- 1Division of Pediatric Infectious Diseases, Department of Pediatrics, University of Pittsburgh School of Medicine, Pittsburgh, PA, United States
- 2Department of Pediatrics, Division of Blood and Marrow Transplantation and Cellular Therapies, University of Pittsburgh School of Medicine, Pittsburgh, PA, United States
- 3Department of Pediatrics, Division of Blood and Marrow Transplantation & Cellular Therapy, University of Minnesota Medical School, Minneapolis, MN, United States
- 4Division of Allergy and Immunology, Department of Pediatrics, University of Pittsburgh School of Medicine, Pittsburgh, PA, United States
- 5Department of Pediatrics, University of Pittsburgh Medical Center (UPMC) Children’s Hospital of Pittsburgh, Pittsburgh, PA, United States
Introduction: Bacterial pneumonia is a burdensome, costly disease and increasingly challenging to treat due to antibiotic resistance. Complex host-pathogen interactions regulate protective immunity. Neutrophils play a central role in pulmonary bacterial immunity, and mechanistic understanding of neutrophil functions in bacterial pneumonia has potential clinical and fundamental application. Nuclear receptor 4a1 (Nr4a1), a member of the nuclear orphan receptor family, has been described to regulate inflammation and immune development in a cell type-specific manner, but its role in pulmonary host defense is not well understood.
Methods: Wild-type (WT) and Nr4a1-/- mice, as well as bone marrow chimeric and Gr-1+ antibody depleted mice, were infected with Klebsiella pneumoniae and assessed for bacterial burden in the lung and spleen, gene transcription, protein levels, histology and cellular abundance by flow cytometry in the lung. WT and Nr4a1-/- neutrophils were exposed to live Klebsiella pneumoniae to quantify bacterial killing, as well as bulk RNA sequencing to assess transcriptomic differences.
Results: Nr4a1-deficient mice are highly susceptible to Klebsiella pneumoniae pneumonia, which was mediated by Nr4a1 expression in immune cells. Gr-1+ antibody depletion ameliorated the Nr4a1-dependent phenotype. Ex vivo, Nr4a1-deficient neutrophils had impaired bactericidal capacity, and transcriptomic analysis identified an Nr4a1-dependent host defense program in neutrophils.
Discussion: Neutrophil Nr4a1 expression is critical for defense against K. pneumoniae infection by regulating the neutrophil transcriptome. These findings suggest targeting Nr4a1 signaling pathways in neutrophils may be useful for bacterial pneumonia treatment.
Introduction
Pneumonia is the leading cause of death among children worldwide, responsible for 15% of overall child mortality, surpassed only by prematurity (1). In the US, pneumonia is the most common infectious cause of hospitalization and death, with annual costs in excess of $17 billion (2–5). While antibiotics are the standard treatment for bacterial pneumonia, antibiotic resistance (AR) (6–9) is increasingly common, and AR pathogen treatment is associated with increased financial costs, morbidity and mortality (10–12). Klebsiella pneumoniae, a common cause of hospital-acquired pneumonia, is a U.S. Centers for Disease Control and Prevention-defined “Urgent Threat” to public health due to its virulence and increasing rate of antibiotic resistance (12). Improved treatments for AR pneumonia are urgently needed. The pathophysiology of pneumonia involves complex host-pathogen interactions, and insight into the critical mediators of host immunity holds the promise for developing immune-targeting treatments as novel therapeutic strategies, approaches that have transformed the fields of cancer biology and autoimmunity.
Neutrophils and macrophages are key myeloid immune populations in pulmonary antibacterial immunity, but the cell-specific molecular mechanisms remain only partially understood (13, 14). Nuclear receptor 4a1 (Nr4a1 or Nur77) is a steroid thyroid receptor family member of the orphan nuclear receptor subfamily 4a. Nr4a1, Nr4a2 (Nurr1), and Nr4a3 (NOR-1), have been shown to regulate homeostatic myeloid cell development. Prior studies have shown that Nr4a1 modulates macrophage and T cell-mediated inflammation as well as modulating metabolic pathways in the context of autoimmunity and oncology to constrain proinflammatory signaling and promote tolerance via transcriptional and epigenetic regulation (15–26). Few studies have examined the role of Nr4a1 in pulmonary host defense, specifically in the context of common bacterial pathogens. Thus, we sought to investigate whether Nr4a1 was critical for pulmonary immunity to pneumonia using the murine model of Klebsiella pneumoniae (15, 17, 22, 26–28). This work identified that Nr4a1 deficiency reduced survival, increased lung bacterial burden, and enhanced bacterial dissemination. We further observed that neutrophil-specific Nr4a1 is critical for defense against K. pneumoniae by regulating a defense response transcriptional program. These findings suggest that targeting neutrophil-specific Nr4a1 could offer a promising avenue for developing novel immunotherapies against bacterial pneumonia.
Methods
Animals
All animal protocols were approved by the University of Pittsburgh Institutional Animal Care and Use Committee (IACUC, protocols #22061470 and #23104015), and all experiments were conducted in accordance with the guidelines and regulations set forth in the Animal Welfare Act (AWA) and PHS Policy on Humane Care and Use of Laboratory Animals. WT C57BL/6J and Nr4a1-/- (B6;129S2-Nr4a1tm1Jmi/J) mice were purchased from the Jackson Laboratory. Age- and sex-matched male and female mice between 8-12 weeks old at the beginning of experiments were used for this study. Ages of BM donor mice ranged between 8-16 weeks old.
Klebsiella pneumoniae infection/bacterial burden analysis
In vivo infection of K. pneumoniae 396 (Kp396), a hypervirulent mucoid clinical isolate (29), via intratracheal injection and bacterial burden quantification in left lung lobe and whole spleen were performed as described previously (30) except that each animal received Kp396 at 5,000 – 10,000 CFU.
Histology
Lungs were fixed in 4% paraformaldehyde and embedded in paraffin and sectioned at 4 µm. Lung sections were stained with hematoxylin and eosin (H&E). H&E-stained slides were scored for bacterial burden in a blinded fashion with respect to neutrophil infiltration in alveoli, neutrophil infiltration in interstitial, hyaline membrane, alveolar wall thickening, and abscesses (Supplementary Table S1) (31).
Quantitative PCR
Right upper lung lobes were processed for qPCR as described previously (30). See Supplementary Table S2 for primer assay information.
Flow cytometry
Right middle and lower lung lobes were processed and stained for flow cytometry as described previously (30). See Supplementary Table S3 for antibody information.
Enzyme-linked immunosorbent assay
Lung homogenates were subjected to anti-IL-1β (ThermoFisher Scientific 88-7013-22) and anti-MPO (R&D DY3667) by ELISA as described previously (30).
Bone marrow chimera generation
WT and Nr4a1-/- mice were conditioned with 1100 cGy total body irradiation in a split dose of 550 cGy 3-4 hours apart from an X-ray source (X-rad 320; Precision X-ray, North Branford, CT), followed by intravenous infusion of 5x106 WT or Nr4a1-/- bone marrow (BM) cells. Transplanted mice were housed in sterile microisolator cages. BM cells were allowed to engraft for 8 weeks prior to infection.
Isolation of primary BM neutrophils and macrophages/K. pneumoniae killing assay
Primary neutrophils were isolated from WT and Nr4a1-/- BM using 40/70% Percoll gradient (Fisher) following red blood cell lysis with increasing NaCl concentrations (0.2% followed by 1.6%). For BM macrophage isolation, red blood cell lysed BM cells with ACK lysis buffer (Gibco) was cultured for 7 days at 37 °C in 5.0% CO2 and DMEM media supplemented with 10% fetal bovine albumin (FBS), 100 U/ml penicillin, 100 μg/ml streptomycin, 292 μg/ml L-glutamine (all Gibco), and 20 ng/mL M-CSF (Life Technologies). Isolated neutrophils and macrophages were incubated with Kp396 at an MOI of 10 in the killing assay media (IMDM media supplemented with 10% FBS, 292 μg/ml L-glutamine, 1x MEM non-essential amino acids, 10 mM HEPES, and 1 mM sodium pyruvate (all Gibco)) for 1 hour in the 37 °C with 5.0% CO2 on an orbital shaker at 250 rpm. Resulting supernatant was subjected to bacterial burden analysis as described above. Remaining cells were stored for gene expression analysis.
RNA sequencing
Primary neutrophils were isolated via negative magnetic selection from WT and Nr4a1-/- BM using EasySep™ Mouse Neutrophil Enrichment Kit (StemCell Technologies) following manufacturer’s instructions. Cells were incubated with or without Kp396 at a MOI of 0.4 in the killing assay media (IMDM media supplemented with 10% FBS, 292 μg/ml L-glutamine, 1x MEM non-essential amino acids, 10 mM HEPES, and 1 mM sodium pyruvate (all Gibco)) for 30 minutes at 37 °C prior to RNA isolation using RNeasy Micro Kit (Qiagen).
RNA was assessed for quality using an Agilent TapeStation 4150/Fragment Analyzer 5300 and RNA concentration was quantified on a Qubit FLEX fluorometer. Libraries were generated with the Illumina Stranded mRNA Library Prep kit (Illumina: 20040534) according to the manufacturer’s instructions. Briefly, 50 ng of input RNA was used for each sample. Following adapter ligation, 15 cycles of indexing PCR were completed, Illumina RNA UD Indexes. Library quantification and assessment was done using a Qubit FLEX fluorometer and an Agilent TapeStation 4150/Fragment Analyzer 5300. Libraries were normalized and pooled to 2 nM by calculating the concentration based off the fragment size (base pairs) and the concentration (ng/μl) of the libraries.
Sequencing was performed on an Illumina NextSeq 2000, using a P2 flow cell. The pooled library was loaded at 750 pM and sequencing was carried out with read lengths of 58 bp, with a target of 30 million reads per sample. Sequencing data was demultiplexed by the on-board Illumina DRAGEN FASTQ Generation software (v3.10.12).
Raw reads underwent pre-alignment quality control (QC) and trimming. Reads were then aligned using STAR (2.7.8a) via Partek Flow Bioinformatics software. Transcript normalization and gene expression analysis were performed using DESeq2 with FDR step-up used for multiple test correction. Hierarchical clustering was performed in both supervised and unsupervised manners by genotype/treatment. Gene set enrichment analysis using gene ontogeny terms were generated by significant hits (FDR<0.001).
RNA-seq data has been deposited at the Gene Expression Omnibus (GEO) database (accession code: IN PROGRESS).
Results
Nr4a1-deficient mice are highly susceptible to K. pneumoniae pneumonia
To evaluate the role of Nr4a1 in pulmonary infection, wild-type (WT) and Nr4a1-/- mice were infected with a clinical isolate of Klebsiella pneumoniae (Kp396) (29). We found that Nr4a1 transcription increased in WT mice following K. pneumoniae infection while Nr4a1-/- mice had no detectable Nr4a1 gene transcription (Supplementary Figure S1A). Nr4a1-/- mice exhibited a significantly increased bacterial burden in the lungs compared to WT mice (Figure 1A). Additionally, there was a significantly higher bacterial burden in the spleens of Nr4a1-/- mice (Figure 1B), indicating greater bacterial dissemination. This increased bacterial burden and dissemination was associated with a reduced survival rate in Nr4a1-/- mice (14% vs. 0%) at 72 hours post-infection (Figure 1C). Consistent with these findings, histological analysis identified greater infection-driven lung pathology in Nr4a1-/- mice (Figures 1D, E). These findings demonstrate the critical importance of Nr4a1 in controlling K. pneumoniae pneumonia.
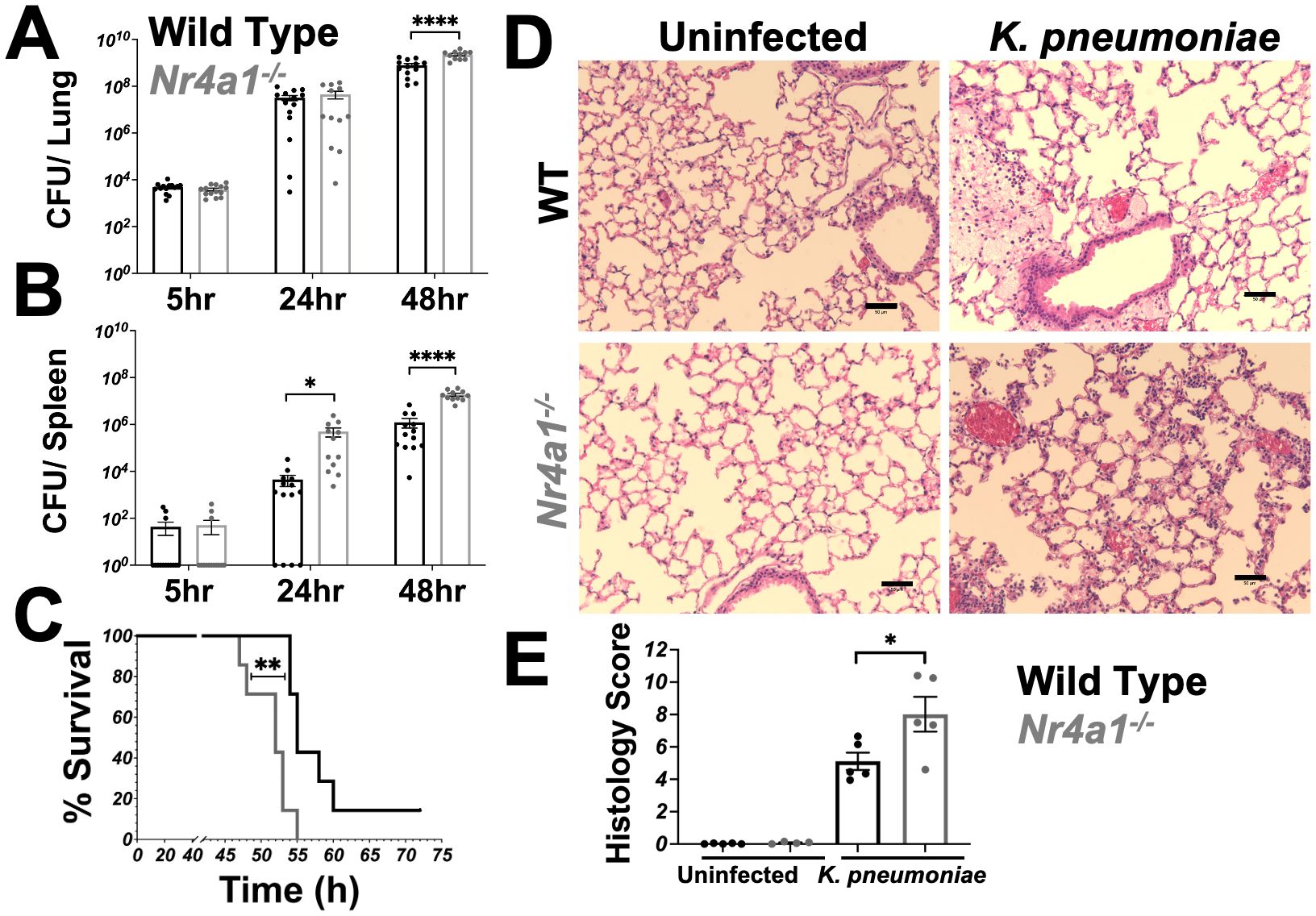
Figure 1. Nr4a1-deficient mice are highly susceptible to K. pneumoniae pneumonia. (A, B) WT and Nr4a1-/- mice were infected with K. pneumoniae intratracheally (IT). Colony forming unit (CFU) from lung (A) or spleen (B) homogenates were analyzed 5, 24, or 48 hours post infection. (C) Survival of WT and Nr4a1-/- mice following K. pneumoniae infection (n=7-8/group). (D, E) H&E staining of lungs 24 hours post infection ± K. pneumoniae. (D) Representative images of lung pathology. Scale bars indicate 50 μm. (E) Quantification of histology score. n= 11-15 (A, B), 7-8 (D) 5 (E), mean ± SEM., comparison by unpaired t-test (A-B, E) or Mentel-Cox test (C) where *p < 0.05, **p < 0.01 and ****p < 0.0001.
Nr4a1 expression in neutrophils is protective against K. pneumoniae infection
As shown in Supplementary Figure S1B, transcripts of cytokines and chemokines essential for neutrophil activation and recruitment were reduced in the lungs of Nr4a1-/- mice. Further, prior to differences in lung or spleen bacterial burden, Nr4a1-/- mice had reduced neutrophil recruitment to the lungs, while the recruitment of alveolar and inflammatory macrophages was not different between WT and Nr4a1-/- mice following K. pneumoniae infection (Supplementary Figure S1C). Additionally, the expression of proteins associated with neutrophil effector functions (IL-1ß and MPO) were impaired in the lungs of Nr4a1-/- mice following K. pneumoniae infection (Supplementary Figure S1D). Together, these data demonstrate that Nr4a1-/- mice exhibit multiple aspects of impaired neutrophil-associated immune responses following K. pneumoniae infection.
To experimentally validate whether hematopoietic versus non-hematopoietic Nr4a1 expression was required for protection against K. pneumoniae pulmonary infection, we generated bone marrow (BM) chimeric mice, where the BM of WT or Nr4a1-/- mice was transplanted into lethally irradiated WT or Nr4a1-/- recipients (Figure 2A, Supplementary Figures S3A, B). Regardless of the recipient genotype, mice transplanted Nr4a1-/- BM failed to control lung bacterial burden and dissemination (Figure 2A), indicating that Nr4a1 expression in BM-derived cells is crucial for protection against K. pneumoniae pulmonary infection.
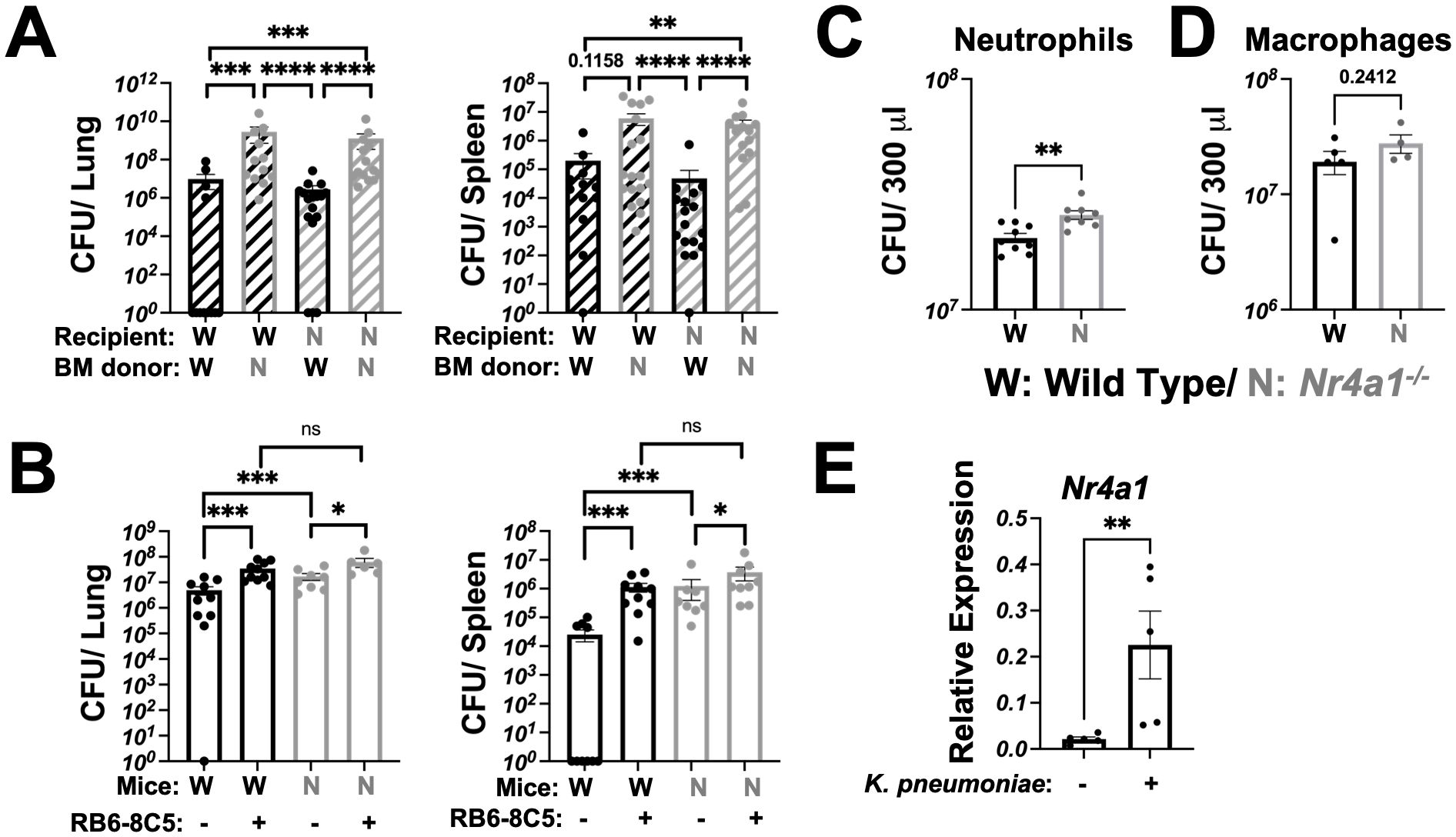
Figure 2. Nr4a1 expression in neutrophils is protective against K. pneumoniae infection. (A) Bone marrow (BM) chimera mice were generated using WT and Nr4a1-/- mice as either donor, recipient or both, as denoted. K. pneumoniae-infected burden was quantified by CFU in lung (left) or spleen (right) of recipient mice 48 hours post-infection. (B) WT and Nr4a1-/- mice were treated with 100 μg RB6-8C5 antibody (or isotype control) to deplete Gr-1+ cells. 24 hours post treatment, WT and Nr4a1-/- mice were infected with K. pneumoniae and bacterial burden in lung (left) or spleen (right) quantified 48 hours post infection. Purified BM primary neutrophils (C) or macrophages (D) from WT or Nr4a1-/- mice were incubated with K. pneumoniae at an MOI of 5 for 1 hour ex vivo at 37 °C, with supernatants were collected for K. pneumoniae quantification by CFU. (E) Expression of Nr4a1 gene in purified WT neutrophils ± K. pneumoniae incubation for 1 hour at 37 °C via qPCR. n = 11-15 (A), 6-10 (B), 8-9 (C), 4-5 (D, E), mean ± SEM., comparison by Mann-Whitney tests (A/B) or unpaired t-test (C-E) where *p < 0.05, **p < 0.01, ***p < 0.001, and ****p < 0.0001.
To further investigate the immune cell specific Nr4a1 expression requirement, we directly examined the impact of Gr-1+ cell-depletion on bacterial burdens in WT and Nr4a1-/- mice. RB6-8C5 monoclonal antibody treatment depleted cells (primarily neutrophils) and led to significantly increased bacterial burden in lung and increased bacterial dissemination post-infection in both WT and Nr4a1-/- mice, reinforcing the central role of neutrophils in K. pneumoniae clearance (Figure 2B). Notably, there was no difference in lung or spleen bacterial burden between WT and Nr4a1-/- mice after Gr-1+ depletion, suggesting that Nr4a1-dependent susceptibility is mediated by Gr-1+ cells.
To isolate the Nr4a1-mediated effect within the key myeloid cell populations, we employed an ex vivo primary cell bacterial killing assay. Here, we confirmed that purified BM neutrophils (Figure 2C), but not BM macrophages (Figure 2D), are directly responsible for the killing of K. pneumoniae ex vivo in a Nr4a1-dependent manner (Figures 2C, D, Supplementary Figure S3C). Correspondingly, WT neutrophils upregulated Nr4a1 gene expression upon incubation with K. pneumoniae ex vivo (Figure 2E), consistent with observations in vivo (Supplementary Figure S1A).
Nr4a1 controls neutrophil defense response genes
To investigate how neutrophil-specific Nr4a1 expression contributes to defense against K. pneumoniae, bulk RNA-sequencing was performed on purified WT and Nr4a1-/- neutrophils, both with and without incubation with K. pneumoniae ex vivo, which revealed transcriptomic differences between WT and Nr4a1-/- PMNs, regardless of K. pneumoniae stimulation (Figure 3A, Supplementary Figure S5). 209 differentially expressed genes (DEGs) were identified in both unstimulated and Kp-stimulated conditions (DEGs) including Nr4a1 as expected, as well as innate antibacterial effector genes Tlr2, Myd88, Sod2, Ctss, and Fpr2 (Figure 3B; Supplementary Table S5). 182 DEGs were identified only under stimulated conditions, including phagocyte activation genes Nod2, Elmo2, Rel and Ccl3 while 153 DEGs were identified under baseline conditions.
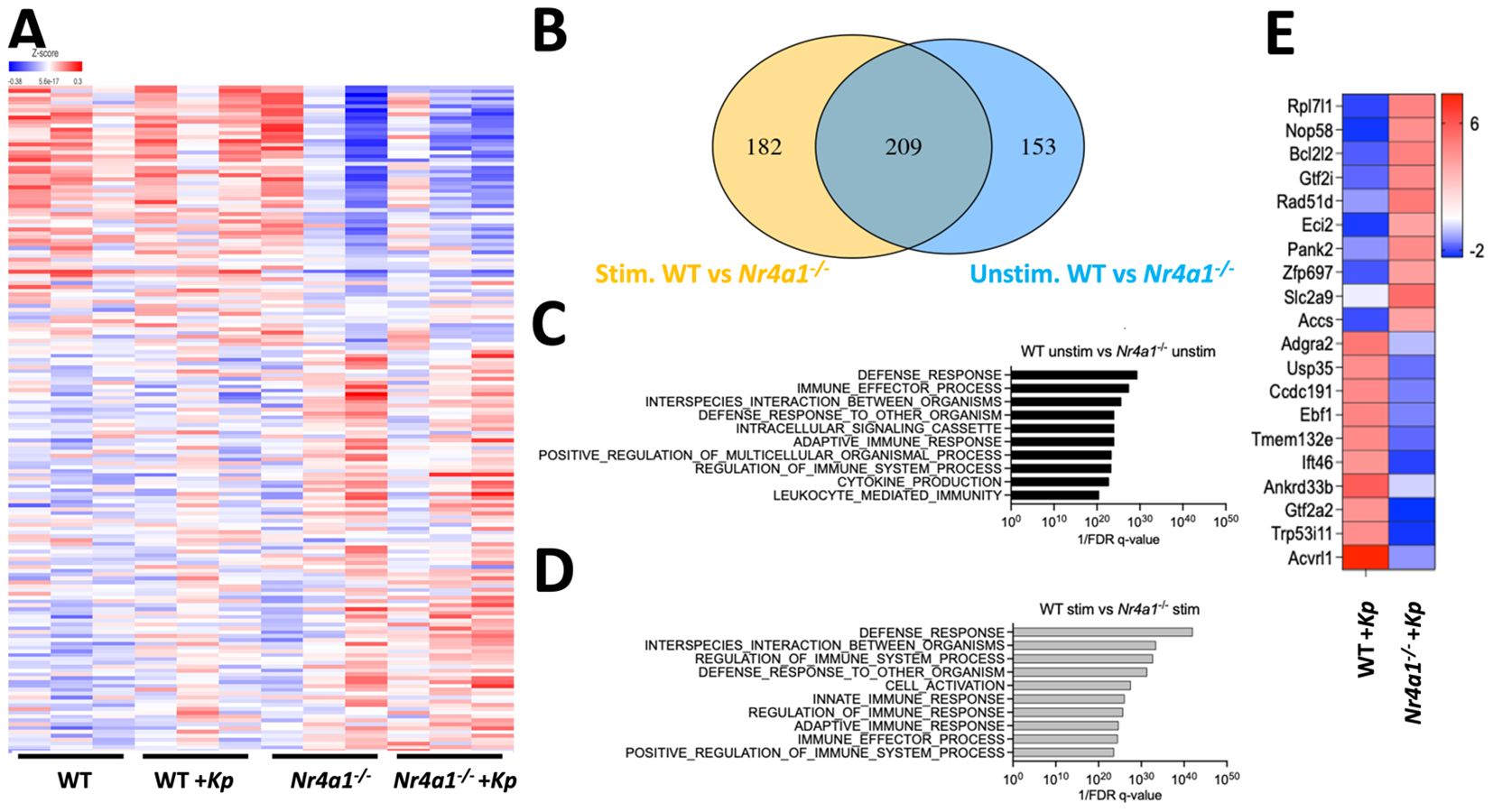
Figure 3. Nr4a1 regulates neutrophil defense response transcriptome. (A-D) RNA sequencing of WT and Nr4a1-/- primary neutrophils (PMNs). PMNs were isolated from bone marrow of indicated mice and incubated ± K. pneumoniae ex vivo for 30 minutes prior to RNA seq. (A) Heatmap comparing the transcriptome of K. pneumoniae stimulated and unstimulated WT and Nr4a1-/- PMNs. (B) Venn diagram of differentially expressed transcripts between Kp-stimulated (yellow) and unstimulated (blue) WT and Nr4a1-/- PMNs. GEO analysis of unstimulated (C) or stimulated (D) WT and Nr4a1-/- PMNs. (E) Heatmap of 20 most differentially expressed genes (fold change) comparing WT and Nr4a1-/- Kp-stimulated neutrophils.
Pathway analysis of WT and Nr4a1-/- PMNs DEGs at baseline were enriched for terms associated with several neutrophil functions, including “Defense response,” “Immune effector process” and “Interspecies interaction between organisms” (Figure 3C). After K. pneumoniae infection, WT and Nr4a1-/- differentially expressed neutrophil transcripts yielded further enrichment for the terms “Defense response”, “Interspecies interaction between organisms”, “Regulation of Immune System Process”, “Defense Response to other organism”, as well as “Cell Activation” and “Innate Immune Response” (Figure 3D). Thus, RNA sequencing analysis identified that prior to infection Nr4a1-/- neutrophils have altered antibacterial transcriptomic profiles, and that K. pneumoniae infection activates a transcriptional program that fails to enrich in the context of Nr4a1 deficiency (Figure 3B, Supplementary Table S4). Examining the 20 most differentially expressed transcripts in the context of K. pneumoniae stimulation (Figure 3E) identified genes with no reported role in neutrophil antibacterial function, to the best of our knowledge, though 19 of these DEGs have been shown to be expressed in neutrophils. Cumulatively, these RNA sequencing data align with the in vitro and in vivo observations that Nr4a1 deficiency impairs neutrophil lung recruitment and bactericidal activity against K. pneumoniae.
Discussion
Nr4a1 exhibits diverse cellular expression and pleotropic functions in mammalian biology, with its role in regulating immune cell transcription and cellular function being well described (15–20). Most of these studies focused on its role in macrophages and T cells (21–26). Despite documented expression in human and murine neutrophils, the function of Nr4a1 in neutrophils remain underappreciated (32, 33). Ballasteros et al. identified that neutrophil gene expression and function are influenced by the tissue environment, showing that Nr4a1 expression was induced in lung-specific neutrophils, which was acquired after arrival in the lung (34). This finding in particular suggests a significant role for Nr4a1 in neutrophil-mediated lung immunity.
Our work uncovered a novel neutrophil-specific requirement for Nr4a1 in pulmonary immunity against Klebsiella pneumoniae. Nr4a1 deficiency led to delayed neutrophil recruitment to the lung, downregulated genes involved in neutrophil activation and recruitment, and reduced expression of proteins critical for effector functions in vivo. RNA-seq analysis of purified neutrophils identified Nr4a1-dependent, antibacterial transcriptional signatures, and Nr4a1-deficient neutrophils exhibited impaired bacterial killing in vitro.
This neutrophil-specific role of Nr4a1 in promoting anti-bacterial activity is notably distinct from its effects on other immune cell populations. In macrophages, Nr4a1 deficiency increased TNFα production (22), along with other proinflammatory cytokines (23), exacerbating inflammation driven atherosclerosis (24). Similarly, Nr4a1 expression in T cells has been shown to suppress inflammation associated with autoimmunity (25) and cancer (26). This unique cell-specific function of Nr4a1 in neutrophils suggests that targeting neutrophil-specific Nr4a1 could be a promising approach developing immunotherapies against bacterial pneumonia.
A deeper understanding of how Nr4a1 influences neutrophil recruitment, activation, and effector functions compared to other immune cells is critical for optimizing Nr4a1-directed pneumonia treatment strategies. Several innate immune signaling pathways are activated during bacterial infection, including Toll-like receptors (e.g.CD14/TLR4) and Nod-like receptors (35–37). Previous studies have shown that Nr4a1 limits MAPK-mediated NF-κB p65 phosphorylation and downstream inflammation in alveolar macrophages (21, 22). The present data identified 544 Nr4a1-dependent DEGs, some of which are well-established neutrophil-associated mediators of bacterial immunity, such as the innate pathogen recognition receptors Toll-like 2 (Tlr2) and formyl peptide receptor 2 (Fpr2), and Myd88, Sod2, and Rel which regulate intracellular signaling and effector function. However, the most highly differentially expressed transcripts have no established role in neutrophil-mediated bacterial immunity, though some have been described to mediate neutrophil-dependent disease in non-infectious pathologies, such as enoyl-CoA delta isomerase 2 (Eci2) in colorectal cancer and solute carrier family 2 member 9 (Slc2a9) in hyperuricemic neutrophil inhibition (38, 39). Whether these pathways are modulated by Nr4a1 in neutrophil-dependent immunity to pulmonary infection remains unknown, and further examination could provide valuable insights.
In addition to investigating the differential roles of Nr4a1 in distinct immune cell populations, it is crucial to determine whether the Nr4a1-mediated immune response is pathogen-specific in pneumonia. In our study, Nr4a1 deficiency did not affect macrophage recruitment or change bone marrow-derived macrophage bactericidal capacity against K. pneumoniae. However, in a murine E. coli lung infection model, Cui et al. reported that Nr4a1 deficiency increased alveolar macrophage phagocytosis and enhanced bacterial clearance (40), alongside an increase in macrophage abundance in BAL fluid, despite a reduction in neutrophils. This discordance suggests that Nr4a1 not only functions in a cell-specific manner, but that pathogen-specific immune responses this may govern the lung phenotype. Investigating Nr4a1-regulated immune responses to different pneumonic pathogens, such as S. pneumoniae, influenza, and Aspergillus, will help delineate the critical mechanisms underlying these phenotypes of host-pathogen interaction during pulmonary infection.
Finally, it is crucial to validate findings from mouse models of neutrophil biology in human neutrophils, given the known differences in granule formation and transcriptional and proteomic profiles. Additionally, the tissue-specific role of neutrophil-specific Nr4a1 expression remains unexplored and could be investigated in other sites commonly infected by K. pneumoniae, including the liver, abdomen, and urinary tract.
In summary, we have demonstrated that neutrophil-specific Nr4a1 is essential for protective immunity against K. pneumoniae pneumonia. Further, our study identifies the neutrophil transcriptome regulated by Nr4a1. Continued research into the cell- and pathogen-specific regulation by Nr4a1, along with a deeper understanding of its downstream molecular pathways, may inform the development of new immunotherapies for bacterial infections.
Data availability statement
The data presented in the study are deposited in the NCBI GEO repository, accession number GSE290097.
Ethics statement
The animal study was approved by University of Pittsburgh Institutional Animal Care and Use Committee. The study was conducted in accordance with the local legislation and institutional requirements.
Author contributions
NS: Conceptualization, Data curation, Formal analysis, Investigation, Methodology, Project administration, Validation, Visualization, Writing – original draft, Writing – review & editing. MH: Conceptualization, Data curation, Formal analysis, Investigation, Methodology, Project administration, Validation, Visualization, Writing – review & editing. JP: Conceptualization, Data curation, Formal analysis, Investigation, Methodology, Project administration, Validation, Visualization, Writing – original draft, Writing – review & editing. AA: Data curation, Formal analysis, Investigation, Writing – review & editing. FK: Data curation, Investigation, Writing – review & editing. CB: Writing – review & editing, Conceptualization, Resources. TE: Conceptualization, Writing – review & editing, Data curation, Formal analysis, Investigation, Validation, Visualization. BC: Conceptualization, Data curation, Formal analysis, Investigation, Validation, Visualization, Writing – review & editing, Funding acquisition, Methodology, Project administration, Resources, Supervision, Writing – original draft.
Funding
The author(s) declare that financial support was received for the research, authorship, and/or publication of this article. This research was supported by NIH K08AI182486 (TE), K08HL128809 and R01HL158576 (BC).
Acknowledgments
We thank the UPMC Children’s Hospital of Pittsburgh (CHP) Histology Core Laboratory for tissue processing, slide preparation, and H&E staining. We are grateful for the microscopy support by Dr. John F. Alcorn, and flow cytometer assistance by the Flow Cytometry Core at the UPMC CHP (RRID: SCR_025223). We also thank the Health Sciences Sequencing Core at UPMC CHP for Library generation and sequencing (RRID: SCR_023116). Services and instruments used in this project were graciously supported by the University of Pittsburgh, the Office of the Senior Vice Chancellor for Health Sciences, the Department of Pediatrics, the Institute for Precision Medicine, and the Richard K. Mellon Foundation for Pediatric Research.
Conflict of interest
The authors declare that the research was conducted in the absence of any commercial or financial relationships that could be construed as a potential conflict of interest.
Generative AI statement
The author(s) declare that no Generative AI was used in the creation of this manuscript.
Publisher’s note
All claims expressed in this article are solely those of the authors and do not necessarily represent those of their affiliated organizations, or those of the publisher, the editors and the reviewers. Any product that may be evaluated in this article, or claim that may be made by its manufacturer, is not guaranteed or endorsed by the publisher.
Supplementary material
The Supplementary Material for this article can be found online at: https://www.frontiersin.org/articles/10.3389/fimmu.2025.1558252/full#supplementary-material
References
1. Walker CLF, Rudan I, Liu L, Nair H, Theodoratou E, Bhutta ZA, et al. Global burden of childhood pneumonia and diarrhoea. Lancet. (2013) 381:1405–16. doi: 10.1016/S0140-6736(13)60222-6
2. File TM Jr., Marrie TJ. Burden of community-acquired pneumonia in North American adults. Postgrad Med. (2010) 122:130–41. doi: 10.3810/pgm.2010.03.2130
3. Collaborators, G. B. D. L. R. I. Estimates of the global, regional, and national morbidity, mortality, and aetiologies of lower respiratory infections in 195 countries, 1990-2016: a systematic analysis for the Global Burden of Disease Study 2016. Lancet Infect Dis. (2018) 18:1191–210. doi: 10.1016/S1473-3099(18)30310-4
4. Jain S, Williams DJ, Arnold SR, Ampofo K, Bramley AM, Reed C, et al. Community-acquired pneumonia requiring hospitalization among U.S. children. N Engl J Med. (2015) 372:835–45. doi: 10.1056/NEJMoa1405870
5. Jain S, Self WH, Wunderink RG, Fakhran S, Balk R, Bramley AM, et al. Community-acquired pneumonia requiring hospitalization among U.S. Adults. New Engl J Med. (2015) 373:415–27. doi: 10.1056/NEJMoa1500245
6. Miyazaki T, Fukushima K, Hashiguchi K, Ide S, Kobayashi T, Sawai T, et al. A high alpha1-antitrypsin/interleukin-10 ratio predicts bacterial pneumonia in adults with community-acquired pneumonia: a prospective cohort study. Pneumonia (Nathan). (2023) 15:16. doi: 10.1186/s41479-023-00118-4
7. Bruxvoort KJ, Fischer H, Lewnard JA, Hong VX, Pomichowski M, Grant LR, et al. Risk of subsequent lower respiratory tract infection (LRTI) after hospitalization for COVID-19 LRTI and non-COVID-19 LRTI: a retrospective cohort study. Pneumonia (Nathan). (2023) 15:15. doi: 10.1186/s41479-023-00117-5
8. Morens DM, Taubenberger JK, Fauci AS. Predominant role of bacterial pneumonia as a cause of death in pandemic influenza: implications for pandemic influenza preparedness. J Infect Dis. (2008) 198:962–70. doi: 10.1086/591708
9. Obar JJ, Shepardson KM. Coinfections in the lung: How viral infection creates a favorable environment for bacterial and fungal infections. PloS Pathog. (2023) 19:e1011334. doi: 10.1371/journal.ppat.1011334
10. Han X, Yao J, He J, Liu H, Jiang Y, Zhao D, et al. Clinical and laboratory insights into the threat of hypervirulent Klebsiella pneumoniae. Int J Antimicrob Agents. (2024) 64(3):107275. doi: 10.1016/j.ijantimicag.2024.107275
11. Chenoweth CE, Saint S, Martinez F, Lynch JP 3rd, Fendrick AM. Antimicrobial resistance in Streptococcus pneumoniae: implications for patients with community-acquired pneumonia. Mayo Clin Proc. (2000) 75:1161–8. doi: 10.4065/75.11.1161
12. Carbapenem-resistant enterobacteriaceae (2019). Available online at: https://chrome-extension://efaidnbmnnnibpcajpcglclefindmkaj/https://www.cdc.gov/antimicrobial-resistance/media/pdfs/cre-508.pdf. (CDC), C. f. D. C. a. P. (Accessed November 3, 2021).
13. Robinson E, Sawhney S, Cortina-Borja M, David AL, Smith CM, Smyth RL. Neutrophil responses to RSV infection show differences between infant and adult neutrophils. Thorax. (2024) 79:545–52. doi: 10.1136/thorax-2023-220081
14. Lawrence SM, Corriden R, Nizet V. Age-appropriate functions and dysfunctions of the neonatal neutrophil. Front Pediatr. (2017) 5:23. doi: 10.3389/fped.2017.00023
15. Mullican SE, Zhang S, Konopleva M, Ruvolo V, Andreeff M, Milbrandt J, et al. Abrogation of nuclear receptors Nr4a3 and Nr4a1 leads to development of acute myeloid leukemia. Nat Med. (2007) 13:730–5. doi: 10.1038/nm1579
16. Lee SL, Wesselschmidt RL, Linette GP, Kanagawa O, Russell JH, Milbrandt J. Unimpaired thymic and peripheral T cell death in mice lacking the nuclear receptor NGFI-B (Nur77). Science. (1995) 269:532–5. doi: 10.1126/science.7624775
17. Hanna RN, Carlin LM, Hubbeling HG, Nackiewicz D, Green AM, Punt JA, et al. The transcription factor NR4A1 (Nur77) controls bone marrow differentiation and the survival of Ly6C- monocytes. Nat Immunol. (2011) 12:778–85. doi: 10.1038/ni.2063
18. Maxwell MA, Cleasby ME, Harding A, Stark A, Cooney GJ, Muscat GE. Nur77 regulates lipolysis in skeletal muscle cells. Evidence for cross-talk between the beta-adrenergic and an orphan nuclear hormone receptor pathway. J Biol Chem. (2005) 280:12573–84. doi: 10.1074/jbc.M409580200
19. Pei L, Waki H, Vaitheesvaran B, Wilpitz DC, Kurland IJ, Tontonoz P. NR4A orphan nuclear receptors are transcriptional regulators of hepatic glucose metabolism. Nat Med. (2006) 12:1048–55. doi: 10.1038/nm1471
20. Fassett MS, Jiang W, D'Alise AM, Mathis D, Benoist C. Nuclear receptor Nr4a1 modulates both regulatory T-cell (Treg) differentiation and clonal deletion. Proc Natl Acad Sci U.S.A. (2012) 109:3891–6. doi: 10.1073/pnas.1200090109
21. Henkel M, Partyka J, Gregory AD, Forno E, Cho MH, Eddens T, et al. FSTL-1 attenuation causes spontaneous smoke-resistant pulmonary emphysema. Am J Respir Crit Care Med. (2020) 201:934–45. doi: 10.1164/rccm.201905-0973OC
22. Li L, Liu Y, Chen HZ, Li FW, Wu JF, Zhang HK, et al. Impeding the interaction between Nur77 and p38 reduces LPS-induced inflammation. Nat Chem Biol. (2015) 11:339–46. doi: 10.1038/nchembio.1788
23. Hanna RN, Shaked I, Hubbeling HG, Punt JA, Wu R, Herrley E, et al. NR4A1 (Nur77) deletion polarizes macrophages toward an inflammatory phenotype and increases atherosclerosis. Circ Res. (2012) 110:416–27. doi: 10.1161/CIRCRESAHA.111.253377
24. Koenis DS, Medzikovic L, van Loenen PB, van Weeghel M, Huveneers S, Vos M, et al. Nuclear receptor nur77 limits the macrophage inflammatory response through transcriptional reprogramming of mitochondrial metabolism. Cell Rep. (2018) 24:2127–2140 e2127. doi: 10.1016/j.celrep.2018.07.065
25. Liebmann M, Hucke S, Koch K, Eschborn M, Ghelman J, Chasan AI, et al. Nur77 serves as a molecular brake of the metabolic switch during T cell activation to restrict autoimmunity. Proc Natl Acad Sci U.S.A. (2018) 115:E8017–26. doi: 10.1073/pnas.1721049115
26. Liu X, Wang Y, Lu H, Li J, Yan X, Xiao M, et al. Genome-wide analysis identifies NR4A1 as a key mediator of T cell dysfunction. Nature. (2019) 567:525–9. doi: 10.1038/s41586-019-0979-8
27. Hamid T, Malik MT, Millar RP, Kakar SS. Protein kinase A serves as a primary pathway in activation of Nur77 expression by gonadotropin-releasing hormone in the LbetaT2 mouse pituitary gonadotroph tumor cell line. Int J Oncol. (2008) 33:1055–64. doi: 10.3892/ijo_00000094
28. Reddy AT, Lakshmi SP, Banno A, Jadhav SK, Pulikkal Kadamberi I, Kim SC, et al. Cigarette smoke downregulates Nur77 to exacerbate inflammation in chronic obstructive pulmonary disease (COPD). PloS One. (2020) 15:e0229256. doi: 10.1371/journal.pone.0229256
29. Chen K, McAleer JP, Lin Y, Paterson DL, Zheng M, Alcorn JF, et al. Th17 cells mediate clade-specific, serotype-independent mucosal immunity. Immunity. (2011) 35:997–1009. doi: 10.1016/j.immuni.2011.10.018
30. Henkel M, Dutta JA, Partyka J, Eddens T, Hirsch R, Kolls JK, et al. Regulation of pulmonary bacterial immunity by follistatin-like protein 1. Infect Immun. (2020) 89:1–13. doi: 10.1128/IAI.00298-20
31. Matute-Bello G, Downey G, Moore BB, Groshong SD, Matthay MA, Slutsky AS, et al. An official American Thoracic Society workshop report: features and measurements of experimental acute lung injury in animals. Am J Respir Cell Mol Biol. (2011) 44:725–38. doi: 10.1165/rcmb.2009-0210ST
32. Zhang X, Kluger Y, Nakayama Y, Poddar R, Whitney C, DeTora A, et al. Gene expression in mature neutrophils: early responses to inflammatory stimuli. J Leukoc Biol. (2004) 75:358–72. doi: 10.1189/jlb.0903412
33. Ericson JA, Duffau P, Yasuda K, Ortiz-Lopez A, Rothamel K, Rifkin IR, et al. Gene expression during the generation and activation of mouse neutrophils: implication of novel functional and regulatory pathways. PloS One. (2014) 9:e108553. doi: 10.1371/journal.pone.0108553
34. Ballesteros I, Rubio-Ponce A, Genua M, Lusito E, Kwok I, Fernandez-Calvo G, et al. Co-option of neutrophil fates by tissue environments. Cell. (2020) 183:1282–1297 e1218. doi: 10.1016/j.cell.2020.10.003
35. McKeown-Longo PJ, Higgins PJ. Integration of canonical and noncanonical pathways in TLR4 signaling: complex regulation of the wound repair program. Adv Wound Care (New Rochelle). (2017) 6:320–9. doi: 10.1089/wound.2017.0736
36. Kuzmich NN, Sivak KV, Chubarev VN, Porozov YB, Savateeva-Lyubimova TN, Peri F. TLR4 signaling pathway modulators as potential therapeutics in inflammation and sepsis. Vaccines (Basel). (2017) 5:1–25. doi: 10.3390/vaccines5040034
37. Andersson U, Tracey KJ, Yang H. Post-translational modification of HMGB1 disulfide bonds in stimulating and inhibiting inflammation. Cells. (2021) 10:1–17. doi: 10.3390/cells10123323
38. Ma Q, Immler R, Pruenster M, Sellmayr M, Li C, von Brunn A, et al. Soluble uric acid inhibits beta2 integrin-mediated neutrophil recruitment in innate immunity. Blood. (2022) 139:3402–17. doi: 10.1182/blood.2021011234
39. Chen L, Dai P, Liu L, Chen Y, Lu Y, Zheng L, et al. The lipid-metabolism enzyme ECI2 reduces neutrophil extracellular traps formation for colorectal cancer suppression. Nat Commun. (2024) 15:7184. doi: 10.1038/s41467-024-51489-1
Keywords: Nr4a1, Nur77, neutrophil, bacterial pneumonia, lung immunity, Klebsiella pneumoniae
Citation: Sugitani N, Henkel M, Partyka J, Applegate A, Kemp F, Byersdorfer CA, Eddens T and Campfield BT (2025) Nuclear receptor 4A1 is critical for neutrophil-dependent pulmonary immunity to Klebsiella pneumoniae infection. Front. Immunol. 16:1558252. doi: 10.3389/fimmu.2025.1558252
Received: 09 January 2025; Accepted: 31 January 2025;
Published: 06 March 2025.
Edited by:
Ahmed Sherif Attia, Cairo University, EgyptReviewed by:
Atul Verma, University of Texas Medical Branch at Galveston, United StatesKristina Zec, University of Oxford, United Kingdom
Copyright © 2025 Sugitani, Henkel, Partyka, Applegate, Kemp, Byersdorfer, Eddens and Campfield. This is an open-access article distributed under the terms of the Creative Commons Attribution License (CC BY). The use, distribution or reproduction in other forums is permitted, provided the original author(s) and the copyright owner(s) are credited and that the original publication in this journal is cited, in accordance with accepted academic practice. No use, distribution or reproduction is permitted which does not comply with these terms.
*Correspondence: Brian T. Campfield, YnJpYW4uY2FtcGZpZWxkQGNocC5lZHU=
†These authors have contributed equally to this work
‡ORCID: Norie Sugitani, orcid.org/0000-0002-5582-8855
Alexander Applegate, orcid.org/0009-0002-3413-5504
Felicia Kemp, orcid.org/0000-0001-8400-3089
Craig A. Byersdorfer, orcid.org/0000-0002-0030-3699
Taylor Eddens, orcid.org/0000-0003-2969-3624
Brian T. Campfield, orcid.org/0000-0002-5380-9837