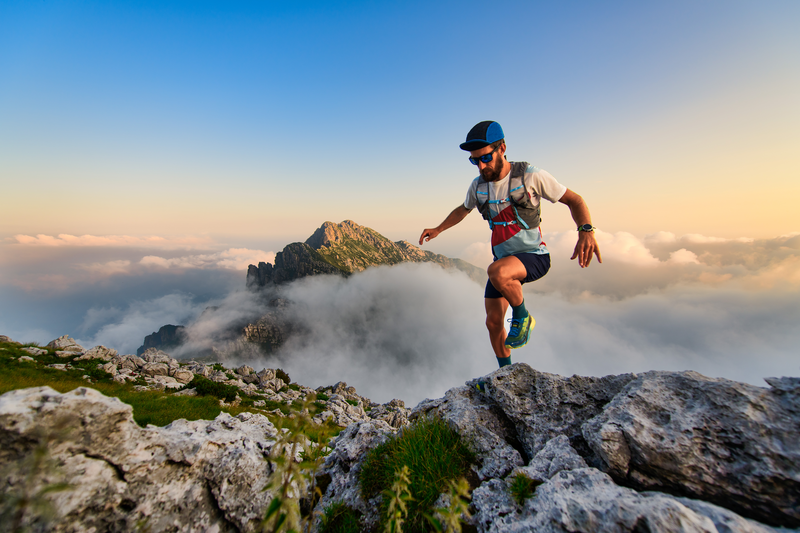
95% of researchers rate our articles as excellent or good
Learn more about the work of our research integrity team to safeguard the quality of each article we publish.
Find out more
ORIGINAL RESEARCH article
Front. Immunol. , 26 February 2025
Sec. Comparative Immunology
Volume 16 - 2025 | https://doi.org/10.3389/fimmu.2025.1557256
This article is part of the Research Topic Community Series in Aquatic Nutrition and Intestine Immunity: Volume II View all 4 articles
Introduction: The aim of this study is to investigate the effects of supplementing Clostridium butyricum (C. butyricum) on hybrid grouper (Epinephelus fuscoguttatus ♀ × Epinephelus lanceolatus ♂), with a particular focus on its impact on growth performance, blood composition, intestinal antioxidant capacity, gut microbiota, tight junction protein (ZO-1) expression, and inflammatory gene expression. The study seeks to uncover the potential health benefits of C. butyricum supplementation for hybrid grouper.
Methods: The experiment included four groups: a control group (CON) and three experimental groups, each supplemented with different strains of C. butyricum (KM, DZN, and CLH), with a concentration of 1 × 10⁷ colony-forming units per gram. These groups were designated as CB1 (KM), CB2 (DZN), and CB3 (CLH). The study evaluated growth performance, blood composition, intestinal antioxidant capacity, gut microbiota, ZO-1 protein expression, and inflammatory gene expression (IL-1β and Ikk-β).
Result: The results indicated that supplementation with C. butyricum had no significant effect on body weight gain (WG), feed efficiency (FE), or body composition. However, the CB3 group significantly increased the activity of superoxide dismutase (SOD) and glutathione peroxidase (GSH-Px) in the intestine, as well as the expression of ZO-1. In addition, the CB3 group significantly increased serum lysozyme (LZM) activity, complement 4 (C4) levels, and immunoglobulin M (IgM) concentration, while significantly reducing the expression of pro-inflammatory genes (IL-1β and Ikk-β). After supplementation with C. butyricum, the level of malondialdehyde (MDA) in the intestine was significantly lower than that in the control group, indicating a reduction in intestinal oxidative stress. Supplementation with C. butyricum also altered the composition of the gut microbiota, promoting the growth of beneficial bacteria and inhibiting pathogenic bacteria, thereby further enhancing ZO-1 expression and intestinal barrier function.
Discussion: This study suggests that supplementing C. butyricum has a significant immunomodulatory effect on hybrid grouper, enhancing serum immune parameters, alleviating intestinal inflammation and oxidative stress, and promoting intestinal health. Although no significant impact was observed on growth performance, the role of C. butyricum in improving intestinal barrier function and modulating the gut microbiota highlights its potential for enhancing fish health.
It is well known that gut is an important organ of fish that performs the absorption and metabolism of nutrients and the essential maintenance of gut health and homeostasis of fish in the healthy state (1) as a matter of gut microbiota contributions (2, 3). For instance, gut microbiota participate in the development and repair of the host’s gut epithelia and maintain the integrity of the gut barrier (4), resisting the invasion of pathogenic bacteria via inhibiting the proliferation of pathogenic bacteria (5–7). In contrast, unhealthy gut is linked to the imbalance of gut flora, accompanied with disruption of gut mucosal barrier function and the colonization and proliferation of pathogenic microbiota, eventually leading to diarrhea or enteritis (8). The practice of intensive farming has become an important factor contributing to the unhealthy conditions of farmed animals, resulting in the development of many diseases (9). Therefore, how to manipulate gut microbiota to achieve a more favorable balance received widespread attention, from a perspective view of improving health by feed measures (10).
Historically, antibiotics have been used extensively for routine disease prevention and control (11). However, there is still an urgent shift to use environmentally friendly and safe measures to replace antibiotics. This is not only a drug resistance issue, but also a major concern for human health (12, 13). Probiotics are live bacteria that confer a health benefit on animals as their consumption is sufficient (14). As a functional substance, they can regulate gut microbiota by lowering gut pH, providing nutrients, secreting antibacterial substances, adhering to pathogenic bacteria and regulating host immune responses (15, 16). Numerous feeding experiments have shown that the addition of probiotics to feed can improve growth performance and health status of fish and shellfish, enhancing resistance to pathogen infections (17–24). With these potential health benefits of probiotics on aquatic animals, the use of probiotics in aquaculture has gained increasing attention in recent years.
Clostridium butyricum (C. butyricum) is a specific anaerobic, Gram-positive, which is widely present in animals and soil (25, 26). It not only has excellent environmental adaptability to heat, acidity, alkalinity, and bile salts, but can also regulate intestinal function by secreting bioactive substances such as short chain fatty acids, B vitamins, digestive enzymes, etc. in the intestine (27). It is precisely because of many excellent characteristics mentioned above that C. butyricum has attracted widespread attention from aquaculture as a highly promising feed probiotic (11, 25, 28–35). At present, many studies have emphasized the positive effects of adding C. butyricum to feed, particularly on aquatic animals (9, 29). However, limited direct comparative studies have been conducted the effects of C. butyricum strains derived from different sources. The different origins of C. butyricum strains may also have varying effects on fish growth and intestinal health. Over the past three decades, probiotic microorganisms have been widely used in aquaculture, sourced from both host and non-host-derived organisms (36, 37). Host-derived probiotics have demonstrated significant potential for application in feed due to their unique biochemical characteristics (36). When selecting suitable probiotics, various factors should be evaluated, including microbial type, source, strain type, biological activity, and spore formation, all of which are crucial for their successful application in aquaculture (38, 39). Therefore, selecting the appropriate C. butyricum strains remains a critical step for its effective application in aquaculture.
The hybrid grouper (E. lanceolatus ♂ × E. fuscoguttatus ♀) is a predatory marine fish that is extensively cultivated in China and other Southeast Asian coastal nations due to its good flavor and benefits for intensive farming (40). However, the frequency of disease occurrence is increasing with the continuous promotion of intensive farming of hybrid grouper, which brings about difficulties in disease prevention and control, and has become one of the major issues that need to be addressed in the healthy culture of grouper. In particular, three types of enteric pathogen such as Vibrio anguillarum, Edwardsiella tarda and Aeromonas hydrophila pose a major threat to grouper enteritis (41). Recent studies reported that C. butyricum addition in feed can improve growth performance and intestinal health in hybrid grouper fed with commercial feed or the feed with fish meal replacement by cottonseed protein concentrate via promoting digestive ability, gut morphometry, and disease resistance (25, 42). However, it remains unknown how C. butyricum exerts the beneficial effects on growth and gut health improvement in this species. Therefore, with the use of microbiome, this study explored the mechanisms by which C. butyricum improves growth and intestinal health of hybrid grouper. The present study will provide a scientific basis for the rational application of C. butyricum in the diet of hybrid grouper.
Three distinct strains of C. butyricum were purchased as powdered products for this experiment. The KM strain (C. butyricum strain number GDMCC NO 1.4721, with a viable bacterial concentration of 1 × 10⁹ cfu/g) was isolated from the intestinal tract of fish in natural aquatic environments and obtained from Guangzhou Kemu Biotechnology Co., Ltd. The DZN strain (C. butyricum strain number GDMCC NO M2016421, with a viable bacterial concentration of 1 × 10⁹ cfu/g) was sourced from Guangdong Dazhe Agricultural Biotechnology Co., Ltd., and isolated from the intestines of healthy terrestrial animals. The CLH strain (C. butyricum strain number GDMCC NO 1.676, with a viable bacterial concentration of 1 × 10⁹ cfu/g) was isolated from natural aquatic environments and obtained from Zhanjiang Hengxing Aquaculture Technology Service Co., Ltd. The control diet (CON) was formulated using fish meal and gelatin as the protein sources and fish oil, soybean oil, and soybean lecithin as the fat sources (Table 1). Based on this formulation, three strains of C. butyricum (KM (CB1), DZN (CB2), and CLH (CB3), respectively) were evenly sprayed onto the diets to achieve a viable bacterial count of 1 × 107 cfu/g. The feed ingredients were ground using a ZFJ-300 grinder (Jiangyin Ruizong Machinery Manufacturing Co., Ltd., Jiangyin, Jiangsu, China) passed through a 60-mesh sieve, weighed, and homogenized. Liquid ingredients were then added to the dry feed ingredients to prepare malt syrup. The dough was shaped into strips using a double-helix feed extruder (F-76, Guangzhou Huagong Optoelectronic Technology Co., Ltd., Guangzhou, Guangdong, China) and a feed pellet-forming machine (GY-500, Changzhou Beicheng Drying Equipment Engineering Co., Ltd., Changzhou, Jiangsu, China), and then pelletized using 2.5 mm and 5 mm dies. The feed was dried in a ventilated oven at 55°C for 24 hours to reduce its moisture content to below 100 g/kg. The feed was then ventilated at room temperature for 24 hours, sealed in plastic bags, and stored in a refrigerator at -20°C.
The experiment was conducted at the fishery base of Dabeinong Group, located in Zhaoan County, Zhangzhou City, Fujian Province, China. Prior to the experiment, juvenile hybrid groupers were raised in blue polypropylene tanks and fed with the control diet (CON) for two weeks. At the commencement of the experiment, 300 juvenile hybrid groupers, with an initial average weight of 24.91 ± 0.01 g, were randomly distributed into 12 tanks (500 L/tank), with a density of 25 fish per tank. Under the natural photoperiod, triplicate groups of tanks were hand-fed one of the diets to satiation twice daily, at 8:00 AM and 5:00 PM,for a duration of 56 days. Any excess feed was collected 30 minutes after each meal, dried at 65℃ for 24 hours, and then weighed to calculate the feed intake.
Upon completion of the culture experiment, the total weight of the fish in each tank was measured, and the number of fish in each tank was recorded. The fish were subsequently returned to their respective tanks and subjected to a 2-day feeding cessation to mitigate the stress effects induced by the weighing process, prior to sampling. At the time of sampling, 10 fish were randomly selected from each tank, anesthetized with eugenol and then weighed, measured, and recorded. Blood was drawn from the tail vein using a 1 mL syringe and collected in a 1.5 mL centrifuge tube. The blood allowed to stand for 12 h at 3000 rpm, centrifuged at 4°C for 10 min, and then combined with serum by tank. The sample was stored in a refrigerator at -80°C for serum biochemical index determination. The liver of each fish was then excised, weighed and recorded for the calculation of the hepatosomatic index (HSI). Intestinal tissues (proximal intestine, middle intestine and distal intestine) from the same portion of each fish were collected and stored in Bonn solution for paraffin sectioning. Intestinal tissues from four fish were collected, quickly placed in a freezing tube, frozen in liquid nitrogen, and stored in a refrigerator at -80°C. Three fish were then randomly selected and stored in a refrigerator at -20°C for determination of whole fish body composition.
Whole fish samples were processed following the method described by Ye et al. (43). Specifically, after thawing the whole fish sample, its surface was wiped with gauze, followed by weighing. The fish was then placed in a weighed aluminum box, autoclave at 121°C for 30 min, cooled, cut into pieces, dried in an oven at 65°C for 24 h, and reweighed. After 24 h at room temperature, the sample was reweighed and then crushed with a small grinder, packed in a self-sealing bag and stored in a refrigerator at -20°C. Measurements of moisture, crude protein, crude lipid and ash content in experimental diets and whole fish samples were made according to AOAC method (44).
Serum aspartate aminotransferase (AST), alanine aminotransferase (ALT), C4, IgM, and LZM and total antioxidant capacity (T-AOC), MDA, GSH-Px, catalase (CAT) and SOD in the intestine were measured using the kit from Nanjing Jiancheng Bioengineering Institute, Nanjing, Jiangsu, China according to the manufacturer’s instructions (7, 11, 18, 19, 27).
Intestinal morphology was visualized using hematoxylin and eosin (H&E) staining. Following standard fixation, intestinal tissue samples were embedded in paraffin and sectioned into 5 μm-thick slices. After dewaxing in xylene I and xylene II for 20 min each, the intestinal slices underwent gradient dehydration in ethanol baths of decreasing concentrations, with 5 min per bath. The intestinal slices were washed three times with distilled water, followed by incubation with hematoxylin for 3 min, and then rinsed again with distilled water. Gradient dehydration of the intestinal slices was also performed in ethanol baths of increasing concentrations for 3 min per bath, followed by incubation with eosin for 3 min. The slices were then transferred to xylene and immersed for 3 min to render them transparent, prior to sealing with neutral resin. Images were examined under a light microscope (Leica DM5500B), and digital photographs were captured using a digital camera (Leica DFC450) equipped with LAS AF imaging software (Version 4.3.0 Leica).
Intestinal samples were collected for microbiological analysis. The E.Z.N.A.TM kit (Omega Bio-Tek, Norcross, GA, USA) was used to extract total DNA from the microbial genome of the intestinal samples. The instructions provided with the kit were followed for specific steps. A 1% agarose gel was employed to assess the concentration and purity of the extracted genomic DNA. Based on the concentration, the DNA was uniformly diluted to 15 ng/μL with sterile water. Amplification primers (338F: 5’-ACTCCTACGGGAGGCAGCAG-3’; 806R: 5’-GTGGACTACHVGGGTWTCTAAT-3’) were used to amplify total DNA using an ABI 9700 PCR instrument (Applied Biosystems, Inc., USA). An 8-bp barcode sequence was added to the 5’ end of the upstream and downstream primers to distinguish between different samples. All PCR reactions were conducted in 25 μL reaction volumes, using 12.5 μL of 2xTaq Plus Master Mix. 0.2 μM forward and reverse primers and approximately 30 ng of template DNA. The PCR reaction conditions were as follows: pre-denaturation at 94°C for 5 minutes; followed by 40 s denaturation at 94°C, 50 s of annealing at 50°C, 60 s extension at 72°C, repeated for 30 cycles, and a final extension at 72°C for 7 min. The PCR product was visualized using 1% agarose gel electrophoresis (170V 30 min). followed by automatic purification using the Agencourtam Pure XP nucleic acid purification kit (Beckman Coulter, Inc., USA). PCR libraries were constructed using the NebNext Ultra II DNA Library PrepKit (New England Biolabs, Inc., USA). The constructed library was purified using the AM Pure XP nucleic acid purification kit (Beckman Coulter, Inc., USA). The library was then sequenced on the Nextseq 2000 platform (Illumina, Inc., USA) using the PE300 sequencing strategy. The off-board data were classified according to the barcode sequence. Pear software was employed to filter and splice the sequencing data. After splicing, low-quality and chimera sequences were removed using Vsearch software, and the sequences were clustered into operational taxonomic units (OTUs) using the UPARSE algorithm, with a sequence similarity threshold of 97%. The OTU was then compared with the Silva138 database using BLAST algorithm, with an e-value threshold set to 1e-5 to obtain species classification information for each OTU. Based on the results of OTU abundance, the α diversity index and β diversity distance matrix were calculated using QIIME software, plotted with R software.
Total RNA was extracted from individual livers using the SYBR Premix Ex Taq Kit (Takara, Dalian, China), followed by quantification of RNA concentration and purity via spectrophotometry and quality assessment using agarose gel electrophoresis. Reverse transcription was performed with 1 μg of total RNA using a reverse transcription kit (Thermo). Target gene expression was quantified by quantitative real-time PCR (qRT-PCR) using an ABI 7500 real-time PCR Detection System (Applied Biosystems, Foster City, CA, USA) with SYBR Green Real-time PCR Master Mix (Toyobo, Shanghai, China). Primers for the amplification of gene-specific PCR products were designed using Primer-BLAST (https://www.ncbi.nlm.nih.gov/tools/primer-blast/), and primer details for qRT-PCR are provided in Table 2. All primers were commercially supplied by Integrated DNA Technologies (Hunan Accurate Biological Engineering Co., Ltd., Changsha, China). The real-time PCR procedure involved an initial denaturation step at 95°C for 30 s, 40 cycles at 95°C for 5 s, annealing and extension temperature at 60°C for 30 s, and the final dissociation step. The final step was performed to confirm amplification of a single product. qRT-PCR efficiency (E) was achieved using the equation E=10(−1/slope)-1. Gene expression results were analyzed using the 2-ΔΔCt method only after primers were verified to have an efficiency of approximately 100% through amplification, with data from all treatments compared to the control group.
All data were presented as mean and standard error of the mean (SEM). The data were analyzed using one-way analysis of variance (ANOVA) to assess differences between treatments, followed by the Student-Newman-Keuls multiple comparison test. Normality and homogeneity of variance were confirmed using the Kolmogorov-Smirnov and Levene’s tests, and all analyses were conducted in SPSS Statistics 25.0 (SPSS, Michigan Avenue, Chicago, IL, USA). Data expressed as percentages or ratios underwent data conversion before statistical analysis. P-values < 0.05 were considered statistically significant.
Table 3 shows that the addition of C. butyricum to the diet did not have no adverse effects on the growth performance and whole-body proximate composition of hybrid grouper. No significant differences were observed in WG, FE, FR, HSI, CF, SR, SGR, or whole-body proximate composition (moisture, crude protein, crude lipid and ash) among the groups (P > 0.05).
Table 3. Effects of dietary C. butyricum addition on growth performance and whole-body proximate composition of hybrid grouper1.
Table 4 demonstrates the effect of adding C. butyricum to the feed on the intestinal antioxidant capacity of the hybrid grouper. The activities of GSH-Px and SOD in the experimental group were significantly higher than those in the control group (P < 0.05). In the CON group, the CB3 group exhibited the highest values (P < 0.05). The addition of CB1 and CB3 to the feed significantly decreased MDA content in the intestine (P < 0.05), while no significant difference was observed between CB2 and CON (P > 0.05). No statistically significant differences were observed in the activities of CAT and T-AOC among the groups (P > 0.05).
Table 4. Effects of dietary C. butyricum addition on intestinal antioxidant capacity of hybrid grouper.
Table 5 illustrates that dietary C. butyricum influenced the plasma composition of hybrid grouper. Compared to the CON group, the C4 content and LZM activity were significantly elevated in the other groups (P < 0.05). Furthermore, the activities of AST and ALT were unaffected by the dietary treatments (P > 0.05).
As shown in Table 6, the muscle layer thickness (MT) and mucosal fold height (HMF) of the midgut were significantly increased in the C. butyricum group compared to the CON group (P < 0.05). The inclusion of C. butyricum in the feed had no effect on the morphological indicators of the anterior and posterior intestinal tissues of hybrid grouper (P > 0.05). The observations of intestinal tissue slice are presented in Figure 1. As shown in Figure 1, the tissue structure of the anterior and middle intestines in the CB1, CB2, and CB3 groups appears clearer and more structurally intact than that of the CON group, with well-developed mucosal folds. The results indicated that adding C. butyricum to the feed significantly improved the tissue structure of the anterior and middle intestines of hybrid grouper, with the CB2 group demonstrating the most pronounced improvements.
Figure 1. Effects of dietary C. butyricum addition on the intestinal morphology of hybrid grouper (100×). A, B, C and D represent the CON group, CB1 group, CB2 group and CB3 group, respectively. Muscle thickness (MT) is indicated by black bidirectional arrows, the lamina propria (LP) by yellow unidirectional arrows, and goblet cells (GC) by red unidirectional arrows.
By comparing the alpha diversity indices of microbial communities in different treatment groups, significant differences in species richness and diversity were observed among the groups (Figure 2).
Chao1 index: The CB1 group exhibited the highest estimated species richness, followed by the CON group, while the CB2 and CB3 groups exhibited significantly lower species richness.
Observed species index: The CB1 group had the highest number of observed species, followed by the CON group, while the CB2 and CB3 groups had lower numbers of observed species.
Shannon index: The Shannon diversity index of the CB1 group was the highest, indicating the highest community diversity and evenness. The CON group ranked second, while the CB2 and CB3 groups exhibited lower levels.
Simpson index: The CB1 group had the highest Simpson diversity index, further demonstrating that the CB1 group exhibited the highest community diversity and stability. The CON group ranked second, while the CB2 and CB3 groups exhibited lower levels.
Based on the heatmap and hierarchical clustering tree, β-diversity analysis revealed significant differences in microbial community structure among the treatment groups (Figure 3). Samples within the same group (e.g., con_1, con_2, con_3 in the CON group) exhibited a predominance of blue coloration in the heatmap, indicating high similarity among these samples. In contrast, samples from different groups (e.g., the CON group compared with the CB1 group) displayed a predominance of red coloration in the heatmap, indicating lower similarity between these groups. The hierarchical clustering tree illustrated the relationships between samples, with highly similar samples clustering together. The results indicate that samples within the same group shared high similarity, while significant intergroup differences were observed. Specifically, the CON group exhibited low similarity with the CB1 and CB3 groups, while a higher similarity was observed with the CB2 group. These findings highlight the significant structural variations in microbial communities among the different treatment groups (CON, CB1, CB2, and CB3).
The classification diagram of intestinal microorganisms at the phylum level among groups is shown in Figure 4A and Supplementary Table 1. At the phylum level, the dominant microflora in the intestine of hybrid grouper were Proteobacteria, Firmicutes, and Bacteroidota, accounting for more than 90%. A higher relative abundance of Firmicutes and Bacteroidota and a lower relative abundance of Proteobacteria were observed in the CB1 and CB3 groups compared to the CON group, and CB2 exhibited the opposite trend. In addition, although not significantly different among groups (P > 0.05), the relative abundance of Actinobacteriota was lower in the CON group than in any of the experimental groups.
Figure 4. Classification diagram of intestinal microbiota in each group at the phylum (A) and genus (B) level of the hybrid grouper.
The classification diagram of intestinal microorganisms at the genus level among groups is shown in Figure 4B and Supplementary Table 1. At the genus level, the bacterial communities in the dietary treatments included the following genera in particular: Bacteroides, Brevundimonas, Lactobacillus, Mitsuaria, Massilia, Pseudomonas, Stenotrophomonas and Subdoligranulum. Decreased abundances of the genera Massilia, Pseudomonas, Stenotrophomonas, and Subdoligranulum, and increased abundances of the genera Lactobacillus, Bacteroides and Mitsuaria were observed in the experimental groups compared to the CON group.
The effect of dietary C. butyricum supplementation on the expression levels of intestinal inflammatory factors and tight junction structural protein genes in hybrid grouper were illustrated in Figure 5. Compared to the CON group, the gene expression levels of pro-inflammatory factors IL-1β and Ikk-β in the experimental groups generally exhibited a down-regulation trend (P < 0.05), whereas the gene expression level of anti-inflammatory factor IL-10 was up-regulated, although no significant differences were observed (P > 0.05). Regarding tight junction protein genes, the expression levels of the ZO-1 gene in the CB1 group were significantly higher than those in the CON group (P < 0.05). However, no significant differences were found in the expression levels of claudin 15a and occludin proteins (P > 0.05).
Figure 5. Effects of dietary C. butyricum on the gene expression levels of intestinal inflammatory factors and tight junction structural protein in hybrid grouper. Different lowercase letters in the same trade indicate significant differences (P < 0.05).
In this study, the addition of C. butyricum strains from different sources to the diets had no discernible effect on the growth performance of hybrid grouper. This outcome is consistent with the observations reported for other animals, including carp (45, 46), Chinese drum (47), and goats (48). Incorporating C. butyricum into a dietary formulation comprising a low fish meal content has been shown to significantly promote fish growth (17, 25, 49). This effect may be attributed to C. butyricum’s ability to enhance intestinal metabolic function and facilitate nutrient digestion and absorption through the secretion of a multitude of short-chain fatty acids and digestive enzymes within the intestine (45, 50). These disparities may be due to the fact that the protein and fat levels in the diets are tailored to meet the nutritional requirements of hybrid grouper in this study. Consequently, the incorporation of C. butyricum did not result in a pronounced growth-promoting effect in hybrid grouper, suggesting that the growth-promoting effects of C. butyricum cannot be fully realized by merely incorporating it into feeds with high-quality protein sources.
The antioxidant capacity of the body serves as an indicator of overall health and well-being. During physiological stress or illness, the body generates an excess of free radicals, leading to oxidative damage (51). The intestine, as a conduit between the internal and external environments, is constantly exposed to free radicals from both exogenous substances and endogenous metabolites, resulting in intestinal oxidative damage (52). Previous studies have demonstrated that administering C. butyricum to animals enhances antioxidant enzyme activity and reduces MDA levels (17, 28, 33, 53, 54), thus preventing damage to the body, which is consistent with the present study’s findings. The results of the present study demonstrated that dietary C. butyricum administration led to a significant increase in SOD and GSH-Px levels in the intestine of hybrid grouper, along with a decrease in MDA levels. The antioxidant effect of C. butyricum is linked to its production of short-chain fatty acids, such as butyric and propionic acids, in the intestinal lumen, providing an energy source for intestinal mucosal cells and supporting intestinal homeostasis (55). Additionally, C. butyricum can produce nicotinamide adenine dinucleotide phosphate, scavenge reactive oxygen species, and enhance antioxidant potential, which may further contribute to the observed improvement in antioxidant capacity (56).
The present study demonstrated that dietary C. butyricum administration led to a significant increase in serum LZM levels, with the CB1 group showing a marked elevation in serum IgM. These findings indicate that dietary supplementation with C. butyricum enhances immune competence in fish. The observed outcomes were consistent across diverse species, including Miichthys miiuy (57) silver pomfret (24) large yellow croaker (58), tilapia (17), shrimp (53), and gibel carp (30). These results may be attributed to increased IgM and LZM levels, which are known to enhance the organism’s capacity to eliminate pathogens and pathogenic microorganisms (59, 60). Furthermore, the incorporation of C. butyricum into the diet elevated the serum C4 concentration of tilapia (11) and broilers (61, 62), thereby reinforcing the immune system, findings corroborated by this study.
The integrity of the intestinal tissue structure in fish is essential for the efficient maintenance of nutrient digestion and absorption (63). Greater intestinal plica height corresponds to an increased intestinal surface area and improved nutrient absorption capacity (64). Additionally, intestinal muscular layer thickness reflects its peristaltic capacity, which affects chyme digestion rate and intestinal emptying (65). Short-chain fatty acids act as an energy source for epithelial cells, promoting the growth and development of intestinal villi, while mitigating mucosal injury and reducing intestinal cell apoptosis (33). Our experimental findings, consistent with prior studies (17, 27), demonstrate that the addition of C. butyricum to the feed effectively improves the intestinal structure in fish.
Furthermore, C. butyricum enhances intestinal health by modulating the intestinal microbiota. This study identifies Proteobacteria, Firmicutes, and Bacteroidetes as the dominant intestinal flora in hybrid grouper, consistent with previous studies on grouper (66). The addition of C. butyricum significantly altered the dominant intestinal flora, increasing the relative abundance of Firmicutes and Bacteroidetes while reducing that of Proteobacteria and Actinobacteria. This finding is consistent with studies on Epinephelus coioides (67) and Procambarus clarkii (68). Firmicutes and Bacteroidetes are known to produce substantial amounts of short-chain fatty acids (69, 70), which may partly explain why C. butyricum increases short-chain fatty acid content in the intestine. Many bacteria within Proteobacteria and Actinobacteria are considered potential pathogens, and their increased relative abundance may disrupt microbial balance, increasing the risk of enteritis or pathogen invasion (71, 72). In this study, C. butyricum significantly decreased the relative abundance of these two phyla, suggesting its potential to mitigate the risk of enteritis by improving microbial structure and enhancing intestinal health.
This study evaluated the impact of C. butyricum supplementation on the expression of intestinal inflammatory cytokine and tight junction (TJ) protein genes in hybrid grouper (E. lanceolatus × E. fuscoguttatus). The results demonstrated that C. butyricum supplementation significantly regulated inflammation and improved intestinal barrier integrity. Pro-inflammatory cytokine gene expression (e.g., IL-1β and Ikk-β) was significantly downregulated in the experimental group, consistent with findings reported by Lu et al. (73). These cytokines are critical mediators of the inflammatory response, and their overexpression is closely linked to tissue damage and compromised intestinal function (74).
Beyond its anti-inflammatory effects, C. butyricum supplementation significantly upregulated ZO-1 expression levels. Tight junctions (TJs) are critical intercellular connections. Occludin, a key functional protein, interacts with tight junction proteins such as ZOs, playing an indispensable role in biological barrier function and tight junction formation. ZO proteins, particularly ZO-1, link occludin to the actin cytoskeleton and play a vital role in maintaining tight junction integrity, reducing the risk of intestinal tumors and related disease (75, 76). This study revealed that C. butyricum supplementation significantly upregulated ZO-1 expression levels. This crucial TJ protein enhances intestinal barrier integrity and elasticity by regulating paracellular permeability, potentially reducing the risk of pathogen and endotoxin translocation into systemic circulation (77). Notably, no significant changes were observed in claudin-15a and occludin expression levels, indicating that C. butyricum may selectively regulate specific TJ proteins.
In this study, the addition of C. butyricum to the diet did not significant impact on the growth performance of hybrid grouper but notably enhanced intestinal antioxidant enzyme activity and improved intestinal morphology and structure. From the perspective of intestinal flora composition, tight junction proteins, and inflammatory gene expression, the addition of C. butyricum to the diet reduced intestinal inflammation, promoted the proliferation of beneficial bacteria, and suppressed pathogenic bacteria. These findings highlighted its potential role in promoting intestinal structural integrity and health.
The datasets presented in this study can be found in online repositories. The names of the repository/repositories and accession number(s) can be found below: https://www.ncbi.nlm.nih.gov/genbank/, KF861990.1 https://www.ncbi.nlm.nih.gov/genbank/, MK809394 https://www.ncbi.nlm.nih.gov/genbank/, MK809396 https://www.ncbi.nlm.nih.gov/genbank/, EF582837.1 https://www.ncbi.nlm.nih.gov/genbank/, KM669150.1 https://www.ncbi.nlm.nih.gov/genbank/, EU219847.1 https://www.ncbi.nlm.nih.gov/genbank/, EF582837.1.
The animal study was approved by Jimei University Institutional Animal Care and Use Committee. The study was conducted in accordance with the local legislation and institutional requirements.
WC: Writing – original draft. TS: Writing – review & editing. DL: Writing – review & editing. MC: Writing – review & editing. PW: Writing – review & editing. JY: Writing – review & editing.
The author(s) declare that no financial support was received for the research, authorship, and/or publication of this article.
The authors declare that the research was conducted in the absence of any commercial or financial relationships that could be construed as a potential conflict of interest.
The author(s) declare that no Generative AI was used in the creation of this manuscript.
All claims expressed in this article are solely those of the authors and do not necessarily represent those of their affiliated organizations, or those of the publisher, the editors and the reviewers. Any product that may be evaluated in this article, or claim that may be made by its manufacturer, is not guaranteed or endorsed by the publisher.
The Supplementary Material for this article can be found online at: https://www.frontiersin.org/articles/10.3389/fimmu.2025.1557256/full#supplementary-material
1. Ding C, Jia R, Wang Y, Hou Y, Zhang L, Li B, et al. Comparison of intestinal bacteria of procambarus clarkii farmed in various rice paddy regions. Anim (Basel). (2024) 14:935. doi: 10.3390/ani14060935
2. Heinken A, Thiele I. Systematic prediction of health-relevant human-microbial co-metabolism through a computational framework. Gut Microbes. (2015) 6:120–30. doi: 10.1080/19490976.2015.1023494
3. Wang L, Li C, Wang X, Wang G, Shang S, Dou Z, et al. Gut lignocellulose activity and microbiota in asian longhorned beetle and their predicted contribution to larval nutrition. Front Microbiol. (2022) 13:899865. doi: 10.3389/fmicb.2022.899865
4. Eslami M. Importance of probiotics in the prevention and treatment of colorectal cancer. J Cell Physiol. (2019) 234:17127–43. doi: 10.1002/jcp.v234.10
5. Wang J, Xie Y, Zhang G, Pan L. Microbial community structure and diversity in fish-flower (mint) symbiosis. AMB Expr. (2023) 13:46. doi: 10.1186/s13568-023-01549-4
6. Flores EM, Nguyen AT, Odem MA, Eisenhoffer GT, Krachler AM. The zebrafish as a model for gastrointestinal tract–microbe interactions. Cell Microbiol. (2020) 22:1–15. doi: 10.1111/cmi.13152
7. Zhang D, Zheng Y, Wang X, Wang D, Luo H, Zhu W, et al. Effects of dietary fish meal replaced by fish steak meal on growth performance, antioxidant capacity, intestinal health and microflora, inflammatory response, and protein metabolism of large yellow croaker. Larimichthys crocea. Aquac Nutr. (2023) 2023:1–13. doi: 10.1155/2023/2733234
8. Bao W, He Y, Yu J, Liu M, Yang X, Ta N, et al. Regulatory effect of lactiplantibacillus plantarum 2-33 on intestinal microbiota of mice with antibiotic-associated diarrhea. Front Nutr. (2022) 9:921875. doi: 10.3389/fnut.2022.921875
9. Tran NT, Liang H, Li J, Deng T, Zhang M, Li S. Health benefits of butyrate and its producing bacterium, Clostridium butyricum, on aquatic animals. Fish Shellfish Immunol Rep. (2023) 4:100088–8. doi: 10.1016/j.fsirep.2023.100088
10. Mirghaed AT, Yarahmadi P, Soltani M, Paknejad H, Hoseini SM. Dietary sodium butyrate (Butirex® C4) supplementation modulates intestinal transcriptomic responses and augments disease resistance of rainbow trout (Oncorhynchus mykiss). Fish Shellfish Immunol. (2019) 92:621–8. doi: 10.1016/j.fsi.2019.06.046
11. Li H, Zhou Y, Ling H, Luo L, Qi D, Feng L. The effect of dietary supplementation with Clostridium butyricum on the growth performance, immunity, intestinal microbiota and disease resistance of tilapia (Oreochromis niloticus). PloS One. (2019) 14:e0223428. doi: 10.1371/journal.pone.0223428
12. Zhou L, Lian K, Wang M, Jing X, Zhang Y, Cao J. The antimicrobial effect of a novel peptide LL-1 on Escherichia coli by increasing membrane permeability. BMC Microbiol. (2022) 22:220. doi: 10.1186/s12866-022-02621-y
13. Dong J, Zhang D, Li J, Liu Y, Zhou S, Yang Y, et al. Genistein inhibits the pathogenesis of aeromonas hydrophila by disrupting quorum sensing mediated biofilm formation and aerolysin production. Front Pharmacol. (2021) 12:753581. doi: 10.3389/fphar.2021.753581
14. Ye JD, Wang K, Li FD, Sun YZ. Single or combined effects of fructo- and mannan oligosaccharide supplements and Bacillus clausii on the growth, feed utilization, body composition, digestive enzyme activity, innate immune response and lipid metabolism of the Japanese flounder Paralichth. Aquac Nutr. (2011) 17:e902–11. doi: 10.1111/j.1365-2095.2011.00863.x
15. Saputri FA, Kang D, Kusuma ASW, Rusdiana T, Hasanah AN, Mutakin, et al. Lactobacillus plantarum IS-10506 probiotic administration increases amlodipine absorption in a rabbit model. J Int Med Res. (2018) 46:5004–10. doi: 10.1177/0300060518788994
16. Mirzabekyan S, Harutyunyan N, Manvelyan A, Malkhasyan L, Balayan M, Miralimova S, et al. Fish probiotics: cell surface properties of fish intestinal. Lactobacilli Escherichia coli. Microorganisms. (2023) 11:595. doi: 10.3390/microorganisms11030595
17. Poolsawat L, Li X, He M, Ji D, Leng X. Clostridium butyricum as probiotic for promoting growth performance, feed utilization, gut health and microbiota community of tilapia (Oreochromis niloticus × O. aureus). Aquac Nutr. (2019) 26:657–70. doi: 10.1111/anu.13025
18. Son VM, Chang C-C, Wu M-C, Guu Y-K, Chiu C-H, Cheng W. Dietary administration of the probiotic, Lactobacillus plantarum, enhanced the growth, innate immune responses, and disease resistance of the grouper Epinephelus coioides. Fish Shellfish Immunol. (2009) 26:691–8. doi: 10.1016/j.fsi.2009.02.018
19. Xu W, Hu X, Li H, Tian X, Ouyang Z, Du Y, et al. Effects of Lactobacillus plantarum Ep-M17 on growth, immunity and intestinal microbiota of Penaeus vannamei under Microcystin-LR stress. Aquat Toxicol. (2023) 265:106763. doi: 10.1016/j.aquatox.2023.106763
20. Van Doan H, Hoseinifar SH, Tapingkae W, Seel-Audom M, Jaturasitha S, Dawood MAO, et al. Boosted Growth Performance, Mucosal and Serum Immunity, and Disease Resistance Nile Tilapia (Oreochromis niloticus) Fingerlings Using Corncob-Derived Xylooligosaccharide and Lactobacillus plantarum CR1T5. Probiotics Antimicrob Proteins. (2019) 12:400–11. doi: 10.1007/s12602-019-09554-5
21. Mazlumi A, Panahi B, Hejazi MA, Nami Y. Probiotic potential characterization and clustering using unsupervised algorithms of lactic acid bacteria from saltwater fish samples. Sci Rep. (2022) 12:11952. doi: 10.1038/s41598-022-16322-z
22. Jiang N, Hong B, Luo K, Li Y, Fu H, Wang J. Isolation of Bacillus subtilis and Bacillus pumilus with Anti-Vibrio parahaemolyticus Activity and Identification of the Anti-Vibrio parahaemolyticus Substance. Microorganisms. (2023) 11:1667. doi: 10.3390/microorganisms11071667
23. Guimarães MC, Cerezo IM, Fernandez-Alarcon MF, Natori MM, Sato LY, Kato CAT, et al. Oral Administration of Probiotics (Bacillus subtilis and Lactobacillus plantarum) in Nile Tilapia (Oreochromis niloticus) Vaccinated and Challenged with Streptococcus agalactiae. Fishes. (2022) 7:211. doi: 10.3390/fishes7040211
24. Gao Q, Xiao C, Min M, Zhang C, Peng S, Shi Z. Effects of probiotics dietary supplementation on growth performance, innate immunity and digestive enzymes of silver pomfret. Pampus argenteus. Indian J Anim Res. (2016) 50:936–41. doi: 10.18805/ijar.9640
25. Pan L, Li W, Xie R, Liu H, Tan B, Dong X, et al. Effects of Clostridium butyricum on Growth Performance, Intestinal Health, and Disease Resistance of Hybrid Grouper (Epinephelus fuscoguttatus ♀ × E. lanceolatus ♂) Fed with Cottonseed Protein Concentrate (CPC) Replacement of Fishmeal. Aquac Nutr. (2023) 2023:1–14. doi: 10.1155/2023/1184252
26. Cummins CS, Johnson JL. Taxonomy of the clostridia: wall composition and DNA homologies in clostridium butyricum and other butyric acid-producing clostridia. J Gen Microbiol. (1971) 67:33–46. doi: 10.1099/00221287-67-1-33
27. Li W, Pan L, Liu H, Tan B, Dong X, Yang Q, et al. Effects of the Clostridium butyricum on growth performance, antioxidant capacity, immunity and disease resistance of Litopenaeus vannamei fed with cottonseed protein concentrate (CPC) replacement of fishmeal in diet. Fish Shellfish Immunol. (2022) 126:283–91. doi: 10.1016/j.fsi.2022.05.030
28. Zhang M, Dong B, Lai X, Chen Z, Hou L, Shu R, et al. Effects of Clostridium butyricum on growth, digestive enzyme activity, antioxidant capacity and gut microbiota in farmed tilapia (Oreochromis niloticus). Aquac Res. (2020) 52:1573–84. doi: 10.1111/are.15009
29. Tran NT, Li Z, Ma H, Zhang Y, Zheng H, Gong Y, et al. Clostridium butyricum: a promising probiotic confers positive health benefits in aquatic animals. Rev Aquacult. (2020) 12:2573–89. doi: 10.1111/raq.12459
30. Li T, Ke F, Gui J-F, Zhou L, Zhang X-J, Zhang Q-Y. Protective effect of Clostridium butyricum against Carassius auratus herpesvirus in gibel carp. Aquacult Int. (2019) 27:905–14. doi: 10.1007/s10499-019-00377-3
31. Li H, Tian X, Dong S. Growth performance, non-specific immunity, intestinal histology and disease resistance of Litopenaeus vannamei fed on a diet supplemented with live cells of Clostridium butyricum. Aquaculture. (2019) 498:470–81. doi: 10.1016/j.aquaculture.2018.09.003
32. Foysal MJ, Nguyen TTT, Chaklader MR, Siddik MAB, Tay C-Y, Fotedar R, et al. Marked variations in gut microbiota and some innate immune responses of fresh water crayfish, marron (Cherax cainii, Austin 2002) fed dietary supplementation of. Clostridium butyricum. PeerJ. (2019) 7:e7553. doi: 10.7717/peerj.7553
33. Duan Y, Zhang Y, Dong H, Wang Y, Zheng X, Zhang J. Effect of dietary Clostridium butyricum on growth, intestine health status and resistance to ammonia stress in Pacific white shrimp Litopenaeus vannamei. Fish Shellfish Immunol. (2017) 65:25–33. doi: 10.1016/j.fsi.2017.03.048
34. Chen D, Jin D, Huang S, Wu J, Xu M, Liu T, et al. Clostridium butyricum, a butyrate-producing probiotic, inhibits intestinal tumor development through modulating Wnt signaling and gut microbiota. Cancer Lett. (2019) 469:456–67. doi: 10.1016/j.canlet.2019.11.019
35. Cassir N, Benamar S, La Scola B. Clostridium butyricum: from beneficial to a new emerging pathogen. Clin Microbiol Infect. (2015) 22:37–45. doi: 10.1016/j.cmi.2015.10.014
36. Lazado CC, Caipang CMA, Estante EG. Prospects of host-associated microorganisms in fish and penaeids as probiotics with immunomodulatory functions. Fish Shellfish Immunol. (2015) 45:2–12. doi: 10.1016/j.fsi.2015.02.023
37. Lazado CC, Caipang CMA. Bacterial viability differentially influences the immunomodulatory capabilities of potential host-derived probiotics in the intestinal epithelial cells of Atlantic cod. Gadus morhua. J Appl Microbiol. (2013) 116:990–8. doi: 10.1111/jam.12414
38. Hai van N. A review of probiotics in shrimp aquaculture. J Appl Aquac. (2010) 22:251–66. doi: 10.1080/10454438.2010.500597
39. Nayak SK. Probiotics and immunity: a fish perspective. Fish Shellfish Immunol. (2010) 29:2–14. doi: 10.1016/j.fsi.2010.02.017
40. Zhang W, Tan B, Deng J, Haitao Z. Multiomics analysis of soybean meal induced marine fish enteritis in juvenile pearl gentian grouper, Epinephelus fuscoguttatus ♀ × Epinephelus lanceolatus ♂. Sci Rep. (2021) 11:22319. doi: 10.1038/s41598-021-02278-z
41. Wang D, Cao Z, Xie D, Gan X. Research progress in Epinephelus industry. J South Agric. (2012) 43:1058–65. doi: 10.1007/s11783-011-0280-z
42. Gao X, Liu X, Wang Y, Wang T, Fang D, Hu K. Effects of Clostridium butyricum on Intestinal Microflora and Metabolism of Eriocheir sinensis. IJMS. (2023) 24:13784. doi: 10.3390/ijms241813784
43. Ye JD, Liu XH, Wang ZJ, Wang K. Effect of partial fish meal replacement by soybean meal on the growth performance and biochemical indices of juvenile Japanese flounder. Paralichthys olivaceus. Aquacult Int. (2010) 19:143–53. doi: 10.1007/s10499-010-9348-1
44. Cunniff P, AOAC International. Official methods of analysis of AOAC international. Gaithersburg, MD: AOAC International (1999).
45. Meng X, Wu S, Hu W, Zhu Z, Yang G, Zhang Y, et al. Clostridium butyricum improves immune responses and remodels the intestinal microbiota of common carp (Cyprinus carpio L.). Aquaculture. (2020) 530:735753–3. doi: 10.1016/j.aquaculture.2020.735753
46. Du Y, Cheng L, Zhao J, de Cruz CR, Xu H, Wang L, et al. Effects of Clostridium butyricum and sodium butyrate on growth performance, immunity, and gut microbiota of mirror carp Cyprinus carpio fed with soybean meal based diet. Aquac Rep. (2023) 29:101501. doi: 10.1016/j.aqrep.2023.101501
47. Pan X, Wu T, Song Z, Tang H, Zhao Z. Immune responses and enhanced disease resistance in Chinese drum, Miichthys miiuy (Basilewsky), after oral administration of live or dead cells of Clostridium butyrium CB2. J Fish Dis. (2008) 31:679–86. doi: 10.1111/j.1365-2761.2008.00955.x
48. Zhang C, Yu Q, Wang J, Yu Y, Zhang Y, Sun Y. Effects of dietary supplementation with clostridium butyricum on growth performance, apparent digestibility, blood metabolites, ruminal fermentation and bacterial communities of fattening goats. Front Nutr. (2022) 9:888191. doi: 10.3389/fnut.2022.888191
49. Li Y, Wang Y, Lv J, Dou X, Zhang Y. Effects of dietary supplementation with clostridium butyricum on the amelioration of growth performance, rumen fermentation, and rumen microbiota of holstein heifers. Front Nutr. (2021) 8:763700. doi: 10.3389/fnut.2021.763700
50. Li W, Xu B, Wang L, Sun Q, Deng W, Wei F, et al. Effects of clostridium butyricum on growth performance, gut microbiota and intestinal barrier function of broilers. Front Microbiol. (2021) 12:777456. doi: 10.3389/fmicb.2021.777456
51. Qi Y, Liu G, Jin S, Jian R, Zou Z, Wang C, et al. Neuroprotective effect of acetoxypachydiol against oxidative stress through activation of the Keap1-Nrf2/HO-1 pathway. BMC Complement Med Ther. (2024) 24:175. doi: 10.1186/s12906-024-04474-6
52. Reis B, Ramos-Pinto L, Cunha SA, Pintado M, da Silva JL, Dias J, et al. Chlorella vulgaris Extracts as Modulators of the Health Status and the Inflammatory Response of Gilthead Seabream Juveniles (Sparus aurata). Mar Drugs. (2022) 20:407. doi: 10.3390/md20070407
53. Li H, Tian X, Zhao K, Jiang W, Dong S. Effect of Clostridium butyricum in different forms on growth performance, disease resistance, expression of genes involved in immune responses and mTOR signaling pathway of Litopenaeus vannamai. Fish Shellfish Immunol. (2018) 87:13–21. doi: 10.1016/j.fsi.2018.12.069
54. Duan Y, Dong H, Wang Y, Zhang Y, Zhang J. Effects of the Dietary Probiotic Clostridium butyricum on Intestine Digestive and Metabolic Capacities, SCFA Content and Body Composition in Marsupenaeus japonicus. J Ocean Univ China. (2018) 17:690–6. doi: 10.1007/s11802-018-3464-3
55. Zhao H, Jia L, Yan Q, Deng Q, Wei B. Effect of clostridium butyricum and butyrate on intestinal barrier functions: study of a rat model of severe acute pancreatitis with intra-abdominal hypertension. Front Physiol. (2020) 11:561061. doi: 10.3389/fphys.2020.561061
56. Kawasaki S, Nakagawa T, Nishiyama Y, Benno Y, Uchimura T, Komagata K, et al. Effect of oxygen on the growth of Clostridium butyricum (type species of the genus Clostridium), and the distribution of enzymes for oxygen and for active oxygen species in Clostridia. J Ferment Bioeng. (1998) 86:368–72. doi: 10.1016/s0922-338x(99)89006-0
57. Song Z, Wu T, Cai L, Zhang L, Zheng X. Effects of dietary supplementation with Clostridium butyricum on the growth performance and humoral immune response in Miichthys miiuy. J Zhejiang Univ - Sci B. (2006) 7:596–602. doi: 10.1631/jzus.2006.b0596
58. Yin Z, Liu Q, Liu Y, Gao S, He Y, Yao C, et al. Early Life Intervention Using Probiotic Clostridium butyricum Improves Intestinal Development, Immune Response, and Gut Microbiota in Large Yellow Croaker (Larimichthys crocea) Larvae. Front Immunol. (2021) 12:640767. doi: 10.3389/fimmu.2021.640767
59. Khorshidian N, Khanniri E, Koushki MR, Sohrabvandi S, Yousefi M. An overview of antimicrobial activity of lysozyme and its functionality in cheese. Front Nutr. (2022) 9:833618. doi: 10.3389/fnut.2022.833618
60. Mu Q, Dong Z, Kong W, Wang X, Yu J, Ji W, et al. Response of immunoglobulin M in gut mucosal immunity of common carp (Cyprinus carpio) infected with. Aeromonas hydrophila. Front Immunol. (2022) 13:1037517. doi: 10.3389/fimmu.2022.1037517
61. Zhang L, Cao GT, Zeng XF, Zhou L, Ferket PR, Xiao YP, et al. Effects of Clostridium butyricum on growth performance, immune function, and cecal microflora in broiler chickens challenged with Escherichia coli K88. Poult Sci. (2014) 93:46–53. doi: 10.3382/ps.2013-03412
62. Yang CM, Cao GT, Ferket PR, Liu TT, Zhou L, Zhang L, et al. Effects of probiotic, Clostridium butyricum, on growth performance, immune function, and cecal microflora in broiler chickens. Poult Sci. (2012) 91:2121–9. doi: 10.3382/ps.2011-02131
63. Wang X, Luo H, Wang D, Zheng Y, Zhu W, Zhang W, et al. Partial substitution of fish meal with soy protein concentrate on growth, liver health, intestinal morphology, and microbiota in juvenile large yellow croaker (Larimichthys crocea). Aquac Nutr. (2023) 2023:1–15. doi: 10.1155/2023/3706709
64. Li H, Liu Y, Wei L, Lin Q, Zhang Z. Effects of feeding fermented medicago sativa (Plus soybean and DDGS) on growth performance, blood profiles, gut health, and carcass characteristics of lande (Meat) geese. Front Physiol. (2022) 13:902802. doi: 10.3389/fphys.2022.902802
65. Jiao F, Zhang L, Limbu SM, Yin H, Xie Y, Yang Z, et al. A comparison of digestive strategies for fishes with different feeding habits: Digestive enzyme activities, intestinal morphology, and gut microbiota. Ecol Evol. (2023) 13:1–14. doi: 10.1002/ece3.10499
66. Ye G, Dong X, Yang Q, Chi S, Liu H, Zhang H, et al. Low-gossypol cottonseed protein concentrate used as a replacement of fish meal for juvenile hybrid grouper (Epinephelus fuscoguttatus ♀ × Epinephelus lanceolatus ♂): Effects on growth performance, immune responses and intestinal microbiota. Aquaculture. (2020) 524:735309. doi: 10.1016/j.aquaculture.2020.735309
67. Liu ZY, Yang HL, Hu LH, Yang W, Ai CX, Sun YZ. Autochthonous probiotics alleviate the adverse effects of dietary histamine in juvenile grouper (Epinephelus coioides). Front Microbiol. (2021) 12:792718. doi: 10.3389/fmicb.2021.792718
68. Fan C. The effect of butyrate-producing bacteria on the intestinal health of Procambarus clarkii. Huazhong Agricultural University, Wuhan, China (2021). Master's thesis.
69. Louis P, Flint HJ. Formation of propionate and butyrate by the human colonic microbiota. Environ Microbiol. (2016) 19:29–41. doi: 10.1111/1462-2920.13589
70. den Besten G, van Eunen K, Groen AK, Venema K, Reijngoud D-J, Bakker BM. The role of short-chain fatty acids in the interplay between diet, gut microbiota, and host energy metabolism. J Lipid Res. (2013) 54:2325–40. doi: 10.1194/jlr.r036012
71. Li H, Liu S, Zhang K, Zhu X, Dai J, Lu Y. Gut microbiome and plasma metabolome alterations in myopic mice. Front Microbiol. (2023) 14:1251243. doi: 10.3389/fmicb.2023.1251243
72. Karlsson FH, Ussery DW, Nielsen J, Nookaew I. A closer look at bacteroides: phylogenetic relationship and genomic implications of a life in the human gut. Microb Ecol. (2011) 61:473–85. doi: 10.1007/s00248-010-9796-1
73. Lu J, Yao J, Xu Q, Zheng Y, Dong X. Clostridium butyricum relieves diarrhea by enhancing digestive function, maintaining intestinal barrier integrity, and relieving intestinal inflammation in weaned piglets. Livest Sci. (2020) 239:104112. doi: 10.1016/j.livsci.2020.104112
74. Fu J, Wang T, Xiao X, Cheng Y, Wang F, Jin M, et al. Clostridium butyricum ZJU-F1 benefits the intestinal barrier function and immune response associated with its modulation of gut microbiota in weaned piglets. Cells. (2021) 10:527–7. doi: 10.3390/cells10030527
75. Robinson K, Deng Z, Hou Y, Zhang G. Regulation of the intestinal barrier function by host defense peptides. Front Vet Sci. (2015) 2:57. doi: 10.3389/fvets.2015.00057
76. Zhu H, Lu J, Wang X, Zhang H, Tang X, Zhu J, et al. Upregulated ZO-1 correlates with favorable survival of gastrointestinal stromal tumor. Med Oncol. (2013) 30:1–7. doi: 10.1007/s12032-013-0631-7
Keywords: Clostridium butyricum, hybrid grouper, growth, immune function, gut microbiota
Citation: Chen W, Song T, Li D, Chen M, Wang P and Ye J (2025) Effect of dietary Clostridium butyricum supplementation on growth performance, immune function, and intestinal health of hybrid grouper (Epinephelus fuscoguttatus ♀ × Epinephelus lanceolatus ♂). Front. Immunol. 16:1557256. doi: 10.3389/fimmu.2025.1557256
Received: 08 January 2025; Accepted: 10 February 2025;
Published: 26 February 2025.
Edited by:
Liansheng Wang, Chinese Academy of Fishery Sciences, ChinaReviewed by:
Xian-de Huang, South China Agricultural University, ChinaCopyright © 2025 Chen, Song, Li, Chen, Wang and Ye. This is an open-access article distributed under the terms of the Creative Commons Attribution License (CC BY). The use, distribution or reproduction in other forums is permitted, provided the original author(s) and the copyright owner(s) are credited and that the original publication in this journal is cited, in accordance with accepted academic practice. No use, distribution or reproduction is permitted which does not comply with these terms.
*Correspondence: Jidan Ye, eWp5amRAam11LmVkdS5jbg==
Disclaimer: All claims expressed in this article are solely those of the authors and do not necessarily represent those of their affiliated organizations, or those of the publisher, the editors and the reviewers. Any product that may be evaluated in this article or claim that may be made by its manufacturer is not guaranteed or endorsed by the publisher.
Research integrity at Frontiers
Learn more about the work of our research integrity team to safeguard the quality of each article we publish.