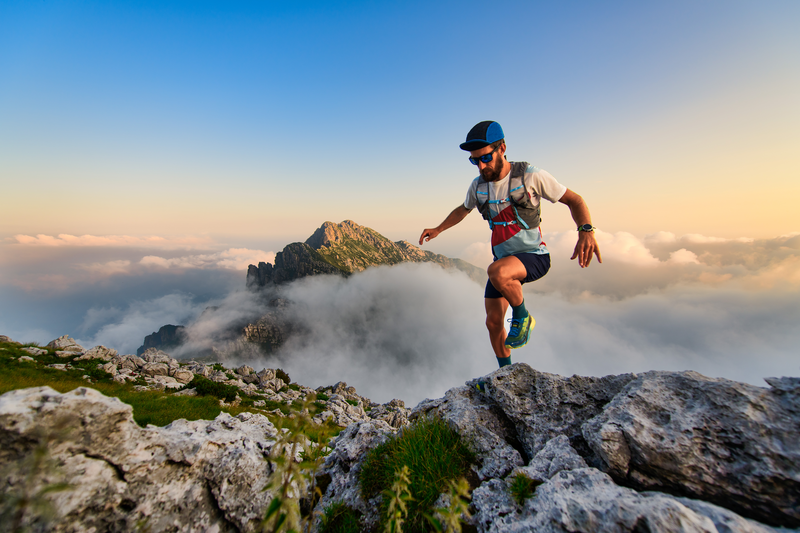
94% of researchers rate our articles as excellent or good
Learn more about the work of our research integrity team to safeguard the quality of each article we publish.
Find out more
ORIGINAL RESEARCH article
Front. Immunol.
Sec. Comparative Immunology
Volume 16 - 2025 | doi: 10.3389/fimmu.2025.1547193
The final, formatted version of the article will be published soon.
You have multiple emails registered with Frontiers:
Please enter your email address:
If you already have an account, please login
You don't have a Frontiers account ? You can register here
Catfish production is the primary aquaculture sector in the United States, and the key cultured species is channel catfish (Ictalurus punctatus). The major cause of production losses are pathogenic diseases, and the spleen, an important site of adaptive immunity, is implicated in these diseases. To examine the channel catfish immune system, single-nuclei transcriptomes of sorted and captured IgM + cells were produced from adult channel catfish. Three channel catfish (~1 kg) were euthanized, the spleen dissected, and tissue dissociated. The lymphocytes were isolated using a Ficoll gradient, then IgM + cells were sorted with flow cytometry. The IgM + cells were lysed and single-nuclei libraries generated using a Chromium Next GEM Single Cell 3' GEM Kit and the Chromium X Instrument (10x Genomics) and sequenced with the Illumina NovaSeq X Plus sequencer. The reads were aligned to the I. punctatus reference assembly (Coco_2.0) using Cell Ranger, and normalization, cluster analysis and differential gene expression analysis were carried out with Seurat. Across the three samples approximately 753.5 million reads were generated for 18,686 cells. After filtering, 10,637 cells remained for the cluster analysis. The cluster analysis identified 16 clusters which were classified as B cells (10,276), natural killer-like (NK-like) cells (178), T cells or natural killer cells (45), hematopoietic stem and progenitor cells (HSPC) (66), myeloid/epithelial cells (40) and plasma cells (32). The B cell clusters were further defined as immature B cells, mature B cells, cycling B cells and plasma cells. The plasma cells highly expressed ighm and we demonstrate that the secreted form of the transcript was largely being expressed by these cells. This atlas provides an insight into the gene expression of IgM + immune cells in the channel catfish. The atlas is publicly available and could be used garner more important information regarding the gene expression of splenic immune cells.
Keywords: single-cell RNA sequencing, Single-nuclei RNA sequencing, B cell, Plasma cell, T cell, lymphocyte, Natural Killer-like, Hematopoietic stem and progenitor cells
Received: 17 Dec 2024; Accepted: 14 Feb 2025.
Copyright: © 2025 Aldersey, Abernathy, Beck and Lange. This is an open-access article distributed under the terms of the Creative Commons Attribution License (CC BY). The use, distribution or reproduction in other forums is permitted, provided the original author(s) or licensor are credited and that the original publication in this journal is cited, in accordance with accepted academic practice. No use, distribution or reproduction is permitted which does not comply with these terms.
* Correspondence:
Miles D Lange, Aquatic Animal Health Research, Agricultural Research Service (USDA), Auburn, United States
Disclaimer: All claims expressed in this article are solely those of the authors and do not necessarily represent those of their affiliated organizations, or those of the publisher, the editors and the reviewers. Any product that may be evaluated in this article or claim that may be made by its manufacturer is not guaranteed or endorsed by the publisher.
Research integrity at Frontiers
Learn more about the work of our research integrity team to safeguard the quality of each article we publish.