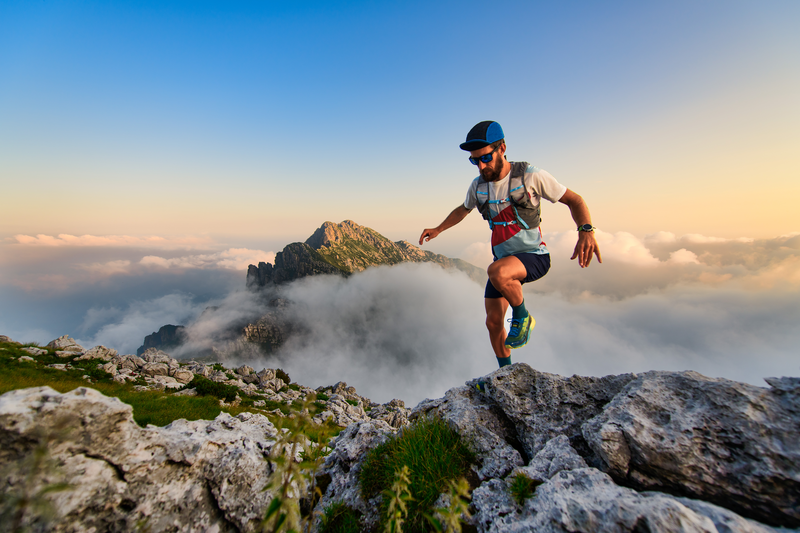
94% of researchers rate our articles as excellent or good
Learn more about the work of our research integrity team to safeguard the quality of each article we publish.
Find out more
REVIEW article
Front. Immunol.
Sec. Alloimmunity and Transplantation
Volume 16 - 2025 | doi: 10.3389/fimmu.2025.1548735
This article is part of the Research Topic Multi-omics Assessment for the Discovery of Promising Novel Molecules in the Treatment of Transplant Organ Injury View all articles
The final, formatted version of the article will be published soon.
You have multiple emails registered with Frontiers:
Please enter your email address:
If you already have an account, please login
You don't have a Frontiers account ? You can register here
Given the inevitable hypoxia and reperfusion injury that occur in organs donated after circulatory death (DCD), the quality and function of these organs are significantly compromised, greatly limiting their application in clinical organ transplantation. Recently, the advancement of functional omics technologies has enabled us to deeply analyze the mechanisms underlying DCD donor organ damage from multiple perspectives. This review systematically integrates the studies from transcriptomics, proteomics, and metabolomics to reveal the key biological mechanisms associated with the declines in DCD donor organ quality, including oxidative stress, inflammatory responses, cell death pathways, and metabolic disturbances. Additionally, we summarized emerging therapeutic strategies based on findings from omics perspectives, offering new possibilities to improve the quality of DCD organ for better transplant prognosis. Finally, we discussed the challenges in current research and future directions to provide scientific evidence for clinical practice and promote the application of DCD donors in organ transplantation.
Keywords: Donation after circulatory death, Liver Transplantation, multi-omics, ischemia-reperfusion injury, Oxidative Stress, Inflammatory Response
Received: 20 Dec 2024; Accepted: 03 Mar 2025.
Copyright: © 2025 Zhou, Lu and Liu. This is an open-access article distributed under the terms of the Creative Commons Attribution License (CC BY). The use, distribution or reproduction in other forums is permitted, provided the original author(s) or licensor are credited and that the original publication in this journal is cited, in accordance with accepted academic practice. No use, distribution or reproduction is permitted which does not comply with these terms.
* Correspondence:
Zhengtao Liu, Zhejiang Shuren University, Hangzhou, China
Disclaimer: All claims expressed in this article are solely those of the authors and do not necessarily represent those of their affiliated organizations, or those of the publisher, the editors and the reviewers. Any product that may be evaluated in this article or claim that may be made by its manufacturer is not guaranteed or endorsed by the publisher.
Research integrity at Frontiers
Learn more about the work of our research integrity team to safeguard the quality of each article we publish.