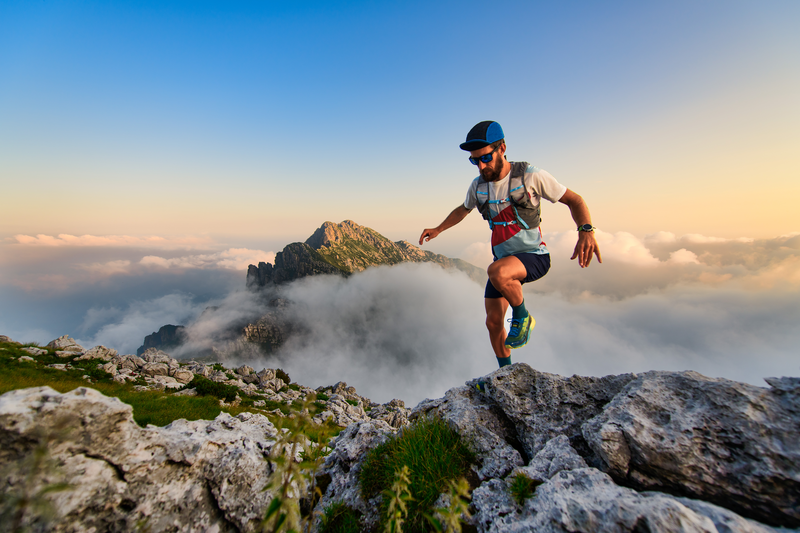
94% of researchers rate our articles as excellent or good
Learn more about the work of our research integrity team to safeguard the quality of each article we publish.
Find out more
ORIGINAL RESEARCH article
Front. Immunol.
Sec. Inflammation
Volume 16 - 2025 | doi: 10.3389/fimmu.2025.1558596
This article is part of the Research Topic Understanding Chronic Inflammation: Mechanisms Behind Its Persistence View all articles
The final, formatted version of the article will be published soon.
You have multiple emails registered with Frontiers:
Please enter your email address:
If you already have an account, please login
You don't have a Frontiers account ? You can register here
Atopic dermatitis (AD) is a chronic inflammatory skin disease characterized by pruritus, immune dysregulation, and compromised skin barrier function. Although there are some reports that indicate a link between AD and chronic kidney disease (CKD), the prevalence and underlying mechanism of the association between AD and CKD are still unclear. We aimed to clarify the mechanism underlying the association between AD and CKD using an AD-like mouse model. Human serum and urine samples from adults in the U.S. were analyzed using data from the National Health and Nutrition Examination Survey (NHANES). An AD-like mouse model was established by repeatedly applying 2,4-dinitrochlorobenzene to the backs and ears of the mice. Kidney inflammation and podocyte function were evaluated via PAS and H&E staining, immunofluorescence staining, and electron microscopy. We found that compared to healthy subjects in the NHANES cohort study, patients with AD had altered kidney function. AD-like model mice exhibited albuminuria and renal dysfunction one to three months after the induction of AD. In addition, there were remarkable decreases in triglyceride and very-low-density lipoprotein levels and increases in low-density lipoprotein and non-high-density lipoprotein levels in AD-like model mice. After histological staining of the kidneys of AD-like model mice, macrophage and neutrophil infiltration was detected, and the foot process effacement of podocytes was observed via electron microscopy. In addition, the gene expression of slit diaphragm- and podocyte-related proteins such as nephrin, podocin, and synaptopodin decreased, whereas the gene expression of inflammatory mediators such as S100A8 and S100A9 increased. Following improvements in skin inflammation, alleviation of albuminuria, renal dysfunction and dyslipidemia were observed. These findings suggest that AD-related cutaneous inflammation is associated with albuminuria and podocyte dysfunction.
Keywords: Atopic dermatitis (AD), Albuminuria, Dyslipidemia, Inflammation, podocyte, renal dysfunction, S100A calcium-binding protein
Received: 10 Jan 2025; Accepted: 03 Mar 2025.
Copyright: © 2025 Ikeda, Peng, Zhao, Abudouwanli, Ikeda, Niyonsaba and Suzuki. This is an open-access article distributed under the terms of the Creative Commons Attribution License (CC BY). The use, distribution or reproduction in other forums is permitted, provided the original author(s) or licensor are credited and that the original publication in this journal is cited, in accordance with accepted academic practice. No use, distribution or reproduction is permitted which does not comply with these terms.
* Correspondence:
Francois Niyonsaba, Atopy/Allergy Research Center, Juntendo University Graduate School of Medicine, Tokyo, Japan
Yusuke Suzuki, Department of Nephrology, Juntendo University Graduate School of Medicine, Tokyo, Japan
Disclaimer: All claims expressed in this article are solely those of the authors and do not necessarily represent those of their affiliated organizations, or those of the publisher, the editors and the reviewers. Any product that may be evaluated in this article or claim that may be made by its manufacturer is not guaranteed or endorsed by the publisher.
Research integrity at Frontiers
Learn more about the work of our research integrity team to safeguard the quality of each article we publish.