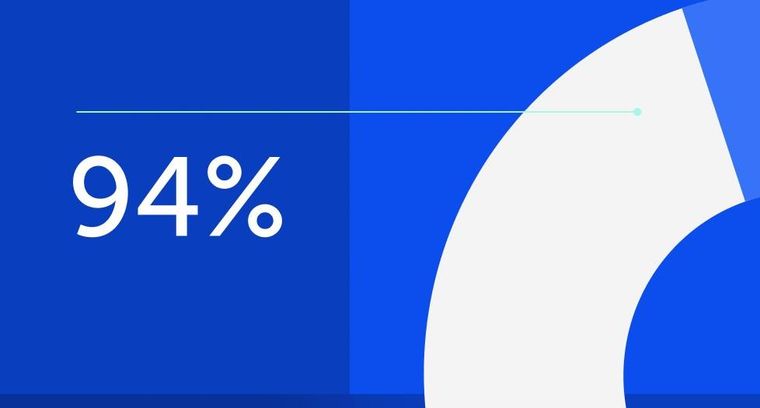
94% of researchers rate our articles as excellent or good
Learn more about the work of our research integrity team to safeguard the quality of each article we publish.
Find out more
ORIGINAL RESEARCH article
Front. Immunol., 16 April 2025
Sec. Cancer Immunity and Immunotherapy
Volume 16 - 2025 | https://doi.org/10.3389/fimmu.2025.1559211
This article is part of the Research TopicMicrobiota-Immunity Dynamics in Cancer: Mechanisms and Implications for Treatment StrategiesView all articles
Background: To research the clinical characteristics, risk factors, the correlation between bacterial pulmonary infection and immune function of advanced lung adenocarcinoma patients complicated with bacterial pulmonary infection.
Methods: 334 stage III and IV lung adenocarcinoma patients admitted to the first affiliated hospital of Zhengzhou University from January 2020 to March 2023 were selected and divided into an infection group (n = 240) and a control group (n= 72) according to whether complicated with bacterial pulmonary infection. The clinical characteristics were analyzed. The pulmonary microbiota and human T lymphocyte subsets (CD3+, CD4+, CD8+) were detected. Multivariate logistic regression analysis was performed to explore the risk factors for pulmonary bacterial infection in advanced lung adenocarcinoma patients.
Results: Among 334 patients, 264 cases were complicated with pulmonary bacterial infection, and 70 cases had no pulmonary bacterial infection. In total, 544 pathogenic bacteria were isolated from the patients. Of these, 170 strains (31.25%) were Gram-negative bacilli, 162 strains (29.78%) were Gram-positive cocci, 27 strains (4.96%) Gram-positive bacilli. There were statistically significant differences in age, smoking, combined diseases, TNM staging, CD3+ T cell percentage, and CD4+ T cell percentage between the two groups (P < 0.05). Multivariate logistic regression analysis revealed smoking, bronchiectasis, and diabetes were independent risk factors leading to late-stage lung adenocarcinoma patients with bacterial pulmonary infection (P < 0.05). In those patients on immune checkpoint inhibitors, the lung Gram-positive group has a higher number of CD4+ T cells and CD4+/CD8+ T cell ratio than the Gram-negative group (P < 0.05).
Conclusion: Smoking, bronchiectasis, and diabetes are risk factors for lung bacterial infection in patients with advanced lung adenocarcinoma. The effect of immune checkpoint inhibitor treatment on T cells is more pronounced in Gram positive bacteria.
Lung adenocarcinoma (LUAD) is a major subtype of lung cancer and the most common form of non-small cell lung cancer (NSCLC) (1, 2). LUAD remains a leading cause of mortality worldwide, with its development intricately linked to disruptions in immune regulation (3, 4). Emerging research highlights the critical role of the lung microbiota, particularly bacterial communities, in lung cancer pathogenesis (5–7). Under normal conditions, the lung microbiota helps maintain immune homeostasis (8–10). However, dysbiosis, or the imbalance of specific bacterial populations, can trigger inflammation, facilitate immune evasion, and promote tumor progression (11, 12). For instance, veillonella parvula has been identified as a dominant taxon in dysbiotic profiles that correlate with poor prognosis and increased tumor progression in lung cancer patients (13). Certain bacterial pathogens contribute to an inflammatory microenvironment by secreting metabolites or interacting with host cells, creating conditions conducive to tumor development (14, 15). Therefore, investigating the interplay between lung microbiota dysbiosis, immune modulation, and lung cancer progression offers promising avenues for precision therapy.
Bacterial pulmonary infection (BLI) can influence the development of LUAD (16). BLIs, caused by microbial infections in the lungs, can disrupt the local microbiome and provoke inflammation (17–19). There are increasing reports of pathological and clinical associations between bacterial pneumonia and lung cancer (20, 21). For example, lung cancer patients are particularly susceptible to bacterial pneumonia due to factors such as compromised immune function and airway obstruction (22, 23). Conversely, bacterial infections can exacerbate lung cancer development by inducing chronic inflammation, altering the tumor microenvironment, and enabling immune escape (24–26). Recent studies underscore that bacterial infections may serve as a catalyst for tumor growth (27, 28). However, the pathogenesis of bacterial infection in lung adenocarcinoma patients and how bacteria affect the progression of lung cancer by changing immunity remain poorly understood. Patients with advanced lung adenocarcinoma often have concurrent infection (29, 30). Therefore, we statistically analyzed the clinical characteristics of patients with advanced lung adenocarcinoma, as well as the risk factors for bacterial infection in the lungs and the changes in T cell immune function in these patients, aiming to provide clinical evidence for the selection of appropriate treatment methods.
This study analyzed the clinical data of patients with advanced lung adenocarcinoma who were admitted to the First Affiliated Hospital of Zhengzhou University between January 2020 and March 2023. The collection and analysis flowchart of patient data is shown in Figure 1.
Inclusion criteria: ①Pathologically confirmed diagnosis of NSCLC. ②Disease stage III or IV, classified according to the 8th edition of the International Association for the Study of Lung Cancer (IASLC) staging system. ③Patients who completed the sixth cycle of standard chemotherapy were assessed 30-60 days post-treatment.
Exclusion criteria: ①Presence of autoimmune diseases. ②Diagnosis of primary extrapulmonary tumors. ③History of prior surgical intervention. ④Presence of skin infections, such as diabetic foot ulcers or open wounds. ⑤Severe hepatic or renal dysfunction, or bone marrow suppression leading to irregular chemotherapy cycles. ⑥Prolonged use of antibiotics (≥3 days) before blood collection.
Patients were categorized into two groups based on the presence of bacterial pulmonary infections, determined through sputum smear results, bronchoalveolar lavage fluid Next Generation Sequencing (NGS), or chest high-resolution computed tomography (HRCT). The two cohorts included those with late-stage LUAD and BLI (LUAD-BLI group) and those with late-stage LUAD without BLI (LUAD group).
This study was approved by the Ethics Committee of the First Affiliated Hospital of Zhengzhou University (approval number: 2022-KY-0677-003, Supplementary Materials 1).
The microbial genomic DNA (gDNA) was extracted using the Zymo Research BIOMICS DNA Microprep Kit (Product Code: D4301). The quality of the extracted gDNA was assessed through 0.8% agarose gel electrophoresis, and its concentration was measured using the Tecan F200 system with the PicoGreen dye-based quantification method. Specific primers targeting the full-length 16S rRNA gene, including the forward primer 8F (5’AGAGTTTGATCATGGCTCAG3’) and the reverse primer 1492R (5’CGGTTACCTTGTTACGACTT3’), were employed to amplify the target region of the samples. Each sample was processed in triplicate, and the PCR reactions were stopped during the linear amplification phase. The PCR amplicons from the same sample were pooled and analyzed by electrophoresis. Subsequently, the desired DNA fragments were excised from the gel and purified using a gel extraction kit, followed by washing and elution with TE buffer. The purified PCR products were quantified using the Qubit 2.0 Fluorometer. After quality control verification, the Nanopore R9.4.1 library preparation kit was utilized to construct sequencing libraries. Finally, the libraries were sequenced on the MinION platform, and real-time high-accuracy base calling was performed during the sequencing process.
Medical records were reviewed to collect crucial clinical characteristics.
General information: gender, age, tumor node metastasis (TNM) staging, Performance Status (PS score), immune checkpoint inhibitor treatment.
Clinical characteristics: underlying diseases, smoking history, body mass index (BMI).
Laboratory indicators: CD3+ T cell percentage, CD4+ T cell count, CD8+T cell count, CD4+/CD8+T cell ratio, sputum smear or bronchoalveolar lavage fluid NGS test results.
Survival follow-up: Follow up will be conducted after 1 year, and if the patient dies during this period, it will be marked as poor prognosis.
All data were analyzed using SPSS 21.0 software. Continuous variables are expressed as mean ± standard deviation and compared between groups through student t-test. When the sample size within the group is less than 30 and does not follow a normal distribution, rank sum test is used for interring group comparison. Categorical variables are expressed as percentages (%) and chi-square tests are used for interring group comparisons. After single factor screening, multiple logistic regression analysis was used to determine the relevant independent risk factors, and all relevant hazard ratios (HR) and 95% confidence intervals (CI) were calculated. P < 0.05 is considered statistically significant.
A total of 544 strains of pathogenic bacteria were isolated from the infected individuals, among which 162 strains of Gram-positive cocci were detected, accounting for 29.78%; 27 strains of Gram-positive bacteria were detected, accounting for 4.96%; 170 strains of Gram-negative bacteria were detected, accounting for 31.25%; 38 strains of Candida were detected, accounting for 6.99%, 38 strains of aspergillus were detected, accounting for 6.99%. In addition, 109 strains of non-bacterial infections, such as SARS-CoV-2, Influenza, Epstein-Barr virus, Cytomegalovirus, mycobacterium tuberculosis, nontuberculous mycobacteria, Mycoplasma, non-bacterial infections were also detected, accounting for 20.0% of the total (Table 1).
Table 1. Detection of pathogens in patients with adenocarcinoma of the lung complicated with pulmonary infection.
The 334 advanced stage lung adenocarcinoma patients were then divided into a group of lung cancer patients with bacterial infections (LUAD-BLI group, n = 264) and a group of lung cancer patients without bacterial infection (LUAD group, n = 70) based on whether there is a bacterial infection in the lungs. We examined the baseline clinical characteristics of lung adenocarcinoma patients and observed some significant difference. Compared with the non-infected group, the infected group is older in age. Compared to non-smokers, the number of infected patients in the smoking population has significantly increased. The infection rate of stage 4 patients has increased compared to stage 3 patients. The infection rate in patients with bronchiectasis and diabetes was significantly higher. Compared with the non-infected group, the proportion of CD3+ T cell percentage and the CD4+ T cell count in the infected group patients was significantly reduced. In addition, there were no statistically significant differences in other clinical indicators such as gender, BMI, chronic obstructive pulmonary disease, hypertension, PS score, CD8+T cell count and CD4+/CD8+T cell ratio between the two groups (Table 2).
In the univariate analyses (Table 3), infection was significantly associated with age (OR: 1.032, 95% CI: 1.008-1.056; P = 0.008), smoking (OR: 2.728, 95% CI: 1.365-5.452; P = 0.005), bronchiectasis (OR: 3.906, 95% CI: 1.505-10.137; P = 0.005), Diabetes (OR: 8.216, 95% CI: 2.504-26.958; P = 0.001), stages (OR: 2.616, 95% CI: 1.074-6.372; P = 0.034), CD3+T cell percentage (OR: 0.966, 95% CI: 0.943-0.989; P = 0.005), and CD4+T cell count (OR: 0.999, 95% CI: 0.998-1.000; P = 0.004). In the multivariate analyses, infection was independently associated with smoking (OR: 2.305, 95% CI: 1.091-4.867; P = 0.029), bronchiectasis (OR: 5.054, 95% CI: 1.885-13.554; P = 0.001), and Diabetes (OR: 7.290, 95% CI: 2.146-24.760; P = 0.001).
Table 3. Cox regression analysis of variables impacting advanced stage lung adenocarcinoma patients with concurrent infections.
Immune checkpoint inhibitors are widely used in the treatment of advanced lung adenocarcinoma. After their application, the number of cytotoxic T cells in tumor tissues often increases. On the other hand, the above results show that if bacterial infection occurs in the lungs, the number of T cells in patients will decrease. In view of this, it is of great significance to explore the impact of immune checkpoint inhibitors on cellular immunity in patients for optimizing treatment regimens. Different bacteria lead to different changes in cellular immunity. By distinguishing these changes, the role of bacteria in immune checkpoint treatment can be clarified. Therefore, we divided lung adenocarcinoma patients treated with immune checkpoint inhibitors and complicated with pulmonary bacterial pneumonia infection into two groups according to the types of bacteria: the group with immune checkpoint treatment combined with Gram-negative bacteria and the group with immune checkpoint treatment combined with Gram-positive bacteria. The results showed that compared with the group with Gram-negative bacteria, the number of CD4+ T cells and the ratio of CD4/CD8 in the immune inhibitor treatment group of the Gram-positive bacteria group were higher (Table 4).
Table 4. Differences in T cell subpopulation in immune checkpoint inhibitor treatment for lung adenocarcinoma patients with pulmonary bacterial pneumonia infection by Gram-positive (G-) vs Gram-negative (G+) bacteria.
The occurrence and development of LUAD are complex, multi-step process involving the interaction of genetic and environmental factors (31–33). In recent years, the relationship between lung microbiota, including bacteria, viruses, and fungi, and lung cancer has garnered increasing attention (5, 34, 35). The lung microbiota, which is composed of bacteria, fungi, and viruses, plays a critical role in respiratory health. It interacts with host immunity and maintains microbial balance (36). Dysbiosis disrupts this equilibrium. It contributes to chronic inflammation, fibrosis, and malignancy through pathogen overgrowth, toxin production, and aberrant immune signaling (37). Research indicates that lung microbiota can influence the development of cancer by regulating the host’s immune system (38, 39). Additionally, lung cancer patients often suffer from pulmonary bacterial infections. Alterations in the pulmonary bacterial community may impact immune surveillance, thereby affecting the growth and progression of lung cancer (29, 40). Consequently, understanding the clinical characteristics of bacterial lung infections in lung cancer patients and their relationship with cellular immunity offers valuable insights into how microbial communities affect the onset and progression of lung cancer through immune mechanisms.
In this study, the infection rate of Gram-negative bacteria was the highest, at 31.25% (170/544). Compared to other bacteria, Gram-negative bacteria dominated, which was consistent with the following research findings (16, 41). The first reason is the outer membrane of Gram-negative bacteria contains lipopolysaccharide (LPS). LPS not only protects bacteria from environmental stress, such as antibiotics and immune system attacks, but also has a strong immunostimulatory effect. This effect triggers a more severe inflammatory response (42, 43). Moreover, the pore proteins in the outer membrane have low selectivity and are difficult for antibiotics to penetrate. This makes Gram-negative bacteria more resistant to antibiotic treatment (44, 45). Among Gram-negative bacteria, Escherichia coli accounted for 91 cases, representing 16.73% (91/544), followed by Pseudomonas aeruginosa at 5.51% (30/544). Studies have shown that persistent infections by Gram-negative bacteria, such as Pseudomonas aeruginosa or Klebsiella pneumoniae, can lead to chronic inflammation. This inflammation produces a microenvironment. The microenvironment is conducive to cancer development as it promotes cell proliferation, angiogenesis, and immune evasion (46, 47). Therefore, the preventing and controlling Gram-negative bacterial infections and the managing antibiotic resistance are key focuses in the treatment of pulmonary infections.
Considering the high incidence rate of lung cancer-associated infection and its impact on patients’ survival rate, understanding the predictive factors of the development of lung cancer-associated infection is the first step for clinicians to establish a monitoring program. This investigation analyzed the risk factors for bacterial lung infection in patients with advanced lung adenocarcinoma and found that age, smoking, stage, bronchiectasis, diabetes, CD3+ T cell percentage, and CD4+ T cell count were closely related to bacterial lung infection. Smoking, bronchiectasis, and diabetes were determined to be predictive factors of bacterial infection in the lungs of patients with advanced lung adenocarcinoma. Advanced age has been shown to increase the risk of bacterial lung infection in patients with lung adenocarcinoma. These patients have weakened lung function, decreased resistance, and are thus more susceptible to bacterial invasion. The later the stage of lung cancer, the higher the risk and severity of bacterial lung infection. This association is caused by the impact of the tumor itself on the airway and immune system and the side effects of treatment measures, such as chemotherapy and radiotherapy (48, 49). Strengthening infection management and providing comprehensive treatment for lung cancer patients are important parts of improving survival rate and quality of life (50). Moreover, there is a close two-way interaction between bronchiectasis and lung infection in lung cancer patients. Bronchiectasis provides a good breeding ground for pathogens, increasing the risk of infection and the difficulty of treatment in lung cancer patients. Meanwhile, lung cancer and its treatment may aggravate the condition of bronchiectasis, forming a vicious cycle (51, 52). Effective airway management, infection control, and comprehensive treatment are the keys to improving patient prognosis. Furthermore, diabetes and lung cancer work together to significantly increase the incidence and complexity of lung infections. Diabetes greatly increases the susceptibility of lung cancer patients to infection through immune function suppression, a hyperglycemic environment, and metabolic disorders. Lung cancer and its treatment further increase the risk of infection (42). Therefore, preventing and controlling of pulmonary infection in patients with diabetes and lung cancer requires focusing on glycemic control, individualized anti-infective treatment and nutritional support. This is of great significance for improving the patient’s prognosis.
While both B and T lymphocytes play critical roles in the immune response against tumors, it is the T lymphocytes that are particularly effective in mediating cellular immunity against tumors (53, 54). T lymphocytes, the primary contributors, are essential for recognizing and targeting tumor cells. They help to prevent the growth, and spread of cancer (55). T lymphocytes are further classified into different types based on their functions. These types include delayed hypersensitivity T cells, killer T cells, cytotoxic T cells, and helper T cells. Each type of T lymphocyte plays a distinct role in the immune system’s defense mechanisms (56). Among these, CD4+ T cells, which are markers of helper T cells, are crucial for anti-tumor immunity. CD4+ T cells assist other immune cells in recognizing and responding to cancer cells. As a result, they amplify the immune response (57). On the other hand, CD8+ T cells include both cytotoxic T cells and suppressor T cells. Cytotoxic T cells are directly involved in killing tumor cells, while suppressor T cells can regulate immune responses by dampening excessive immune activity (58). In our results, the proportions of CD4+ cells and CD8+ cells were decreased. This suggests that bacterial infection can affect the immune function of patients with lung adenocarcinoma. Moreover, while both CD4+ and CD8+ T cells contribute to anti-tumor immunity, the balance between these two cell types is crucial for maintaining effective immune function. The results of the study showed that the ratio of CD4+ to CD8+ cells has decreased. In this study, we conducted a multivariate logistic regression analysis to explore the relationship between T lymphocytes and infection. The results revealed that T lymphocytes were not an independent factor influencing infection. This finding suggests that their role in immune defense may be more complex than previously thought. It implies that lung immune dysfunction does not directly lead to bacterial infections. Instead, it may be influenced by a combination of other factors. One such factor is imbalances in the respiratory flora, which could contribute to infection susceptibility (59). Thus, the interaction between immune dysfunction and environmental factors, such as the microbiome, may play a significant role in determining the overall immune response to infections and cancer.
Studies have shown that bacteria and their metabolites can directly act on T cells, interfere with their normal metabolism, and inhibit their proliferation (60). Bacterial pathogens use metabolic and molecular strategies to evade immune responses, including cytokine manipulation (e.g., Mycobacterium tuberculosis and Bordetella pertussis), metabolic interference (e.g., via short-chain fatty acids and pathogens like Salmonella), and commensal microbiota interactions (gut microbiota engaging with pattern recognition receptors) (10, 61, 62). ICIs, which block T-cell inhibitory receptors, have dual effects. They increase susceptibility to certain infections, such as disrupting M. tuberculosis control and triggering T-cell responses against skin microbiota (63). Immunosuppressive therapies for ICI-related side effects also heighten infection risks (64). Patients with advanced lung cancer often use immunosuppressant therapy and are prone to bacterial infections (63, 65). Therefore, analyzing different types of bacterial infections can provide guidance for adjusting immunosuppressant therapy. We found during data analysis that Gram-positive and Gram-negative bacteria may have different effects on the immune system of cancer patients. In lung adenocarcinoma patients treated with immune checkpoint inhibitors, those with Gram-positive bacterial pneumonia show higher CD4+ T cell counts and CD4+/CD8+ T ratios than those with Gram-negative infections. This finding underscores the need to study how these inhibitors affect cellular immunity and the role of bacteria. Many reports have paid attention to infection risk and prognosis caused by ICIs use (66, 67). In recent years, some research has still focused on how bacterial infections directly impact the efficacy of immune checkpoint inhibitors and the modulation of T cells. For example, mice were engrafted with MC38 colon adenocarcinoma or B16-OVA melanoma cells, the tumor volumes of non-H.pylori-infected mice undergoing anti-CTLA-4 and/or PD-1 or anti-cancer vaccine treatments were significantly smaller than those of H.pylori-infected mice. They observed a decreased number and activation status of tumor-specific CD8+ T cells in the tumors of infected mice treated with cancer immunotherapies independent of the gut microbiome composition (68). In more in-depth mechanism research, the immune checkpoint T-cell immunoglobulin and ITIM domain (TIGIT) was significantly upregulated on lymphocytes of S. Typhimurium-infected C57BL/6 mice, particularly on CD4+ T cells. This drastically limited their proinflammatory function (69). These studies and our results indicate that different bacterial infections can affect the use of immunosuppressants, but it is very difficult to explain the mechanism behind this phenomenon. Our findings suggest that immune checkpoint inhibitors may have better therapeutic effects in patients with Gram-positive infections, which can provide a reference for treatment strategies. Future research should focus on the specific impacts of ICIs on T cell subsets during Gram-positive and Gram-negative infections to clarify their roles. Despite the clinical success of immune checkpoint inhibitors, a significant subset of patients experiences immune-related adverse effects (irAEs) that can limit treatment efficacy and tolerability. Factors influencing ICI response and mechanisms of resistance, including immune dysregulation and host-specific variables, have been extensively reviewed (70). Understanding these mechanisms is crucial for optimizing patient selection and improving therapeutic strategies.
In conclusion, age, diabetes, and bronchiectasis are independent risk factors for pulmonary bacterial infections in patients with advanced lung adenocarcinoma. Understanding these risk factors is crucial for effective prevention, early diagnosis, and timely treatment in clinical practice. The effect of immune checkpoint inhibitor treatment on T cells is more pronounced in Gram positive bacteria. However, the sample size in this study is quite small, and no long-term follow-up was conducted. As a result, there may be potential bias in the findings. To confirm these results, further research involving a larger sample size and long-term follow-up through a multicenter study is needed.
The original contributions presented in the study are included in the article/Supplementary Material. Further inquiries can be directed to the corresponding author.
This retrospective study was approved by the Ethics Committee of The First Affiliated Hospital of Zhengzhou University. The studies were conducted in accordance with the local legislation and institutional requirements. Written informed consent for participation was not required from the participants or the participants’ legal guardians/next of kin in accordance with the national legislation and institutional requirements.
KY: Formal analysis, Writing – original draft. HTW: Formal analysis, Writing – review & editing. WZ: Data curation, Writing – review & editing. YX: Data curation, Writing – review & editing. SW: Data curation, Writing – review & editing. FF: Data curation, Writing – review & editing. KZ: Data curation, Writing – review & editing. QY: Data curation, Writing – review & editing. HMW: Funding acquisition, Supervision, Writing – review & editing.
The author(s) declare that financial support was received for the research and/or publication of this article. This study was funded by The Cymbidium Public Welfare-Hansoh Pharmaceutical Special Fund Project for Precision Medicine Scientific Research on Lung Cancer (No. HL-HS2020-105).
The authors declare that the research was conducted in the absence of any commercial or financial relationships that could be construed as a potential conflict of interest.
The author(s) declare that no Generative AI was used in the creation of this manuscript.
All claims expressed in this article are solely those of the authors and do not necessarily represent those of their affiliated organizations, or those of the publisher, the editors and the reviewers. Any product that may be evaluated in this article, or claim that may be made by its manufacturer, is not guaranteed or endorsed by the publisher.
The Supplementary Material for this article can be found online at: https://www.frontiersin.org/articles/10.3389/fimmu.2025.1559211/full#supplementary-material
1. Duan G, Huang C, Zhao J, Zhang Y, Zhao W, Dai H. Investigating subtypes of lung adenocarcinoma by oxidative stress and immunotherapy related genes. Sci Rep. (2023) . 13:20930. doi: 10.1038/s41598-023-47659-8
2. Wang W, Liu H, Li G. What’s the difference between lung adenocarcinoma and lung squamous cell carcinoma? Evidence from a retrospective analysis in a cohort of Chinese patients. Front Endocrinol (Lausanne). (2022) . 13:947443. doi: 10.3389/fendo.2022.947443
3. Li C, Lei S, Ding L, Xu Y, Wu X, Wang H, et al. Global burden and trends of lung cancer incidence and mortality. Chin Med J (Engl). (2023) . 136:1583–90. doi: 10.1097/cm9.0000000000002529
4. Sharma R. Mapping of global, regional and national incidence, mortality and mortality-to-incidence ratio of lung cancer in 2020 and 2050. Int J Clin Oncol. (2022) . 27:665–75. doi: 10.1007/s10147-021-02108-2
5. Kashyap P, Dutt N, Ahirwar DK, Yadav P. Lung microbiome in lung cancer: A new horizon in cancer study. Cancer Prev Res (Phila). (2024) . 17:401–14. doi: 10.1158/1940-6207.Capr-24-0147
6. McLean AEB, Kao SC, Barnes DJ, Wong KKH, Scolyer RA, Cooper WA, et al. The emerging role of the lung microbiome and its importance in non-small cell lung cancer diagnosis and treatment. Lung Cancer. (2022) . 165:124–32. doi: 10.1016/j.lungcan.2022.01.011
7. Zhao Y, Liu Y, Li S, Peng Z, Liu X, Chen J, et al. Role of lung and gut microbiota on lung cancer pathogenesis. J Cancer Res Clin Oncol. (2021) . 147:2177–86. doi: 10.1007/s00432-021-03644-0
8. Li R, Li J, Zhou X. Lung microbiome: new insights into the pathogenesis of respiratory diseases. Signal Transduct Target Ther. (2024) . 9:19. doi: 10.1038/s41392-023-01722-y
9. Sommariva M, Le Noci V, Bianchi F, Camelliti S, Balsari A, Tagliabue E, et al. The lung microbiota: role in maintaining pulmonary immune homeostasis and its implications in cancer development and therapy. Cell Mol Life Sci. (2020) . 77:2739–49. doi: 10.1007/s00018-020-03452-8
10. Zheng D, Liwinski T, Elinav E. Interaction between microbiota and immunity in health and disease. Cell Res. (2020) . 30:492–506. doi: 10.1038/s41422-020-0332-7
11. Liu NN, Ma Q, Ge Y, Yi CX, Wei LQ, Tan JC, et al. Microbiome dysbiosis in lung cancer: from composition to therapy. NPJ Precis Oncol. (2020) . 4:33. doi: 10.1038/s41698-020-00138-z
12. Xu N, Wang L, Li C, Ding C, Li C, Fan W, et al. Microbiota dysbiosis in lung cancer: evidence of association and potential mechanisms. Transl Lung Cancer Res. (2020) . 9:1554–68. doi: 10.21037/tlcr-20-156
13. Tsay JJ, Wu BG, Sulaiman I, Gershner K, Schluger R, Li Y, et al. Lower airway dysbiosis affects lung cancer progression. Cancer Discovery. (2021) . 11:293–307. doi: 10.1158/2159-8290.Cd-20-0263
14. Budisan L, Zanoaga O, Braicu C, Pirlog R, Covaliu B, Esanu V, et al. Links between infections, lung cancer, and the immune system. Int J Mol Sci. (2021) 22(17):9394. doi: 10.3390/ijms22179394
15. Zhou X, Kandalai S, Hossain F, Zheng Q. Tumor microbiome metabolism: A game changer in cancer development and therapy. Front Oncol. (2022) . 12:933407. doi: 10.3389/fonc.2022.933407
16. Cheng J, Zhou L, Wang H. Symbiotic microbial communities in various locations of the lung cancer respiratory tract along with potential host immunological processes affected. Front Cell Infect Microbiol. (2024) . 14:1296295. doi: 10.3389/fcimb.2024.1296295
17. D’Anna SE, Maniscalco M, Cappello F, Carone M, Motta A, Balbi B, et al. Bacterial and viral infections and related inflammatory responses in chronic obstructive pulmonary disease. Ann Med. (2021) . 53:135–50. doi: 10.1080/07853890.2020.1831050
18. Marrella V, Nicchiotti F, Cassani B. Microbiota and immunity during respiratory infections: lung and gut affair. Int J Mol Sci. (2024) 25(7):4051. doi: 10.3390/ijms25074051
19. Pettigrew MM, Tanner W, Harris AD. The lung microbiome and pneumonia. J Infect Dis. (2021) 223:S241–s45. doi: 10.1093/infdis/jiaa702
20. Patel AJ, Nightingale P, Naidu B, Drayson MT, Middleton GW, Richter A. Characterising the impact of pneumonia on outcome in non-small cell lung cancer: identifying preventative strategies. J Thorac Dis. (2020) . 12:2236–46. doi: 10.21037/jtd.2020.04.49
21. Weinberg FD, Zhao L, Chellappa N, Kalemkerian GP, Ramnath N. Characterization of pneumonia and other factors leading to poorer survival across all age groups in patients with non-small cell lung cancer (NSCLC). J Thorac Dis. (2021) . 13:986–94. doi: 10.21037/jtd-20-2891
22. Valvani A, Martin A, Devarajan A, Chandy D. Postobstructive pneumonia in lung cancer. Ann Transl Med. (2019) . 7:357. doi: 10.21037/atm.2019.05.26
23. Wong JL, Evans SE. Bacterial pneumonia in patients with cancer: novel risk factors and management. Clin Chest Med. (2017) . 38:263–77. doi: 10.1016/j.ccm.2016.12.005
24. Ma T, Tang Y, Wang T, Yang Y, Zhang Y, Wang R, et al. Chronic pulmonary bacterial infection facilitates breast cancer lung metastasis by recruiting tumor-promoting MHCII(hi) neutrophils. Signal Transduct Target Ther. (2023) . 8:296. doi: 10.1038/s41392-023-01542-0
25. Nakano-Narusawa Y, Yokohira M, Yamakawa K, Ye J, Tanimoto M, Wu L, et al. Relationship between lung carcinogenesis and chronic inflammation in rodents. Cancers (Basel). (2021) 13(12):2910. doi: 10.3390/cancers13122910
26. Wen Y, Zhu Y, Zhang C, Yang X, Gao Y, Li M, et al. Chronic inflammation, cancer development and immunotherapy. Front Pharmacol. (2022) . 13:1040163. doi: 10.3389/fphar.2022.1040163
27. Li Q. Bacterial infection and microbiota in carcinogenesis and tumor development. Front Cell Infect Microbiol. (2023) . 13:1294082. doi: 10.3389/fcimb.2023.1294082
28. Schorr L, Mathies M, Elinav E, Puschhof J. Intracellular bacteria in cancer-prospects and debates. NPJ Biofilms Microb. (2023) . 9:76. doi: 10.1038/s41522-023-00446-9
29. Cao Q, Wu X, Chen Y, Wei Q, You Y, Qiang Y, et al. The impact of concurrent bacterial lung infection on immunotherapy in patients with non-small cell lung cancer: a retrospective cohort study. Front Cell Infect Microbiol. (2023) . 13:1257638. doi: 10.3389/fcimb.2023.1257638
30. Zhang D, Jin J, Dou J, Huang Y, Zhang H. Impact on hospitalization and infection patterns of advanced lung cancer with lower respiratory tract infections: Targeted therapy vs. chemoradiother Oncol Lett. (2024) . 27:154. doi: 10.3892/ol.2024.14287
31. Benusiglio PR, Fallet V, Sanchis-Borja M, Coulet F, Cadranel J. Lung cancer is also a hereditary disease. Eur Respir Rev. (2021) 30(162):210045. doi: 10.1183/16000617.0045-2021
32. Chalela R, Curull V, Enríquez C, Pijuan L, Bellosillo B, Gea J. Lung adenocarcinoma: from molecular basis to genome-guided therapy and immunotherapy. J Thorac Dis. (2017) . 9:2142–58. doi: 10.21037/jtd.2017.06.20
33. Hill W, Lim EL, Weeden CE, Lee C, Augustine M, Chen K, et al. Lung adenocarcinoma promotion by air pollutants. Nature. (2023) . 616:159–67. doi: 10.1038/s41586-023-05874-3
34. Dong Q, Chen ES, Zhao C, Jin C. Host-microbiome interaction in lung cancer. Front Immunol. (2021) . 12:679829. doi: 10.3389/fimmu.2021.679829
35. Zong Z, Zhou F, Zhang L. The fungal mycobiome: a new hallmark of cancer revealed by pan-cancer analyses. Signal Transduct Target Ther. (2023) . 8:50. doi: 10.1038/s41392-023-01334-6
36. Paudel KR, Dharwal V, Patel VK, Galvao I, Wadhwa R, Malyla V, et al. Role of lung microbiome in innate immune response associated with chronic lung diseases. Front Med (Lausanne). (2020) . 7:554. doi: 10.3389/fmed.2020.00554
37. Zhou J, Hou W, Zhong H, Liu D. Lung microbiota: implications and interactions in chronic pulmonary diseases. Front Cell Infect Microbiol. (2024) . 14:1401448. doi: 10.3389/fcimb.2024.1401448
38. Belaid A, Roméo B, Rignol G, Benzaquen J, Audoin T, Vouret-Craviari V, et al. Impact of the lung microbiota on development and progression of lung cancer. Cancers (Basel). (2024) 16(19):3342. doi: 10.3390/cancers16193342
39. Ramírez-Labrada AG, Isla D, Artal A, Arias M, Rezusta A, Pardo J, et al. The influence of lung microbiota on lung carcinogenesis, immunity, and immunotherapy. Trends Cancer. (2020) . 6:86–97. doi: 10.1016/j.trecan.2019.12.007
40. Belluomini L, Caldart A, Avancini A, Dodi A, Trestini I, Kadrija D, et al. Infections and immunotherapy in lung cancer: A bad relationship? Int J Mol Sci. (2020) 22(1):42. doi: 10.3390/ijms22010042
41. Liu H, Liu B, Zheng F, Chen X, Ye L, He Y. Distribution of pathogenic bacteria in lower respiratory tract infection in lung cancer patients after chemotherapy and analysis of integron resistance genes in respiratory tract isolates of uninfected patients. J Thorac Dis. (2020) . 12:4216–23. doi: 10.21037/jtd-20-928
42. Bertani B, Ruiz N. Function and biogenesis of lipopolysaccharides. EcoSal Plus. (2018) 8(1):10. doi: 10.1128/ecosalplus.ESP-0001-2018
43. Garcia-Vello P, Di Lorenzo F, Zucchetta D, Zamyatina A, De Castro C, Molinaro A. Lipopolysaccharide lipid A: A promising molecule for new immunity-based therapies and antibiotics. Pharmacol Ther. (2022) . 230:107970. doi: 10.1016/j.pharmthera.2021.107970
44. Choi U, Lee CR. Distinct roles of outer membrane porins in antibiotic resistance and membrane integrity in escherichia coli. Front Microbiol. (2019) . 10:953. doi: 10.3389/fmicb.2019.00953
45. Zhou G, Wang Q, Wang Y, Wen X, Peng H, Peng R, et al. Outer membrane porins contribute to antimicrobial resistance in gram-negative bacteria. Microorganisms. (2023) 11(7):1690. doi: 10.3390/microorganisms11071690
46. Comen EA, Bowman RL, Kleppe M. Underlying causes and therapeutic targeting of the inflammatory tumor microenvironment. Front Cell Dev Biol. (2018) . 6:56. doi: 10.3389/fcell.2018.00056
47. Sun M, Bai Y, Zhao S, Liu X, Gao Y, Wang L, et al. Gram-negative bacteria facilitate tumor progression through TLR4/IL-33 pathway in patients with non-small-cell lung cancer. Oncotarget. (2018) . 9:13462–73. doi: 10.18632/oncotarget.24008
48. Bertaglia V, Morelli AM, Solinas C, Aiello MM, Manunta S, Denaro N, et al. Infections in lung cancer patients undergoing immunotherapy and targeted therapy: An overview on the current scenario. Crit Rev Oncol Hematol. (2023) . 184:103954. doi: 10.1016/j.critrevonc.2023.103954
49. Jang HJ, Park MS, Kim YS, Chang J, Lee JH, Lee CT, et al. The relationship between the severity of pulmonary fibrosis and the lung cancer stage. J Cancer. (2021) . 12:2807–14. doi: 10.7150/jca.51445
50. Petrella F, Rizzo S, Attili I, Passaro A, Zilli T, Martucci F, et al. Stage III non-small-cell lung cancer: an overview of treatment options. Curr Oncol. (2023) . 30:3160–75. doi: 10.3390/curroncol30030239
51. Choi H, Park HY, Han K, Yoo J, Shin SH, Yang B, et al. Non-cystic fibrosis bronchiectasis increases the risk of lung cancer independent of smoking status. Ann Am Thorac Soc. (2022) . 19:1551–60. doi: 10.1513/AnnalsATS.202111-1257OC
52. Kim YW. Complex relationship between bronchiectasis and lung cancer. Ann Am Thorac Soc. (2022) . 19:1455–56. doi: 10.1513/AnnalsATS.202206-484ED
53. Waldman AD, Fritz JM, Lenardo MJ. A guide to cancer immunotherapy: from T cell basic science to clinical practice. Nat Rev Immunol. (2020) . 20:651–68. doi: 10.1038/s41577-020-0306-5
54. Zeng Z, Chew HY, Cruz JG, Leggatt GR, Wells JW. Investigating T cell immunity in cancer: achievements and prospects. Int J Mol Sci. (2021) 22(6):2907. doi: 10.3390/ijms22062907
55. Want MY, Bashir Z, Najar RA. T cell based immunotherapy for cancer: approaches and strategies. Vaccines (Basel). (2023) 11(4):835. doi: 10.3390/vaccines11040835
56. Raskov H, Orhan A, Christensen JP, Gögenur I. Cytotoxic CD8(+) T cells in cancer and cancer immunotherapy. Br J Cancer. (2021) . 124:359–67. doi: 10.1038/s41416-020-01048-4
57. Kravtsov DS, Erbe AK, Sondel PM, Rakhmilevich AL. Roles of CD4+ T cells as mediators of antitumor immunity. Front Immunol. (2022) . 13:972021. doi: 10.3389/fimmu.2022.972021
58. Weigelin B, den Boer AT, Wagena E, Broen K, Dolstra H, de Boer RJ, et al. Cytotoxic T cells are able to efficiently eliminate cancer cells by additive cytotoxicity. Nat Commun. (2021) . 12:5217. doi: 10.1038/s41467-021-25282-3
59. Guo MY, Chen HK, Ying HZ, Qiu FS, Wu JQ. The role of respiratory flora in the pathogenesis of chronic respiratory diseases. BioMed Res Int. (2021) . 2021:6431862. doi: 10.1155/2021/6431862
60. Mohseni AH, Taghinezhad SS, Casolaro V, Lv Z, Li D. Potential links between the microbiota and T cell immunity determine the tumor cell fate. Cell Death Dis. (2023) . 14:154. doi: 10.1038/s41419-023-05560-2
61. Hornef MW, Wick MJ, Rhen M, Normark S. Bacterial strategies for overcoming host innate and adaptive immune responses. Nat Immunol. (2002) . 3:1033–40. doi: 10.1038/ni1102-1033
62. Kogut MH, Lee A, Santin E. Microbiome and pathogen interaction with the immune system. Poult Sci. (2020) . 99:1906–13. doi: 10.1016/j.psj.2019.12.011
63. Papadakis M, Karniadakis I, Mazonakis N, Akinosoglou K, Tsioutis C, Spernovasilis N. Immune checkpoint inhibitors and infection: what is the interplay? In Vivo. (2023) . 37:2409–20. doi: 10.21873/invivo.13346
64. Hu ZI, Link VM, Lima-Junior DS, Delaleu J, Bouladoux N, Han SJ, et al. Immune checkpoint inhibitors unleash pathogenic immune responses against the microbiota. Proc Natl Acad Sci U.S.A. (2022) . 119:e2200348119. doi: 10.1073/pnas.2200348119
65. Yado S, Dassa B, Zoabi R, Reich-Zeliger S, Friedman N, Geiger B. Molecular mechanisms underlying the modulation of T-cell proliferation and cytotoxicity by immobilized CCL21 and ICAM1. J Immunother Cancer. (2024) 12(6):e009011. doi: 10.1136/jitc-2024-009011
66. Kim HW, Kim JS, Lee SH. Incidence of tuberculosis in advanced lung cancer patients treated with immune checkpoint inhibitors - A nationwide population-based cohort study. Lung Cancer. (2021) . 158:107–14. doi: 10.1016/j.lungcan.2021.05.034
67. Qin Y, Chen Y, Chen J, Xu K, Xu F, Shi J. The relationship between previous pulmonary tuberculosis and risk of lung cancer in the future. Infect Agent Cancer. (2022) . 17:20. doi: 10.1186/s13027-022-00434-2
68. Oster P, Vaillant L, Riva E, McMillan B, Begka C, Truntzer C, et al. Helicobacter pylori infection has a detrimental impact on the efficacy of cancer immunotherapies. Gut. (2022) . 71:457–66. doi: 10.1136/gutjnl-2020-323392
69. McCulloch TR, Rossi GR, Miranda-Hernandez S, Valencia-Hernandez AM, Alim L, Belle CJ, et al. The immune checkpoint TIGIT is upregulated on T cells during bacterial infection and is a potential target for immunotherapy. Immunol Cell Biol. (2024) . 102:721–33. doi: 10.1111/imcb.12794
Keywords: lung adenocarcinoma, bacterial pulmonary infection, immune function, microbial infection, immune checkpoint inhibitors
Citation: Yang K, Wei H, Zhu W, Xu Y, Wang S, Fan F, Zhang K, Yuan Q and Wang H (2025) Clinical characteristics and risk factors of late-stage lung adenocarcinoma patients with bacterial pulmonary infection and its relationship with cellular immune function. Front. Immunol. 16:1559211. doi: 10.3389/fimmu.2025.1559211
Received: 12 January 2025; Accepted: 28 March 2025;
Published: 16 April 2025.
Edited by:
Beiwen Zheng, Zhejiang University, ChinaReviewed by:
Wenxue Ma, University of California, San Diego, United StatesCopyright © 2025 Yang, Wei, Zhu, Xu, Wang, Fan, Zhang, Yuan and Wang. This is an open-access article distributed under the terms of the Creative Commons Attribution License (CC BY). The use, distribution or reproduction in other forums is permitted, provided the original author(s) and the copyright owner(s) are credited and that the original publication in this journal is cited, in accordance with accepted academic practice. No use, distribution or reproduction is permitted which does not comply with these terms.
*Correspondence: Hongmin Wang, ZmNjd2FuZ2htQHp6dS5lZHUuY24=
†These authors have contributed equally to this work and share first authorship
Disclaimer: All claims expressed in this article are solely those of the authors and do not necessarily represent those of their affiliated organizations, or those of the publisher, the editors and the reviewers. Any product that may be evaluated in this article or claim that may be made by its manufacturer is not guaranteed or endorsed by the publisher.
Research integrity at Frontiers
Learn more about the work of our research integrity team to safeguard the quality of each article we publish.