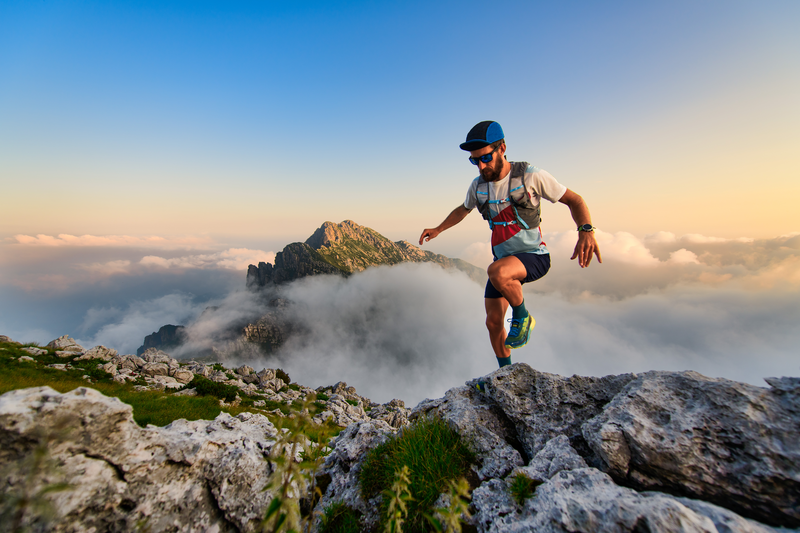
95% of researchers rate our articles as excellent or good
Learn more about the work of our research integrity team to safeguard the quality of each article we publish.
Find out more
OPINION article
Front. Immunol. , 17 March 2025
Sec. Cytokines and Soluble Mediators in Immunity
Volume 16 - 2025 | https://doi.org/10.3389/fimmu.2025.1557176
This article is part of the Research Topic Cytokine Network Dynamics: Influence on Autoimmune Disorders and Cancer Therapy View all 8 articles
The immune response is orchestrated by a complex network of cytokines, which play a fundamental role in both autoimmune diseases and cancer therapy. Immune checkpoint inhibitors (ICIs), which target key inhibitory pathways such as PD-1/PD-L1 and CTLA-4, have revolutionized cancer immunotherapy by reinstating T-cell activity and overcoming immune evasion mechanisms (1). Despite their success in cancers like melanoma and lung cancer, a substantial number of patients fail to respond to ICIs. This resistance is often due to the lack of pre-existing T-cell immunity, the emergence of immune resistance mechanisms, or an immunosuppressive tumor microenvironment (TME), highlighting the need for more targeted therapeutic strategies (2). In this context, cytokines and their receptor networks, particularly those within the tumor necrosis factor superfamily (TNFSF) and its receptors (TNFRSF), have emerged as pivotal players in regulating immune responses. Figure 1 illustrates the TNFSF signaling pathway. It shows the structure of TNFSF in its unbound state and its interaction with the TNFSF receptor. Upon binding, the TNFSF receptor forms a receptor-ligand complex, as depicted in the middle portion. The lower part highlights the downstream signaling events following receptor-ligand clustering. This clustering activates the TRAF2 signaling complex, which plays a critical role in modulating immune responses. These interactions are fundamental to immune regulation and enhance the therapeutic potential of targeting TNFSF pathways (3). Cytokines such as OX40, 4-1BB, and CD40 promote T-cell activation, survival, and proliferation, contributing to both anti-tumor immunity and immune homeostasis (3). However, their therapeutic potential is often constrained by T-cell exhaustion and the immunosuppressive effects of the TME, which can limit their efficacy, similarly to ICIs (4).
Figure 1. TNFSF and receptor-ligand complex formation. The structure of TNFSF (light purple) in its unbound state (left), followed by its binding to the TNFSF receptor (green, blue, and pink) (middle). The right panel shows the receptor-ligand complex, with the TNFSF (orange) bound to the receptor, initiating the formation of the signaling complex. The lower part illustrates how ligand-receptor clustering triggers signaling activation, leading to the formation of the TRAF2 signaling complex, essential for modulating immune responses.
To overcome these limitations, combining ICIs with TNFSF agonists presents a synergistic strategy. While ICIs unmask immune suppression and restore T-cell responses, TNFSF agonists, like OX40 and 4-1BB, can enhance T-cell activation and proliferation, providing a more robust immune response (5). Furthermore, TNFR2 agonists could modulate immune regulation, particularly by influencing regulatory T-cell activity, offering potential benefits for autoimmune diseases.
However, the integration of ICIs and TNFSF agonists also faces challenges, such as immune-related adverse events (irAEs), optimal dosing strategies, and identifying patients who would benefit the most from these therapies (6). Precision medicine, guided by biomarkers and advanced diagnostic techniques, will be critical in overcoming these hurdles. This Opinion Article explores how combining ICIs with TNFSF agonists could offer a novel approach for modulating cytokine networks and advancing treatment outcomes in both autoimmune diseases and cancer.
ICIs have revolutionized cancer therapy by targeting inhibitory pathways such as PD-1, PD-L1, and CTLA-4, restoring T-cell activity and enhancing tumor elimination. However, ICIs often fail in patients with low T-cell infiltration or TME, emphasizing the need for complementary strategies (7). TNFSF agonists, targeting receptors like OX40, 4-1BB, and CD40, provide such a complement by amplifying T-cell activation and proliferation through co-stimulatory signals (8). OX40 agonists enhance CD4+ and CD8+ T-cell responses, while 4-1BB agonists promote CD8+ T-cell cytotoxicity and natural killer (NK) cell activity. Similarly, CD40 agonists stimulate antigen-presenting cells (APCs), fostering robust adaptive immunity. These agonists can reduce T-cell exhaustion and counteract the immunosuppressive influences of regulatory T cells (Tregs) and myeloid-derived suppressor cells (MDSCs) within the TME. Table 1 summarizes key TNFSF agonists in clinical development, including Urelumab and Selicrelumab for cancer and ABBV-927 for autoimmune diseases. These agents highlight the diverse applications and potential of TNFSF agonists in combination therapies. However, combining these potent immunotherapies raises concerns about immune-related adverse events (irAEs), such as cytokine release syndrome and autoimmunity. Careful patient selection, biomarker-driven stratification, and optimized dosing strategies are essential to mitigate these risks.
The synergy between ICIs and TNFSF agonists arises from their complementary mechanisms. ICIs unmask suppressed T-cell activity, creating a fertile ground for TNFSF agonists to amplify immune responses. Preclinical and early clinical data demonstrate that combinations like PD-1 blockade with 4-1BB or OX40 agonists significantly enhance T-cell infiltration, tumor regression, and survival.
Recent preclinical and clinical studies have highlighted the potential benefits of combining ICIs with TNFSF agonists. TNFSF agonists activate immune effector functions by enhancing T-cell proliferation, survival, and cytokine production while reducing T-cell exhaustion. For example, OX40 agonists promote CD4+ and CD8+ T-cell responses and facilitate the generation of memory T cells, which are crucial for long-term anti-tumor immunity. Similarly, 4-1BB agonists increase the cytotoxic capacity of CD8+ T cells and natural killer (NK) cells, amplifying their ability to target tumor cells. CD40 agonists, on the other hand, enhance the antigen-presenting capacity of dendritic cells and macrophages, promoting the activation of tumor-specific T cells (18).
When combined with ICIs, these effects are further magnified. ICIs release the brakes on T-cell activity by blocking inhibitory pathways like PD-1/PD-L1 and CTLA-4, allowing TNFSF agonists to act as accelerators that drive immune responses to a higher magnitude. Preclinical models have demonstrated that the combination of PD-1 blockade and 4-1BB agonists significantly improves tumor infiltration by effector T cells and reduces the suppressive influence of regulatory Tregs and myeloid-derived suppressor cells (MDSCs). Similarly, combining OX40 agonists with CTLA-4 inhibitors has shown superior anti-tumor efficacy compared to either agent alone, underscoring the synergistic potential of these therapies (19, 20). The combination of OX40 agonists and CTLA-4 inhibitors has shown both positive and inconclusive results in clinical trials. In preclinical studies, it promotes robust anti-tumor activity and tumor-free survival. However, in some clinical trials, the combination therapies with ipilimumab (a CTLA-4 inhibitor) and OX40 agonists like BMS-986178 did not improve the clinical response rate compared to monotherapy. The safety profiles of these combinations varied, with common side effects including lymphopenia, rash, pyrexia, and fatigue. Further research is needed to optimize this combination for better clinical outcomes (21).
Clinical trials have started to explore these combinations in various cancers, including melanoma, non-small cell lung cancer, and colorectal cancer. Early-phase trials of 4-1BB agonists with PD-1 inhibitors have shown promising results, with improved objective response rates and progression-free survival (22–24). Furthermore, the combination of PD-1 blockade and 4-1BB agonists shows a synergistic survival benefit in a CD8+ T-cell-dependent manner in murine gliomas. It reduces TIL exhaustion and improves TIL functionality. Efficacy correlates with 4-1BB expression on CD8+ TILs, not with tumor location or histology, and can license combination therapy in models where TIL 4-1BB levels were previously low (25). OX40 agonists are also being investigated in combination with ICIs, with preliminary data suggesting enhanced tumor regression and durable responses (26–28). Furthermore, CD40 agonists, when paired with ICIs, have demonstrated the ability to convert immunologically cold tumors into hot ones, rendering them more susceptible to immune attack (29, 30). In an ongoing Phase I/II study, the combination of intratumoral sotigalimab (CD40 agonist) and systemic pembrolizumab (anti-PD-1) was evaluated in treatment-naïve, unresectable stage III/IV metastatic melanoma patients. As of December 2021, 30 participants were enrolled, and the combination was well-tolerated with no treatment-related discontinuations or deaths. The most common adverse events were injection-site reactions, with 6 patients experiencing grade-3 immune-related adverse events. The overall response rate (ORR) was 50% (5 complete responses, 10 partial responses), with a disease control rate of 67%. Notably, responses were observed in PD-L1 negative patients and those with elevated LDH. Immunologic analyses showed increased immune activation, including higher T cell clonality and gene expression related to antigen presentation, correlating with clinical responses. This combination therapy demonstrated promising efficacy and immune activation, warranting further investigation (31).
Despite the encouraging progress, significant challenges remain in realizing the full potential of ICI and TNFSF agonist combinations. One of the primary concerns is the risk of irAEs. Both ICIs and TNFSF agonists can independently induce immune activation-related toxicities, such as cytokine release syndrome (CRS), autoimmunity, and systemic inflammation. When combined, these therapies may exacerbate such adverse effects, necessitating careful dose optimization and patient monitoring (32).
Another challenge lies in target specificity. TNFSF receptors are often expressed on multiple cell types, including Tregs and activated T cells, leading to potential off-target effects. For instance, while TNFR2 agonists can enhance Treg function in autoimmune settings, their role in the TME may be context-dependent, potentially favoring either immune suppression or activation. Similarly, OX40 and 4-1BB agonists may inadvertently activate pathogenic T-cell subsets, complicating therapeutic outcomes. Patient stratification and biomarker identification also pose significant hurdles. The heterogeneity of immune responses across different cancers and patient populations requires a precision medicine approach to identify those most likely to benefit from these combinations. Biomarkers such as PD-L1 expression, T-cell infiltration, and TNFSF receptor expression levels could guide patient selection, but their predictive value needs further validation in clinical trials (33).
Finally, logistical challenges in trial design and execution must be addressed (34). Combining ICIs and TNFSF agonists requires careful consideration of dosing schedules, administration sequences, and combination partners to maximize efficacy while minimizing toxicity. Developing standardized protocols and establishing robust clinical endpoints are critical to accelerating the translation of these therapies into practice.
The combination of ICIs and TNFSF agonists offers significant opportunities in the treatment of autoimmune diseases, addressing the delicate balance between immune activation and suppression. ICIs, such as PD-1/PD-L1 inhibitors, have shown potential in modulating immune responses in autoimmune contexts, but their broad activation of T cells often leads to the risk of exacerbating autoimmunity (35, 36). TNFSF agonists, by contrast, provide a more targeted approach, leveraging their co-stimulatory or regulatory properties to fine-tune immune responses (37).
For example, TNFR2 agonists have emerged as promising candidates for promoting Treg expansion, which is critical in maintaining immune tolerance and mitigating hyperactive immune responses (38). This mechanism is particularly relevant in diseases like rheumatoid arthritis, where inflammation driven by effector T cells and myeloid pathways is predominant. Studies have demonstrated that TNFR2 activation not only enhances Treg function but also reduces the activity of inflammatory cells such as macrophages and dendritic cells. Additionally, OX40 and DR3 agonists have shown potential in selectively targeting pathogenic T-cell subsets without overactivating the entire immune system. This specificity is especially valuable in chronic autoimmune conditions like lupus and multiple sclerosis, where the immune system is persistently hyperactive. By restoring immune homeostasis, these therapies could reduce disease flares while minimizing the risk of global immunosuppression (38, 39). Furthermore, in systemic lupus erythematosus (SLE), Anifrolumab has shown positive results in Phase II and III clinical trials. In August 2021, the drug was approved by the FDA for the treatment of moderate to severe active SLE. Clinical trial results demonstrated significant efficacy in improving disease activity and organ-specific markers, particularly in patients with elevated interferon-I levels (40, 41).
The complementary mechanisms of ICIs and TNFSF agonists create unique therapeutic synergies. ICIs can reactivate suppressed immune responses, while TNFSF agonists enhance the regulation and functionality of these responses. For instance, ICIs could reverse T-cell exhaustion in autoimmune diseases with underlying viral infections, while TNFSF agonists like TNFR2 or CD40 could enhance the tolerogenic capacity of immune cells. Furthermore, combining these therapies with existing treatments such as biologics or small-molecule inhibitors opens new avenues for improving outcomes. Targeted use of such combinations has the potential to achieve a more durable remission in diseases traditionally refractory to single-agent therapies, representing a significant advancement in the field of autoimmune therapeutics (42).
The combination of ICIs and TNFSF agonists represents a rapidly evolving area of immunotherapy with vast potential for addressing unmet needs in cancer and autoimmune diseases. However, to fully realize this potential, several key areas demand focused research and innovation.
While first-generation TNFSF agonists have shown promise, their broad activity on multiple cell types poses challenges, including off-target effects and systemic toxicity. Next-generation TNFSF agonists should focus on achieving higher target specificity and controllable activation (43). For example, a recent study demonstrated the development of a bispecific OX40–PD-L1 antibody, which selectively targets tumors while minimizing off-target effects (9, 44, 45). Advances in antibody engineering, such as bispecific antibodies or conditional agonists, could allow for more precise delivery of TNFSF signals to specific immune cells within the tumor microenvironment (TME) or sites of autoimmune pathology. For example, designing agonists that activate only in the presence of high levels of PD-L1 or other markers characteristic of tumors could reduce systemic activation and associated toxicities.
One of the most critical challenges in combinatory immunotherapy is identifying the right patient populations that will benefit from ICIs and TNFSF agonists. Biomarker development is paramount for patient stratification and therapy optimization (46). A study by Zhang et al. (2022) highlighted how TNFSF receptor expression levels can predict the efficacy of TNFSF agonist therapies, especially in patients with high immune infiltration (35). For instance, biomarkers such as PD-L1 expression, immune cell infiltration (e.g., CD8+ T cells), or TNFRSF receptor density could guide patient selection and predict therapeutic responses. Advances in high-throughput sequencing, single-cell analysis, and artificial intelligence could further enhance our ability to identify predictive and prognostic biomarkers, paving the way for truly personalized immunotherapy.
While ICIs and TNFSF agonists offer complementary benefits, their efficacy may be further amplified when combined with other therapeutic modalities (47). Such as Traditional Therapies: Combining these agents with chemotherapy or radiotherapy could enhance tumor antigen presentation, creating a more immunogenic TME. Targeted Therapies: Small-molecule inhibitors targeting oncogenic pathways could synergize with ICIs and TNFSF agonists by reducing immunosuppressive signaling in tumors. Vaccines and Oncolytic Viruses: Immunotherapy combinations with tumor vaccines or oncolytic viruses may further boost immune activation and generate robust memory T-cell responses. These multimodal approaches could significantly enhance therapeutic outcomes, particularly in refractory cancers or advanced autoimmune diseases. Combining these agents with chemotherapy or radiotherapy could enhance tumor antigen presentation, creating a more immunogenic TME (20).
While the focus of current research is predominantly on cancer therapy, the combination of ICIs and TNFSF agonists holds untapped potential in autoimmune diseases. TNFR2 agonists, for example, could enhance Treg function to counteract immune hyperactivation, while OX40 and DR3 agonists could selectively modulate pathogenic T-cell responses. Expanding clinical investigations to include autoimmune indications such as rheumatoid arthritis, lupus, and multiple sclerosis could unlock new therapeutic avenues. For instance, a Phase II trial evaluating the combination of PD-1 inhibitors and OX40 agonists in lupus patients demonstrated promising results, with increased remission rates (36).
Robust preclinical models that accurately recapitulate human disease are essential for predicting therapeutic efficacy and toxicity (48). Advances in organoid systems, humanized mouse models, and computational simulations could improve the translation of preclinical findings into clinical success. Recent trials have shown that personalized treatment regimens, guided by biomarkers such as immune checkpoint expression, can significantly improve patient outcomes (19). On the clinical front, adaptive trial designs incorporating biomarker-driven stratification and real-time monitoring could accelerate the development of effective combination therapies.
The complexity of combining ICIs and TNFSF agonists necessitates collaboration across disciplines (49). Immunologists, oncologists, computational biologists, and pharmaceutical scientists must work together to integrate insights from basic research, clinical practice, and technological innovation. Partnerships between academia, industry, and regulatory agencies will also be crucial in streamlining the pathway from discovery to clinical implementation. Successful examples of cross-disciplinary collaboration include the development of combination therapies that have progressed through both preclinical and clinical stages (22).
The integration of ICIs and TNFSF agonists provides a promising approach to modulating cytokine networks, enhancing immune responses in both cancer and autoimmune diseases. ICIs work by lifting immune suppression, allowing for the reactivation of T-cell activity, while TNFSF agonists, such as OX40 and 4-1BB, further amplify T-cell activation and overcome the immunosuppressive TME, effectively transforming “cold” tumors into “hot” ones and bolstering immune responses in autoimmune diseases.
Despite the immense potential of this combined approach, several challenges remain, particularly with regard to irAEs, target specificity, and optimizing patient selection. Advances in precision medicine, including biomarker discovery and personalized diagnostics, will be key in identifying patients most likely to benefit from these therapies and minimizing toxicity. Additionally, next-generation TNFSF agonists and combination strategies are essential to further improve efficacy and safety. By integrating ICIs and TNFSF agonists with other therapeutic modalities and fostering interdisciplinary collaboration, this strategy holds significant promise in advancing immunotherapy and providing new hope for patients with both cancer and autoimmune diseases.
LS: Writing – original draft, Writing – review & editing. CL: Writing – original draft. TG: Writing – original draft. ZL: Writing – original draft. YH: Writing – original draft, Writing – review & editing. WH: Writing – original draft, Writing – review & editing.
The author(s) declare that no financial support was received for the research and/or publication of this article.
The authors declare that the research was conducted in the absence of any commercial or financial relationships that could be construed as a potential conflict of interest.
The author(s) declare that no Generative AI was used in the creation of this manuscript.
All claims expressed in this article are solely those of the authors and do not necessarily represent those of their affiliated organizations, or those of the publisher, the editors and the reviewers. Any product that may be evaluated in this article, or claim that may be made by its manufacturer, is not guaranteed or endorsed by the publisher.
1. Zhang D, Zhao J, Zhang Y, Jiang H, Liu D. Revisiting immune checkpoint inhibitors: new strategies to enhance efficacy and reduce toxicity. Front Immunol. (2024) 15:1490129. doi: 10.3389/fimmu.2024.1490129
2. Liu Z, Zhu Y, Xie H, Zou Z. Immune-mediated hepatitis induced by immune checkpoint inhibitors: Current updates and future perspectives. Front Pharmacol. (2023) 13:1077468. doi: 10.3389/fphar.2022.1077468
3. Croft M, Salek-Ardakani S, Ware CF. Targeting the TNF and TNFR superfamilies in autoimmune disease and cancer. Nat Rev Drug Discovery. (2024) 23:939–61. doi: 10.1038/s41573-024-01053-9
4. Zhang N, Liu X, Qin J, Sun Y, Xiong H, Lin B, et al. LIGHT/TNFSF14 promotes CAR-T cell trafficking and cytotoxicity through reversing immunosuppressive tumor microenvironment. Mol Ther. (2023) 31:2575–90. doi: 10.1016/j.ymthe.2023.06.015
5. Kong BY, Bolton H, Kim JW, Silveira PA, Fromm PD, Clark GJ. On the other side: manipulating the immune checkpoint landscape of dendritic cells to enhance cancer immunotherapy. Front Oncol. (2019) 9:50. doi: 10.3389/fonc.2019.00050
6. Montfort A, Virazels M, Colacios C, Meyer N, Ségui B. Combining TNF blockade with immune checkpoint inhibitors in patients with cancer. Nat Rev Rheumatol. (2021) 17:577–7. doi: 10.1038/s41584-021-00653-8
7. Bagchi S, Yuan R, Engleman EG. Immune checkpoint inhibitors for the treatment of cancer: clinical impact and mechanisms of response and resistance. Annu Rev Pathology: Mech Dis. (2021) 16:223–49. doi: 10.1146/annurev-pathol-042020-042741
8. Obermajer N, Zwolak A, Wajant H. Editorial: TNFRSF agonists: mode of action and therapeutic opportunities. Front Immunol. (2023) 14:1328100. doi: 10.3389/fimmu.2023.1328100
9. Kierkus J, Pesegova M, Klopocka M, Brankovic M, Kasai N, Efuni S, et al. : randomized, ascending dose, phase 2 study of KHK4083, an anti-OX40 monoclonal antibody, in moderately active ulcerative colitis. Crohn’s Colitis 360. (2020) 2:otaa049.
10. Claus C, Ferrara-Koller C, Klein C. The emerging landscape of novel 4-1BB (CD137) agonistic drugs for cancer immunotherapy. mAbs. (2023) 15:2167189. doi: 10.1080/19420862.2023.2167189
11. Barve M, Tolcher A, Carvajal R, Izar B, El-Khoueiry A, Hanna D, et al. A phase 1 study of AGEN2373, a novel CD137 agonist antibody designed to avoid hepatoxicity, in patients with advanced solid tumors. J Clin Oncol. (2023) 41:2524–4. doi: 10.1200/JCO.2023.41.16_suppl.2524
12. Byrne KT, Betts CB, Mick R, Sivagnanam S, Bajor DL, Laheru DA, et al. Neoadjuvant selicrelumab, an agonist CD40 antibody, induces changes in the tumor microenvironment in patients with resectable pancreatic cancer. Clin Cancer Res. (2021) 27:4574–86. doi: 10.1158/1078-0432.CCR-21-1047
13. Malhotra D, Turman S, McGlinchey K, Wang Y, Wang Y. Abstract 2267: MEDI5083, a novel CD40L-Fc fusion protein, activates the CD40 pathway on antigen-presenting cells and promotes robust anti-tumor immune responses in both hot and cold murine tumor models. Cancer Res. (2020) 80:2267–7. doi: 10.1158/1538-7445.AM2020-2267
14. Powderly Ii J, Tolcher A, Lorusso P, Blaney M, Dacosta D, Henner W, et al. A phase I study of CD40 agonist ABBV-927 plus OX40 agonist ABBV-368 with or without the PD-1 inhibitor budigalimab in patients with advanced solid tumors. J Clin Oncol. (2020) 38:TPS3147–TPS3147.
15. Sanborn R, Pishvaian M, Callahan M, Weise A, Sikic B, Osama R, et al. Safety, tolerability and efficacy of agonist anti-CD27 antibody (varlilumab) administered in combination with anti-PD-1 (nivolumab) in advanced solid tumors. J ImmunoTherapy Cancer. (2022) 10:e005147. doi: 10.1136/jitc-2022-005147
16. Danese S, Klopocka M, Scherl EJ, Romatowski J, Allegretti JR, Peeva E, et al. Anti-TL1A antibody PF-06480605 safety and efficacy for ulcerative colitis: A phase 2a single-Arm study. Clin Gastroenterol Hepatol. (2021) 19:2324–2332.e2326. doi: 10.1016/j.cgh.2021.06.011
17. Perlin DS, Neil GA, Anderson C, Zafir-Lavie I, Raines S, Ware CF, et al. Randomized, double-blind, controlled trial of human anti-LIGHT monoclonal antibody in COVID-19 acute respiratory distress syndrome. J Clin Invest. (2022) 132. doi: 10.1172/JCI153173
18. Fu Y, Lin Q, Zhang Z, Zhang L. Therapeutic strategies for the costimulatory molecule OX40 in T-cell-mediated immunity. Acta Pharm Sin B. (2020) 10:414–33. doi: 10.1016/j.apsb.2019.08.010
19. Chen S, Lee L-F, Fisher TS, Jessen B, Elliott M, Evering W, et al. Combination of 4-1BB agonist and PD-1 antagonist promotes antitumor effector/memory CD8 T cells in a poorly immunogenic tumor model. Cancer Immunol Res. (2015) 3:149–60. doi: 10.1158/2326-6066.CIR-14-0118
20. Redmond W, Linch S, Kasiewicz M. Combined targeting of costimulatory (OX40) and coinhibitory (CTLA-4) pathways elicits potent effector T cells capable of driving robust antitumor immunity. Cancer Immunol Res. (2014) 2:142–53. doi: 10.1158/2326-6066.CIR-13-0031-T
21. Yadav R, Redmond WL. Current clinical trial landscape of OX40 agonists. Curr Oncol Rep. (2022) 24:951–60. doi: 10.1007/s11912-022-01265-5
22. Falchook G, LoRusso P, Goldman J, El-Khoueiry A, Tolcher A, Xing Y, et al. Phase 1 trial safety and efficacy of ragistomig, a bispecific antibody targeting PD-L1 and 4-1BB, in advanced solid tumors. J Clin Oncol. (2024) 42:2529–9. doi: 10.1200/JCO.2024.42.16_suppl.2529
23. Dunn Z, Qu Y, MacMullan M, Chen X, Cinay G. 111 Armored CAR T cells secreting 4–1BB ligand crosslinked to PD-1 checkpoint inhibitor for enhanced solid tumor efficacy. J ImmunoTherapy Cancer. (2021) 9:A121–1. doi: 10.1136/jitc-2021-SITC2021.111
24. Park J, Berz D, Sharma M, Massarelli E, Hauke R, Tsai F, et al. 742 Phase 1/2 study of the bispecific 4–1BB and PD-L1 antibody INBRX-105 alone and in combination with pembrolizumab in select solid tumors. (2023) 11:. doi: 10.1136/jitc-2023-SITC2023.0742
25. Woroniecka KI, Rhodin KE, Dechant C, Cui X, Chongsathidkiet P, Wilkinson D, et al. 4-1BB agonism averts TIL exhaustion and licenses PD-1 blockade in glioblastoma and other intracranial cancers. Clin Cancer Res. (2020) 26:1349–58. doi: 10.1158/1078-0432.CCR-19-1068
26. Linch SN, McNamara MJ, Redmond WL. OX40 agonists and combination immunotherapy: putting the pedal to the metal. Front Oncol. (2015) 5. doi: 10.3389/fonc.2015.00034
27. Li Y, Zhang Y, Qiu X, Feng D, Sun R, He DX. Abstract 3739: Different combination regimens for OX40 agonists and PD-1 inhibitors exert different antitumor effects in OX40 humanized mice. Cancer Res. (2024) 84:3739–9. doi: 10.1158/1538-7445.AM2024-3739
28. Redmond WL. Challenges and opportunities in the development of combination immunotherapy with OX40 agonists. Expert Opin Biol Ther. (2023) 23:901–12. doi: 10.1080/14712598.2023.2249396
29. Andersson H, Nyesiga B, Hermodsson T, Enell Smith K, Hägerbrand K, Lindstedt M, et al. Next-generation CD40 agonists for cancer immunotherapy. Expert Opin Biol Ther. (2024) 24:351–63. doi: 10.1080/14712598.2024.2357714
30. Wiehagen KR, Girgis NM, Yamada DH, Smith AA, Chan SR, Grewal IS, et al. Combination of CD40 agonism and CSF-1R blockade reconditions tumor-associated macrophages and drives potent antitumor immunity. Cancer Immunol Res. (2017) 5:1109–21. doi: 10.1158/2326-6066.CIR-17-0258
31. Bentebibel S-E, Johnson D, Amariae R, McGrail D, Lecagoonporn S, Haymaker C, et al. Abstract CT039: Intratumoral CD40 agonist sotigalimab with pembrolizumab induces broad innate and adaptive immune activation in local and distant tumors in metastatic melanoma. Cancer Res. (2022) 82:CT039–9. doi: 10.1158/1538-7445.AM2022-CT039
32. Ababneh O, Nishizaki D, Kato S, Kurzrock R. Tumor necrosis factor superfamily signaling: life and death in cancer. Cancer Metastasis Rev. (2024) 43:1137–63. doi: 10.1007/s10555-024-10206-6
33. Yun S, Peter B. TNF superfamily protein-protein interactions: feasibility of small- molecule modulation. Curr Drug Targets. (2015) 16:393–408. doi: 10.2174/1389450116666150223115628
34. Dutton R, Hauser C, Boffard K, Dimsits J, Bernard G, Holcomb J, et al. Scientific and logistical challenges in designing the CONTROL trial: Recombinant factor VIIa in severe trauma patients with refractory bleeding. Clin trials (London England). (2009) 6:467–79. doi: 10.1177/1740774509344102
35. Zhang K, Kong X, Li Y, Wang Z, Zhang L, Xuan L. PD-1/PD-L1 inhibitors in patients with preexisting autoimmune diseases. Front Pharmacol. (2022) 13:854967. doi: 10.3389/fphar.2022.854967
36. Zamani MR, Aslani S, Salmaninejad A, Javan MR, Rezaei N. PD-1/PD-L and autoimmunity: A growing relationship. Cell Immunol. (2016) 310:27–41. doi: 10.1016/j.cellimm.2016.09.009
37. Šedý J, Bekiaris V, Ware C. Tumor necrosis factor superfamily in innate immunity and inflammation. Cold Spring Harbor Perspect Biol. (2014) 7.
38. Croft M. The role of TNF superfamily members in T-cell function and diseases. Nat Rev Immunol. (2009) 9:271–85. doi: 10.1038/nri2526
39. Croft M. The TNF family in T cell differentiation and function – Unanswered questions and future directions. Semin Immunol. (2014) 26:183–90. doi: 10.1016/j.smim.2014.02.005
40. Baker T, Sharifian H, Newcombe PJ, Gavin PG, Lazarus MN, Ramaswamy M, et al. Type I interferon blockade with anifrolumab in patients with systemic lupus erythematosus modulates key immunopathological pathways in a gene expression and proteomic analysis of two phase 3 trials. Ann Rheumatic Dis. (2024) 83:1018–27. doi: 10.1136/ard-2023-225445
41. Bruce IN, Furie RA, Morand EF, Manzi S, Tanaka Y, Kalunian KC, et al. Concordance and discordance in SLE clinical trial outcome measures: analysis of three anifrolumab phase 2/3 trials. Ann Rheumatic Dis. (2022) 81:962–9. doi: 10.1136/annrheumdis-2021-221847
42. Tison A, Garaud S, Chiche L, Cornec D, Kostine M. Immune-checkpoint inhibitor use in patients with cancer and pre-existing autoimmune diseases. Nat Rev Rheumatol. (2022) 18:641–56. doi: 10.1038/s41584-022-00841-0
43. Tsumoto K, Takeuchi T. Next-generation anti-TNFα Agents: the example of ozoralizumab. BioDrugs. (2024) 38:341–51. doi: 10.1007/s40259-024-00648-3
44. Li B, Gong S, Zhang N, Shi B, Lv Z, Zhang Y, et al. A novel designed anti–PD-L1/OX40 bispecific antibody augments both peripheral and tumor-associated immune responses for boosting antitumor immunity. Mol Cancer Ther. (2025) 2024:OF1–OF14. doi: 10.1158/1535-7163.MCT-24-0330
45. Kuang Z, Pu P, Wu M, Wu Z, Wang L, Li Y, et al. A novel bispecific antibody with PD-L1–assisted OX40 activation for cancer treatment. Mol Cancer Ther. (2020) 19:2564–74. doi: 10.1158/1535-7163.MCT-20-0226
46. Garg P, Krishna M, Subbalakshmi AR, Ramisetty S, Mohanty A, Kulkarni P, et al. Emerging biomarkers and molecular targets for precision medicine in cervical cancer. Biochim Biophys Acta (BBA) - Rev Cancer. (2024) 1879:189106. doi: 10.1016/j.bbcan.2024.189106
48. Huang W, Percie du Sert N, Vollert J, Rice ASC. General principles of preclinical study design. In: Bespalov A, Michel MC, Steckler T, editors. Good research practice in non-clinical pharmacology and biomedicine. Springer International Publishing, Cham (2020). p. 55–69.
Keywords: immune checkpoints, TNFSF agonists, cancer therapy, autoimmune diseases, immune response, biomarkers
Citation: Sun L, Li C, Gao T, Liu Z, Hou Y and Han W (2025) Combining immune checkpoints with TNFSF agonists: a new horizon for cancer and autoimmune therapies. Front. Immunol. 16:1557176. doi: 10.3389/fimmu.2025.1557176
Received: 08 January 2025; Accepted: 03 March 2025;
Published: 17 March 2025.
Edited by:
Ming Yu Lien, China Medical University Hospital, TaiwanReviewed by:
Marcio Chaim Bajgelman, National Center for Research in Energy and Materials, BrazilCopyright © 2025 Sun, Li, Gao, Liu, Hou and Han. This is an open-access article distributed under the terms of the Creative Commons Attribution License (CC BY). The use, distribution or reproduction in other forums is permitted, provided the original author(s) and the copyright owner(s) are credited and that the original publication in this journal is cited, in accordance with accepted academic practice. No use, distribution or reproduction is permitted which does not comply with these terms.
*Correspondence: Yanli Hou, MTQ2NDE4MzgwOEBxcS5jb20=; Wei Han, aHd4eWsxOTg5QDE2My5jb20=
Disclaimer: All claims expressed in this article are solely those of the authors and do not necessarily represent those of their affiliated organizations, or those of the publisher, the editors and the reviewers. Any product that may be evaluated in this article or claim that may be made by its manufacturer is not guaranteed or endorsed by the publisher.
Research integrity at Frontiers
Learn more about the work of our research integrity team to safeguard the quality of each article we publish.