymyositis/dermatomyositis-associated interstitial lung disease. J Rheumatol. (2017) 44:1394–401. doi: 10.3899/jrheum.170373
83. Kumagai E, Mano Y, Yoshio S, Shoji H, Sugiyama M, Korenaga M, et al. Serum YKL-40 as a marker of liver fibrosis in patients with non-alcoholic fatty liver disease. Sci Rep. (2016) 6:10. doi: 10.1038/srep35282
84. Aomatsu T, Imaeda H, Matsumoto K, Kimura E, Yoden A, Tamai H, et al. Faecal chitinase 3-like-1: a novel biomarker of disease activity in paediatric inflammatory bowel disease. Alimentary Pharmacol Ther. (2011) 34:941–8. doi: 10.1111/j.1365-2036.2011.04805.x
85. Buisson A, Vazeille E, Quinard RM, Goutte M, Bouvier D, Goutorbe F, et al. Faecal chitinase 3-like 1 is a reliable marker as accurate as faecal calprotectin in detecting endoscopic activity in adult patients with inflammatory bowel diseases. J Crohns Colitis. (2016) 10:S85–6. doi: 10.1111/apt.2016.43.issue-10
86. Jafari B, Mohsenin V. Chitinase-3-like protein-1 (YKL-40) as a marker of endothelial dysfunction in obstructive sleep apnea. Sleep Med. (2016) 25:87–92. doi: 10.1016/j.sleep.2016.08.001
87. Aguilera E, Serra-Planas E, Granada ML, Pellitero S, Reverter JL, Alonso N, et al. Relationship of YKL-40 and adiponectin and subclinical atherosclerosis in asymptomatic patients with type 1 diabetes mellitus from a European Mediterranean population. Cardiovasc Diabetol. (2015) 14:7. doi: 10.1186/s12933-015-0287-z
Keywords: CHI3L1, chronic sinusitis, eosinophils, inflammatory mechanisms, immunomodulation
Citation: Guo L, Peng Y, Yang C, Liu X, Xiong W, Liao W and Fan J (2025) Mechanistic studies on the role of CHI3L1 in eosinophilic inflammation in chronic sinusitis. Front. Immunol. 16:1562546. doi: 10.3389/fimmu.2025.1562546
Received: 17 January 2025; Accepted: 05 March 2025;
Published: 25 March 2025.
Edited by:
Susetta Finotto, Universitätsklinikum Erlangen, GermanyReviewed by:
Masanori Fujii, Meijo University, JapanCopyright © 2025 Guo, Peng, Yang, Liu, Xiong, Liao and Fan. This is an open-access article distributed under the terms of the Creative Commons Attribution License (CC BY). The use, distribution or reproduction in other forums is permitted, provided the original author(s) and the copyright owner(s) are credited and that the original publication in this journal is cited, in accordance with accepted academic practice. No use, distribution or reproduction is permitted which does not comply with these terms.
*Correspondence: Jiangang Fan, ZW50c2NmamdAMTYzLmNvbQ==
Disclaimer: All claims expressed in this article are solely those of the authors and do not necessarily represent those of their affiliated organizations, or those of the publisher, the editors and the reviewers. Any product that may be evaluated in this article or claim that may be made by its manufacturer is not guaranteed or endorsed by the publisher.
Research integrity at Frontiers
Learn more about the work of our research integrity team to safeguard the quality of each article we publish.