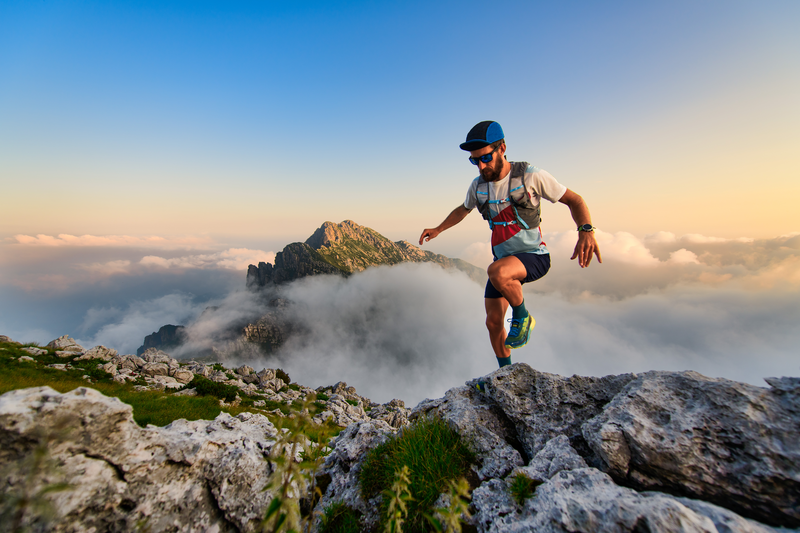
94% of researchers rate our articles as excellent or good
Learn more about the work of our research integrity team to safeguard the quality of each article we publish.
Find out more
REVIEW article
Front. Immunol.
Sec. Microbial Immunology
Volume 16 - 2025 | doi: 10.3389/fimmu.2025.1551289
The final, formatted version of the article will be published soon.
You have multiple emails registered with Frontiers:
Please enter your email address:
If you already have an account, please login
You don't have a Frontiers account ? You can register here
Inflammatory bowel disease (IBD) is a chronic recurrent gastrointestinal disease that seriously affects the quality of life of patients around the world. It is characterized by recurrent abdominal pain, diarrhea, and mucous bloody stools. There is an urgent need for more accurate diagnosis and effective treatment of IBD. Accumulated evidence suggests that gut microbiota plays an important role in the occurrence and development of gut inflammation. However, most studies on the role of gut microbiota in IBD have focused on bacteria, while fungal microorganisms have been neglected. Fungal dysbiosis can activate the host protective immune pathway related to the integrity of the epithelial barrier and release a variety of pro-inflammatory cytokines to trigger the inflammatory response. Dectin-1, CARD9, and IL-17 signaling pathways may be immune drivers of fungal dysbacteriosis in the development of IBD. In addition, fungal-bacterial interactions and fungal-derived metabolites also play an important role. Based on this information, we explored new strategies for IBD treatment targeting the intestinal fungal group and its metabolites, such as fungal probiotics, antifungal drugs, diet therapy, and fecal microbiota transplantation (FMT). This review aims to summarize the fungal dysbiosis and pathogenesis of IBD, and provide new insights and directions for further research in this emerging field.
Keywords: inflammatory bowel disease, Microbiology, Fungi, Pathogenesis, Treatment
Received: 25 Dec 2024; Accepted: 11 Mar 2025.
Copyright: © 2025 Chen, Yi, Yi, Zhou, Song and Zeng. This is an open-access article distributed under the terms of the Creative Commons Attribution License (CC BY). The use, distribution or reproduction in other forums is permitted, provided the original author(s) or licensor are credited and that the original publication in this journal is cited, in accordance with accepted academic practice. No use, distribution or reproduction is permitted which does not comply with these terms.
* Correspondence:
Houpan Song, Hunan University of Chinese Medicine, Changsha, China
Meiyan Zeng, Hunan University of Chinese Medicine, Changsha, China
Disclaimer: All claims expressed in this article are solely those of the authors and do not necessarily represent those of their affiliated organizations, or those of the publisher, the editors and the reviewers. Any product that may be evaluated in this article or claim that may be made by its manufacturer is not guaranteed or endorsed by the publisher.
Research integrity at Frontiers
Learn more about the work of our research integrity team to safeguard the quality of each article we publish.