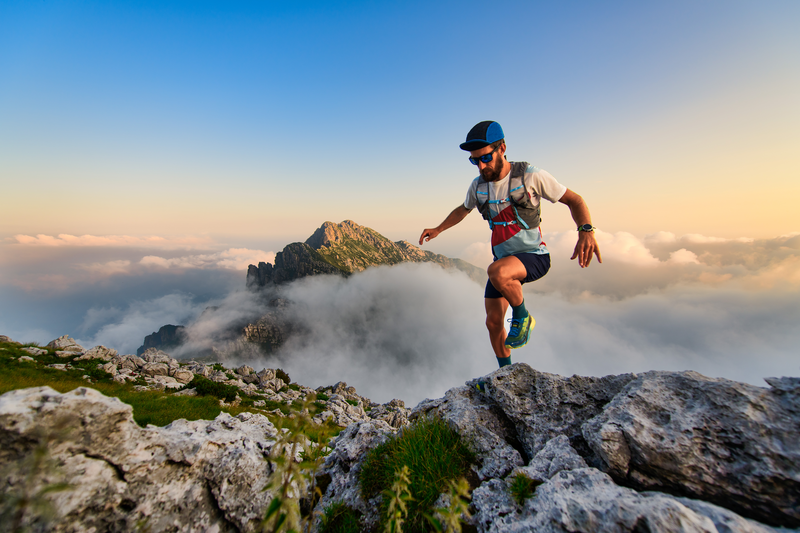
94% of researchers rate our articles as excellent or good
Learn more about the work of our research integrity team to safeguard the quality of each article we publish.
Find out more
HYPOTHESIS AND THEORY article
Front. Immunol.
Sec. Immunological Tolerance and Regulation
Volume 16 - 2025 | doi: 10.3389/fimmu.2025.1549739
This article is part of the Research Topic Community Series in Autoantibodies: Volume II View all 10 articles
The final, formatted version of the article will be published soon.
You have multiple emails registered with Frontiers:
Please enter your email address:
If you already have an account, please login
You don't have a Frontiers account ? You can register here
Although some reports suggest that COVID-19 vaccination may exacerbate existing autoimmune diseases or trigger new-onset cases, a definitive causal relationship between the vaccines and these conditions has not been established. Several potential mechanisms have been proposed to explain this association, including: (i) molecular mimicry, which refers to a structural similarity between SARS-CoV-2 and human antigens; (ii) bystander activation, involving both B and T lymphocytes; and (iii) the effects of adjuvants. In this study, we investigated whether two doses of the mRNA COVID-19 vaccine influenced blood cytokine levels associated with major T helper cell populations, which are known to play a significant role in autoimmunity and revisited the role of the humoral autoimmune response directed against heat shock proteins (Hsps) in individuals with no history of COVID-19. While no significant differences were found in the levels of IFN-γ, IL-6, IL-22, IL-4, IL-8, IL-10, and IL-17A, between vaccinated and unvaccinated people, several positive correlations were observed between serum cytokine levels and circulating autoantibodies directed against self-Hsps exclusively in vaccinated individuals. These findings suggest that the mRNA COVID-19 vaccine does not impact cytokines involved in the pathogenesis of autoimmune diseases.Further research is required to evaluate the safety of COVID-19 vaccination in patients with autoimmune conditions, particularly those in whom anti-Hsps autoantibodies are suspected to contribute to disease development.
Keywords: Cytokines, T helper cells, COVID-19, Heat shock proteins, SARS-CoV-2, Vaccine
Received: 21 Dec 2024; Accepted: 28 Feb 2025.
Copyright: © 2025 Tukaj, Sitna and Sitko. This is an open-access article distributed under the terms of the Creative Commons Attribution License (CC BY). The use, distribution or reproduction in other forums is permitted, provided the original author(s) or licensor are credited and that the original publication in this journal is cited, in accordance with accepted academic practice. No use, distribution or reproduction is permitted which does not comply with these terms.
* Correspondence:
Stefan Tukaj, University of Gdansk, Gdansk, Poland
Disclaimer: All claims expressed in this article are solely those of the authors and do not necessarily represent those of their affiliated organizations, or those of the publisher, the editors and the reviewers. Any product that may be evaluated in this article or claim that may be made by its manufacturer is not guaranteed or endorsed by the publisher.
Research integrity at Frontiers
Learn more about the work of our research integrity team to safeguard the quality of each article we publish.