- 1State Key Laboratory of Oncology in South China, Guangdong Key Laboratory of Nasopharyngeal Carcinoma Diagnosis and Therapy, Guangdong Provincial Clinical Research Center for Cancer, Sun Yat-sen University Cancer Center, Guangzhou, China
- 2Department of Radiation Oncology, Sun Yat-sen University Cancer Center, Guangzhou, China
- 3Department of Radiology, Sun Yat-sen University Cancer Center, Guangzhou, China
- 4Department of Pathology, Sun Yat-sen University Cancer Center, Guangzhou, China
- 5Department of Oncology, The Second Affiliated Hospital of Hengyang Medical School, Hengyang, Hunan, China
Purpose: Platinum-based chemotherapy plus PD-1 inhibitors (chemoimmunotherapy) was the standard systemic treatment for recurrent or metastatic nasopharyngeal carcinoma (R/M NPC). However, biomarkers to predict the survival outcomes remained unsatisfying. This study aimed to establish a simple but easily applicable model to predict the survival outcomes of R/M NPC receiving chemoimmunotherapy.
Materials and methods: A total of 319 R/M NPC patients treated by chemoimmunotherapy with or without local therapy at our hospital were randomly divided into training (n=223) and validation (n=96) cohorts at a ratio of 7:3. An easily applicable prognostic risk grouping model was created using common independent predictors of progression-free survival (PFS) and overall survival (OS) in the training set. Model performance was assessed in the validation set.
Results: Pretreatment IL-6 and EBV DNA levels were identified as independent prognostic factors (scored on 0-4 points), and used to develop a prognostic risk grouping model with distinct survivals: 0-1 point (low risk), 2-3 points (intermediate risk), and 4 points (high risk). In the training set, the median PFS were not reached (NR), 18.90, and 7.73 months (P<0.001) respectively in the low-, intermediate-, and high-risk groups, while the median OS were NR, NR and 13.6 months (P<0.001). Results were further confirmed in the validation set.
Conclusion: This model predicted both PFS and OS in R/M NPC patients undergoing chemoimmunotherapy. This finding may help clinicians with an initial prognostic estimation but warrants further prospective investigation for the value of IL-6 and EBV DNA.
Introduction
Nasopharyngeal carcinoma (NPC), a malignancy originating from the nasopharynx epithelium, is endemic in southern China and Southeast Asia (1). Platinum-based concurrent chemoradiotherapy (CRT) has significantly improved survival outcomes for primary non-metastatic NPC (2). However, 5%-15% of NPC patients still experienced locoregional failure, and 15%-30% developed distant metastases after definitive chemoradiotherapy (3, 4). Furthermore, 4-10% presented with metastatic disease at the time of diagnosis (1, 3, 5). Currently, programmed death receptor antagonist 1 (PD-1) monoclonal antibody (PD-1 mAb), combined with cisplatin-based doublet or triplet chemotherapy regimens, have become the standard first-line treatment for recurrent or metastatic nasopharyngeal carcinoma (R/M NPC) (6–12). Despite the efficacy, the median progression-free survival (PFS) of patients treated with chemoimmunotherapy ranged from 9.6-21.4 months (7, 9, 12). This variability highlights the urgent need for reliable biomarkers or predictive indicators to identify patients who are most likely to benefit from chemoimmunotherapy.
Although nonkeratinizing NPC is characterized by a rich lymphocytic infiltration and high programmed death ligand 1 (PD-L1) expression in tumor tissue, the expression levels of PD-1/PD-L1 was limited to predict the efficacy of immunotherapy (13–17). Other ICIs-associated markers, such as microsatellite instability (MSI) and tumor mutational burden (TMB), were also difficult to identify patients who could benefit from immunotherapy (18). Genetic mutation analyses have identified several altered genes, including TP53, CDKN2A, and CDKN2B, but none have demonstrated sufficient predictive value for clinical efficacy (18, 19). Similarly, the density of MHC-II+ cell remains inadequate as a predictive factor (20). Inflammatory factors, like C-response protein (CRP), white blood cells (WBC), and neutrophils (NE) in the tumor or immune microenvironment, were demonstrated to play an important role in promoting tumor progression (19, 21, 22), but they were susceptible to interfering with treatment (like chemotherapy). To date, simple and reliable biomarkers for predicting the survival outcomes of R/M NPC patients undergoing chemoimmunotherapy remain unsatisfactory (18, 23).
Epstein-Barr virus (EBV) DNA, a stable biomarker, has been shown to be useful in predicting the treatment efficacy and monitoring disease progression in NPC patients (18, 24). However, its prognostic value in R/M NPC patients receiving chemoimmunotherapy was uncertain. Interleukin-6 (IL-6), an inflammation cytokine, was associated with cytotoxic T-cell (CTL) differentiation. Blocking the IL-6 receptor has been demonstrated to enhance the efficacy of immunotherapy by promoting tumor immunity while reducing toxicity (25–28). Recent results from prospective clinical trials have also indicated that elevated IL-6 levels were related to tumor progression and poor survival outcomes in patients undergoing ICIs for colon cancer or advanced non–small cell lung cancer (28–30). However, the relationship between IL-6 levels and the prognosis of NPC is unclear, particularly in R/M NPC patients receiving chemoimmunotherapy.
Therefore, our study aimed to evaluate whether pretreatment IL-6 levels could be a prognostic factor for R/M NPC. If possible, we want to combine EBV DNA and IL-6 levels to develop a simple but useful prognostic risk grouping model for R/M NPC receiving PD-1 mAb and platinum-containing chemotherapy.
Materials and methods
Data collection and patient enrollment
From January 1, 2020 to September 30, 2023, a total of 1590 patients with R/M NPC at Sun Yat-sen University Cancer Center (SYSUCC) were screened for inclusion in this study. The entry criteria were as follows: (1) patients with pathologically confirmed WHO type I, II, or III NPC; (2) patients with local or regional recurrence confirmed pathologically or distant metastasis confirmed pathologically or radiologically; (3) patients receiving palliative platinum-based chemotherapy combined with PD-1 mAb as first-line or later-line treatment; (4) patients who had completed at least two cycles of chemoimmunotherapy; (5) availability of pretreatment clinical information, laboratory data and electronic medical records. The exclusion criteria included: (1) Patients who received chemoimmunotherapy as adjuvant treatment following curative therapies; (2) patients with active infection at the time of serum IL-6 testing. (3) patients diagnosed with other malignancies. At last, 319 patients were eligible for analysis, and randomly divided into a training cohort (n=223) and an independent validation cohort (n=96) at a ratio of 7:3 (Figure 1).
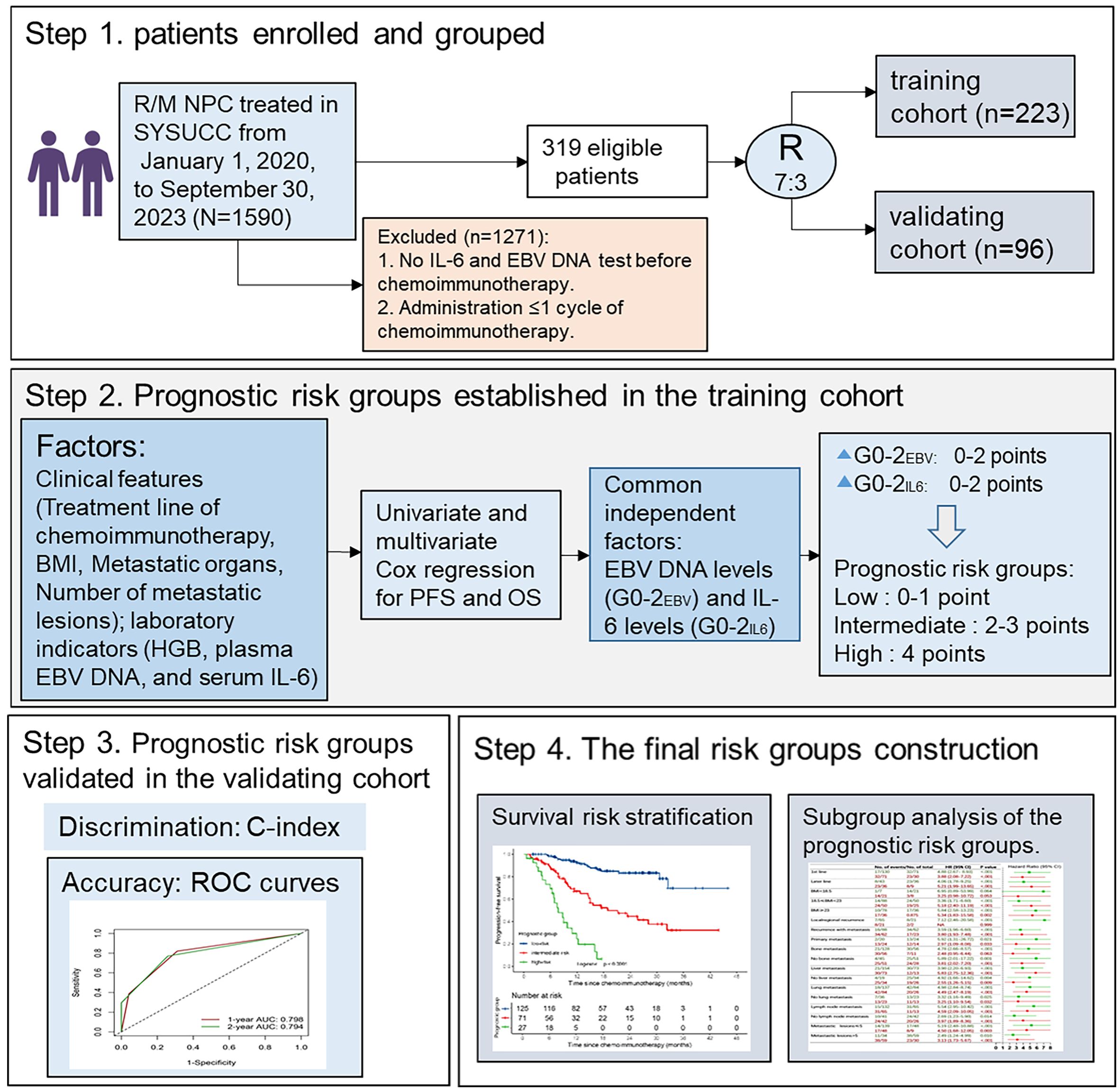
Figure 1. Flowchart of the study. BMI, body mass index; HB, Hemoglobin; IL-6, interleukin-6; EBV, Epstein-Barr virus; G, grade.
This study was approved by the Ethics Committee of Sun Yat-sen University Cancer Center (SL-B2024-565-01) with exempting the need for informed consent as it did not involve any special interventions.
Treatment
R/M NPC patients receiving one of the following chemotherapy regimens: GP (gemcitabine+cisplatin), TPF (docetaxel+cisplatin+5-fluorouracil), TPC (docetaxel+cisplatin+capecitabine), TP (docetaxel+cisplatin) or PF (cisplatin+5-fluorouracil), combined with PD-1 mAb (Toripalimab, Camrelizumab, Sintilimab, Tislelizumab, Pembrolizumab, or Nivolumab) for a maximum of six cycles. Maintenance therapy with PD-1 mAb was continued until disease progression, death, the occurrence of intolerable toxicities, a maximum of two years or upon the patient’s request to discontinue. Carboplatin, nedaplatin, or lobaplatin were accepted as alternatives to cisplatin (31–34). Radiotherapy or surgery therapy for locoregional or metastatic lesions was permitted during chemoimmunotherapy according to the oncologists’ decisions. Detailed information on radiotherapy was provided in Supplementary Methods in the Supplementary Material.
Study endpoints
The endpoint of this study was PFS, defined as the time from the initiation of chemoimmunotherapy to either disease progress or death caused by any reason, censored (patients alive without progression at the last clinic date), or the last follow-up. Another endpoint was OS, defined as the time from the initiation of chemoimmunotherapy to death caused by any reason, censored, or the last follow-up visit.
Follow-up and evaluation
During chemoimmunotherapy, patients underwent regular examinations every 2-3 cycles. For those on PD-1 mAb monotherapy, examinations were conducted every 2-4 cycles. After completing treatment, follow-up visits were scheduled at least every three months during the first two years, every six months from the third to fifth year, and annually thereafter. The last follow-up date was May 30, 2024. Regular evaluations included nasopharyngeal and cervical magnetic resonance imaging (MRI), thoracic computed tomography (CT) scans, abdominal CT scans or ultrasound, bone scans, or positron emission tomography/computed tomography (PET/CT). All radiological imaging assessments were according to the RECIST 1.1 criteria.
Predictors
Potential predictors were pre-specified based on clinical experience and current literature regarding risk stratification for disease progression and mortality in NPC (25, 35–37), All predictors were collected at pretreatment, including sex, age, T stage, N stage, metastatic organs, number of metastatic lesions, treatment line of chemoimmunotherapy, hemoglobin, plasma EBV DNA copy number and serum IL-6 concentration. Laboratory values (including hemoglobin, plasma EBV DNA copy number, and serum IL-6 concentration) were included if obtained within one week prior to chemoimmunotherapy initiation. If multiple values were available, the value closest to the treatment start date was selected. These values were frequently, though not always, obtained on day 1 of cycle 1.
EBV DNA copy number and IL-6 concentration were tested in the laboratory of our hospital. Plasma EBV DNA copy numbers were measured using real-time quantitative polymerase chain reaction (qPCR, Sansure Biotech, Changsha, China). Amplification was performed on an Applied Biosystems 7500 sequence detector, with data analysis conducted using the sequence detection system software (version 1.6.3, Foster City, CA) developed by Applied Biosystems, with a detection limit of 0 copy/mL. Serum IL-6 concentrations was determined using commercially available human IL-6 quantitative enzyme-linked immunosorbent assay (ELISA) kits (R&D Systems, Minneapolis, MN, USA), with a detection limit of 2.5pg/mL.
Statistical analysis
Both IL-6 concentration and EBV DNA copy number values were converted into categorical variables for analysis. Firstly, in the training cohort, IL-6 values were divided into four subgroups using integral quartile cut-off values. Then, pairwise comparisons were performed using the log-rank test to merge subgroups with similar survival outcomes. Finally, the subgroups with distinct survival differences were identified. The same method was applied to group EBV DNA values. To prevent over-optimistic results from developing and testing solely within the training set, validation was performed in the independent validation cohort.
The T-test or Mann-Whitney U test was used to compare continuous variables, while the chi-square or Fisher’s exact test was used to compare categorical variables. Cox proportional hazard models were employed to calculate hazard ratios (HRs) and 95% confidence intervals (CIs) to assess the relationship between variables and PFS/OS. Survival curves were estimated utilizing the Kaplan-Meier method and compared with the log-rank test.
The prognostic risk grouping model was established in the training cohort using the following steps: Firstly, variables P< 0.2 in the univariate Cox regression analyses were selected for further evaluation. Secondly, a backward stepwise multivariable Cox regression model was used to identify independent predictors of PFS and OS. Variables with a significance level of P < 0.05 in the multivariate analyses were retained. Thirdly, a prognostic score was constructed based on the significant and common predictors identified for PFS and OS. Finally, subgroups with similar survival curves were merged to create the final prognostic risk grouping model. The model was evaluated in two aspects: (1) discrimination was measured by the C-index and its 95% CI; (2) accuracy was evaluated by time-dependent ROC curves and area under the curve (AUC) for PFS and OS at 12 and 24 months.
The prognostic risk grouping model was confirmed in the validation set and across different subgroups of the full set, including first and later lines, primary and recurrent metastasis, different body mass indexes (BMI), bone metastasis or not, liver metastasis or not, lung metastasis nor not lymph node metastasis or not or different metastatic lesions.
All statistical analyses were performed using R studio 4.3.2 (Boston, MA, USA), GraphPad Prism 8 (GraphPad Software, Inc., San Diego, CA) and IBM SPSS Statistics 26 for Windows (SPSS Inc, Chicago, IL). Statistical significance thresholds were set at a two-tailed P value of <0.05.
Results
Patient characteristics (the whole cohort)
In the whole cohort of 319 patients, the median age were 49.0 years (interquartile range [IQR] 40.0-57.0), and 231 (72.41%) were treated with chemotherapy plus PD-1 mAb as first-line therapy. 58 patients (18.18%) had de novo metastatic NPC, while 173 patients (54.23%) experienced both local and distant recurrence. The median serum concentration was 7.51 pg/mL (IQR 3.00-18.39) for IL-6 and 996.00 copies/mL (IQR 64.20-13911.00) for EBV DNA.
The median follow-up time of the full set was 18.5 months (IQR 8.9-25.1). During the study period, 111 patients (34.80%) experienced disease progression, and 69 patients (21.63%) died. The pooled analysis of 319 patients revealed a median PFS (mPFS) of 28.10 months (95% CI 24.2-33.5), while the median OS (mOS) was not reached. As shown in Table 1, the pre-treatment clinical characteristics and laboratory results of the training and validating cohorts were balanced.
Determination of different grades for IL-6 and EBV-DNA values (training cohort)
The integral quartile ranges of IL-6 values were as follows: <3.0 (q1), 3.0-7.0 (q2), 7.0-18.0 (q3), and >18.0 (q4) pg/mL (Supplementary Table S1). No significant differences in PFS or OS were observed between subgroups q1 and q2 (Figures 2A, B). Therefore, we combined subgroups q1 and q2 into a single group, G0IL6. Three distinct survival subgroups of IL-6 were identified, representing three different survival risk grades (G0-2IL6), as shown in Figures 2C, D.
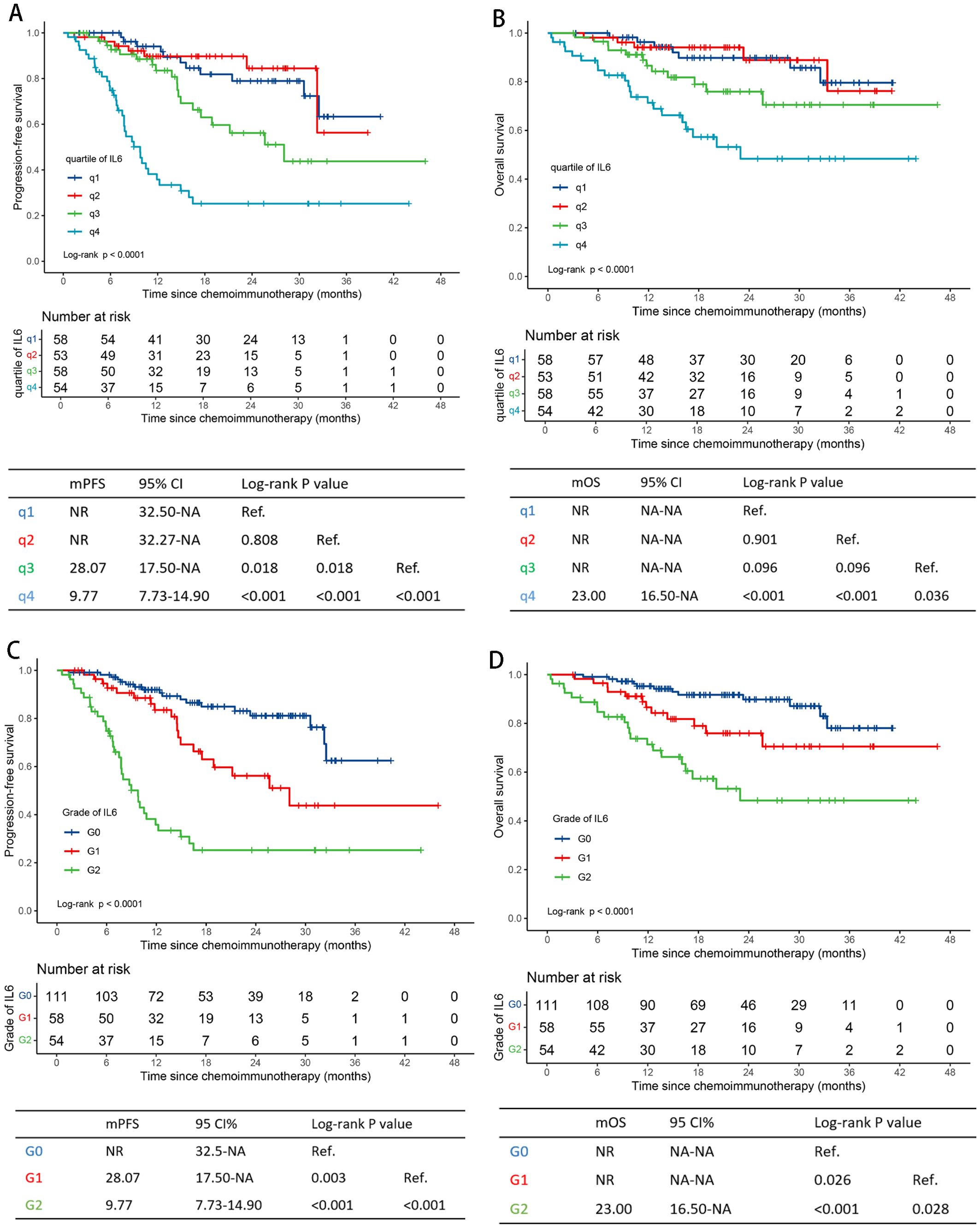
Figure 2. Survival curves by serum IL-6 levels. Progression-free survival curves for q1-4IL6 levels and G0-2IL6 levels (A, C). Overall survival curves for q1-4IL6 levels and G0-2IL6 levels (B, D) in the training cohort. IL-6, interleukin-6; q, quartile; G, grade; NR, not reached; NA, not applicable.
Similarly to EBV DNA value grouping, three different survival risk groups (G0-2EBV) were formed after merging subgroups q1 and q2. The cut-off values were 1000 and 15000 copies/ml (Supplementary Table S1, Supplementary Figures S2A–D).
Establishment of the prognostic risk grouping (training cohort)
In the training cohort, the number of metastatic lesions (>5 VS. ≤5, HR =2.09 [95% CI 1.23-3.55], P=0.006), pretreatment serum IL-6 levels (G1IL6 VS. G0IL6; HR=1.98 [1.05-3.72], P=0.034; G2IL6 VS. G0IL6, HR=4.80 [2.54-9.06], P < 0.001) and EBV DNA levels (G1EBV VS. G0EBV, HR=1.72 [0.91-3.28], P=0.097; G2EBV VS. G0EBV, HR=2.92 [1.57-5.43], P < 0.001) were identified as independent prognostic factors for PFS in multivariable analyses. For OS, EBV DNA (G1EBV VS. G0EBV, HR=2.16 [0.89-5.21], P=0.087; G2EBV VS. G0EBV, HR=5.82 [2.56-13.25], P < 0.001) and IL-6 levels (G1IL6 VS. G0IL6, HR=1.52 [0.68-3.40], P=0.311; G2IL6 VS. G0IL6, HR=2.47 [1.17-5.20], P =0.017) were identified as independent prognostic factors (Table 2).
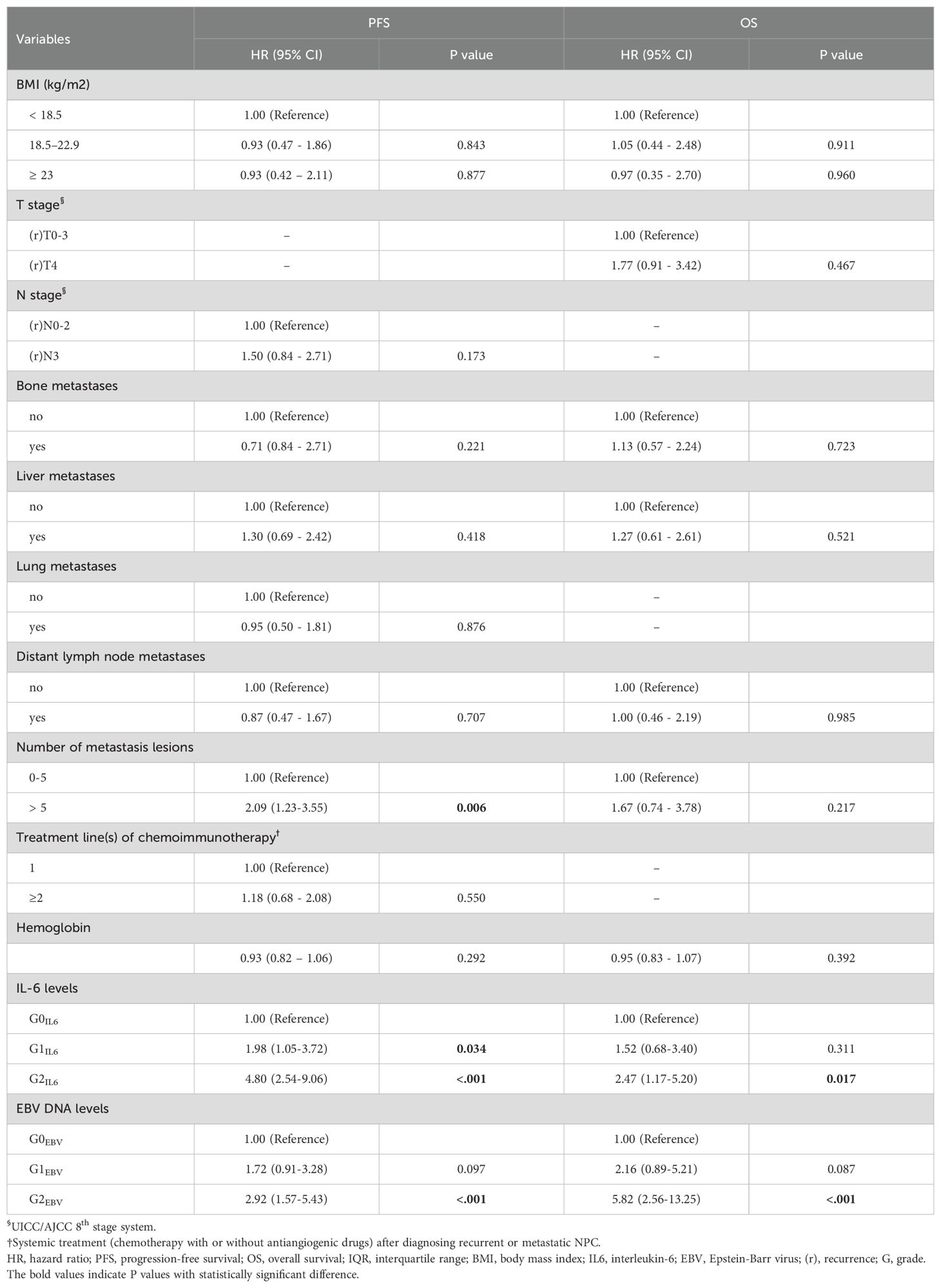
Table 2. Multivariate Cox regression analyses of prognostic factors for PFS and OS in the training cohort.
As both EBV DNA and IL-6 levels were prognostic factors independently for PFS and OS, we developed a simple prognostic score based on these two variables. Given that the prognostic HRs of GEBV0-2 or GIL60-2 approximately doubled with the increasing risk grades in multivariable survival analysis, we assigned 0-2 points for GEBV0-2 and GIL60-2 separately. This resulted in a prognostic score ranging from 0 to 4 points. Pairwise comparisons using the log-rank test revealed no significant difference in survival outcomes between subgroups with 0 and 1 point, or between subgroups with 2 and 3 points (Supplementary Figures S2A, B). Therefore, we combined 0 and 1 point subgroups as the low-risk group, subgroups 2 and 3 points as the intermediate-risk group. The final prognostic risk grouping model consisted of three categories: low risk (0-1 point), intermediate risk (2-3 points), and high risk (4 points) (Figure 3A, Supplementary Figure S3A).
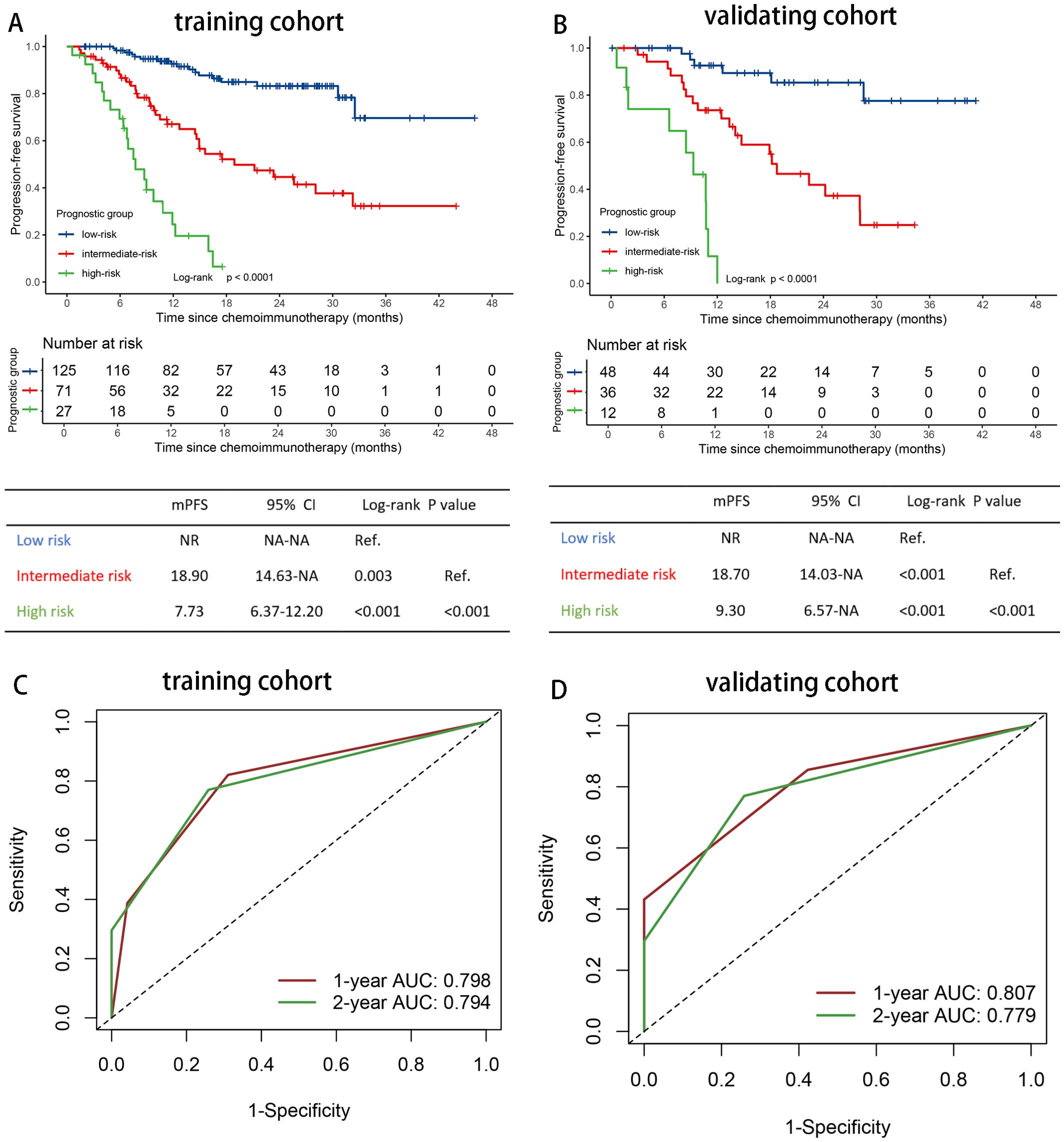
Figure 3. Risk stratification and model evaluation. Prognostic risk stratification and model evaluation. Kaplan Meier curves of PFS for patients stratified by prognostic risk groups in (A) the training and (B) the validation cohort. The time-dependent ROC curves of the prognostic risk grouping for predicting the 1-, and 2-year PFS rate in (C) the training and (D) the validation cohort. ROC, receiver operating characteristic curves; AUC, area under the curve; HR, hazard ratio; PFS, progression-free survival; IL-6, interleukin-6; NR, not reached; NA, not applicable.
Evaluation of the prognostic risk grouping (training cohort)
The C-indexes were 0.753 (95% CI 0.700-0.806) for PFS, and 0.740 (95% CI 0.667-0.813) for OS in the training set. Using time-dependent AUCs, PFS discrimination was 0.798 (95% CI 0.724-0.872) at 12 months and 0.794 (95% CI 0.722-0.866) at 24 months (Figure 3C). For OS, the discrimination were 0.732 (95% CI 0.620-0.819) at 12 months and 0.827 (95% CI 0.748-0.908) at 24 months (Supplementary Figure S3C).
Patients with 0-1 point (low risk, n=125) had the longest median PFS and OS (PFS: NR; OS: NR), followed by those with 2-3 points (intermediate risk, n=71) (PFS, 18.90 months [95%CI 9.30-28.50]; OS, NR), and those with 4 points (high risk, n=27) had the poorest outcomes (PFS, 7.73 months [95% CI 4.94-10.53]; 13.6 months [95% CI 5.49-21.71]) (Figure 3A, Supplementary Figure S3A). The overall P value of PFS and OS were <0.001.
Validation of the prognostic risk grouping (validation cohort)
In the validating set, the C-indexes of the prognostic risk grouping model were 0.757 (95% CI 0.682-0.832) for PFS and 0.766 (95% CI 0.683-0.850) for OS. For the time-dependent AUCs, PFS discrimination was 0.807 (95% CI 0.700-0.916) at 12 months and 0.779 (95% CI 0.672-0.886) at 24 months (Figure 3D). For OS, the AUCs were 0.787 (95% CI 0.672-0.902) at 12 months and 0.782 (95% CI 0.667-0.897) at 24 months (Supplementary Figure S3D).
The median PFS of patients with 0-1 point (low risk, n=48) was not reached, while for those with 2-3 points (intermediate risk, n=36), the median PFS was 18.73 months (95%CI 12.39-25.08). For patients with 4 points (high risk, n=12), the median PFS was 9.30 months (95%CI 6.14-12.46) (P < 0.001) (Figure 3B). The median OS for patients in the high-risk group (4 points) was 17.93 months (95% CI 0.00-37.96), while the median OS for the low- and intermediate-risk groups were not reached (P < 0.001) (Supplementary Figure S3B).
The prognostic risk grouping in different subgroups (the whole cohort)
In the whole set, the median PFS was not reached for the low-risk group (n = 173), 18.73 months (95% CI 11.87-25.60) for the intermediate-risk group (n = 107), and 8.73 months (95% CI 6.68-10.79) for the high-risk group (n = 39). The median OS was 16.47 months (95% CI 8.40-24.53) for the high-risk group, which was not reached for the low- and intermediate-risk groups. To assess the sensitivity of the prognostic risk grouping model, we applied it to different subgroups in the entire cohort (n=319).
In patients who received chemoimmunotherapy as first-line, median PFS was not reached for the low-risk group (n = 130), 25.03 months (95% CI 15.93-34.13) for the intermediate-risk group (n = 71), and 13.60 months (95% CI 8.65-18.55) for the high-risk group (n = 30). In patients who underwent immunotherapy in the later line, median PFS was not reached for the low-risk group (n = 43), 23.33 months (95% CI 14.34-32.32) for the intermediate-risk group (n = 36), and 8.47 months (95% CI 3.50-13.43) for the high-risk group (n = 9) (p <0.001) (Figure 4). A similar trend was observed for OS (Supplementary Figure S4).
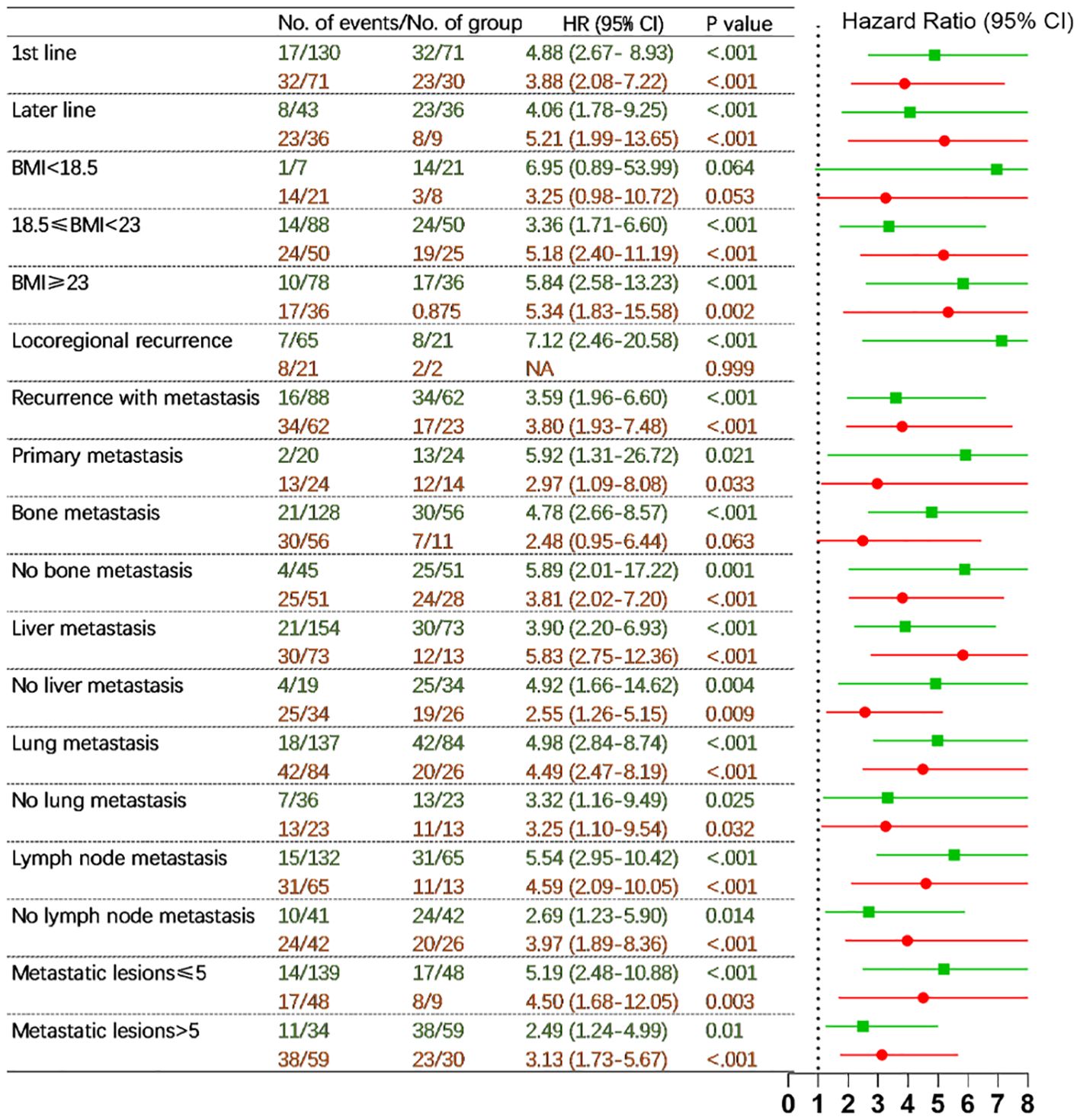
Figure 4. Hazard ratios for disease progression comparing three prognostic risk groups (low- vs. intermediate-risk in green and intermediate- vs. high-risk in red) in different subgroups in the full cohort (adjusting sex, age, hemoglobin, and chemotherapy regimens). HR, hazard ratio; CI, confidence interval; BMI, body mass index; NA, not applicable. P values indicated the levels of statistical differences.
Comparable results were also obtained in further subgroups including patients with BMI <18.5, 18.5-23 and ≥ 23 kg/m2, locoregional recurrence, recurrence with metastasis and primary metastasis, absence and presence of bone metastasis, absence and presence of liver metastasis, absence and presence of lung metastasis, absence and presence of lymph node metastasis, metastatic lesions ≤ 5 and >5 (Figure 4, Supplementary Figure S4).
Discussion
In our study, pretreatment IL-6 levels were identified as an independent prognostic factor for survival in R/M NPC patients receiving chemoimmunotherapy. And we combined pretreatment serum IL-6 and plasma EBV DNA levels to construct a simple but applicable prognostic risk grouping model. This model effectively predicted the likelihood of improved PFS and OS in R/M NPC patients treated with chemoimmunotherapy. Specifically, patients with pretreatment serum IL-6 concentrations exceeding 18.0 pg/ml and EBV DNA copy numbers exceeding 15,000 copies/ml had poorer survival outcomes compared to those who met neither of these criteria. Results were highly consistent in an independent validation and various subgroups. Importantly, our prognostic risk grouping model demonstrated a strong association not only with PFS but also with OS for R/M NPC patients undergoing chemoimmunotherapy.
There is a strong rationale for combining IL-6 and EBV DNA to predict survival outcomes in R/M NPC patients undergoing chemoimmunotherapy. The secretion of IL-6 was stimulated by inflammation or cancer processes (38). Inflammation, regarded as a hallmark of cancer, contributed to tumorigenesis and cancer progression (39), closely linking IL-6 to the mechanisms underlying cancer development and advancement. Furthermore, IL-6 played an critical role in regulating B cell and T cell responses and coordinating the activities of the innate and adaptive immune systems (38), suggesting its association with both the efficacy and toxicity of immunotherapy (25, 26, 38, 40).
High level of IL-6 was correlative with tumor proliferation, poorer chemoimmunotherapy efficacy and worse survival outcomes (26, 28, 29). As presented in Supplementary Table S1, patients with IL-6 levels of 18pg/mL or higher had the poorest median PFS (10.40 months) and OS (20.10 months). Conversely, patients with IL-6 levels of 7pg/mL or lower had the longest median PFS (33.60 months) and a not-reached median OS. Higher serum IL-6 levels were also significantly associated with aggressive disease behaviors, such as M1 stage, multi-metastases, and liver metastases (Supplementary Table S3).
IL-6 has been shown to potentiate several essential transcriptional molecules involved in cell proliferation, including G1/S- specific cyclin D1, the proto-oncogene MYC, BCL-XL, and the apoptosis inhibitor surviving. IL-6 also promoted angiogenesis, tumor invasion, and metastasis through the regulation of hypoxia-inducible factor 1α (HIF-1α), matrix metalloproteinases (MMP2, MMP7, MMP9), and vascular endothelial growth factor (VEGF) (41). Moreover, IL-6 facilitates the metastatic spread of invasive cancer cells by inducing transcriptional activators of epithelial-mesenchymal transition (41). Elevated IL-6 levels also inhibited the production of CD8+ and CD4+ T lymphocyte cells, creating a “cold” tumor microenvironment (TME) that was unfavorable for anti-PD-1 therapy (40). Besides, IL-6 promoted the recruitment of myeloid-derived suppressor cells (MDSCs), which further inhibited the antitumor effectiveness of active T cells (42). Meanwhile, IL-6 induced chemotherapeutic resistance by activating autophagy via IL-6/JAK2/BECN1 signaling pathway, as seen in colorectal cancer (43).
EBV DNA levels were widely recognized as a robust predictor of treatment efficacy and long-term prognosis in NPC, showing correlations with progression-free and recurrence-free survival (15, 18, 20, 44, 45). Our results were consistent with these established findings. Prospective clinical studies have demonstrated that pre-treatment EBV-DNA levels exceeding 2000 or 4000 copies/ml were indicative of higher risks of metastasis and mortality in patients with locally advanced NPC (46, 47). Interestingly, it was observed that EBV-positive NPC cells would contribute to treatment resistance by inducing cancer-associated fibroblast-mediated immunosuppression through YAP1/FAPα signaling (48).
Together, these findings suggested that both IL-6 and EBV DNA levels influence tumor cells directly or indirectly by promoting an immunosuppressive milieu and treatment resistance, which ultimately hampered the efficacy of chemoimmunotherapy. Based on these insights, we integrated IL-6 and EBV DNA levels to develop a prognostic risk stratification model for R/M NPC patients treated with chemoimmunotherapy, with the aim to predict patient prognosis and optimize therapeutic strategies in clinical practice.
This study had several limitations. As a retrospective analysis, the lack of scheduled radiological assessments might affect the evaluation of PFS. Furthermore, the sample size was limited, however, we reviewed all patients consecutively to minimize selection bias. Additionally, 35.5% of patients received chemoimmunotherapy as a second-line or later treatment rather than a first-line option, thus we analyzed the model’s sensitivity across first- and later-line subgroups. Additionally, mOS could not be determined due to the insufficient follow-up duration, so we used PFS as an alternative endpoint and focused on survival outcomes at 12 and 24 months. Last but not least, an external validation was absent because of a rare detection of pretreatment IL-6 value and we can’t collect enough external data.
In conclusion, our prognostic risk grouping model was simple but associated with the survival outcomes of both PFS and OS in patients with R/M NPC receiving chemoimmunotherapy. This model could assist clinicians in making an initial prognostic assessments. However, a prospective, large-scale, long-term, randomized controlled clinical trial is warranted to validate this prognostic risk stratification model and explore the potential benefits of anti-IL6 therapies.
Data availability statement
The datasets presented in this study can be found in online repositories. The names of the repository/repositories and accession number(s) can be found in the article/Supplementary Material.
Ethics statement
The studies involving humans were approved by Ethics Committee of Sun Yat-sen University Cancer Center. The studies were conducted in accordance with the local legislation and institutional requirements. Written informed consent for participation was not required from the participants or the participants’ legal guardians/next of kin in accordance with the national legislation and institutional requirements.
Author contributions
YL: Conceptualization, Investigation, Methodology, Resources, Validation, Visualization, Writing – review & editing, Formal analysis, Software, Writing – original draft. ZH: Validation, Writing – original draft, Writing – review & editing. CC: Formal analysis, Software, Validation, Visualization, Writing – review & editing, Supervision. SL: Writing – review & editing. YH: Formal analysis, Writing – review & editing, Investigation, Methodology. YT: Writing – review & editing, Investigation. PF: Writing – review & editing. SZ: Investigation, Writing – review & editing, Conceptualization, Data curation, Formal analysis, Funding acquisition, Methodology, Project administration, Resources, Software, Supervision, Validation, Visualization, Writing – original draft. YX: Writing – review & editing, Conceptualization, Data curation, Funding acquisition, Investigation, Methodology, Project administration, Resources, Supervision, Validation, Visualization.
Funding
The author(s) declare that financial support was received for the research and/or publication of this article. This work was supported by the National Natural Science Foundation of China (81872464), the National High Technology Research and Development Program Project (No. 2006AA02Z4B4), and Science and Technology Projects in Guangzhou (2023A04J2120).
Conflict of interest
The authors declare that the research was conducted in the absence of any commercial or financial relationships that could be construed as a potential conflict of interest.
Generative AI statement
The author(s) declare that no Generative AI was used in the creation of this manuscript.
Publisher’s note
All claims expressed in this article are solely those of the authors and do not necessarily represent those of their affiliated organizations, or those of the publisher, the editors and the reviewers. Any product that may be evaluated in this article, or claim that may be made by its manufacturer, is not guaranteed or endorsed by the publisher.
Supplementary material
The Supplementary Material for this article can be found online at: https://www.frontiersin.org/articles/10.3389/fimmu.2025.1560897/full#supplementary-material
References
1. Chen YP, Chan A, Le QT, Blanchard P, Sun Y, Ma J. Nasopharyngeal carcinoma. Lancet. (2019) 394:64–80. doi: 10.1016/S0140-6736(19)30956-0
2. Ribassin-Majed L, Marguet S, Lee A, Ng WT, Ma J, Chan A, et al. What is the best treatment of locally advanced nasopharyngeal carcinoma? An individual patient data network meta-analysis. J Clin Oncol. (2017) 35:498–505. doi: 10.1200/JCO.2016.67.4119
3. Lee AW, Ma BB, Ng WT, Chan AT. Management of nasopharyngeal carcinoma: current practice and future perspective. J Clin Oncol. (2015) 33:3356–64. doi: 10.1200/JCO.2015.60.9347
4. Li ZQ, Xia YF, Liu Q, Yi W, Liu XF, Han F, et al. Radiotherapy-related typing in 842 patients in canton with nasopharyngeal carcinoma. Int J Radiat Oncol Biol Phys. (2006) 66:1011–16. doi: 10.1016/j.ijrobp.2006.06.028
5. Liao W, Tian M, Chen N. Characteristic and novel therapeutic strategies of nasopharyngeal carcinoma with synchronous metastasis. Cancer Manag Res. (2019) 11:8431–42. doi: 10.2147/CMAR.S219994
6. Mai HQ, Chen QY, Chen D, Hu C, Yang K, Wen J, et al. Toripalimab or placebo plus chemotherapy as first-line treatment in advanced nasopharyngeal carcinoma: a multicenter randomized phase 3 trial. Nat Med. (2021) 27:1536–43. doi: 10.1038/s41591-021-01444-0
7. Yang Y, Qu S, Li J, Hu C, Xu M, Li W, et al. Camrelizumab versus placebo in combination with gemcitabine and cisplatin as first-line treatment for recurrent or metastatic nasopharyngeal carcinoma (CAPTAIN-1st): a multicentre, randomised, double-blind, phase 3 trial. Lancet Oncol. (2021) 22:1162–74. doi: 10.1016/S1470-2045(21)00302-8
8. Hong S, Zhang Y, Yu G, Peng P, Peng J, Jia J, et al. Gemcitabine plus cisplatin versus fluorouracil plus cisplatin as first-line therapy for recurrent or metastatic nasopharyngeal carcinoma: final overall survival analysis of GEM20110714 phase III study. J Clin Oncol. (2021) 39:3273–82. doi: 10.1200/JCO.21.00396
9. Mai HQ, Chen QY, Chen D, Hu C, Yang K, Wen J, et al. Toripalimab plus chemotherapy for recurrent or metastatic nasopharyngeal carcinoma: the JUPITER-02 randomized clinical trial. Jama. (2023) 330:1961–70. doi: 10.1001/jama.2023.20181
10. Li WZ, Lv X, Hu D, Lv SH, Liu GY, Liang H, et al. Effect of induction chemotherapy with paclitaxel, cisplatin, and capecitabine vs cisplatin and fluorouracil on failure-free survival for patients with stage IVA to IVB nasopharyngeal carcinoma: A multicenter phase 3 randomized clinical trial. JAMA Oncol. (2022) 8:706–14. doi: 10.1001/jamaoncol.2022.0122
11. Liu GY, Ye YF, Jiang YF, Chen GJ, Xia WX, Huang YS, et al. Nab-paclitaxel, cisplatin, and capecitabine versus cisplatin and gemcitabine as first line chemotherapy in patients with recurrent or metastatic nasopharyngeal carcinoma: randomised phase 3 clinical trial. Bmj. (2024) 385:e77890. doi: 10.1136/bmj-2023-077890
12. Yang Y, Pan J, Wang H, Zhao Y, Qu S, Chen N, et al. Tislelizumab plus chemotherapy as first-line treatment for recurrent or metastatic nasopharyngeal cancer: A multicenter phase 3 trial (RATIONALE-309). Cancer Cell. (2023) 41:1061–72. doi: 10.1016/j.ccell.2023.04.014
13. Zhang J, Fang W, Qin T, Yang Y, Hong S, Liang W, et al. Co-expression of PD-1 and PD-L1 predicts poor outcome in nasopharyngeal carcinoma. Med Oncol. (2015) 32:86. doi: 10.1007/s12032-015-0501-6
14. Zhu Q, Cai MY, Chen CL, Hu H, Lin HX, Li M, et al. Tumor cells PD-L1 expression as a favorable prognosis factor in nasopharyngeal carcinoma patients with pre-existing intratumor-infiltrating lymphocytes. Oncoimmunology. (2017) 6:e1312240. doi: 10.1080/2162402X.2017.1312240
15. Fang W, Zhang J, Hong S, Zhan J, Chen N, Qin T, et al. EBV-driven LMP1 and IFN-γ up-regulate PD-L1 in nasopharyngeal carcinoma: Implications for oncotargeted therapy. Oncotarget. (2014) 5:12189–202. doi: 10.18632/oncotarget.2608
16. Ma B, Lim WT, Goh BC, Hui EP, Lo KW, Pettinger A, et al. Antitumor activity of nivolumab in recurrent and metastatic nasopharyngeal carcinoma: an international, multicenter study of the mayo clinic phase 2 consortium (NCI-9742). J Clin Oncol. (2018) 36:1412–18. doi: 10.1200/JCO.2017.77.0388
17. Huang ZL, Liu S, Wang GN, Zheng SH, Ding SR, Tao YL, et al. The prognostic significance of PD-L1 and PD-1 expression in patients with nasopharyngeal carcinoma: a systematic review and meta-analysis. Cancer Cell Int. (2019) 19:141. doi: 10.1186/s12935-019-0863-5
18. Wang FH, Wei XL, Feng J, Li Q, Xu N, Hu XC, et al. Efficacy, safety, and correlative biomarkers of toripalimab in previously treated recurrent or metastatic nasopharyngeal carcinoma: A phase II clinical trial (POLARIS-02). J Clin Oncol. (2021) 39:704–12. doi: 10.1200/JCO.20.02712
19. Guo G, Wang Y, Zhou Y, Quan Q, Zhang Y, Wang H, et al. Immune cell concentrations among the primary tumor microenvironment in colorectal cancer patients predicted by clinicopathologic characteristics and blood indexes. J Immunother Cancer. (2019) 7:179. doi: 10.1186/s40425-019-0656-3
20. Yang Y, Zhou T, Chen X, Li J, Pan J, He X, et al. Efficacy, safety, and biomarker analysis of Camrelizumab in Previously Treated Recurrent or Metastatic Nasopharyngeal Carcinoma (CAPTAIN study). J Immunother Cancer. (2021) 9:e003790. doi: 10.1136/jitc-2021-003790
21. Chung IC, Chen LC, Tsang NM, Chuang WY, Liao TC, Yuan SN, et al. Mitochondrial oxidative phosphorylation complex regulates NLRP3 inflammasome activation and predicts patient survival in nasopharyngeal carcinoma. Mol Cell Proteomics. (2020) 19:142–54. doi: 10.1074/mcp.RA119.001808
22. Miao S, Lei H, Li X, Zhou W, Wang G, Sun A, et al. Development and validation of a risk prediction model for overall survival in patients with nasopharyngeal carcinoma: a prospective cohort study in China. Cancer Cell Int. (2022) 22:360. doi: 10.1186/s12935-022-02776-8
23. Yuan L, Jia GD, Lv XF, Xie SY, Guo SS, Lin DF, et al. Camrelizumab combined with apatinib in patients with first-line platinum-resistant or PD-1 inhibitor resistant recurrent/metastatic nasopharyngeal carcinoma: a single-arm, phase 2 trial. Nat Commun. (2023) 14:4893. doi: 10.1038/s41467-023-40402-x
24. Xu JY, Wei XL, Ren C, Zhang Y, Hu YF, Li JY, et al. Association of plasma Epstein-Barr virus DNA with outcomes for patients with recurrent or metastatic nasopharyngeal carcinoma receiving anti-programmed cell death 1 immunotherapy. JAMA Netw Open. (2022) 5:e220587. doi: 10.1001/jamanetworkopen.2022.0587
25. Huseni MA, Wang L, Klementowicz JE, Yuen K, Breart B, Orr C, et al. CD8(+) T cell-intrinsic IL-6 signaling promotes resistance to anti-PD-L1 immunotherapy. Cell Rep Med. (2023) 4:100878. doi: 10.1016/j.xcrm.2022.100878
26. Hailemichael Y, Johnson DH, Abdel-Wahab N, Foo WC, Bentebibel SE, Daher M, et al. Interleukin-6 blockade abrogates immunotherapy toxicity and promotes tumor immunity. Cancer Cell. (2022) 40:509–23. doi: 10.1016/j.ccell.2022.04.004
27. Tsai MS, Chen WC, Lu CH, Chen MF. The prognosis of head and neck squamous cell carcinoma related to immunosuppressive tumor microenvironment regulated by IL-6 signaling. Oral Oncol. (2019) 91:47–55. doi: 10.1016/j.oraloncology.2019.02.027
28. Liu C, Yang L, Xu H, Zheng S, Wang Z, Wang S, et al. Systematic analysis of IL-6 as a predictive biomarker and desensitizer of immunotherapy responses in patients with non-small cell lung cancer. BMC Med. (2022) 20:187. doi: 10.1186/s12916-022-02356-7
29. Tan D, Felip E, de Castro G, Solomon BJ, Greystoke A, Cho BC, et al. Canakinumab versus placebo in combination with first-line pembrolizumab plus chemotherapy for advanced non-small-cell lung cancer: results from the CANOPY-1 trial. J Clin Oncol. (2024) 42:192–204. doi: 10.1200/JCO.23.00980
30. Cheng E, Shi Q, Shields AF, Nixon AB, Shergill AP, Ma C, et al. Association of inflammatory biomarkers with survival among patients with stage III colon cancer. JAMA Oncol. (2023) 9:404–13. doi: 10.1001/jamaoncol.2022.6911
31. Tang LQ, Chen DP, Guo L, Mo HY, Huang Y, Guo SS, et al. Concurrent chemoradiotherapy with nedaplatin versus cisplatin in stage II-IVB nasopharyngeal carcinoma: an open-label, non-inferiority, randomised phase 3 trial. Lancet Oncol. (2018) 19:461–73. doi: 10.1016/S1470-2045(18)30104-9
32. Lv X, Cao X, Xia WX, Liu KY, Qiang MY, Guo L, et al. Induction chemotherapy with lobaplatin and fluorouracil versus cisplatin and fluorouracil followed by chemoradiotherapy in patients with stage III-IVB nasopharyngeal carcinoma: an open-label, non-inferiority, randomised, controlled, phase 3 trial. Lancet Oncol. (2021) 22:716–26. doi: 10.1016/S1470-2045(21)00075-9
33. Tan EH, Khoo KS, Wee J, Fong KW, Lee KS, Lee KM, et al. Phase II trial of a paclitaxel and carboplatin combination in Asian patients with metastatic nasopharyngeal carcinoma. Ann Oncol. (1999) 10:235–37. doi: 10.1023/a:1008390929826
34. Chan AT, Ma BB, Lo YM, Leung SF, Kwan WH, Hui EP, et al. Phase II study of neoadjuvant carboplatin and paclitaxel followed by radiotherapy and concurrent cisplatin in patients with locoregionally advanced nasopharyngeal carcinoma: therapeutic monitoring with plasma Epstein-Barr virus DNA. J Clin Oncol. (2004) 22:3053–60. doi: 10.1200/JCO.2004.05.178
35. Gao J, Hu JY, Xia YF, Yi W, Tao YL, Li G. Continuous fall in hemoglobin level is a poor prognostic factor in patients with nasopharyngeal carcinoma treated with radiotherapy. Chin J Cancer. (2010) 29:561–66. doi: 10.5732/cjc.009.10533
36. Du XJ, Wang GY, Zhu XD, Han YQ, Lei F, Shen LF, et al. Refining the 8th edition TNM classification for EBV related nasopharyngeal carcinoma. Cancer Cell. (2024) 42:464–73. doi: 10.1016/j.ccell.2023.12.020
37. Qi Q, Peng Y, Zhu M, Zhang Y, Bao Y, Zhang X, et al. Association between serum levels of 12 different cytokines and short-term efficacy of anti-PD-1 monoclonal antibody combined with chemotherapy in advanced gastric cancer. Int Immunopharmacol. (2023) 114:109553. doi: 10.1016/j.intimp.2022.109553
38. Garbers C, Heink S, Korn T, Rose-John S. Interleukin-6: designing specific therapeutics for a complex cytokine. Nat Rev Drug Discovery. (2018) 17:395–412. doi: 10.1038/nrd.2018.45
39. Hanahan D, Weinberg RA. Hallmarks of cancer: the next generation. Cell. (2011) 144:646–74. doi: 10.1016/j.cell.2011.02.013
40. Yang H, Kang B, Ha Y, Lee SH, Kim I, Kim H, et al. High serum IL-6 correlates with reduced clinical benefit of atezolizumab and bevacizumab in unresectable hepatocellular carcinoma. JHEP Rep. (2023) 5:100672. doi: 10.1016/j.jhepr.2023.100672
41. Jones SA, Jenkins BJ. Recent insights into targeting the IL-6 cytokine family in inflammatory diseases and cancer. Nat Rev Immunol. (2018) 18:773–89. doi: 10.1038/s41577-018-0066-7
42. Chen MF, Kuan FC, Yen TC, Lu MS, Lin PY, Chung YH, et al. IL-6-stimulated CD11b+ CD14+ HLA-DR- myeloid-derived suppressor cells, are associated with progression and poor prognosis in squamous cell carcinoma of the esophagus. Oncotarget. (2014) 5:8716–28. doi: 10.18632/oncotarget.2368
43. Hu F, Song D, Yan Y, Huang C, Shen C, Lan J, et al. IL-6 regulates autophagy and chemotherapy resistance by promoting BECN1 phosphorylation. Nat Commun. (2021) 12:3651. doi: 10.1038/s41467-021-23923-1
44. Hui EP, Li WF, Ma BB, Lam W, Chan K, Mo F, et al. Integrating postradiotherapy plasma Epstein-Barr virus DNA and TNM stage for risk stratification of nasopharyngeal carcinoma to adjuvant therapy. Ann Oncol. (2020) 31:769–79. doi: 10.1016/j.annonc.2020.03.289
45. Chan A, Hui EP, Ngan R, Tung SY, Cheng A, Ng WT, et al. Analysis of plasma Epstein-Barr virus DNA in nasopharyngeal cancer after chemoradiation to identify high-risk patients for adjuvant chemotherapy: A randomized controlled trial. J Clin Oncol. (2018), JCO2018777847. doi: 10.1200/JCO.2018.77.7847
46. Guo R, Tang LL, Mao YP, Du XJ, Chen L, Zhang ZC, et al. Proposed modifications and incorporation of plasma Epstein-Barr virus DNA improve the TNM staging system for Epstein-Barr virus-related nasopharyngeal carcinoma. Cancer. (2019) 125:79–89. doi: 10.1002/cncr.31741
47. Chen WH, Tang LQ, Guo SS, Chen QY, Zhang L, Liu LT, et al. Prognostic value of plasma Epstein-Barr virus DNA for local and regionally advanced nasopharyngeal carcinoma treated with cisplatin-based concurrent chemoradiotherapy in intensity-modulated radiotherapy era. Med (Baltimore). (2016) 95:e2642. doi: 10.1097/MD.0000000000002642
Keywords: interleukin-6, EBV DNA, prognostic risk grouping model, recurrent or metastatic nasopharyngeal carcinoma, chemoimmunotherapy
Citation: Liu Y, Huang Z, Chen C, Hu Y, Tao Y, Liu S, Feng P, Zheng S and Xia Y (2025) Combining interleukin 6 and EBV DNA levels predicts survival outcomes for patients with recurrent or metastatic nasopharyngeal carcinoma receiving chemoimmunotherapy. Front. Immunol. 16:1560897. doi: 10.3389/fimmu.2025.1560897
Received: 15 January 2025; Accepted: 03 March 2025;
Published: 14 March 2025.
Edited by:
Xiangpeng Dai, Jilin University, ChinaReviewed by:
Xiaoyan Qian, Henan Provincial People’s Hospital, ChinaQiao He, University of Electronic Science and Technology of China, China
Copyright © 2025 Liu, Huang, Chen, Hu, Tao, Liu, Feng, Zheng and Xia. This is an open-access article distributed under the terms of the Creative Commons Attribution License (CC BY). The use, distribution or reproduction in other forums is permitted, provided the original author(s) and the copyright owner(s) are credited and that the original publication in this journal is cited, in accordance with accepted academic practice. No use, distribution or reproduction is permitted which does not comply with these terms.
*Correspondence: Shuohan Zheng, emhlbmdzaDFAc3lzdWNjLm9yZy5jbg==; Yunfei Xia, eGlheWZAc3lzdWNjLm9yZy5jbg==