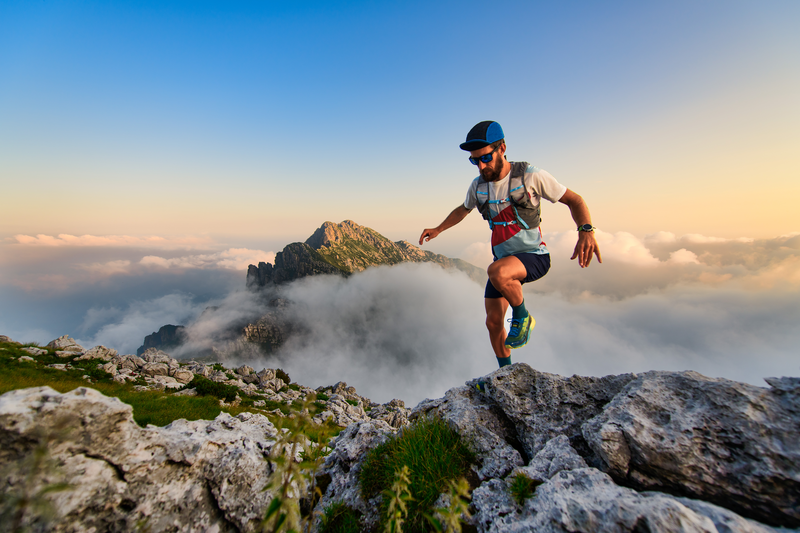
94% of researchers rate our articles as excellent or good
Learn more about the work of our research integrity team to safeguard the quality of each article we publish.
Find out more
REVIEW article
Front. Immunol.
Sec. Cancer Immunity and Immunotherapy
Volume 16 - 2025 | doi: 10.3389/fimmu.2025.1557223
This article is part of the Research Topic Harnessing Big Data for Precision Medicine: Revolutionizing Diagnosis and Treatment Strategies View all 25 articles
The final, formatted version of the article will be published soon.
You have multiple emails registered with Frontiers:
Please enter your email address:
If you already have an account, please login
You don't have a Frontiers account ? You can register here
Hepatocellular carcinoma (HCC) presents a significant global health challenge, particularly in developing countries where its incidence is markedly elevated. Despite advancements in treatment modalities, the high malignancy, metastatic potential, and drug resistance associated with HCC contribute to poor clinical outcomes, underscoring the necessity for a more profound understanding of its pathogenesis. This review meticulously examines the role of copper apoptosis, a novel form of programmed cell death linked to dysregulated copper metabolism, in the development and progression of HCC. By conducting a comprehensive review of recent literature and experimental studies, we elucidate the molecular mechanisms through which excess copper induces oxidative stress, pyroptosis, and ferroptosis, thereby influencing tumorigenesis and progression. This review offers valuable insights into the intricate relationship between copper metabolism and HCC, positioning copper apoptosis as a potential therapeutic target to enhance treatment strategies and improve patient outcomes.
Keywords: Hepatocellular Carcinoma, cuproptosis, prognosis, treatment resistance, immune microenvironment
Received: 08 Jan 2025; Accepted: 24 Feb 2025.
Copyright: © 2025 Zhu, Zhao, Wang, Wei and Liu. This is an open-access article distributed under the terms of the Creative Commons Attribution License (CC BY). The use, distribution or reproduction in other forums is permitted, provided the original author(s) or licensor are credited and that the original publication in this journal is cited, in accordance with accepted academic practice. No use, distribution or reproduction is permitted which does not comply with these terms.
* Correspondence:
Guohua Wei, Nantong Tumor Hospital, Nantong, 226000, Jiangsu Province, China
Jibin Liu, Nantong Tumor Hospital, Nantong, 226000, Jiangsu Province, China
Disclaimer: All claims expressed in this article are solely those of the authors and do not necessarily represent those of their affiliated organizations, or those of the publisher, the editors and the reviewers. Any product that may be evaluated in this article or claim that may be made by its manufacturer is not guaranteed or endorsed by the publisher.
Research integrity at Frontiers
Learn more about the work of our research integrity team to safeguard the quality of each article we publish.