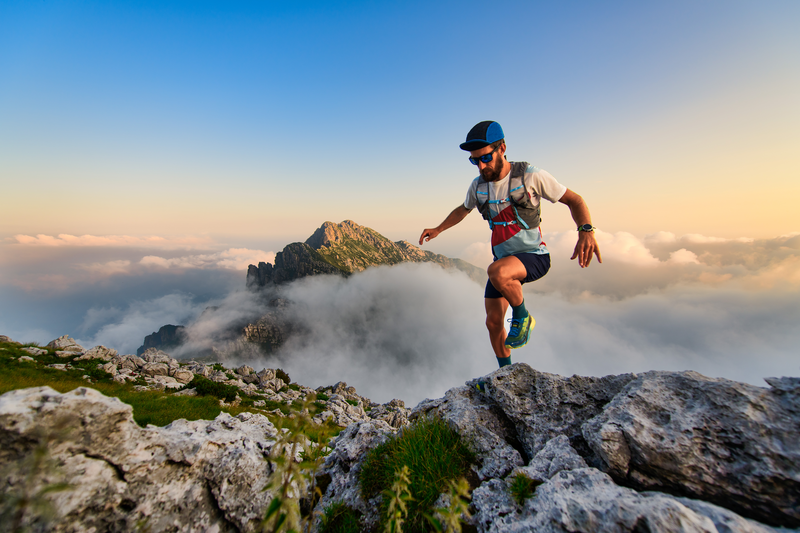
94% of researchers rate our articles as excellent or good
Learn more about the work of our research integrity team to safeguard the quality of each article we publish.
Find out more
ORIGINAL RESEARCH article
Front. Immunol.
Sec. Inflammation
Volume 16 - 2025 | doi: 10.3389/fimmu.2025.1558903
This article is part of the Research Topic Harnessing Big Data for Precision Medicine: Revolutionizing Diagnosis and Treatment Strategies View all 29 articles
The final, formatted version of the article will be published soon.
You have multiple emails registered with Frontiers:
Please enter your email address:
If you already have an account, please login
You don't have a Frontiers account ? You can register here
Pulmonary fibrosis (PF) is a fatal pathological subtype of interstitial lung disease, frequently manifests as a pulmonary complication of connective tissue disease. Iguratimod (IGU) is a new class of anti-rheumatic drugs used in the treatment of rheumatoid arthritis (RA). Studies have reported that RA patients treated with IGU have better lung function, and IGU effectively ameliorates PF. However, the mechanism by which IGU improves PF is still unclear. This study aims to elucidate the therapeutic efficacy and mechanisms of IGU in PF through in vivo and in vitro investigations, so as to provide a new treatment method for PF. In our research, bleomycin (BLM)-induced PF of mice were used to observe the therapeutic effect of different concentrations of IGU. And the effects of IGU on macrophage polarization and activation pathway TLR4/NF-κB in lung tissue were analyzed. In addition, Raw264.7 macrophages were induced to M1 and M2 polarization in vitro, and the effects of IGU on Raw264.7 macrophage polarization and related pathways were observed. In our study, database analysis suggested that macrophage polarization-relative genes and pathways as well as TLR4 activation played important roles in BLM-induced PF in mice. Besides, we found that IGU effectively ameliorated BLM-induced PF and epithelial-mesenchymal transition in mice, and inhibited the polarization of M1/M2 macrophages at different stages of PF. Moreover, In vitro studies further demonstrated that IGU suppressed M1 polarization of Raw264.7 and its activation pathway TLR4/NF-κB. In summary, IGU inhibits the activation of macrophages and M1 polarization through inhibiting the TLR4/NF-κB pathway, thereby improving BLM-induced pulmonary inflammation and fibrosis in mice. It is suggested that IGU may be a new therapeutic option for interstitial pulmonary fibrosis.
Keywords: Interstitial pulmonary fibrosis, Inflammation, Iguratimod, M1 polarization, TLR4
Received: 11 Jan 2025; Accepted: 28 Feb 2025.
Copyright: © 2025 Xu, Ma, Ma, Zhuang and Lin. This is an open-access article distributed under the terms of the Creative Commons Attribution License (CC BY). The use, distribution or reproduction in other forums is permitted, provided the original author(s) or licensor are credited and that the original publication in this journal is cited, in accordance with accepted academic practice. No use, distribution or reproduction is permitted which does not comply with these terms.
* Correspondence:
Ling Lin, First Affiliated Hospital of Shantou University Medical College, Shantou, China
Disclaimer: All claims expressed in this article are solely those of the authors and do not necessarily represent those of their affiliated organizations, or those of the publisher, the editors and the reviewers. Any product that may be evaluated in this article or claim that may be made by its manufacturer is not guaranteed or endorsed by the publisher.
Research integrity at Frontiers
Learn more about the work of our research integrity team to safeguard the quality of each article we publish.