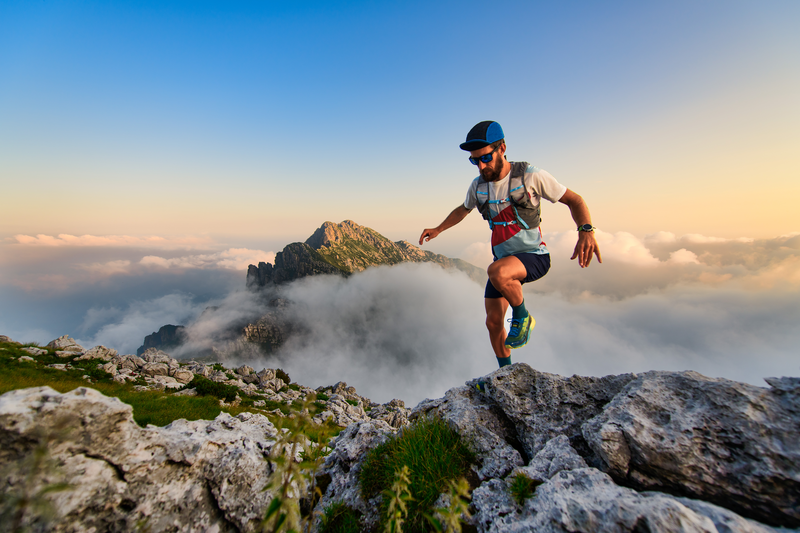
95% of researchers rate our articles as excellent or good
Learn more about the work of our research integrity team to safeguard the quality of each article we publish.
Find out more
REVIEW article
Front. Immunol. , 01 April 2025
Sec. Cancer Immunity and Immunotherapy
Volume 16 - 2025 | https://doi.org/10.3389/fimmu.2025.1547377
This article is part of the Research Topic Precision Immunotherapy and Novel Target Discovery in Hematological Malignancy View all 11 articles
Primary large B-cell lymphomas of immune-privileged sites (IP-LBCLs) include primary central nervous system large B-cell lymphoma (PCNSL), primary vitreoretinal large B-cell lymphoma (PVRL), and primary testicular large B-cell lymphoma (PTL). These tumors not only have a unique anatomical distribution but also exhibit specific biological and clinical characteristics. Given the high biological overlap between intravascular large B-cell lymphoma (IVLBCL) and IP-LBCLs, and the fact that IVLBCL is confined to the intravascular microenvironment, IVLBCL is currently included in the category of IP-LBCLs. IP-LBCLs are associated with suboptimal prognosis. However, advancements in biomarker detection technologies have facilitated novel therapeutic approaches for this disease entity. This review aims to summarize and analyze the latest research progress in IP-LBCLs, with a focus on new treatment strategies in the era of targeted therapy and immunotherapy. It is intended to further understand the biological characteristics, treatment, and latest advancements of this disease.
Primary large B-cell lymphomas of immune-privileged sites (IP-LBCLs) are classified as an independent entity in the 5th edition of the World Health Organization (WHO) classification of lymphoid neoplasms (1). These lymphomas occur specifically in immunocompetent individuals, within specific anatomic sites behind immune barriers such as the blood-brain barrier (BBB), blood-retinal barrier, and blood-testicular barrier, as well as within the immunoregulatory systems that form immune sanctuaries in these primary sites (2). IP-LBCLs include primary central nervous system large B-cell lymphoma (PCNSL), primary vitreoretinal large B-cell lymphoma (PVRL), and primary testicular large B-cell lymphoma (PTL) (1, 2). Lymphomas arising at other sites, such as intravascular large B-cell lymphoma (IVLBCL), also appear to share similar characteristics (2). In IP-LBCLs, PCNSL is the most common, accounting for 4-6% of all extranodal lymphomas (3, 4). The clinical presentation is most commonly characterized by focal neurologic deficits, such as fatigue, sensory changes, and aphasia (4). Compared with other extranodal large B-cell lymphomas, PCNSL has a poorer prognosis with a 5-year overall survival (OS) of only 30% (5–7). Despite the fact that high-dose methotrexate (HD-MTX)-based combination chemotherapy is the first-line treatment for PCNSL, there is a recurrence rate of up to 50% in patients (8–10). Recurrent or refractory PCNSL (R/R PCNSL) has an extremely poor prognosis, with no standard treatment protocol. PVRL is a rare subtype of PCNSL that originates in the vitreoretinal area without CNS involvement (11). The clinical presentation is mostly localized ocular symptoms, such as blurred vision, decreased visual acuity, and floaters. Unlike PCNSL, PVRL can be treated locally, such as by intraocular injection of chemotherapy drugs or ocular radiotherapy (8, 12–15). PTL accounts for 1-2% of non-Hodgkin’s lymphomas (NHL) and is the most common malignant testicular tumor in men over 60 (16–18). PTL is usually confined to the testicle but can also spread throughout the body, such as to the CNS, skin, lung, soft tissue, and contralateral testicle. The majority of patients present with painless testicular enlargement, and up to 40% of cases also have scrotal hydrocele. Surgery is the first-line treatment for suspected PTL. IVLBCL is a rare subtype of NHL characterized by a large number of tumor cells aggregating in small or medium-sized vascular lumens, particularly capillaries and postcapillary venules. It is prone to invasion of the CNS but rarely involves lymph nodes (19). IVLBCL is divided into three subtypes, namely the classic subtype, skin subtype, and hemophagocytic-related subtype, with different clinical presentations among the subtypes. However, the overall prognosis is extremely poor, with a median survival of approximately 1 year (20)(Table 1).
IP-LBCLs are associated with a poor prognosis and currently lack standardized treatment regimens. Molecular characterization reveals distinct heterogeneity in IP-LBCLs, primarily manifesting through the following features: High-frequency genetic mutations (2): MYD88 mutations drive constitutive activation of the NF-κB signaling pathway, promoting tumor cell proliferation and survival. CD79B mutations amplify BCR signaling, synergizing with MYD88 mutations to enhance NF-κB pathway activation. Genomic instability (21): Frequent 9p24.1/PD-L1/PD-L2 copy number alterations. Increased PD-L1/PD-L2 copy numbers may underlie immune evasion mechanisms in IP-LBCLs. These molecular hallmarks have catalyzed the development of novel targeted therapies and immunotherapeutic strategies, offering promising alternatives for IP-LBCL management (Figure 1).
Figure 1. Therapeutic Advances in IP-LBCLs: Targeting and Immunotherapy in the Modern Era. (IP-LBCLs: primary large B-cell lymphomas of immune-privileged sites; PCNSL, primary central nervous system lymphoma; PVRL, primary vitreoretinal lymphoma; PTL, primary testicular lymphoma; IVLBCL, intravascular large B-cell lymphoma; R-MBVP, rituximab/methotrexate/carmustine/teniposide/prednisone; R-MA, rituximab/methotrexate/cytarabine; MARix, methotrexate/cytarabine/rituximab/thioepa; R-CHOP, rituximab/cyclophosphamide/doxorubicin/vincristine/prednisone; MIT, methotrexate/ibrutinib/temozolomide; ZA, zanubrutinib/cytarabine; ZR-MTX, zanubrutinib/rituximab/methotrexate; CR-MTX, chidamide/rituximab/methotrexate; R-MTO, methotrexate/orelabrutinib/thiotepa/rituximab; R2, lenalidomide/rituximab; POM, pomalidomide; SMTR, sintilimab/methotrexate/temozolomide/rituximab; CAR, chimeric antigen receptor).
In normal B cells, the Toll-like receptor (TLR) signaling pathway and the B-cell receptor (BCR) signaling pathway synergistically activate the NF-κB signaling pathway. However, when MYD88 and CD79B mutations occur, they abnormally activate the NF-κB signaling pathway, thereby promoting malignant proliferation of B cells, which is an important mechanism in the formation of B-cell lymphoma. IP-LBCLs exhibit similar phenotypes and molecular characteristics, with most belonging to the MCD subtype, harboring mutations in MYD88 and CD79B (2). Notably, more than 60% of IP-LBCLs patients have MYD88 mutations, with mutation rates reaching up to 88% in subtypes such as PVRL, 67% in PCNSL, between 68-82% in PTL, and about 60% in IVLBCL (11, 22–24). In systemic DLBCL, molecular subtyping based on next-generation sequencing has stratified the disease into four distinct genetic classifications: EZB (EZH2 mutations and BCL2 translocations), MCD (MYD88 and CD79B mutations), BN2 (BCL6 translocations and NOTCH2 mutations), and N1 (NOTCH1 mutations) subtypes. Notably, both MCD and N1 subtypes exhibit inferior prognosis, with the MCD cohort demonstrating a particularly dismal 5-year OS rate of 40% (25, 26). This molecular heterogeneity likely contributes to the poor clinical outcomes observed in IP-LBCLs. MYD88, TLR9, and BCR form the “My-T-BCR” supercomplex, which is the activation site of NF-κB. More significantly, this site is highly sensitive to BTKi (27, 28). Moreover, as a small molecule inhibitor, BTKi can effectively cross the BBB (29–31). Therefore, the application of BTKi in IP-LBCLs treatment has become a hot topic in current research.
In the phase I/II study of ibrutinib monotherapy in R/R PCNSL (32), an objective response rate (ORR) of up to 77% was observed regardless of BCR and MYD88 status. In a phase II study involving 46 patients with R/R CNSL (33), the ORR of monotherapy ibrutinib in PCNSL patients reached 74%. Moreover, in a multi-center, prospective phase II study (34) using the MIT regimen (HD-MTX + ibrutinib + temozolomide) for the initial treatment of PCNSL, a total of 33 patients were included. After receiving MIT induction therapy, they were given either sequential autologous stem cell transplantation (ASCT) consolidation treatment or ibrutinib maintenance treatment. The best ORR was 94%, with a median follow-up of 17.5 months, with a 1-year progression-free survival (PFS) and OS rate of 66.4% and 90.5%, respectively, and no severe adverse events (SAEs)-related deaths were observed during treatment. In another study from China (35), the ZR-MTX regimen (zanubrutinib+rituximab+HD-MTX) was used to treat newly diagnosed PCNSL in 15 patients, with an ORR of 66.7% and a complete response rate (CRR) of 40.0%. With a median follow-up of 20.2 months, the 2-year PFS and OS were 38.9% and 67.5%, respectively. Notably, no unexpected toxicities were observed throughout the treatment process. In a phase II prospective study by Bairey et al. (36), ibrutinib monotherapy was used as a maintenance treatment for newly diagnosed PCNSL after HD-MTX-based induction therapy. A total of 20 patients were included, and the median duration of ibrutinib maintenance was 12.5 months. The results showed a 2-year PFS and OS of 72.6% and 89%, respectively, with most AEs being of grade 1/2. In another ongoing single-center, single-arm, open-label study (37), 19 patients with newly diagnosed PCNSL were given 5-8 cycles of R-MTO (rituximab+HD-MTX+thiotepa+orelabrutinib) followed by sequential ASCT consolidation treatment. After 4 cycles of induction therapy, the CRR reached 89.47%, and the most common AE related to the R-MTO regimen were grade 1-2 neutropenia.
Tirabrutinib, as a second-generation BTKi, exhibits higher selectivity than ibrutinib. In a phase I/II study of tirabrutinib monotherapy for R/R PCNSL (38), a total of 44 patients with R/R PCNSL were enrolled, receiving single-agent tirabrutinib oral administration at different dosages. The ORR was 64%, with a median PFS of 2.9 months. Among these, 1 patient was observed to have a grade 5 AE. HZ-A-018 is a novel, highly selective covalent BTK inhibitor, which also possesses the ability to cross the BBB. The ongoing HZ-A-018-102 study is a phase I/II clinical trial (39), designed to assess the efficacy and safety of HZ-A-018 in CNS lymphoma. A total of 29 patients with R/R CNSL were enrolled, including 26 with PCNSL. As of the latest data analysis, with a median follow-up of 19 months, the ORR was 50%, and the median OS had not been reached. In terms of safety, only 5 patients experienced treatment-related AEs(TRAEs) of grade ≥3, and none of the patients had their dosages reduced or treatment discontinued due to TRAEs. Further exploratory studies have shown that the combination of HZ-A-018 with HD-MTX demonstrates preliminary efficacy in patients with R/R and newly diagnosed PCNSL, with an ORR of 55.6% among 9 R/R PCNSL patients (40). In 10 patients with newly diagnosed PCNSL, 2 have completed induction therapy and achieved a PR.
A prospective, single-arm, phase II study (41) conducted at Peking Union Medical College Hospital in China included 9 patients with IVLBCL, who received zanubrutinib combined with R-CHOP (rituximab + cyclophosphamide + doxorubicin + vincristine + prednisone) for 8 cycles, achieving an ORR of 100%. At a median follow-up of 10 months, there was no recurrence observed in any of the patients.
In summary, the application of BTKi in IP-LBCLs has undoubtedly been successful, regardless of whether it is used for newly diagnosed or relapsed/refractory patients, whether as induction or maintenance therapy, it has yielded extremely impressive results.
RTX is a human-mouse chimeric monoclonal antibody that primarily targets the CD20 antigen on the surface of B cells. Binding of this antibody to the CD20 antigen effectively blocks the normal function of B cells and rapidly triggers their depletion (42, 43). Upon binding to the CD20 antigen on the surface of B cells, RTX activates the complement system, leading to the lysis of B cells through complement-dependent cytotoxicity (CDC). Concurrently, macrophages and natural killer cells (NK cells) can bind to the Fc portion of RTX, mediating antibody-dependent cellular cytotoxicity (ADCC), which further clears CD20-positive B cells (44, 45).
Due to the large molecular weight of RTX, uncertainties persist regarding its ability to accumulate in the CNS at therapeutically effective concentrations required for anti-lymphoma activity. Pharmacokinetic studies demonstrate that intravenous RTX administration achieves cerebrospinal fluid concentrations <0.1% of serum levels, insufficient for sustained target engagement in CNS compartments (46). Notably, Lwamoto et al (47). demonstrated that radiation-induced BBB disruption via stereotactic radiosurgery significantly enhanced RTX penetration into the CNS parenchyma, thereby achieving therapeutic concentrations. These data highlight the critical dependency of RTX efficacy on BBB permeability modulation, contributing to ongoing controversies in its clinical utility for PCNSL management.
Several key clinical studies currently provide important evidence for clinical practice. Among them, the HOVON 105/ALLG study (48) is an open-label, randomized, controlled, multicenter phase III trial that enrolled 200 patients with normal immune function who had been newly diagnosed with PCNSL. These patients were randomly assigned to two groups in a 1:1 ratio, receiving either MBVP (HD-MTX+ carmustine + teniposide + prednisone) or R-MBVP induction therapy. With a median follow-up of 32.9 months, there were no significant differences between the two groups in terms of event-free survival (EFS) (HR=1.00, 95% CI: 0.70-1.43, P=0.99), PFS (HR=0.77, 95% CI: 0.52-1.13, P=0.18), and OS (HR=0.93, 95% CI: 0.59-1.44, P=0.74). Another study, IELSG32 (49, 50) is a multicenter, randomized, phase II trial that included a total of 227 patients with newly diagnosed PCNSL. These patients were randomly divided into three groups and received different induction chemotherapy regimens: (A) HD-MTX + cytarabine; (B) HD-MTX + cytarabine + RTX; (C) HD-MTX + cytarabine + RTX + thiotepa. With a median follow-up of 88 months, the results showed that the PFS of group B was slightly better than that of group A, although there was no significant statistical difference (P=0.06), but group B significantly prolonged the OS (P=0.04). In addition, a 1:1 propensity-matched study based on the National Cancer Database (51) found that compared with conventional chemotherapy, the combination of RTX immunotherapy significantly improved the OS of PCNSL patients (HR=0.75, 95% CI: 0.67-0.83). A meta-analysis (52) demonstrated improved PFS with RTX-containing regimens in PCNSL patients, while failing to establish significant OS benefit.
In the treatment of PTL, the immunotherapy regimen R-CHOP, has become the mainstream treatment option. Recent research data show that the 5-year OS rate for patients treated with the R-CHOP regimen is 86.6% (53). In addition, the prospective results from studies IELSG-10 and IELSG-30 further confirm the advantages of the R-CHOP regimen in the treatment of PTL (54, 55). These studies used the R-CHOP regimen as induction therapy and combined it with radiotherapy and intensive CNS prophylaxis. This comprehensive treatment approach can effectively reduce the risk of CNS recurrence, thereby further improving the overall efficacy of PTL treatment. In the treatment of IVLBCL, the PRIMEUR-IVL study is a significant multicenter, single-arm phase II clinical trial (56). This study included 37 patients with de novo IVLBCL who received immunotherapy based on RTX, combined with CNS prophylactic treatment. With a median follow-up of 3.9 years, the results showed that the 2-year PFS and OS rates were 76% and 92%, respectively. The latest 7-year follow-up data demonstrated 5-year PFS and OS rates of 68% and 78% respectively, with a cumulative CNS event incidence of 3% (57).
On the whole, despite the fact that there is no unified consensus on the application of RTX in PCNSL treatment, immunotherapy based on RTX has demonstrated significant efficacy in the treatment of PTL and IVLBCL, providing clinicians with an effective therapeutic approach.
As a new strategy in immune therapy, IMiDs have demonstrated significant anti-tumor potential. These drugs exert multiple effects such as regulating the tumor microenvironment, inhibiting angiogenesis, enhancing immune surveillance, modulating cell signaling pathways with immunological activity, and promoting tumor cell apoptosis (58). IMiDs, exemplified by lenalidomide (Len), exert antitumor effects through multifaceted molecular mechanisms. The central mechanism involves specific binding to the E3 ubiquitin ligase cereblon (CRBN), forming an E3 ubiquitin ligase complex that induces rapid ubiquitination and degradation of transcription factors Ikaros (IKZF1) and Aiolos (IKZF3). This process mediates therapeutic effects via several key pathways: Downregulation of BCR signaling through suppression of critical molecules (e.g., IRF4 and MYC), inhibiting tumor proliferation; Inhibition of NF-κB pathway activation, blocking pro-survival inflammatory cytokine effects in the tumor microenvironment; Upregulation of interferon signaling, enhancing tumor cell susceptibility to immune-mediated destruction. In addition, Len demonstrates synergistic efficacy when combined with RTX, primarily by augmenting ADCC through Fcγ receptor IIIa (CD16a) potentiation (59–61).
A multicenter, prospective phase II study (62) conducted by French Oculo-Cerebral lymphoma (LOC) Network and the Lymphoma Study Association (LYSA) aimed to evaluate the efficacy and safety of Len in combination with RTX (R2) for treating R/R PCNSL or PVRL. A total of 45 patients who were eligible for efficacy assessment were enrolled, with an ORR of 67% during the best induction period. At a median follow-up of 19.2 months, the median PFS and OS were 7.8 months and 17.7 months, respectively. Notably, no unexpected toxicities were observed throughout the treatment process. On the other hand, a multicenter, single-arm, open-label phase 2 study (63) by Yuan and colleagues explored the efficacy and safety of Len, RTX, and HD-MTX(R2-MTX) combinations for the initial treatment of PCNSL, followed by R2 maintenance therapy. The study included 17 patients, with the best ORR during induction treatment at 94.1%. At a median follow-up of 23 months, the projected 2-year PFS rate was 58.8% and the 3-year OS rate was 76.0%. All patients experienced TRAEs, but no patients discontinued therapy due to AEs.
Pomalidomide (POM) as a third-generation IMiD has a structure similar to Len but exhibits stronger anti-tumor activity with comparable safety (64, 65). Studies in rat models have shown that the cerebrospinal fluid (CSF) penetration rate of POM reaches 39% (66). In a phase I study of POM combined with dexamethasone treatment for R/R PCNSL/PVRL (67), 25 patients were enrolled, with an ORR of 48%. At a median follow-up of 16.5 months, the median PFS was 5.3 months, and the treatment was shown to be well tolerated.
In summary, the aforementioned research findings have revealed the potential of IMiDs in the treatment of IP-LBCLs, demonstrating good tolerance and high efficacy, thus providing clinicians with more treatment options.
ICIs primarily enhance the cytotoxic activity of host T cells by blocking inhibitory signals of T cell activation, rather than directly targeting tumor cells. They work by stimulating the host immune system to exert anti-tumor effects (68). The application of these inhibitors in lymphoma primarily targets two key immune checkpoint proteins: programmed cell death protein 1 (PD-1) and programmed death ligand 1 (PD-L1). PD-1 is predominantly expressed on the surface of T cells, while PD-L1 is often expressed on the surface of tumor cells. Upon binding of PD-1 to PD-L1, the anti-tumor activity of T cells is suppressed. Therefore, PD-1/PD-L1 inhibitors block the interaction between PD-1 and PD-L1, thereby restoring the recognition and attack capabilities of T cells against tumor cell (69). Existing research indicates that increased PD-L1/PD-L2 gene copies or translocations are relatively common in patients with PCNSL and PTL (21, 23, 70). Additionally, there are case reports suggesting that the immune escape mechanism in IVLBCL may be associated with PD-L1 (71). However, some researchers point out that the immune escape mechanism in PTL is mainly related to the loss of HLA, rather than changes in PD-L1/PD-L2 (72).
Recent findings reported by researchers Nayak and colleagues have important implications (73). They observed 5 patients with R/R IP-LBCLs, including 4 with R/R PCNSL and 1 with CNS recurrence of PTL. All patients received nivolumab treatment, with the best ORR reaching 100% during the induction phase, and all patients had PFS exceeding 13 months. Moreover, researchers Terziev and colleagues (74) reported a case of a PCNSL patient who experienced a second recurrence and received three ASCT. Following the third ASCT, the patient received sequential nivolumab maintenance therapy for 1 year, achieving CR and maintaining it for over 24 months. In a phase II study (75) led by Zeng et al., the combination of sintilimab with HD-MTX, temozolomide, and RTX (SMTR) was used for induction therapy in patients with newly diagnosed PCNSL. This regimen demonstrated an extremely high ORR, reaching 96.3%, with a CRR of 92.6%. At a median follow-up of 24.4 months, the 2-year PFS and OS rates were 57.2% and 91.5%, respectively. The most common grade 3 AE was increased alanine aminotransferase levels (17.9%), but these patients with elevated transaminase levels could be managed reversibly with liver protection treatments. None of the patients reduced or ceased treatment due to drug toxicity.
These groundbreaking findings undoubtedly illuminate novel therapeutic avenues and renewed hope for patients with IP-LBCLs. ICIs such as nivolumab and sintilimab have demonstrated substantial clinical efficacy and favorable safety profiles in IP-LBCLs, representing a paradigm shift in treatment algorithms. However, emerging evidence necessitates cautious optimism, as ICIs may precipitate immune-related AEs (irAEs) including pneumonitis, hepatitis, colitis, endocrine dysregulation (e.g., hypothyroidism), and neurotoxicity (e.g., encephalitis) (76). A seminal retrospective analysis by Fonseca et al. (77, 78) of 64 patients developing neurological irAEs post-ICIs therapy revealed alarming outcomes: mortality rates reaching 10-29%, with 40-50% of survivors acquiring persistent severe disability. These findings are corroborated by Farina et al. (79), who documented particularly dismal prognoses for ICI-induced focal encephalitis. While current studies of ICIs in PCNSL cohorts report no CNS-specific toxicity signals, clinicians must maintain heightened vigilance.
PI3K is an intracellular lipid phosphatidylinositol kinase that converts phosphatidylinositol 4,5-bisphosphate (PIP2) to phosphatidylinositol 3,4,5-trisphosphate (PIP3) by specifically catalyzing the phosphorylation of the hydroxyl at the third position of phosphatidylinositol. The production of PIP3 facilitates the recruitment and activation of AKT (80). Post-activation, AKT acts as a signaling molecule, further activating downstream signaling pathways and initiating a cascade of signaling reactions. The PI3K/AKT/mTOR signaling pathway plays a critical role in the regulation of cell growth and proliferation by integrating signals from growth factors, hormones, nutrients, and energy metabolism, thereby regulating protein synthesis and influencing cell survival and proliferation (80, 81). Recent studies have shown that there is abnormal activation of the PI3K/AKT/mTOR signaling pathway in PCNSL patients, and this phenomenon is closely associated with poor prognosis (82). Therefore, exploring targeted drugs against the PI3K/AKT/mTOR signaling pathway, as well as integrating these drugs with other therapeutic strategies, is of great significance for improving the prognosis of IP-LBCL patients.
A multicentric phase II clinical trial, which is part of targeted therapies, investigated the use of the mTOR inhibitor temsirolimus in the treatment of R/R PCNSL (83). The study included 37 patients, with results showing an optimal ORR of 54%, a median PFS of 2.1 months, and an OS of 3.7 months. 56.8% of patients experienced grade ≥3 AEs. TRAEs led to death in 5 patients (13%), including 2 cases of pneumonia, and 1 case each of gastrointestinal infection with sepsis, sepsis, and cerebral hemorrhage. Additionally, 5 patients succumbed to disease progression. In another prospective study (84), the efficacy of the PI3K inhibitor Buparlisib (BKM120) as a monotherapy for R/R CNSL was evaluated. Among the four enrolled patients, the ORR was only 25%, with a median PFS and OS of 39 days and 196 days, respectively. One patient had to discontinue treatment due to psychiatric symptoms. In light of the low efficacy of this study, the trial was suspended. However, in a preclinical study (85), researchers established an orthotopic CNSL model by intracranially injecting A20-Luciferase-GFP cells into SCID mice. The therapeutic efficacy of BEBT-908, a novel PI3K/HDAC dual inhibitor, was subsequently evaluated through intravenous administration. The experiment demonstrated that BEBT-908 exhibited excellent BBB permeability in the intracranial lymphoma model and effectively inhibited tumor growth, extending the survival of host mice. Mechanistically, BEBT-908 downregulated C-MYC expression, induced iron death, and ultimately led to tumor shrinkage. Based on these positive findings, we anticipate that BEBT-908 may serve as a therapeutic option for IP-LBCLs in the future.
Current applications of PI3K/mTOR inhibitors in IP-LBCLs have not demonstrated long-term PFS or OS benefits, while long-term administration is associated with severe TRAEs. However, their potential in combination therapies remains promising for enhancing therapeutic efficacy and safety profiles.
Histone acetyltransferases (HATs) and HDACs dynamically regulate the epigenetic landscape by modulating acetylation post-translational modifications (PTMs) of histones and non-histone substrates. HATs catalyze the transfer of acetyl groups to specific lysine residues on histones and functional proteins (e.g., transcription factors, metabolic enzymes), whereas HDACs remove these acetyl marks. The equilibrium between these opposing activities governs chromatin architecture and transcriptional fidelity. Dysregulation of this balance disrupts cell cycle control, metabolic reprogramming, and epigenetic plasticity, ultimately driving oncogenesis (86, 87).
HDAC inhibitors such as chidamide restore HAT/HDAC-mediated acetylation homeostasis by selectively inhibiting HDAC activity. This reactivates tumor suppressor genes, suppresses pro-survival signaling pathways, and remodels the tumor immune microenvironment (88, 89). Chidamide has demonstrated marked efficacy in hematologic malignancies, particularly in combination therapies. Recent studies highlight its ability to improve outcomes in systemic DLBCL with high-risk molecular features (90–92).
Moreover, chidamide’s unique BBB penetrative capacity positions it as a breakthrough candidate for PCNSL (93). A phase 2 trial by Chen et al. evaluated the CR-MTX regimen (chidamide + RTX + HD-MTX) in newly diagnosed PCNSL (94). Among 9 evaluable patients, the ORR post-induction reached 89% (CRR 78%), with median PFS of 13 months. No grade ≥3 TRAEs were observed. These findings underscore the promise of epigenetic-based combinatorial strategies for IP-LBCLs.
XPO1 is a primary nuclear export protein in cells, primarily responsible for the export of molecules such as proteins and various types of RNA from the nucleus to the cytoplasm (95). Nonetheless, overexpression of XPO1 is a common phenomenon in multiple malignant tumors, leading to uneven distribution of regulatory proteins within the cell and uncontrolled proliferation of tumor cells, thus facilitating tumor progression. Consequently, targeted therapeutic strategies against XPO1 have emerged as a new direction in the field of tumor therapy. Selinexor, as a small molecule inhibitor, can selectively inhibit the activity of XPO1, thus blocking its mediating nuclear export process. This inhibition leads to the accumulation of tumor suppressor proteins and cell cycle regulatory factors within the nucleus, which ultimately induces cell cycle arrest and demonstrates specific antitumor activity (96). In June 2020, the FDA approved selinexor for the treatment of R/R DLBCL, marking a significant advancement in its clinical application (97). It is noteworthy that selinexor possesses the ability to penetrate the BBB. Clinical case reports (98) indicate that selinexor has significant efficacy in inhibiting CNS involvement in R/R DLBCL. This provides strong evidence supporting the use of selinexor as a novel targeted therapeutic agent for IP-LBCLs.
The BCL-2 family plays a crucial role in regulating the intrinsic apoptosis pathway. The BCL-2 protein belongs to a group of anti-apoptosis proteins, and its abnormal expression is closely associated with the occurrence and development of tumors (99). Therefore, specific inhibitors targeting the BCL-2 protein have become one of the key strategies for promoting apoptosis and achieving anti-tumor effects. A recent retrospective study using immunohistochemical technology has found that approximately 60% of patients with PCNSL have overexpression of BCL-2 (100). This finding provides strong clinical evidence for targeted therapy against BCL-2. In SICD mouse models, BCL2 inhibitors (venetoclax or navitoclax) in combination with immunochemotherapy regimens have shown significant synergistic effects, to some extent inhibiting the occurrence of CNSL (101). Although no clinical reports of BCL-2 inhibitors in IP-LBCLs exist to date, a phase Ib clinical trial (102) presented at the 2024 American Society of Hematology (ASH) Annual Meeting investigated the BCL-2 inhibitor venetoclax combined with Pola-R-CHP in treatment-naïve DLBCL patients with high BCL-2 expression (immunohistochemical positivity ≥50%). The study enrolled 50 patients, achieving a CRR exceeding 80%. With a median follow-up of 15.5 months, the 1-year PFS and OS rates were 75.5% and 91.7%, respectively.
These research findings provide important experimental evidence for the clinical application of BCL-2 inhibitors and also offer new options for clinicians treating IP-LBCLs.
IRAK4, a pivotal kinase in the TLR/MYD88-dependent signaling pathway, is critically implicated in the pathogenesis and progression of various hematologic malignancies, including DLBCL and acute myeloid leukemia (103, 104). When MYD88 mutations occur, the sustained activation of IRAK4 can lead to abnormal activation of the NF-κB signaling pathway, thereby promoting cell proliferation (105). Emavusertib (CA-4948) acts as a selective IRAK4 inhibitor and has garnered extensive attention due to its unique mechanism of action and favorable pharmacological properties. Particularly, when used in combination with ibrutinib, emavusertib can significantly increase the number of cells undergoing apoptosis, displaying a potential synergistic antitumor effect (106). Additionally, one notable advantage of emavusertib is its ability to effectively cross the BBB, which is of great significance for the treatment of hematological diseases involving the CNS (107). In a study evaluating the efficacy and safety of emavusertib in combination with ibrutinib for R/R PCNSL (108), an ORR of 50% was observed in 12 assessable patients. At the same time, there have been no reports of fatal TRAEs, and most AEs of ≥ grade 3 are reversible and manageable, indicating good safety characteristics. In summary, emavusertib holds promising prospects for the treatment of IP-LBCLs, and its precise targeting of IRAK4 provides a new therapeutic approach for hematological diseases.
Glofitamab, as an innovative 2:1 structured IgG1-type fully humanized bispecific antibody, has demonstrated significant anti-tumor potential. The antibody possesses two CD20 domains and one CD3 domain, with a unique 2:1 structure that not only enhances the targeting of lymphomas expressing CD20 positivity but also effectively exerts an anti-tumor effect by guiding CD3 effector T cells to the surface or surrounding area of tumor cells (109). In the pivotal NP30180 phase II study (110), glofitamab showed a promising therapeutic effect in 155 patients with R/R DLBCL. The study results indicate that glofitamab’s ORR was 52%, with a CRR of 39%. This achievement not only opens a new chapter in the treatment of R/R DLBCL with bispecific antibodies but also provides new insights and options for clinical treatment of other B-cell lymphomas.
Godfrey et al. conducted the first analysis of glofitamab in the treatment of R/R CNSL (111). In this study, a total of 4 patients were included, and CSF samples were collected from this subset of patients. The results showed that glofitamab was detected in the CSF of all 4 patients, with concentrations ranging from 0.00632 to 0.0296 μg/ml. Notably, 3 of the 4 patients experienced relief after treatment with glofitamab. During the treatment, only 2 patients developed a transient grade 1-2 cytokine release syndrome (CRS), indicating that glofitamab exhibits good efficacy and safety in the treatment of R/R CNSL.
CAR-T immunotherapy represents a revolutionary strategy in tumor treatment. This therapy involves genetically engineering a patient’s own T cells to enable them to specifically recognize and attack tumor cells. Currently, CAR-T cell therapy has demonstrated significant efficacy in the treatment of R/R DLBCL (112). Nevertheless, this therapy is also associated with some unique toxicities, including neurotoxicity. It is noteworthy that CAR-T cells can enter the CNS even in lymphoma patients without pre-existing CNS involvement, leading to immune effector cell associated neurotoxicity syndrome(ICANS) (113). For these reasons, due to concerns about the onset and progression of ICANS, research on the use of CAR-T therapy in PCNSL is mostly limited to small-scale retrospective and prospective studies.
The study by Mercadal and colleagues (114) is a clinical trial investigating the use of CD19 CAR-T cell therapy for R/R PCNSL. The study enrolled 24 patients with R/R PCNSL, of whom 23 were evaluable for response. By day 100, the ORR reached 61%. The median follow-up after CAR-T infusion was 26 months, with a 2-year PFS rate of 28% and an OS rate of 50%. A phase I/II clinical trial led by Frigault et al. (115) evaluated CD19 CAR-T cell therapy in 12 patients with R/R PCNSL, with a median follow-up of 12.2 months. The study demonstrated a best ORR of 58.3% and CRR of 50.0%, with median response duration of 3.1 months. Safety analysis revealed grade 1 CRS in 58.3% (7/12) and grade ≤2 ICANS in 41.6% (5/12), with only 1 case of grade 3 ICANS. No treatment-related mortality was observed.
Another retrospective study conducted within the French LOC network included 25 patients with R/R PCNSL who had received CAR-T treatment (116). This study reported a best ORR of 80%, with a median follow-up of 19.4 months after CAR-T cell infusion, leading to a median PFS of 8.4 months and a median OS of 21.2 months. Two patients (8%) experienced ≥ grade 3 CRS, and five patients (20%) developed ≥ grade 3 ICANS. A meta-analysis evaluating CAR-T therapy in R/R CNSL revealed distinct toxicity profiles, with 30 PCNSL cases. Any-grade CRS and ICANS occurred in 70% and 53% of patients respectively, with grade 3-4 CRS and ICANS observed in 13% and 18% of cases. These toxicity profiles align with CAR-T registrational trials in systemic LBCL, without elevated neurotoxicity signals (117).
The collective evidence of high response rates and manageable toxicity supports CAR-T therapy as a promising therapeutic option for IP-LBCLs. Ongoing research should optimize durability and refine toxicity mitigation strategies.
While this review provides a comprehensive analysis of recent advances in targeted and immunotherapeutic approaches for IP-LBCLs, several limitations warrant consideration. First, given the rarity of IP-LBCLs and consequent limited patient population, conducting large-scale clinical trials remains challenging. Consequently, current evidence predominantly originates from case reports or small cohorts, inherently increasing the risk of stochastic bias and reducing statistical power. Therefore, cautious interpretation is warranted to avoid overgeneralization of findings. Second, inherent language bias must be acknowledged, as our inclusion criteria prioritized English-language publications. This selective approach may not encompass all relevant studies reported in other languages, potentially introducing geographic or institutional reporting biases.
In the era of targeted and immunotherapies, therapeutic strategies for IP-LBCLs have expanded significantly (Figure 2, Table 2). Agents including BTK inhibitors, IMiDs, and ICIs have demonstrated substantial clinical efficacy. Given that most IP-LBCLs belong to the molecularly defined MCD subtype with shared biological features, BTK inhibitors may represent an optimal therapeutic backbone for this lymphoma entity.
Figure 2. Mechanisms of different treatment regimens. (BTKi, Bruton’s tyrosine kinase inhibitor; IRAK4, Interleukin-1 receptor-associated kinase 4; ICIs, Immune checkpoint inhibitors; BsAbs, Bispecific antibodies; CAR, chimeric antigen receptor).
Emerging modalities such as PI3K/mTOR inhibitors, HDAC inhibitors, XOP1 inhibitors, BCL-2 inhibitors, IRAK4 inhibitors, bispecific antibodies, and CAR-T therapies further diversify treatment options. Future research should focus on elucidating the mechanistic underpinnings of these interventions to optimize therapeutic indices and develop personalized approaches tailored to molecular vulnerabilities.
HW: Writing – original draft, Writing – review & editing. YZ: Writing – review & editing. XW: Writing – review & editing. ZL: Writing – review & editing. OB: Funding acquisition, Writing – review & editing.
The author(s) declare that financial support was received for the research and/or publication of this article. This study was funded by the Department of Finance of Jilin Province (JLSWSRCZX2023-8).
We thank all participants for their contributions.
The authors declare that the research was conducted in the absence of any commercial or financial relationships that could be construed as a potential conflict of interest.
The author(s) declare that no Generative AI was used in the creation of this manuscript.
All claims expressed in this article are solely those of the authors and do not necessarily represent those of their affiliated organizations, or those of the publisher, the editors and the reviewers. Any product that may be evaluated in this article, or claim that may be made by its manufacturer, is not guaranteed or endorsed by the publisher.
1. Alaggio R, Amador C, Anagnostopoulos I, Attygalle AD, Araujo IBO, Berti E, et al. The 5th edition of the world health organization classification of haematolymphoid tumours: lymphoid neoplasms. Leukemia. (2022) 36:1720–48. doi: 10.1038/s41375-022-01620-2
2. Roschewski M, Phelan JD, Jaffe ES. Primary large B-cell lymphomas of immune-privileged sites. Blood. (2024). doi: 10.1182/blood.2023020911
3. Schaff LR, Grommes C. Primary central nervous system lymphoma. Blood. (2022) 140:971–9. doi: 10.1182/blood.2020008377
4. Ferreri AJM, Calimeri T, Cwynarski K, Dietrich J, Grommes C, Hoang-Xuan K, et al. Primary central nervous system lymphoma. Nat Rev Dis Primers. (2023) 9:29. doi: 10.1038/s41572-023-00439-0
5. Aquilanti E, Herrity E, Nayak L. Novel therapies for primary central nervous system lymphomas. Curr Neurol Neurosci Rep. (2024) 24:621–9. doi: 10.1007/s11910-024-01376-5
6. Chihara D, Fowler NH, Oki Y, Fanale MA, Nastoupil LJ, Westin JR, et al. Impact of histologic subtypes and treatment modality among patients with primary central nervous system lymphoma: a SEER database analysis. Oncotarget. (2018) 9:28897–902. doi: 10.18632/oncotarget.25622
7. Tang D, Chen Y, Shi Y, Tao H, Tao S, Zhang Q, et al. Epidemiologic characteristics, prognostic factors, and treatment outcomes in primary central nervous system lymphoma: A SEER-based study. Front Oncol. (2022) 12:817043. doi: 10.3389/fonc.2022.817043
8. Ferreri AJM, Illerhaus G, Doorduijn JK, Auer DP, Bromberg JEC, Calimeri T, et al. Primary central nervous system lymphomas: EHA-ESMO Clinical Practice Guideline for diagnosis, treatment and follow-up. Hemasphere. (2024) 8:e89. doi: 10.1002/hem3.89
9. Chen T, Liu Y, Wang Y, Chang Q, Wu J, Wang Z, et al. Evidence-based expert consensus on the management of primary central nervous system lymphoma in China. J Hematol Oncol. (2022) 15:136. doi: 10.1186/s13045-022-01356-7
10. Hoang-Xuan K, Deckert M, Ferreri AJM, Furtner J, Gallego-Perez-Larraya J, Henriksson R, et al. European Association of Neuro-Oncology (EANO) guidelines for treatment of primary central nervous system lymphoma (PCNSL). Neuro Oncol. (2023) 25:37–53. doi: 10.1093/neuonc/noac196
11. Soussain C, Malaise D, Cassoux N. Primary vitreoretinal lymphoma: a diagnostic and management challenge. Blood. (2021) 138:1519–34. doi: 10.1182/blood.2020008235
12. Malaise D, Houillier C, Touitou V, Choquet S, Maloum K, Le-Garff-Tavernier M, et al. Primary vitreoretinal lymphoma: short review of the literature, results of a European survey and French guidelines of the LOC network for diagnosis, treatment and follow-up. Curr Opin Oncol. (2021) 33:420–31. doi: 10.1097/cco.0000000000000776
13. Zhou N, Xu X, Liu Y, Wang Y, Wei W. A proposed protocol of intravitreal injection of methotrexate for treatment of primary vitreoretinal lymphoma. Eye (Lond). (2022) 36:1448–55. doi: 10.1038/s41433-021-01657-0
14. Castellino A, Pulido JS, Johnston PB, Ristow KM, Nora Bennani N, Inwards DJ, et al. Role of systemic high-dose methotrexate and combined approaches in the management of vitreoretinal lymphoma: A single center experience 1990-2018. Am J Hematol. (2019) 94:291–8. doi: 10.1002/ajh.25350
15. Kvopka M, Lake SR, Smith JR. Intraocular chemotherapy for vitreoretinal lymphoma: A review. Clin Exp Ophthalmol. (2020) 48:240–8. doi: 10.1111/ceo.13668
16. Cheah CY, Wirth A, Seymour JF. Primary testicular lymphoma. Blood. (2014) 123:486–93. doi: 10.1182/blood-2013-10-530659
17. Berjaoui MB, Herrera-Caceres JO, Li T, Qaoud Y, Tiwari R, Ma D, et al. Age related differences in primary testicular lymphoma: A population based cohort study. Urol Oncol. (2023) 41:151.e1–.e10. doi: 10.1016/j.urolonc.2022.10.032
18. Sun Y, Yan X, Zhao H, Cui Z, Wang Y, Sun S, et al. Characteristic features of primary testicular lymphoma and survival trends: a multicenter clinical study. Hematology. (2022) 27:1019–25. doi: 10.1080/16078454.2022.2119345
19. Ponzoni M, Ferreri AJ, Campo E, Facchetti F, Mazzucchelli L, Yoshino T, et al. Definition, diagnosis, and management of intravascular large B-cell lymphoma: proposals and perspectives from an international consensus meeting. J Clin Oncol. (2007) 25:3168–73. doi: 10.1200/jco.2006.08.2313
20. Liu Z, Zhang Y, Zhu Y, Zhang W. Prognosis of intravascular large B cell lymphoma (IVLBCL): analysis of 182 patients from global case series. Cancer Manag Res. (2020) 12:10531–40. doi: 10.2147/cmar.S267825
21. Chapuy B, Roemer MG, Stewart C, Tan Y, Abo RP, Zhang L, et al. Targetable genetic features of primary testicular and primary central nervous system lymphomas. Blood. (2016) 127:869–81. doi: 10.1182/blood-2015-10-673236
22. Radke J, Ishaque N, Koll R, Gu Z, Schumann E, Sieverling L, et al. The genomic and transcriptional landscape of primary central nervous system lymphoma. Nat Commun. (2022) 13:2558. doi: 10.1038/s41467-022-30050-y
23. Twa DDW, Mottok A, Savage KJ, Steidl C. The pathobiology of primary testicular diffuse large B-cell lymphoma: Implications for novel therapies. Blood Rev. (2018) 32:249–55. doi: 10.1016/j.blre.2017.12.001
24. Shimada K, Yoshida K, Suzuki Y, Iriyama C, Inoue Y, Sanada M, et al. Frequent genetic alterations in immune checkpoint-related genes in intravascular large B-cell lymphoma. Blood. (2021) 137:1491–502. doi: 10.1182/blood.2020007245
25. Schmitz R, Wright GW, Huang DW, Johnson CA, Phelan JD, Wang JQ, et al. Genetics and pathogenesis of diffuse large B-cell lymphoma. N Engl J Med. (2018) 378:1396–407. doi: 10.1056/NEJMoa1801445
26. Wright GW, Huang DW, Phelan JD, Coulibaly ZA, Roulland S, Young RM, et al. A probabilistic classification tool for genetic subtypes of diffuse large B cell lymphoma with therapeutic implications. Cancer Cell. (2020) 37:551–68.e14. doi: 10.1016/j.ccell.2020.03.015
27. Phelan JD, Young RM, Webster DE, Roulland S, Wright GW, Kasbekar M, et al. A multiprotein supercomplex controlling oncogenic signalling in lymphoma. Nature. (2018) 560:387–91. doi: 10.1038/s41586-018-0290-0
28. Phelan JD, Scheich S, Choi J, Wright GW, Häupl B, Young RM, et al. Response to Bruton’s tyrosine kinase inhibitors in aggressive lymphomas linked to chronic selective autophagy. Cancer Cell. (2024) 42:238–52.e9. doi: 10.1016/j.ccell.2023.12.019
29. Goldwirt L, Beccaria K, Ple A, Sauvageon H, Mourah S. Ibrutinib brain distribution: a preclinical study. Cancer Chemother Pharmacol. (2018) 81:783–9. doi: 10.1007/s00280-018-3546-3
30. Zhang Y, Li Y, Zhuang Z, Wang W, Wei C, Zhao D, et al. Preliminary evaluation of zanubrutinib-containing regimens in DLBCL and the cerebrospinal fluid distribution of zanubrutinib: A 13-case series. Front Oncol. (2021) 11:760405. doi: 10.3389/fonc.2021.760405
31. Yu H, Kong H, Li C, Dong X, Wu Y, Zhuang Y, et al. Bruton’s tyrosine kinase inhibitors in primary central nervous system lymphoma-evaluation of anti-tumor efficacy and brain distribution. Transl Cancer Res. (2021) 10:1975–83. doi: 10.21037/tcr-21-50
32. Grommes C, Pastore A, Palaskas N, Tang SS, Campos C, Schartz D, et al. Ibrutinib unmasks critical role of bruton tyrosine kinase in primary CNS lymphoma. Cancer Discovery. (2017) 7:1018–29. doi: 10.1158/2159-8290.Cd-17-0613
33. Grommes C, Nandakumar S, Schaff LR, Gavrilovic I, Kaley TJ, Nolan CP, et al. A Phase II study assessing Long-Term Response to Ibrutinib Monotherapy in recurrent or refractory CNS Lymphoma. Clin Cancer Res. (2024) 30:4005–15. doi: 10.1158/1078-0432.Ccr-24-0605
34. Ping L, Wang X, Gao Y, Bai B, Huang H, Cai L, et al. High-dose methotrexate, ibrutinib and temozolomide (MIT) for the treatment of newly diagnosed primary central nervous system lymphoma: A multi-center prospective phase II study. Blood. (2023) 142:3086–. doi: 10.1182/blood-2023-185212
35. Xiao X, Chen P, Jiang H, Li X, Xie J, Qian W. Zanubrutinib in combination with R-MTX for newly diagnosed primary central nervous system lymphoma. Blood. (2024) 144:6484–. doi: 10.1182/blood-2024-202792
36. Bairey O, Taliansky A, Glik A, Amiel A, Yust-Katz S, Gurion R, et al. A phase 2 study of ibrutinib maintenance following first-line high-dose methotrexate-based chemotherapy for elderly patients with primary central nervous system lymphoma. Cancer. (2023) 129:3905–14. doi: 10.1002/cncr.34985
37. Xing L, Wei H, Liu D, Wang H, Li P, Xie L, et al. Methotrexate in combination with orelabrutinib, thiotepa, and rituximab (R-MTO) followed by autologous stem-cell transplant for primary central nervous system lymphoma treatment. Blood. (2023) 142:6228–. doi: 10.1182/blood-2023-186219
38. Nagane M, Narita Y, Mishima K, Terui Y, Arakawa Y, Yonezawa H, et al. Phase 1/2 study of tirabrutinib (ONO/GS-4059), a next-generation Bruton’s tyrosine kinase (BTK) inhibitor, monotherapy in patients with relapsed/refractory primary central nervous system lymphoma (PCNSL). Blood. (2019) 134:1586–. doi: 10.1182/blood-2019-123362
39. Li W, Kang Z, Chen F, Li S, Chen X, Yuan X, et al. Preliminary efficacy and safety of Bruton tyrosine kinase (BTK) inhibitor HZ-a-018 in adult patients with relapsed and/or refractory(R/R)Central nervous system lymphoma, results from the phase Ia monotherapy of HZ-a-018-102 study. Blood. (2024) 144:6510–. doi: 10.1182/blood-2024-201137
40. Li W, Kang Z, Chen F, Li S, Chen X, Yuan X, et al. Preliminary efficacy and safety of Bruton tyrosine kinase (BTK) inhibitor HZ-a-018 in adult patients with relapsed and/or refractory (R/R) central nervous system lymphoma or treatment-naïve (TN) central nervous system lymphoma, results from the phase Ib combination part of HZ-a-018-102 study. Blood. (2024) 144:6482–. doi: 10.1182/blood-2024-201166
41. Zhang Y, Jia C, Wang W, Zhang L, Cao X, Li J, et al. The interim analysis from a prospective single-center phase 2 study of zanubrutinib plus R-CHOP in treat-naïve intravascular large B cell lymphoma. Blood. (2021) 138:3563–. doi: 10.1182/blood-2021-152417
42. Glennie MJ, French RR, Cragg MS, Taylor RP. Mechanisms of killing by anti-CD20 monoclonal antibodies. Mol Immunol. (2007) 44:3823–37. doi: 10.1016/j.molimm.2007.06.151
43. Winter MC, Hancock BW. Ten years of rituximab in NHL. Expert Opin Drug Saf. (2009) 8:223–35. doi: 10.1517/14740330902750114
44. Zhou X, Hu W, Qin X. The role of complement in the mechanism of action of rituximab for B-cell lymphoma: implications for therapy. Oncologist. (2008) 13:954–66. doi: 10.1634/theoncologist.2008-0089
45. Czuczman MS, Gregory SA. The future of CD20 monoclonal antibody therapy in B-cell Malignancies. Leuk Lymphoma. (2010) 51:983–94. doi: 10.3109/10428191003717746
46. Rubenstein JL, Combs D, Rosenberg J, Levy A, McDermott M, Damon L, et al. Rituximab therapy for CNS lymphomas: targeting the leptomeningeal compartment. Blood. (2003) 101:466–8. doi: 10.1182/blood-2002-06-1636
47. Iwamoto FM, Schwartz J, Pandit-Taskar N, Peak S, Divgi CR, Zelenetz AD, et al. Study of radiolabeled indium-111 and yttrium-90 ibritumomab tiuxetan in primary central nervous system lymphoma. Cancer. (2007) 110:2528–34. doi: 10.1002/cncr.23077
48. Bromberg JEC, Issa S, Bakunina K, Minnema MC, Seute T, Durian M, et al. Rituximab in patients with primary CNS lymphoma (HOVON 105/ALLG NHL 24): a randomised, open-label, phase 3 intergroup study. Lancet Oncol. (2019) 20:216–28. doi: 10.1016/s1470-2045(18)30747-2
49. Ferreri AJ, Cwynarski K, Pulczynski E, Ponzoni M, Deckert M, Politi LS, et al. Chemoimmunotherapy with methotrexate, cytarabine, thiotepa, and rituximab (MATRix regimen) in patients with primary CNS lymphoma: results of the first randomisation of the International Extranodal Lymphoma Study Group-32 (IELSG32) phase 2 trial. Lancet Haematol. (2016) 3:e217–27. doi: 10.1016/s2352-3026(16)00036-3
50. Ferreri AJM, Cwynarski K, Pulczynski E, Fox CP, Schorb E, Celico C, et al. Long-term efficacy, safety and neurotolerability of MATRix regimen followed by autologous transplant in primary CNS lymphoma: 7-year results of the IELSG32 randomized trial. Leukemia. (2022) 36:1870–8. doi: 10.1038/s41375-022-01582-5
51. Ollila TA, Taher R, Moku P, Olszewski AJ. Immunochemotherapy or chemotherapy alone in primary central nervous system lymphoma: a National Cancer Database analysis. Blood Adv. (2023) 7:5470–9. doi: 10.1182/bloodadvances.2023010352
52. Schmitt AM, Herbrand AK, Fox CP, Bakunina K, Bromberg JEC, Cwynarski K, et al. Rituximab in primary central nervous system lymphoma-A systematic review and meta-analysis. Hematol Oncol. (2019) 37:548–57. doi: 10.1002/hon.2666
53. Mazloom A, Fowler N, Medeiros LJ, Iyengar P, Horace P, Dabaja BS. Outcome of patients with diffuse large B-cell lymphoma of the testis by era of treatment: the M. D. Anderson Cancer Center experience. Leuk Lymphoma. (2010) 51:1217–24. doi: 10.3109/10428191003793358
54. Vitolo U, Chiappella A, Ferreri AJ, Martelli M, Baldi I, Balzarotti M, et al. First-line treatment for primary testicular diffuse large B-cell lymphoma with rituximab-CHOP, CNS prophylaxis, and contralateral testis irradiation: final results of an international phase II trial. J Clin Oncol. (2011) 29:2766–72. doi: 10.1200/jco.2010.31.4187
55. Conconi A, Chiappella A, Ferreri AJM, Stathis A, Botto B, Sassone M, et al. IELSG30 phase 2 trial: intravenous and intrathecal CNS prophylaxis in primary testicular diffuse large B-cell lymphoma. Blood Adv. (2024) 8:1541–9. doi: 10.1182/bloodadvances.2023011251
56. Shimada K, Yamaguchi M, Atsuta Y, Matsue K, Sato K, Kusumoto S, et al. Rituximab, cyclophosphamide, doxorubicin, vincristine, and prednisolone combined with high-dose methotrexate plus intrathecal chemotherapy for newly diagnosed intravascular large B-cell lymphoma (PRIMEUR-IVL): a multicentre, single-arm, phase 2 trial. Lancet Oncol. (2020) 21:593–602. doi: 10.1016/s1470-2045(20)30059-0
57. Shimada K, Yamaguchi M, Kuwatsuka Y, Matsue K, Sato K, Kusumoto S, et al. Rituximab, cyclophosphamide, doxorubicin, vincristine, and prednisolone combined with high-dose methotrexate plus intrathecal chemotherapy for newly diagnosed intravascular large B-cell lymphoma (PRIMEUR-IVL): long-term results of a multicentre, single-arm, phase 2 trial. EClinicalMedicine. (2025) 80:103078. doi: 10.1016/j.eclinm.2025.103078
58. Arora M, Gowda S, Tuscano J. A comprehensive review of lenalidomide in B-cell non-Hodgkin lymphoma. Ther Adv Hematol. (2016) 7:209–21. doi: 10.1177/2040620716652861
59. Gribben JG, Fowler N, Morschhauser F. Mechanisms of action of lenalidomide in B-cell non-Hodgkin lymphoma. J Clin Oncol. (2015) 33:2803–11. doi: 10.1200/jco.2014.59.5363
60. Witzig TE, Nowakowski GS, Habermann TM, Goy A, Hernandez-Ilizaliturri FJ, Chiappella A, et al. A comprehensive review of lenalidomide therapy for B-cell non-Hodgkin lymphoma. Ann Oncol. (2015) 26:1667–77. doi: 10.1093/annonc/mdv102
61. Zhang L, Qian Z, Cai Z, Sun L, Wang H, Bartlett JB, et al. Synergistic antitumor effects of lenalidomide and rituximab on mantle cell lymphoma in vitro and in vivo. Am J Hematol. (2009) 84:553–9. doi: 10.1002/ajh.21468
62. Ghesquieres H, Chevrier M, Laadhari M, Chinot O, Choquet S, Moluçon-Chabrot C, et al. Lenalidomide in combination with intravenous rituximab (REVRI) in relapsed/refractory primary CNS lymphoma or primary intraocular lymphoma: a multicenter prospective ‘proof of concept’ phase II study of the French Oculo-Cerebral lymphoma (LOC) Network and the Lymphoma Study Association (LYSA)†. Ann Oncol. (2019) 30:621–8. doi: 10.1093/annonc/mdz032
63. Yuan X, Xie Y, Xu N, Liu H, Chen P, Zhao A, et al. Lenalidomide, rituximab, and methotrexate are effective in newly diagnosed primary central nervous system lymphoma. Haematologica. (2024) 109:2005–9. doi: 10.3324/haematol.2023.284834
64. Engelhardt M, Ajayi S, Reinhardt H, Müller SJ, Dold SM, Wäsch R. Pomalidomide. Recent Results Cancer Res. (2018) 212:169–85. doi: 10.1007/978-3-319-91439-8_8
65. Hernandez-Ilizaliturri FJ, Reddy N, Holkova B, Ottman E, Czuczman MS. Immunomodulatory drug CC-5013 or CC-4047 and rituximab enhance antitumor activity in a severe combined immunodeficient mouse lymphoma model. Clin Cancer Res. (2005) 11:5984–92. doi: 10.1158/1078-0432.Ccr-05-0577
66. Li Z, Qiu Y, Personett D, Huang P, Edenfield B, Katz J, et al. Pomalidomide shows significant therapeutic activity against CNS lymphoma with a major impact on the tumor microenvironment in murine models. PloS One. (2013) 8:e71754. doi: 10.1371/journal.pone.0071754
67. Tun HW, Johnston PB, DeAngelis LM, Atherton PJ, Pederson LD, Koenig PA, et al. Phase 1 study of pomalidomide and dexamethasone for relapsed/refractory primary CNS or vitreoretinal lymphoma. Blood. (2018) 132:2240–8. doi: 10.1182/blood-2018-02-835496
68. Naimi A, Mohammed RN, Raji A, Chupradit S, Yumashev AV, Suksatan W, et al. Tumor immunotherapies by immune checkpoint inhibitors (ICIs); the pros and cons. Cell Commun Signal. (2022) 20:44. doi: 10.1186/s12964-022-00854-y
69. Li N, Hou X, Huang S, Tai R, Lei L, Li S, et al. Biomarkers related to immune checkpoint inhibitors therapy. BioMed Pharmacother. (2022) 147:112470. doi: 10.1016/j.biopha.2021.112470
70. Takashima Y, Kawaguchi A, Sato R, Yoshida K, Hayano A, Homma J, et al. Differential expression of individual transcript variants of PD-1 and PD-L2 genes on Th-1/Th-2 status is guaranteed for prognosis prediction in PCNSL. Sci Rep. (2019) 9:10004. doi: 10.1038/s41598-019-46473-5
71. Sakakibara A, Inagaki Y, Imaoka E, Ishikawa E, Shimada S, Shimada K, et al. Autopsy case report of intravascular large B-cell lymphoma with neoplastic PD-L1 expression. J Clin Exp Hematop. (2018) 58:32–5. doi: 10.3960/jslrt.17037
72. Zhang W, Yang P, Yang Y, Liu S, Xu Y, Wu C, et al. Genomic landscape and distinct molecular subtypes of primary testicular lymphoma. J Transl Med. (2024) 22:414. doi: 10.1186/s12967-024-05140-8
73. Nayak L, Iwamoto FM, LaCasce A, Mukundan S, Roemer MGM, Chapuy B, et al. PD-1 blockade with nivolumab in relapsed/refractory primary central nervous system and testicular lymphoma. Blood. (2017) 129:3071–3. doi: 10.1182/blood-2017-01-764209
74. Terziev D, Hutter B, Klink B, Stenzinger A, Stögbauer F, Glimm H, et al. Nivolumab maintenance after salvage autologous stem cell transplantation results in long-term remission in multiple relapsed primary CNS lymphoma. Eur J Haematol. (2018) 101:115–8. doi: 10.1111/ejh.13072
75. Zeng Z, Yang A, Yang J, Zhang S, Xing Z, Wang X, et al. Sintilimab (anti-PD-1 antibody) combined with high-dose methotrexate, temozolomide, and rituximab (anti-CD20 antibody) in primary central nervous system lymphoma: a phase 2 study. Signal Transduct Target Ther. (2024) 9:229. doi: 10.1038/s41392-024-01941-x
76. Yin Q, Wu L, Han L, Zheng X, Tong R, Li L, et al. Immune-related adverse events of immune checkpoint inhibitors: a review. Front Immunol. (2023) 14:1167975. doi: 10.3389/fimmu.2023.1167975
77. Tummala S. Immune-checkpoint inhibitors and neurological adverse events. Lancet Neurol. (2023) 22:1093–4. doi: 10.1016/s1474-4422(23)00413-1
78. Fonseca E, Cabrera-Maqueda JM, Ruiz-García R, Naranjo L, Diaz-Pedroche C, Velasco R, et al. Neurological adverse events related to immune-checkpoint inhibitors in Spain: a retrospective cohort study. Lancet Neurol. (2023) 22:1150–9. doi: 10.1016/s1474-4422(23)00335-6
79. Farina A, Villagrán-García M, Fourier A, Pinto AL, Chorfa F, Timestit N, et al. Diagnostic and prognostic biomarkers in immune checkpoint inhibitor-related encephalitis: a retrospective cohort study. Lancet Reg Health Eur. (2024) 44:101011. doi: 10.1016/j.lanepe.2024.101011
80. Glaviano A, Foo ASC, Lam HY, Yap KCH, Jacot W, Jones RH, et al. PI3K/AKT/mTOR signaling transduction pathway and targeted therapies in cancer. Mol Cancer. (2023) 22:138. doi: 10.1186/s12943-023-01827-6
81. Tewari D, Patni P, Bishayee A, Sah AN, Bishayee A. Natural products targeting the PI3K-Akt-mTOR signaling pathway in cancer: A novel therapeutic strategy. Semin Cancer Biol. (2022) 80:1–17. doi: 10.1016/j.semcancer.2019.12.008
82. Zhang X, Wu Y, Sun X, Cui Q, Bai X, Dong G, et al. The PI3K/AKT/mTOR signaling pathway is aberrantly activated in primary central nervous system lymphoma and correlated with a poor prognosis. BMC Cancer. (2022) 22:190. doi: 10.1186/s12885-022-09275-z
83. Korfel A, Schlegel U, Herrlinger U, Dreyling M, Schmidt C, von Baumgarten L, et al. Phase II trial of temsirolimus for relapsed/refractory primary CNS lymphoma. J Clin Oncol. (2016) 34:1757–63. doi: 10.1200/jco.2015.64.9897
84. Grommes C, Pentsova E, Schaff LR, Nolan CP, Kaley T, Reiner AS, et al. Preclinical and clinical evaluation of Buparlisib (BKM120) in recurrent/refractory Central Nervous System Lymphoma. Leuk Lymphoma. (2023) 64:1545–53. doi: 10.1080/10428194.2023.2223734
85. Wang N, Mo Z, Pan L, Zhou M, Ye X, Liu X, et al. Dual PI3K/HDAC inhibitor BEBT-908 exhibits potent efficacy as monotherapy for primary central nervous system lymphoma. Target Oncol. (2023) 18:941–52. doi: 10.1007/s11523-023-01006-z
86. Kuo MH, Allis CD. Roles of histone acetyltransferases and deacetylases in gene regulation. Bioessays. (1998) 20:615–26. doi: 10.1002/(sici)1521-1878(199808)20:8<615::Aid-bies4>3.0.Co;2-h
87. Dawson MA, Kouzarides T. Cancer epigenetics: from mechanism to therapy. Cell. (2012) 150:12–27. doi: 10.1016/j.cell.2012.06.013
88. Cao HY, Li L, Xue SL, Dai HP. Chidamide: Targeting epigenetic regulation in the treatment of hematological Malignancy. Hematol Oncol. (2023) 41:301–9. doi: 10.1002/hon.3088
89. Frew AJ, Johnstone RW, Bolden JE. Enhancing the apoptotic and therapeutic effects of HDAC inhibitors. Cancer Lett. (2009) 280:125–33. doi: 10.1016/j.canlet.2009.02.042
90. Zhang MC, Fang Y, Wang L, Cheng S, Fu D, He Y, et al. Clinical efficacy and molecular biomarkers in a phase II study of tucidinostat plus R-CHOP in elderly patients with newly diagnosed diffuse large B-cell lymphoma. Clin Epigenetics. (2020) 12:160. doi: 10.1186/s13148-020-00948-9
91. Chen X, Xie L, Zhu J, Liang L, Zou B, Zou L. Real-world efficacy of chidamide plus R-CHOP in newly diagnosed double-expressor diffuse large B-cell lymphoma. Ther Adv Hematol. (2024) 15:20406207241292446. doi: 10.1177/20406207241292446
92. Zhang Y, Guo W, Zhao Y, Zhan Z, Wang H, Li Z, et al. Chidamide: A new exploration of maintenance therapy for DLBCL patients with HBV infection. Blood. (2024) 144:4490–. doi: 10.1182/blood-2024-204677
93. Yang H, Li C, Chen Z, Mou H, Gu L. Determination of chidamide in rat plasma and cerebrospinal fluid. Regul Toxicol Pharmacol. (2018) 98:24–30. doi: 10.1016/j.yrtph.2018.07.001
94. Chen G-L, Jin J, Sun H, Liu Y, Zhang Q, Xia Z, et al. Phase II clinical trial on chidamide, rituximab and high-dose methotrexate (C-R-HDMTX) in newly diagnosed primary CNS lymphoma. Blood. (2024) 144:6526–. doi: 10.1182/blood-2024-194111
95. Nachmias B, Schimmer AD. Targeting nuclear import and export in hematological Malignancies. Leukemia. (2020) 34:2875–86. doi: 10.1038/s41375-020-0958-y
96. Azizian NG, Li Y. XPO1-dependent nuclear export as a target for cancer therapy. J Hematol Oncol. (2020) 13:61. doi: 10.1186/s13045-020-00903-4
97. Kalakonda N, Maerevoet M, Cavallo F, Follows G, Goy A, Vermaat JSP, et al. Selinexor in patients with relapsed or refractory diffuse large B-cell lymphoma (SADAL): a single-arm, multinational, multicentre, open-label, phase 2 trial. Lancet Haematol. (2020) 7:e511–e22. doi: 10.1016/s2352-3026(20)30120-4
98. Bobillo S, Abrisqueta P, Carpio C, Raheja P, Castellví J, Crespo M, et al. Promising activity of selinexor in the treatment of a patient with refractory diffuse large B-cell lymphoma and central nervous system involvement. Haematologica. (2018) 103:e92–e3. doi: 10.3324/haematol.2017.181636
99. Xu J, Dong X, Huang DCS, Xu P, Zhao Q, Chen B. Current advances and future strategies for BCL-2 inhibitors: potent weapons against cancers. Cancers (Basel). (2023) 15. doi: 10.3390/cancers15204957
100. Poynton E, Chernucha E, Day J, Prodger C, Hopkins D, Rakesh P, et al. Impact of MYC and BCL2 double expression on outcomes in primary CNS lymphoma: a UK multicenter analysis. Blood Adv. (2024) 8:1772–5. doi: 10.1182/bloodadvances.2023011426
101. Ackler S, Oleksijew A, Chen J, Chyla BJ, Clarin J, Foster K, et al. Clearance of systemic hematologic tumors by venetoclax (Abt-199) and navitoclax. Pharmacol Res Perspect. (2015) 3:e00178. doi: 10.1002/prp2.178
102. Diefenbach CS, Zelenetz AD, Herbaux C, Tani M, Houot R, Bastos-Oreiro M, et al. Final analysis of the safety and efficacy of venetoclax in combination with pola-R-CHP for untreated high-risk BCL-2-positive B-cell lymphoma including double/triple hit lymphoma. Blood. (2024) 144:577–. doi: 10.1182/blood-2024-198624
103. Gummadi VR, Boruah A, Ainan BR, Vare BR, Manda S, Gondle HP, et al. Discovery of CA-4948, an orally bioavailable IRAK4 inhibitor for treatment of hematologic Malignancies. ACS Med Chem Lett. (2020) 11:2374–81. doi: 10.1021/acsmedchemlett.0c00255
104. Yoon SB, Hong H, Lim HJ, Choi JH, Choi YP, Seo SW, et al. A novel IRAK4/PIM1 inhibitor ameliorates rheumatoid arthritis and lymphoid Malignancy by blocking the TLR/MYD88-mediated NF-κB pathway. Acta Pharm Sin B. (2023) 13:1093–109. doi: 10.1016/j.apsb.2022.12.001
105. Parrondo RD, Iqbal M, Von Roemeling R, Von Roemeling C, Tun HW. IRAK-4 inhibition: emavusertib for the treatment of lymphoid and myeloid Malignancies. Front Immunol. (2023) 14:1239082. doi: 10.3389/fimmu.2023.1239082
106. Guidetti F, Arribas AJ, Sartori G, Spriano F, Barnabei L, Tarantelli C, et al. Targeting IRAK4 with emavusertib in lymphoma models with secondary resistance to PI3K and BTK inhibitors. J Clin Med. (2023) 12. doi: 10.3390/jcm12020399
107. Von Roemeling CA, Doonan BP, Klippel K, Schultz D, Hoang-Minh L, Trivedi V, et al. Oral IRAK-4 inhibitor CA-4948 is blood-brain barrier penetrant and has single-agent activity against CNS lymphoma and melanoma brain metastases. Clin Cancer Res. (2023) 29:1751–62. doi: 10.1158/1078-0432.Ccr-22-1682
108. Nowakowski GS, Dabrowska-Iwanicka AP, Grommes C, Tun HW, D’ Angelo C, de la Fuente Burguera A, et al. Preliminary safety and efficacy of emavusertib (CA-4948) in combination with ibrutinib in relapsed/refractory primary central nervous system lymphoma patients with prior exposure to BTK inhibitor. Blood. (2024) 144:6020–. doi: 10.1182/blood-2024-208359
109. Shirley M. Glofitamab: first approval. Drugs. (2023) 83:935–41. doi: 10.1007/s40265-023-01894-5
110. Dickinson MJ, Carlo-Stella C, Morschhauser F, Bachy E, Corradini P, Iacoboni G, et al. Glofitamab for relapsed or refractory diffuse large B-cell lymphoma. N Engl J Med. (2022) 387:2220–31. doi: 10.1056/NEJMoa2206913
111. Godfrey JK, Gao L, Shouse G, Song JY, Pak S, Lee B, et al. Glofitamab stimulates immune cell infiltration of CNS tumors and induces clinical responses in secondary CNS lymphoma. Blood. (2024) 144:457–61. doi: 10.1182/blood.2024024168
112. Berning P, Shumilov E, Maulhardt M, Boyadzhiev H, Kerkhoff A, Call S, et al. Chimeric antigen receptor-T cell therapy shows similar efficacy and toxicity in patients with diffuse large B-cell lymphoma aged 70 and older compared to younger patients: A multicenter cohort study. Hemasphere. (2024) 8:e54. doi: 10.1002/hem3.54
113. Chong EA, Schuster SJ. No CNS sanctuary for lymphoma from CAR T. Blood. (2022) 139:2261–3. doi: 10.1182/blood.2022015802
114. Mercadal S, Ahn KW, Allbee-Johnson M, Ganguly S, Geethakumari PR, Hong S, et al. Outcomes of patients with primary central nervous system lymphoma following CD19-targeted chimeric antigen receptor T-cell therapy. Haematologica. (2024) 110:218–21. doi: 10.3324/haematol.2024.285613
115. Frigault MJ, Dietrich J, Gallagher K, Roschewski M, Jordan JT, Forst D, et al. Safety and efficacy of tisagenlecleucel in primary CNS lymphoma: a phase 1/2 clinical trial. Blood. (2022) 139:2306–15. doi: 10.1182/blood.2021014738
116. Choquet S, Soussain C, Azar N, Morel V, Metz C, Ursu R, et al. CAR T-cell therapy induces a high rate of prolonged remission in relapsed primary CNS lymphoma: Real-life results of the LOC network. Am J Hematol. (2024) 99:1240–9. doi: 10.1002/ajh.27316
117. Abramson JS, Palomba ML, Gordon LI, Lunning MA, Wang M, Arnason J, et al. Lisocabtagene maraleucel for patients with relapsed or refractory large B-cell lymphomas (TRANSCEND NHL 001): a multicentre seamless design study. Lancet. (2020) 396:839–52. doi: 10.1016/s0140-6736(20)31366-0
Keywords: primary large B-cell lymphomas of immune-privileged sites, targeted therapy, immunotherapy, efficacy, safety
Citation: Wang H, Zhang Y, Wan X, Li Z and Bai O (2025) In the era of targeted therapy and immunotherapy: advances in the treatment of large B-cell lymphoma of immune-privileged sites. Front. Immunol. 16:1547377. doi: 10.3389/fimmu.2025.1547377
Received: 18 December 2024; Accepted: 21 March 2025;
Published: 01 April 2025.
Edited by:
Tuoen Liu, West Virginia School of Osteopathic Medicine, United StatesReviewed by:
Francisco Martin, University of Granada, SpainCopyright © 2025 Wang, Zhang, Wan, Li and Bai. This is an open-access article distributed under the terms of the Creative Commons Attribution License (CC BY). The use, distribution or reproduction in other forums is permitted, provided the original author(s) and the copyright owner(s) are credited and that the original publication in this journal is cited, in accordance with accepted academic practice. No use, distribution or reproduction is permitted which does not comply with these terms.
*Correspondence: Ou Bai, YmFpb3VAamx1LmVkdS5jbg==
Disclaimer: All claims expressed in this article are solely those of the authors and do not necessarily represent those of their affiliated organizations, or those of the publisher, the editors and the reviewers. Any product that may be evaluated in this article or claim that may be made by its manufacturer is not guaranteed or endorsed by the publisher.
Research integrity at Frontiers
Learn more about the work of our research integrity team to safeguard the quality of each article we publish.