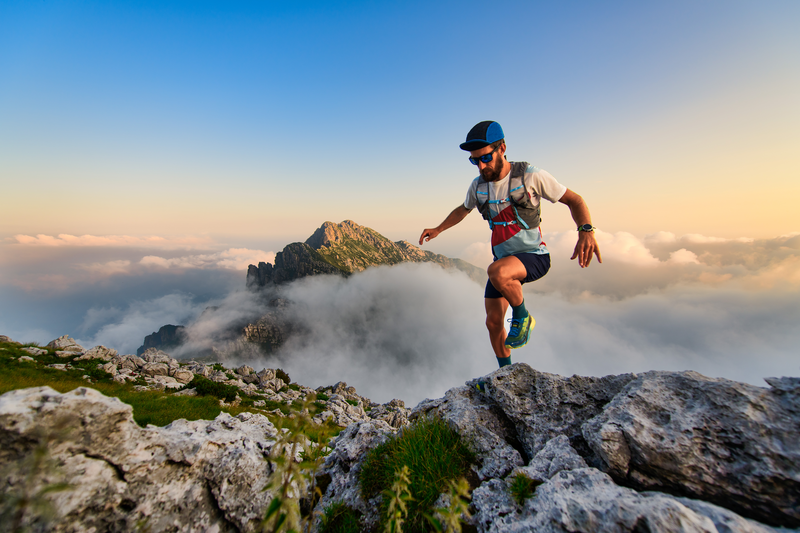
94% of researchers rate our articles as excellent or good
Learn more about the work of our research integrity team to safeguard the quality of each article we publish.
Find out more
ORIGINAL RESEARCH article
Front. Immunol. , 30 January 2025
Sec. Cancer Immunity and Immunotherapy
Volume 16 - 2025 | https://doi.org/10.3389/fimmu.2025.1546167
This article is part of the Research Topic Formation of Immunological Niches in Tumor Microenvironments: Mechanisms and Therapeutic Potential View all 22 articles
Introduction: The impact of distinct primary colorectal cancer (CRC) sites on lung injury and complications remains largely unexplored, despite the palpable differences in surgical positions, procedures, and the resulting mechanically induced respiratory pressures at each site.
Materials and methods: This study employed a forwards-looking approach utilising the propensity score matching (PSM) method; 300 patients with pathological CRC after laparoscopic surgery from April 2019 to May 2023 were enrolled. Two categories were bifurcated based on their surgical locations: the rectosigmoid colon (RSC) group and the descending/ascending colon (DAC) group, with a 2:1 ratio. The occurrence of postoperative pulmonary complications (PPCs) within a 30-day postoperative period was meticulously evaluated. Additionally, assessments have been performed for plasma biomarkers of immune response dynamics and lung injury (plasma soluble advanced glycation end-product receptor [sRAGE], angiopoietin-2 [ANG-2], interleukin-1β/6 [IL-1β/IL-6]) and other parameters.
Results: Although the increase in postoperative lung epithelial damage, as indicated by the plasma sRAGE levels, was significant in the RSC group (DAC vs. RSC; 1029.6 [576.8–1365.2] vs. 1271.6 [896.3–1587.6]; odds ratio=0.999; 95% CI: 0.998 to 1.000; P=0.007), a significantly increased percentage of PPCs was observed in the DAC group (DAC vs. RSC; hazard ratio=1.669; 95% CI, 1.141 to 2.439; P=0.008). A univariate Cox proportional hazards model revealed that sRAGE, ANG-2, IL-1β, and IL-6 levels were not correlated with the incidence of time-to-PPCs across the two cohorts (P>0.05). Propensity score-weighted Cox regression and causal mediation analysis further demonstrated that the DAC site directly affected the incidence of PPCs, regardless of the other baseline confounders and clinical covariates related to the tumour site and PPCs.
Conclusion: The primary site of CRC is an independent predictor of the development of PPCs. Despite the steep Trendelenburg position of the RSC group inciting more pulmonary stress, inflammation and lung epithelial injury, as indicated by higher sRAGE, it demonstrated a lower PPCs occurrence relative to its DAC counterpart, with a slightly inclined or reversed Trendelenburg position. None of the plasma biomarkers of inflammation or lung injury indicated sufficient prognostic value for PPCs.
Postoperative pulmonary complications (PPCs), the second most recurrent complication in general surgical operations (1, 2), are intrinsically associated with perioperative morbidity and mortality (3–5). These complications are particularly predisposed to manifest during major abdominal surgeries (both laparotomy and laparoscopy) (6), with incidence rates ranging from 3–30% (3, 7, 8). This variability in incidence primarily arises from the heterogeneity in the definitions of PPCs (9), surgical/anaesthetic methodologies, and patient populations enrolled. A comprehensive review of the American College of Physicians demonstrated that a composite of pneumonia and respiratory failure defined PPCs in nearly 60% of the 16 preceding publications spanning over a quarter of a century (10). However, the European Perioperative Clinical Outcome (EPCO) consortium proposed that PPCs encompass respiratory failure, acute respiratory distress syndrome (ARDS), aspiration pneumonitis, pneumonia, respiratory infection, atelectasis, pleural effusion, pneumothorax, bronchospasm, and pulmonary embolus (11). Several studies have also incorporated lung injury and the subclinical stage of microatelectasis as PPCs (12–14).
Colorectal cancer (CRC), the most frequently observed type of abdominal tumour, represents the second highest incidence rate of 1,926,118 new cases (9.6% morbidity) and 903,859 deaths (9.3% mortality) globally in 2022 (15). CRC has a diverse global geographical distribution, with the majority of CRCs in Asia manifesting as rectal cancers, constituting more than 50% of the total cases. In contrast, in Europe and North America, rectal cancers represent less than 40% of all (16, 17). Given that the overwhelming majority of CRC patients are treated with laparoscopic procedures, the pneumoperitoneum pressure may amplify the skew in the ventilation−perfusion ratio and the generation of pulmonary atelectasis via enhanced transpulmonary pressure and worsened lung compliance, which can promote lung injury and PPCs even in patients with otherwise healthy lungs (18–20).
Notably, CRC can occur in disparate anatomical locations, including the ascending, transverse, descending and sigmoid colon, and rectum (inclusive of the anus). Thus, the surgical positions utilised in treating CRC are contingent upon the specific site of the disease. Rectosigmoid colectomy (RSC) necessitates a steep Trendelenburg position, whereas ascending and descending colectomy (DAC) merely requires a slightly inclined or reverse Trendelenburg position. Notably, the steep Trendelenburg position may induce substantial diaphragm displacement, haemodynamic compromise, reduced functional residual capacity, augmented pulmonary stress facilitating atelectasis, and compromised oxygenation (21–23). The integration of a large-angle Trendelenburg position along with pneumoperitoneum has also been verified to exacerbate more deterioration of the respiratory system, which can facilitate atelectasis and obstruct oxygenation and related inflammation and lung injury (24). In light of these factors, the primary CRC site, which involves different surgical positions, appears to be a crucial determinant of lung injury and subsequent PPCs incidence.
Despite extensive studies on predictive risk factors for preventing PPCs, including age, surgery type, preoperative spirometry gases, preoperative pulmonary comorbidities, smoking, surgical and anaesthetic strategies (6, 8, 9, 25–29), as well as various predictive risk models (30), research directly exploring how primary CRC sites influence lung injury and whether it correlates with the development of PPCs is limited. This study was designed meticulously to ascertain whether a causal correlation exists between these two variables, utilising propensity score matching to eradicate the bias introduced by other factors.
This retrospective study was rigorously conducted at the Cancer Hospital, Chinese Academy of Medical Sciences (Beijing, China). The experimental protocol was thoroughly reviewed and approved by the institutional ethics committee (Approval No. 22/519-3721). Patients enrolled in the initial clinical trial provided informed written consent before commencing treatment. Both treatment protocols and the collation of clinical data were reserved for future scholarly analysis. Patient follow-up was maintained throughout the patients’ hospital stay and was sustained postdischarge via telecommunication and outpatient records. The inclusion criteria were as follows: (1) patients who underwent radical laparoscopic resection for colorectal cancer; (2) male and female patients aged between 18 and 80 years; (3) American Society of Anaesthesiologists (ASA) physical status ≤ III; (4) body mass index (BMI) < 40 kg/m²; (5) elective laparoscopic colorectal surgery that is expected to last for at least 2 h; and (6) intermediate or high risk of postoperative pulmonary complications (PPCs) as defined by the Assess Respiratory Risk in Surgical Patients in Catalonia (ARISCAT) score (a score of 26–44 is defined as intermediate risk and a score of > 44 is defined as high risk). The exclusion criteria were as follows: (1) aged < 18 years or > 80 years; (2) pregnant or breast-feeding status; (3) ASA physical status of IV or higher; (4) had a history of recent pulmonary infection/prior lung or abdominal surgery; (5) pneumothorax/giant bullae/chronic obstructive pulmonary disease; (6) severe cerebral and cardiovascular dysfunction/chronic renal failure with dialysis/hepatic dysfunction (Child−Pugh grade B or C)/immunologic or neuromuscular disease; (7) emergency or unplanned surgery; (8) expected prolonged mechanical ventilation after surgery; (9) allergies or contraindications to sevoflurane, propofol, or nonsteroidal anti-inflammatory drugs (NSAIDs); (10) participation in other drug trials within 1 preoperative month; and (11) distant metastasis.
A total of 340 patients who underwent laparoscopic procedures at the National Cancer Center between April 2019 and May 2023 and were subsequently pathologically diagnosed with CRC were selected for this study. Comprehensive clinical information, including demographic and baseline data, intraoperative procedures, and follow-up outcomes, was recorded. The patient cohort was segmented into two separate categories according to the surgical position: the rectosigmoid colon group (RSC group), with the steep Trendelenburg position, and the descending/ascending colon group (DAC group), with a slightly inclined or reversed Trendelenburg position. Propensity score matching (PSM) was subsequently applied to achieve equilibrium in clinicopathological features.
Standard clinical practice guided the preoperative preparation and intraoperative monitoring. A lung-protective ventilation approach was utilised for all patients during anaesthesia, maintaining a tidal volume (TV) of 6–8 ml/kg predicted body weight (PBW) (31), accompanied by the same alveolar recruitment manoeuvres (ARMs, every 30 minutes) and positive end-expiratory pressure (PEEP=5 cmH2O).
PPCs were systematically categorised from 1–4 by the criteria delineated by Kroenke et al. (Table 1) (32). The clinical diagnosis of PPCs was substantiated by an independent radiologist utilising a routine low-dose computed tomography (CT) scan of the chest on postoperative day (POD) 3-4. The diagnosis and grading of PPCs were performed independently by a multidisciplinary team comprising medical oncologists, attending surgeons, and intensivists with senior clinical experience. Patients were assessed daily (9:00 to 10:00 a.m.) during the first postoperative week and subsequently weekly from the second to the fourth postoperative week. The plasma soluble forms of the receptor for advanced glycation end products (sRAGE), angiopoietin-2 (ANG-2) and interleukin-1β/6 [IL-1β/IL-6] levels were determined at three time points: preoperatively, on POD 1, and POD 3. In addition, systemic inflammatory markers (interleukin-6, interleukin-1β) and postoperative recovery in the two groups were also analysed for comparison. Furthermore, surgical rating scales (SRS) for the assessment of surgical operating conditions (5 score for optimal; 4 score for good; 3 score for acceptable; 2 score for poor; and 1 score for extremely poor) were administered by experienced surgeons (33), who were blinded to the PnP level to maintain interrater and intrarater agreement.
The process of PSM incorporated factors such as respiratory comorbidity (chronic obstructive pulmonary disease/chronic bronchitis vs. no relevant history), smoking status (either current/former smoker vs. nonsmoker), and age (younger than 60 years vs. 60 years or older), and a 1:2 matching ratio and a calliper of 0.05 were applied via the “MatchIt” R package. The absolute standardised difference (ASD) method, facilitated by the “stddiff” R package, was utilised to juxtapose the characteristics of the two groups and establish a balance, with an ASD value exceeding 0.2 indicating imbalance.
A logistic regression model was used to compare intraoperative procedures and follow-up outcomes between the two groups, and the results are expressed as odds ratios (ORs) and 95% CIs. Kaplan−Meier analysis, along with log-rank tests, was employed to assess the relationship between PPCs incidence and postoperative onset. Univariable or multivariable Cox proportional hazard models were applied to compare the incidence of PPCs within 30 days postoperatively, yielding hazard ratios (HRs) and 95% CIs. The overlap weight-based propensity score weighting method implemented via the R package “WeightIt” was used to eliminate bias by equalizing the distributions of covariates on the basis of the multivariable Cox proportional hazards model.
To determine the valid causal roles of variables of interest in the incidence of PPCs, a causal mediation analysis based on an accelerated failure time (AFT) survival regression model was performed using the R packages “mediation” and “survival”. The primary CRC site was designated as the exposure, the incidence and time of PPCs were the outcomes. A Poisson regression framework was employed to characterise the impact of exposure on postoperative gastrointestinal recovery time (mediator, ordered categorical variable), while age (binary, ≥60 years old and <60 years old) and anaesthetic duration (binary, more than and less than 3 hours), which are associated with PPCs incidence in the univariable Cox proportional hazards model, were included in the analysis as covariates. Additionally, a logistic regression approach was used to analyse the effects of exposure on age (mediator) when postoperative gastrointestinal recovery time and anaesthetic duration were included as covariates. Furthermore, the causal mediation analysis was adjusted for the following baseline and clinical confounders related to the primary CRC site: TNM stage (categorical, Tis-stage I, stage II, and stage III-IV), history of neoadjuvant therapy (binary, yes and no), preoperative haemoglobin levels (continuous), blood product transfusion (binary, yes and no) and duration of surgery (continuous). The average causal mediation effects (ACME) were calculated to measure the mediating impact of gastrointestinal recovery time or age on PPCs incidence. The average direct effects (ADE) were the estimation of exposure on the outcome that mediators cannot explain. The results of the AFT models are described in terms of acceleration regression coefficients, in which a negative coefficient indicates a shorter occurrence time of PPCs.
Quantitative variables were analysed with Student’s t test or the Mann−Whitney U test, whereas categorical data were evaluated via the chi−square test or Fisher’s exact test. A two-sided P value < 0.05 was considered statistically significant. Data analyses were performed using SPSS 25 (IBM SPSS Inc., USA) or R software.
Three hundred forty CRC patients matched the criteria for inclusion in the study database. Following PSM, 300 patients were divided into two groups, comprising 200 patients in the RSC group and 100 patients in the DAC group. The median ages were 63.0 and 61.0 years for the two groups. Most characteristics at baseline were comparable across the two groups, with the exception of a greater prevalence of tumour stage II - IV (RSC vs. DAC; 75.0% [150 of 200] vs. 85.0% [85 of 100], ASD=0.253) and a subsequent lower proportion of neoadjuvant therapy (RSC vs. DAC; 14.5% [29 of 200] vs. 5.0% [5 of 100], ASD=0.324), lower haemoglobin levels (g/L; RSC vs. DAC; 137.5 [127.0-152.8] vs. 130.0 [107.5-144.8], ASD=0.570), an enhanced proportion of anaemia (RSC vs. DAC; 21.0% [42 of 200] vs. 41% [41 of 100], ASD=0.443) and more blood product transfusions (RSC vs. DAC; 5.0% [10 of 200] vs. 15.0% [15 of 100], P=0.003) in the DAC group than in the RSC group (Tables 2, 3). Moreover, the DAC group required extended anaesthetic (min; median [interquartile range, IQR]; RSC vs. DAC; 170.0 (145.0–207.8) vs. 199.5 (162.5–221.8), P<0.001) and surgical duration (RSC vs. DAC; 145.0 (112.0–180.0) vs. 165.0 (132.5–193.0), P=0.002) (Table 3). In a predefined subgroup analysis, there was a notable increase in the duration of anaesthesia from the sigmoid (median, 160.0 min; IQR, 127.3-192.0 min), rectal (median, 170.0 min; IQR, 150.0-225.0 min), and ascending (median, 190.5 min; IQR, 160.0-216.3 min) to the descending colon (median, 212.0 min; IQR, 174.5-235.3 min). Similarly, the surgical duration showed a trend comparable to that of the sigmoid (median, 126.5 min; IQR, 100.0–152.5 min), rectal (median, 150.0 min; IQR, 131.0–200.0 min), ascending (median, 163.5 min; IQR, 131.5–187.0 min), and descending colon (median, 175.0 min; IQR, 149.3–208.8 min).
In addition, the Mann−Whitney rank test revealed that the surgical conditions, as evaluated by the surgical rating scale (SRS), were poorer in the DAC group than in the RSC group (RSC vs. DAC; 5 [5.0–5.0] vs. 5.0 [4.0–5.0]; P=0.003, Table 3). Details of the mechanical parameters of pulmonary compliance and peak and plateau airway pressures at different surgical positions at the tumour sites are provided in Table 4.
First, among the indicators related to postoperative recovery, there was no significant difference in postanaesthesia care unit (PACU) recovery time or postoperative hospitalisation days between the DAC and RSC groups. However, a greater protracted gastrointestinal recovery time (defined as the period of first flatus or stool through the anus after surgery) was observed in the DAC group (DAC vs. RSC; 3.0 [2.0–3.0] vs. 3.0 [2.0–3.0]; OR=1.698; 95% CI, 1.098 to 2.684; P=0.020; Table 5). Moreover, the gastrointestinal recovery time was longer in patients with PPCs than in those without (with PPCs vs. without PPCs; 3.0 [2.0–3.0] vs. 2.5 [2.0–3.0]; OR=2.114; 95% CI, 1.361 to 3.362; P=0.001).
Next, the plasma levels of sRAGE on POD1 were greater in the patients in the RSC group than in those in the DAC group (DAC vs. RSC; 1029.6 [576.8–1365.2] vs. 1271.6 [896.3–1587.6]; OR=0.999; 95% CI, 0.998 to 1.000; P=0.007), which also indicated marginal significance for the two groups on POD3 (DAC vs. RSC; 881.1 [510.4–1060.4] vs. 1063.3 [618.4–1335.4]; OR=0.999; 95% CI, 0.998 to 1.000; P=0.077; Table 5). However, the levels of the endothelial injury indicator ANG-2 and the systemic inflammatory indicators interleukin-1β (IL-1β)/IL-6 remained unchanged across the two cohorts (P>0.05, Table 5). In addition, no significant relationship was detected between the plasma biomarkers sRAGE, ANG-2, IL-1β, or IL-6 and the PPCs incidence (P>0.05).
Furthermore, an unexpected finding was that the DAC group exhibited a significantly greater occurrence of PPCs within a span of 30 days postsurgery. The hazard ratio (HR) was 1.669 (95% CI, 1.141 to 2.439; P=0.008; Table 5), with a 48% incidence (48/100) in the DAC group and a 30.0% incidence (60/200) in the RSC group (Figure 1A). Moreover, the DAC group also had a significantly greater incidence of atelectasis (P=0.002) and pleural effusion (P=0.007; Table 5). Notably, in a preplanned subgroup analysis, a significant increase in the incidence of PPCs in the sigmoid colon, rectum, ascending colon, and descending colon was detected (HR=1.218; 95% CI, 1.019 to 1.457; P=0.031; Figure 1B). Among the observed incidents, microatelectasis was the most prevalent, occurring in 21.7% (65 out of 300) of the cases.
Figure 1. Curves representing the incidence of postoperative time-to-PPCs within a 30-day postoperative period (univariate Cox proportional hazards regression analysis). (A) Incidence of PPCs in the descending/ascending colon (DAC) group and the rectosigmoid colon (RSC) group. (B) Incidence of PPCs in subgroups of various colorectal positions. DC, descending colon; AC, ascending colon; SC, sigmoid colon; RC, rectum of the colon.
The univariable Cox proportional hazards model identified four PPCs-related factors with statistical significance. These factors included an anaesthetic duration exceeding 3 hours (HR=1.508; 95% CI, 1.033 to 2.202; P=0.033), an age of 60 years or more (HR=1.708; 95% CI, 1.126 to 2.593; P=0.012), a primary CRC site in the DAC (HR=1.669; 95% CI, 1.141 to 2.439; P=0.008) and a gastrointestinal recovery time (HR=1.775; 95% CI, 1.219 to 2.584; P=0.003). The time-to-PPCs were subsequently analysed using these high-risk factors through a multivariable Cox proportional hazards regression model. The findings revealed that gastrointestinal recovery time (HR=1.750; 95% CI, 1.198 to 2.555; P=0.004), age (60 years and above vs. under 60 years, HR=1.678; 95% CI, 1.103 to 2.552; P=0.016) and primary CRC site (DAC vs. RSC; HR=1.470; 95% CI, 0.9847 to 2.194; P=0.059) were significant or marginal independent risk factors for PPCs incidence. To acquire unbiased estimates of the influence of these factors on PPCs, the adjusted HRs of the primary CRC site, age and gastrointestinal recovery time were 1.634 (95% CI, 1.115 to 2.396; P=0.012), 1.745 (95% CI, 1.131 to 2.692; P=0.012) and 1.750 (95% CI, 1.159 to 2.644; P=0.008), respectively, according to propensity score weighting via overlap weights. In summary, even when adjustments were made in populations with significant differences in other covariates, such as age and gastrointestinal recovery time, the primary CRC site emerged as a stand-alone risk factor for PPCs.
The aforementioned analyses demonstrated that the primary CRC site was associated with several baseline and clinical characteristics and PPCs incidence. To address whether the primary CRC site is impacted by other clinical characteristics and, in turn, influences the incidence of PPCs, causal mediation analysis using the AFT survival regression model with the quasi-Bayesian Monte Carlo method was performed (Figure 2A). First, when the gastrointestinal recovery time was designated as a mediator, the primary CRC site had a significant average direct effect (ADE; effect=-1.769 [95% CI, -3.742 to -0.49]; P=0.004) and total effect (effect=-2.072 [95% CI, -4.380 to 0.54]; P=0.004) on increasing the incidence of PPCs, whereas the average causal mediation effect (ACME) was not significant (effect=-0.303 [95% CI, -1.512 to 0.58]; P=0.474; Figure 2B). Even after adjusting for the other confounders associated with the primary CRC site, the mediation effect estimates did not significantly change (Figure 2B). When age was considered a mediator, the ACME, ADE, and total effects were 0.063 (95% CI, -0.164 to 0.34; P=0.596), -1.228 (95% CI, -2.218 to -0.37; P=0.002) and -1.165 (95% CI, -2.125 to -0.29; P=0.004), respectively (Figure 2C). Similarly, the tendency was not significantly altered after adjusting for confounders (Figure 2C). Therefore, compared with those in the RSC group, the occurrence of tumours in the descending/ascending colon (DAC group) directly resulted in an increased incidence of PPCs, and other clinical characteristics, such as the gastrointestinal recovery time and age of patients, did not mediate this effect.
Figure 2. Causal mediation analysis of the primary CRC site (exposure), gastrointestinal recovery time (mediator 1), age (mediator 2) and incidence of PPCs (outcome). (A) Directed acyclic graph of the mediation model. The measured covariates are included as fixed effects in each model, in the absence or presence of the confounders that are associated with the primary CRC site. (B) Mediation analysis of the models with mediator 1 (gastrointestinal recovery time) in the absence or presence of confounders. (C) Mediation analysis of the models with mediator 2 (age) in the absence or presence of confounders.
In the laparoscopic procedure for CRC, anterior resection of rectal cancer and sigmoidectomy necessitate the sustained steep Trendelenburg position, where the patient’s head is positioned lower than the feet at an angle of approximately 25–30°. In contrast, ascending and descending colectomies require intermittent transformations of the Trendelenburg position of ±10–15°. Research has indicated that rapid atelectasis involvement can develop in lung areas with high pressure within 10 minutes in the Trendelenburg position (23). The significant discrepancy in peak and plateau airway pressures and pulmonary compliance between these two surgical positions (Table 4) may precipitate various pulmonary stresses and lung injuries (21–23, 29). This process is further complicated by carbon dioxide pneumoperitoneum, which exacerbates atelectasis, inflammation and lung injury via hypercapnia and respiratory acidosis. Consequently, the airway pressure (barotrauma) resulting from laparoscopy may surpass the elastic limits of the lung parenchyma, generating transpulmonary pressures that damage the alveolar epithelium (34, 35). Our results in Table 4 show that patients in the Trendelenburg position (25–30°) had higher peak airway pressure (Ppeak) and plateau airway pressure (Pplat) and lower pulmonary compliance than patients in the reverse Trendelenburg position did (P<0.001, Table 4). The abdominal contents move upwards towards the diaphragm in the Trendelenburg position, increasing intrathoracic cavity pressure and airway pressure and decreasing lung compliance. Once a threshold is overcome, excessive airway pressure and lung strain deform epithelial and endothelial cells (known as barotrauma), resulting in secondary stress fatigue, microcapillary rupture and inflammation (35, 36). Inflammation triggered by damage can be perpetuated by the activation of various signalling pathways, resulting in further biotrauma to the lung. Hence, patients with worse dynamic ventilation parameters can suffer worse lung injuries.
To characterise the magnitude of intraoperative general and pulmonary inflammation and lung injury, our research applied several biological biomarkers. Receptors for advanced glycation end products (RAGE), especially sRAGE, are widely studied as indicators of pulmonary injury because of their high expression in type I pneumocytes (37–39). To the best of our knowledge, plasma sRAGE is the only biomarker that has been correlated with a functional readout of epithelial barrier function and alveolar fluid clearance (40, 41). sRAGE has been extensively implicated in the process of inflammation and lung injury to alveolar epithelial cells (42, 43), particularly in the context of mechanical high tidal volume-induced alveolar overdistension or acute respiratory distress syndrome (ARDS) (44, 45). Previous study demonstrated that sRAGE is strongly linked to the duration of ventilation for POD 1, with a 25% multiplicative increase in hours of ventilation (95% CI, 2% to 52%, P = 0.03) observed for every 50 pg/ml increase in sRAGE (46). In addition to sRAGE, another major form of RAGE is anchored to the cell membrane (mRAGE) (39, 47). mRAGE is capable of binding proinflammatory ligands in the immune microenvironment, such as AGEs, S100 proteins and high mobility group box 1 (HMGB1), and consequently activates downstream inflammatory pathways, such as nuclear factor kappa-light-chain enhancer of activated B cells (NF-κB) and signal transducer and activator of transcription 3 (STAT3). Stimulation of these pathways can lead to cytokine release, cell migration and upregulated RAGE expression, which in turn perpetuates the inflammatory cascade, resulting in the occurrence and progression of many pathological processes and diseases, including airway inflammation and acute lung injury (ALI) (48). Moreover, the extracellular part of mRAGE is cleaved from the membrane upon lung injury and becomes sRAGE, which can be detected in blood and bronchial lavage fluid. sRAGE functions as a “decoy”, as it attaches to ligands of RAGE without activating intracellular pathways and therefore prevents excessive cell injury and airway inflammation by decreasing the expression and release of downstream cytokines, such as tumour necrosis factor-alpha (TNF-α), IL-1β and IL-6 (47, 49). The interactions between RAGE and certain ligands and sequential pathways constitute the RAGE axis. Since the RAGE axis effectively modulates inflammation, several benign and malignant diseases that are strongly associated with the immune microenvironment can be affected by the function of RAGE (50). The extant literature provides substantial support for the proposition that endogenous plasma sRAGE plays a mechanistic role in the pathophysiology of ARDS in models of lung injury that involve acid and hyperoxia (37, 51, 52). Additionally, existing studies further corroborate the pivotal function of endogenous plasma sRAGE within the AGE-RAGE axis as a prospective catalyst of lung injury, indicating that there is potential for reducing plasma sRAGE levels or targeting the AGER/sRAGE axis to have a preventative or therapeutic effect on inflammation-related lung disease (53–55). Notably, sRAGE is also regarded as a promising biomarker for colon carcinoma. Aglago et al. reported an inverse association between prediagnostic sRAGE levels and colorectal risk in men (56).
ANG-2 is another potential candidate for endothelial inflammation and injury caused primarily by ARDS and sepsis (57, 58). ANG-2 originates primarily from endothelial cells and acts as a key factor in the modulation of vascular permeability as an antagonist against ANG-1, which promotes endothelial permeability by preserving cellular junctions and signalling the reduction in certain surface-adhesion molecules through tyrosine kinases with immunoglobulin-like and EGF-like domain 2 (Tie-2) signalling pathways (59). As the highest Tie-2 expression in adult tissues is observed in the lung, disruption of the pathway caused by ANG-2 can lead to pulmonary disorders (60). Environmental factors related to pathogenesis, such as hypoxia and increased levels of cytokines, can lead to the production and release of ANG-2 (61), and existing studies have shown that ANG-2 is an essential biomarker of ALI/ARDS (62, 63). Moreover, ANG-2 has been shown to enhance tumour metastasis and immunosuppression by regulating cytokine levels in the tumour microenvironment, suggesting that ANG-2 is a potential target for cancer treatment therapy (64, 65). ANG-2 and vascular endothelial growth factor (VEGF) may promote the formation of new blood vessels through a synergistic effect, leading to liver metastasis of colorectal cancer (66). ANG-2-targeted comprehensive treatment has sparked new ideas in cancer treatment (67, 68).
Furthermore, IL-1β and IL-6 are major proinflammatory cytokines released by diverse immune and nonimmune cells upon stimulation, such as infection, injury, and stress. IL-1β originates primarily from macrophages, dendritic cells, and neutrophils, although it can also be secreted by epithelial cells and fibroblasts. Canonically, it is synthesised as an inactive precursor, which is cleaved and activated by caspase-1 upon environmental stimulation (69). However, the production of IL-6 is initiated by pathogen-associated molecular patterns (PAMPs) and damage-associated molecular patterns (DAMPs) (70). Various types of cells, including T cells, macrophages, and fibroblasts, are involved in the synthesis and secretion of IL-6. Both cytokines are essential for adjusting the immune microenvironment by triggering the activation of various immune cells, stimulating the differentiation of T helper cells, and contributing to the synthesis and secretion of acute-phase reactants in the liver. Owing to their pivotal roles in inflammation, IL-1β and IL-6 have been proposed as biomarkers of lung injury and airway inflammation, particularly in diseases such as chronic obstructive pulmonary disease (COPD), ALI and ARDS (62). In addition, during general anaesthesia, the expression and release of these cytokines can be stimulated by lung impairment caused by mechanical ventilation and the activation of pathways related to RAGE (47). The presence of these cytokines serves as indicators of ongoing immune activation and the extent of airway remodelling, making them useful for monitoring the magnitude and progression of lung injuries.
Inflammation has been demonstrated to play a critical role in the pathogenesis of PPCs following noncardiac surgery (46). Nevertheless, no correlation between the above inflammatory indicators and the incidence of PPCs was found by the analysis of the univariate Cox proportional hazards model in this study. This result is similar to those of many investigations. Aaron et al. identified sRAGE as a potential indicator of acute lung injury, with no significant correlation with PPCs (46); Neto et al. identified none of the plasma biomarkers expressed for inflammation or lung injury (TNF-α, IL-6 and IL-8; sRAGE, surfactant protein [SP]-D, Clara cell protein [CC]-16 and Krebs von den Lungen 6 [KL6]) is related to PPCs (71); Jabaudon et al. compared the sRAGE levels between patients who received protective ventilation and those who did not and concluded that patients with at least one hypoxemia episode presented elevated sRAGE levels during mechanical ventilation, but no association was observed between intraoperative changes in sRAGE and PPCs within 30 days postsurgery (72). The ambiguity of the correlation between plasma sRAGE and PPCs may be ascribed to the inflammatory consumption of sRAGE, as it works by attaching to ligands. Recent studies have demonstrated that postoperative sRAGE rapidly decreases to or below preoperative levels within 24 hours following cardiac surgery. Consequently, it is plausible that by the time samples were collected on POD 1, sRAGE values may have already returned to normal (46). Additionally, as the heterogeneity of patients and definitions of PPCs vary, not all lung injuries ultimately lead to PPCs.
Notably, patients who undergo proctosigmoidectomy (RSC group) with more pulmonary stress and injury are theoretically more susceptible to PPCs incidence. Our findings revealed a consistent correlation between the RSC group with the steep Trendelenburg position and lung epithelium injury, with higher sRAGE levels in the RSC group on the postoperative day (POD, P=0.007 for POD 1 and P=0.077 for POD 3 [marginal significance]; Table 5). This may be attributed to the greater degree of lung injury in patients in the RSC group suffered from steeper Trendelenburg position than that in the counterpart, as we expected. However, contrary to expectations, univariate and multivariate Cox regression analyses revealed a negative correlation between the primary CRC site and PPCs, with a significantly decreased incidence of PPCs in the RSC group (DAC vs. RSC; HR=1.669; 95% CI, 1.141 to 2.439; P=0.008). Our propensity score weighting method using overlap weights in a Cox proportional hazards regression analysis demonstrated that tumours occurring in the descending/ascending colon (DAC group) indeed increased the risk of PPCs (adjusted HR=1.634; 95% CI, 1.115 to 2.396; P=0.012). The subsequent causal mediation analysis demonstrated that this factor remains a direct effect on the incidence of PPCs when adjusted for several baseline confounders and clinical covariates related to the primary CRC site and PPCs.
The causes of DAC for the high incidence of PPCs are worthy of attention. Postoperative gastrointestinal recovery time, age and anaesthetic duration exceeding 3 hours were also unfavourable factors for the incidence of PPCs according to the univariate Cox proportional hazards model. It is well known that enhanced recovery is essential for reducing postoperative complications (73), especially for PPCs (28, 74). The literature indicates that right hemicolectomy is associated with inferior intestinal function and an elevated prevalence of metabolic syndrome due to factors such as bile acid malabsorption, impaired water reabsorption, small intestinal bacterial overgrowth, and gut microbial dysbiosis, which potentially deteriorates gastrointestinal recovery and facilitates inflammation and PPCs (75–77). In the present study, a greater gastrointestinal recovery time was indeed observed in the DAC group (P=0.020, Table 5), and patients with PPCs exhibited longer gastrointestinal recovery times (patients with PPCs vs. those without PPCs; 3.0 [2.0–3.0] vs. 2.5 [2.0–3.0]; OR=2.114; 95% CI, 1.361 to 3.362; P=0.001). Additionally, age serves as a robust marker for PPCs, even in healthy older patients at elevated risk of PPCs (8, 28). Previous studies have indicated that age over 60 years or 65 years is a risk factor for PPCs development (8, 78). Stratification analysis suggested that the risk of PPCs significantly increased with age, which may be related to the frailty of elderly patients with weakened respiratory muscle function (10, 27, 79). In terms of duration, the literature substantiates that a surgical or anaesthesia duration surpassing two hours independently contributes to PPCs, revealing a significant association between operative duration and PPCs. This association has odds ratios (and confidence intervals) of 4.9 (2.4 to 10.1) and 9.7 (4.7 to 19.9) for operative periods exceeding 2 and 3 hours, respectively (9, 27). Here, we identified an obvious correlation between primary tumour location and surgical/anaesthesia duration, as shown in Table 3 (P<0.001 for anaesthetic duration, P=0.002 for surgical duration). For subgroup analysis, the median anaesthetic durations for the sigmoid (160.0 min), rectal (170.0 min), ascending (190.5 min) and descending (212.0 min) colon regions also showed apparent relevance to the corresponding time-to-PPCs incidence (P=0.031, Figure 1B).
In addition, three other potential explanations were also considered for the high prevalence of PPCs in the DAC group. First, complex surgical interventions inherently involve a greater degree of trauma and an extended period of anaesthesia and surgery, which consequently fosters an environment conducive to the development of PPCs. Compared with sigmoidectomy, ascending and descending colectomies entail intricate vascular trajectories, neighbouring organ interactions, and complex intestinal anastomosis techniques. Moreover, in comparison with the pelvic support surrounding the rectosigmoid colon, the absence of bony support in the DAC group further constrained the surgical field, thereby increasing procedural difficulty and correlating with a lower SRS score for evaluating surgical conditions in laparoscopic procedures in the presence of identical degrees of neuromuscular blockade (Table 3). Consequently, our study revealed that the surgical and anaesthetic times required for ascending and descending colectomy procedures are longer than those for rectosigmoidectomy. As reported in the literature, the incidence of PPCs is notably elevated in patients receiving extended anaesthesia and surgical procedures (8, 27, 78). Second, the surgical incision pathways for different CRC segments notably influence the development of PPCs (28). The ascending and descending colectomies typically necessitate paramedian or transrectal incisions (Figure 3); as a consequence, structures such as the anterior branches of the intercostal nerve, the rectus sheath (both anterior and posterior), and the rectus abdominis muscle are affected. In conjunction with compromised abdominal respiration, this impairment of rectus abdominis muscle function may accelerate the development of concomitant atelectasis and pneumonia. In contrast, procedures within the RSC group generally require a midline incision of the linea alba, exerting less impact on abdominal autonomy and breathing. Finally, as previously indicated, preoperative anaemia is an independent risk factor for PPCs (25, 27, 80). Likewise, relevant transfusions also promote the onset of this complication (81–83). Owing to the clinical characteristics of patients with DAC symptoms appearing later than those with RSC symptoms, anaemia (ASD=0.443) and transfusion (P=0.003) constitute a substantial proportion of patients with DAC symptoms (Tables 2, 3); hence, anaemia is also a major cause of this postoperative complication.
Notably, cancer and inflammation are mutually reinforcing factors. CRC has been reported to induce inflammation through several mechanisms, with the expression of IL-1 being a mediator. Moreover, once the growth of a tumour exceeds its blood and nutrient supply, necrotic cell death in the tumour results in the release of various DAMPs, including IL-1 and HMGB1, both of which are related to lung inflammation (84). On the other hand, the immune microenvironment in the lung parenchyma can be altered by these DAMPs and establish a premetastatic niche for CRC (85). In summary, CRC patients may be at increased risk of developing lung injuries and PPCs because tumours elicit inflammation, and inflammation in the lungs promotes the metastasis of CRC.
Our investigation is subject to certain limitations. We endeavoured to curtail potential imbalances and limit the influence of clinical characteristics on PPCs by performing PSM for factors such as respiratory comorbidities, smoking status, and age. However, given the inherent peculiarities of these clinical variables in demographic and baseline data, achieving absolute balance remains an unrealistic expectation. Furthermore, although this study is based on the hypothesis that the steep Trendelenburg position induces more lung stress in the pathogenesis of pulmonary injury and PPCs, maintaining a sustained surgical position at one angle is impractical clinically because of the variety of surgical procedures performed, not even with prospective randomised controlled trials. Additionally, as a single-centre study with a population limited to a high-resource cancer centre, the generalisability of the results to other settings remains limited; hence, a larger sample size is needed. Despite these limitations, this study signifies progress in assessing the correlation between primary CRC sites, lung injury and the incidence of PPCs in patients who have received colorectal laparoscopy. Subsequent research could explore the impact of disparate surgical positions on the dynamic distribution of pulmonary ventilation, such as leveraging electrical impedance tomography (EIT) imaging. This approach could further elucidate the correlation between inclined surgical position, the distinct ventral and dorsal redistribution of tidal ventilation, and PPCs.
In summary, CRC patients display diverse surgical positions, procedures, and postoperative recovery patterns (75–77). Although the rectosigmoid colon with the Trendelenburg position in laparoscopy triggers epithelial lung injury, as indicated by elevated postoperative sRAGE levels, a lower PPCs incidence was unexpectedly observed than in those with tumours in the descending/ascending colon. Subsequent analyses confirmed that the primary CRC site was an independent risk factor influencing the onset of PPCs. The other baseline, clinical, and postoperative recovery characteristics cannot explain this effect. Therefore, not the Trendelenburg position and the resulting lung injury but rather the primary tumour site occurring in the descending/ascending colon directly affect the incidence of PPCs.
The datasets contain identifiable contents of patients. The datasets studied in this research can be obtained from the corresponding authors upon reasonable request. Requests to access these datasets should be directed to TY, YmxpenphcmR5dEAxNjMuY29t.
The studies involving humans were approved by National Cancer Center/Cancer Hospital, Chinese Academy of Medical Sciences and Peking Union Medical College, National GCP Center for Anticancer Drugs, The Independent Ethics Committee. The studies were conducted in accordance with the local legislation and institutional requirements. The human samples used in this study were acquired from primarily isolated as part of our previous study for which ethical approval was obtained. Written informed consent for participation was not required from the participants or the participants’ legal guardians/next of kin in accordance with the national legislation and institutional requirements.
H-XL: Data curation, Formal analysis, Funding acquisition, Methodology, Writing – original draft, Writing – review & editing. LC: Data curation, Formal analysis, Methodology, Writing – review & editing. YL: Data curation, Formal analysis, Methodology, Writing – review & editing. T-HW: Writing – review & editing, Data curation, Formal analysis, Methodology. F-DM: Data curation, Formal analysis, Methodology, Writing – review & editing. LX: Investigation, Software, Validation, Writing – review & editing. MW: Investigation, Software, Validation, Writing – review & editing. Z-XZ: Investigation, Software, Validation, Writing – review & editing. S-NQ: Investigation, Software, Validation, Writing – review & editing. FW: Resources, Software, Visualization, Writing – review & editing. WT: Investigation, Software, Validation, Writing – review & editing. S-JW: Investigation, Software, Validation, Writing – review & editing. Y-LS: Conceptualization, Funding acquisition, Project administration, Resources, Software, Supervision, Visualization, Writing – original draft, Writing – review & editing. HZ: Conceptualization, Project administration, Supervision, Writing – review & editing. TY: Conceptualization, Funding acquisition, Project administration, Supervision, Writing – original draft, Writing – review & editing.
The author(s) declare financial support was received for the research, authorship, and/or publication of this article. Support for this work was received from the CAMS Innovation Fund for Medical Sciences (CIFMS, 2022-I2M-C&T-B-061 and 2021-I2M-1-066), the Beijing Hope Run Special Fund for the Cancer Foundation of China (LC2021A18, LC2022B06), and the National Natural Science Foundation of China (82073327).
The authors declare that the research was conducted in the absence of any commercial or financial relationships that could be construed as a potential conflict of interest.
The author(s) declare that no Generative AI was used in the creation of this manuscript.
All claims expressed in this article are solely those of the authors and do not necessarily represent those of their affiliated organizations, or those of the publisher, the editors and the reviewers. Any product that may be evaluated in this article, or claim that may be made by its manufacturer, is not guaranteed or endorsed by the publisher.
1. Ladha K, Vidal Melo MF, McLean DJ, Wanderer JP, Grabitz SD, Kurth T, et al. Intraoperative protective mechanical ventilation and risk of postoperative respiratory complications: hospital based registry study. Bmj. (2015) 351:h3646. doi: 10.1136/bmj.h3646
2. Le LM, Chaiyakunapruk N. Urgent need to take action on reducing postoperative respiratory complications. Lancet Reg Health West Pac. (2021) 10:100136. doi: 10.1016/j.lanwpc.2021.100136
3. Abbott TEF, Fowler AJ, Pelosi P, Gama de Abreu M, Moller AM, Canet J, et al. A systematic review and consensus definitions for standardised end-points in perioperative medicine: pulmonary complications. Br J Anaesth. (2018) 120:1066–79. doi: 10.1016/j.bja.2018.02.007
4. Serpa Neto A, Hemmes SN, Barbas CS, Beiderlinden M, Fernandez-Bustamante A, Futier E, et al. Incidence of mortality and morbidity related to postoperative lung injury in patients who have undergone abdominal or thoracic surgery: a systematic review and meta-analysis. Lancet Respir Med. (2014) 2:1007–15. doi: 10.1016/S2213-2600(14)70228-0
5. Schuijt MTU, Hol L, Nijbroek SG, Ahuja S, van Meenen D, Mazzinari G, et al. Associations of dynamic driving pressure and mechanical power with postoperative pulmonary complications-posthoc analysis of two randomised clinical trials in open abdominal surgery. EClinicalMedicine. (2022) 47:101397. doi: 10.1016/j.eclinm.2022.101397
6. Ferrando C, Soro M, Unzueta C, Suarez-Sipmann F, Canet J, Librero J, et al. Individualised perioperative open-lung approach versus standard protective ventilation in abdominal surgery (iPROVE): a randomised controlled trial. Lancet Respir Med. (2018) 6:193–203. doi: 10.1016/S2213-2600(18)30024-9
7. Kirmeier E, Eriksson LI, Lewald H, Jonsson Fagerlund M, Hoeft A, Hollmann M, et al. Post-anaesthesia pulmonary complications after use of muscle relaxants (POPULAR): a multicentre, prospective observational study. Lancet Respir Med. (2019) 7:129–40. doi: 10.1016/S2213-2600(18)30294-7
8. Miskovic A, Lumb AB. Postoperative pulmonary complications. Br J Anaesth. (2017) 118:317–34. doi: 10.1093/bja/aex002
9. Güldner A, Kiss T, Serpa Neto A, Hemmes SN, Canet J, Spieth PM, et al. Intraoperative protective mechanical ventilation for prevention of postoperative pulmonary complications: a comprehensive review of the role of tidal volume, positive end-expiratory pressure, and lung recruitment maneuvers. Anesthesiology. (2015) 123:692–713. doi: 10.1097/ALN.0000000000000754
10. Smetana GW, Lawrence VA, Cornell JE, American College of P. Preoperative pulmonary risk stratification for noncardiothoracic surgery: systematic review for the American College of Physicians. Ann Intern Med. (2006) 144:581–95. doi: 10.7326/0003-4819-144-8-200604180-00009
11. Jammer I, Wickboldt N, Sander M, Smith A, Schultz MJ, Pelosi P, et al. Standards for definitions and use of outcome measures for clinical effectiveness research in perioperative medicine: European Perioperative Clinical Outcome (EPCO) definitions: a statement from the ESA-ESICM joint taskforce on perioperative outcome measures. Eur J Anaesthesiol. (2015) 32:88–105. doi: 10.1097/EJA.0000000000000118
12. Hulzebos EH, Helders PJ, Favié NJ, De Bie RA, Brutel de la Riviere A, Van Meeteren NL. Preoperative intensive inspiratory muscle training to prevent postoperative pulmonary complications in high-risk patients undergoing CABG surgery: a randomized clinical trial. Jama. (2006) 296:1851–7. doi: 10.1001/jama.296.15.1851
13. Huang D, Zhou S, Yu Z, Chen J, Xie H. Lung protective ventilation strategy to reduce postoperative pulmonary complications (PPCs) in patients undergoing robot-assisted laparoscopic radical cystectomy for bladder cancer: A randomized double blinded clinical trial. J Clin Anesth. (2021) 71:110156. doi: 10.1016/j.jclinane.2020.110156
14. Neto AS, Hemmes SN, Barbas CS, Beiderlinden M, Fernandez-Bustamante A, Futier E, et al. Association between driving pressure and development of postoperative pulmonary complications in patients undergoing mechanical ventilation for general anaesthesia: a meta-analysis of individual patient data. Lancet Respir Med. (2016) 4:272–80. doi: 10.1016/S2213-2600(16)00057-6
15. Bray F, Laversanne M, Sung H, Ferlay J, Siegel RL, Soerjomataram I, et al. Global cancer statistics 2022: GLOBOCAN estimates of incidence and mortality worldwide for 36 cancers in 185 countries. CA Cancer J Clin. (2024) 74:229–63. doi: 10.3322/caac.21834
16. Yang Y, Wang HY, Chen YK, Chen JJ, Song C, Gu J. Current status of surgical treatment of rectal cancer in China. Chin Med J (Engl). (2020) 133:2703–11. doi: 10.1097/CM9.0000000000001076
17. Devesa SS, Chow WH. Variation in colorectal cancer incidence in the United States by subsite of origin. Cancer. (1993) 71:3819–26. doi: 10.1002/1097-0142(19930615)71:12<3819::AID-CNCR2820711206>3.0.CO;2-L
18. Nunn JF, Payne JP. Hypoxaemia after general anaesthesia. Lancet. (1962) 2:631–2. doi: 10.1016/S0140-6736(62)92541-2
19. Bendixen HH, Hedley-Whyte J, Laver MB. Impaired oxygenation in surgical patients during general anesthesia with controlled ventilation. A concept of atelectasis. N Engl J Med. (1963) 269:991–6. doi: 10.1056/NEJM196311072691901
20. Wang Y, Wang H, Wang H, Zhao X, Li S, Chen L. Exploring the intraoperative lung protective ventilation of different positive end-expiratory pressure levels during abdominal laparoscopic surgery with Trendelenburg position. Ann Transl Med. (2019) 7:171. doi: 10.21037/atm.2019.03.45
21. Yu J, Park JY, Kim DH, Kim S, Hwang JH, Seo H, et al. Incidence and Risk Factors of Pulmonary Complications after Robot-Assisted Laparoscopic Prostatectomy: A Retrospective Observational Analysis of 2208 Patients at a Large Single Center. J Clin Med. (2019) 8:1509. doi: 10.3390/jcm8101509
22. Awad H, Walker CM, Shaikh M, Dimitrova GT, Abaza R, O'Hara J. Anesthetic considerations for robotic prostatectomy: a review of the literature. J Clin Anesth. (2012) 24:494–504. doi: 10.1016/j.jclinane.2012.03.003
23. Girrbach F, Petroff D, Schulz S, Hempel G, Lange M, Klotz C, et al. Individualised positive end-expiratory pressure guided by electrical impedance tomography for robot-assisted laparoscopic radical prostatectomy: a prospective, randomised controlled clinical trial. Br J Anaesth. (2020) 125:373–82. doi: 10.1016/j.bja.2020.05.041
24. Andersson LE, Baath M, Thorne A, Aspelin P, Odeberg-Wernerman S. Effect of carbon dioxide pneumoperitoneum on development of atelectasis during anesthesia, examined by spiral computed tomography. Anesthesiology. (2005) 102:293–9. doi: 10.1097/00000542-200502000-00009
25. Mazo V, Sabaté S, Canet J, Gallart L, de Abreu MG, Belda J, et al. Prospective external validation of a predictive score for postoperative pulmonary complications. Anesthesiology. (2014) 121:219–31. doi: 10.1097/ALN.0000000000000334
26. Brooks-Brunn JA. Predictors of postoperative pulmonary complications following abdominal surgery. Chest. (1997) 111:564–71. doi: 10.1378/chest.111.3.564
27. Canet J, Gallart L, Gomar C, Paluzie G, Valles J, Castillo J, et al. Prediction of postoperative pulmonary complications in a population-based surgical cohort. Anesthesiology. (2010) 113:1338–50. doi: 10.1097/ALN.0b013e3181fc6e0a
28. Odor PM, Bampoe S, Gilhooly D, Creagh-Brown B, Moonesinghe SR. Perioperative interventions for prevention of postoperative pulmonary complications: systematic review and meta-analysis. Bmj. (2020) 368:m540. doi: 10.1136/bmj.m540
29. Grieco DL, Russo A, Anzellotti GM, Romano B, Bongiovanni F, Dell'Anna AM, et al. Lung-protective ventilation during Trendelenburg pneumoperitoneum surgery: A randomized clinical trial. J Clin Anesth. (2023) 85:111037. doi: 10.1016/j.jclinane.2022.111037
30. Collaborative ST, Collaborative T. Evaluation of prognostic risk models for postoperative pulmonary complications in adult patients undergoing major abdominal surgery: a systematic review and international external validation cohort study. Lancet Digit Health. (2022) 4:e520–31. doi: 10.1016/S2589-7500(22)00069-3
31. Linares-Perdomo O, East TD, Brower R, Morris AH. Standardizing predicted body weight equations for mechanical ventilation tidal volume settings. Chest. (2015) 148:73–8. doi: 10.1378/chest.14-2843
32. Kroenke K, Lawrence VA, Theroux JF, Tuley MR. Operative risk in patients with severe obstructive pulmonary disease. Arch Internal Med. (1992) 152:967–71. doi: 10.1001/archinte.1992.00400170057011
33. Boon M, Martini CH, Aarts L, Dahan A. The use of surgical rating scales for the evaluation of surgical working conditions during laparoscopic surgery: a scoping review. Surg Endosc. (2019) 33:19–25. doi: 10.1007/s00464-018-6424-5
34. Chiumello D, Carlesso E, Cadringher P, Caironi P, Valenza F, Polli F, et al. Lung stress and strain during mechanical ventilation for acute respiratory distress syndrome. Am J Respir Crit Care Med. (2008) 178:346–55. doi: 10.1164/rccm.200710-1589OC
35. Protti A, Cressoni M, Santini A, Langer T, Mietto C, Febres D, et al. Lung stress and strain during mechanical ventilation: any safe threshold? Am J Respir Crit Care Med. (2011) 183:1354–62. doi: 10.1164/rccm.201010-1757O
36. Marini JJ, Rocco PRM, Gattinoni L. Static and dynamic contributors to ventilator-induced lung injury in clinical practice. Pressure, energy, and power. Am J Respir Crit Care Med. (2020) 201:767–74. doi: 10.1164/rccm.201908-1545CI
37. Uchida T, Shirasawa M, Ware LB, Kojima K, Hata Y, Makita K, et al. Receptor for advanced glycation end-products is a marker of type I cell injury in acute lung injury. Am J Respir Crit Care Med. (2006) 173:1008–15. doi: 10.1164/rccm.200509-1477OC
38. Shirasawa M, Fujiwara N, Hirabayashi S, Ohno H, Iida J, Makita K, et al. Receptor for advanced glycation endcationhiw is a marker of type I lung alveolar cells. Genes to Cells. (2004) 9:165–74. doi: 10.1111/j.1356-9597.2004.00712.x
39. Sierra-Colomina M, Garcia-Salido A, Leoz-Gordillo I, Martinez de Azagra-Garde A, Melen G, Garcia-Teresa MA, et al. sRAGE as severe acute bronchiolitis biomarker, prospective observational study. Pediatr Pulmonol. (2020) 55:3429–36. doi: 10.1002/ppul.v55.12
40. Jabaudon M, Blondonnet R, Roszyk L, Bouvier D, Audard J, Clairefond G, et al. Soluble receptor for advanced glycation end-products predicts impaired alveolar fluid clearance in acute respiratory distress syndrome. Am J Respir Crit Care Med. (2015) 192:191–9. doi: 10.1164/rccm.201501-0020OC
41. Ware LB, Matthay MA. Alveolar fluid clearance is impaired in the majority of patients with acute lung injury and the acute respiratory distress syndrome. Am J Respir Crit Care Med. (2001) 163:1376–83. doi: 10.1164/ajrccm.163.6.2004035
42. Jabaudon M, Hamroun N, Roszyk L, Guerin R, Bazin JE, Sapin V, et al. Effects of a recruitment maneuver on plasma levels of soluble RAGE in patients with diffuse acute respiratory distress syndrome: a prospective randomized crossover study. Intensive Care Med. (2015) 41:846–55. doi: 10.1007/s00134-015-3726-0
43. Yehya N, Thomas NJ, Meyer NJ, Christie JD, Berg RA, Margulies SS. Circulating markers of endothelial and alveolar epithelial dysfunction are associated with mortality in pediatric acute respiratory distress syndrome. Intensive Care Med. (2016) 42:1137–45. doi: 10.1007/s00134-016-4352-1
44. Kuipers MT, Aslami H, Tuinman PR, Tuip-de Boer AM, Jongsma G, van der Sluijs KF, et al. The receptor for advanced glycation end products in ventilator-induced lung injury. Intensive Care Med Exp. (2014) 2:22. doi: 10.1186/s40635-014-0022-1
45. Jabaudon M, Berthelin P, Pranal T, Roszyk L, Godet T, Faure JS, et al. Receptor for advanced glycation end-products and ARDS prediction: a multicentre observational study. Sci Rep. (2018) 8:2603. doi: 10.1038/s41598-018-20994-x
46. Mittel A, Drubin C, Hua M, Nitta S, Wagener G, Vidal Melo MF. Association of acute systemic inflammation with patient-centric postoperative pulmonary complications after elective cardiac surgery. Anesth Analg. (2024). doi: 10.1213/ANE.0000000000007122
47. Sparvero LJ, Asafu-Adjei D, Kang R, Tang D, Amin N, Im J, et al. RAGE (Receptor for Advanced Glycation Endproducts), RAGE ligands, and their role in cancer and inflammation. J Transl Med. (2009) 7:17. doi: 10.1186/1479-5876-7-17
48. Hudson BI, Lippman ME. Targeting RAGE signaling in inflammatory disease. Annu Rev Med. (2018) 69:349–64. doi: 10.1146/annurev-med-041316-085215
49. Lin L, Li J, Song Q, Cheng W, Chen P. The role of HMGB1/RAGE/TLR4 signaling pathways in cigarette smoke-induced inflammation in chronic obstructive pulmonary disease. Immun Inflammation Dis. (2022) 10:e711. doi: 10.1002/iid3.v10.11
50. Rojas A, Lindner C, Schneider I, Gonzalez I, Uribarri J. The RAGE axis: A relevant inflammatory hub in human diseases. Biomolecules. (2024) 14:412. doi: 10.3390/biom14040412
51. Reynolds PR, Schmitt RE, Kasteler SD, Sturrock A, Sanders K, Bierhaus A, et al. Receptors for advanced glycation end-products targeting protect against hyperoxia-induced lung injury in mice. Am J Respir Cell Mol Biol. (2010) 42:545–51. doi: 10.1165/rcmb.2008-0265OC
52. Su X, Looney MR, Gupta N, Matthay MA. Receptor for advanced glycation end-products (RAGE) is an indicator of direct lung injury in models of experimental lung injury. Am J Physiol Lung Cell Mol Physiol. (2009) 297:L1–5. doi: 10.1152/ajplung.90546.2008
53. Jones TK, Feng R, Kerchberger VE, Reilly JP, Anderson BJ, Shashaty MGS, et al. Plasma sRAGE acts as a genetically regulated causal intermediate in sepsis-associated acute respiratory distress syndrome. Am J Respir Crit Care Med. (2020) 201:47–56. doi: 10.1164/rccm.201810-2033OC
54. Keefe J, Yao C, Hwang SJ, Courchesne P, Lee GY, Dupuis J, et al. An integrative genomic strategy identifies sRAGE as a causal and protective biomarker of lung function. Chest. (2022) 161:76–84. doi: 10.1016/j.chest.2021.06.053
55. Yonchuk JG, Silverman EK, Bowler RP, Agustí A, Lomas DA, Miller BE, et al. Circulating soluble receptor for advanced glycation end products (sRAGE) as a biomarker of emphysema and the RAGE axis in the lung. Am J Respir Crit Care Med. (2015) 192:785–92. doi: 10.1164/rccm.201501-0137PP
56. Aglago EK, Rinaldi S, Freisling H, Jiao L, Hughes DJ, Fedirko V, et al. Soluble receptor for advanced glycation end-products (sRAGE) and colorectal cancer risk: A case-control study nested within a european prospective cohort. Cancer Epidemiol Biomarkers Prev. (2021) 30:182–92. doi: 10.1158/1055-9965.EPI-20-0855
57. Calfee CS, Gallagher D, Abbott J, Thompson BT, Matthay MA, Network NA. Plasma angiopoietin-2 in clinical acute lung injury: prognostic and pathogenetic significance. Crit Care Med. (2012) 40:1731–7. doi: 10.1097/CCM.0b013e3182451c87
58. Orfanos SE, Kotanidou A, Glynos C, Athanasiou C, Tsigkos S, Dimopoulou I, et al. Angiopoietin-2 is increased in severe sepsis: correlation with inflammatory mediators. Crit Care Med. (2007) 35:199–206. doi: 10.1097/01.CCM.0000251640.77679.D7
59. Parikh SM, Mammoto T, Schultz A, Yuan HT, Christiani D, Karumanchi SA, et al. Excess circulating angiopoietin-2 may contribute to pulmonary vascular leak in sepsis in humans. PloS Med. (2006) 3:e46. doi: 10.1371/journal.pmed.0030046
60. van der Heijden M, van Nieuw Amerongen GP, van Hinsbergh VW, Groeneveld AB. The interaction of soluble Tie2 with angiopoietins and pulmonary vascular permeability in septic and nonseptic critically ill patients. Shock. (2010) 33:263–8. doi: 10.1097/SHK.0b013e3181b2f978
61. Leong A, Kim M. The angiopoietin-2 and TIE pathway as a therapeutic target for enhancing antiangiogenic therapy and immunotherapy in patients with advanced cancer. Int J Mol Sci. (2020) 21:8689. doi: 10.3390/ijms21228689
62. Terpstra ML, Aman J, van Nieuw Amerongen GP, Groeneveld AB. Plasma biomarkers for acute respiratory distress syndrome: a systematic review and meta-analysis*. Crit Care Med. (2014) 42:691–700. doi: 10.1097/01.ccm.0000435669.60811.24
63. Agrawal A, Matthay MA, Kangelaris KN, Stein J, Chu JC, Imp BM, et al. Plasma angiopoietin-2 predicts the onset of acute lung injury in critically ill patients. Am J Respir Crit Care Med. (2013) 187:736–42. doi: 10.1164/rccm.201208-1460OC
64. Khan KA, Wu FT, Cruz-Munoz W, Kerbel RS. Ang2 inhibitors and Tie2 activators: potential therapeutics in perioperative treatment of early stage cancer. EMBO Mol Med. (2021) 13:e08253. doi: 10.15252/emmm.201708253
65. Fukumura D, Kloepper J, Amoozgar Z, Duda DG, Jain RK. Enhancing cancer immunotherapy using antiangiogenics: opportunities and challenges. Nat Rev Clin Oncol. (2018) 15:325–40. doi: 10.1038/nrclinonc.2018.29
66. Ogawa M, Yamamoto H, Nagano H, Miyake Y, Sugita Y, Hata T, et al. Hepatic expression of ANG2 RNA in metastatic colorectal cancer. Hepatology. (2004) 39:528–39. doi: 10.1002/hep.20048
67. Liu N, Liu M, Fu S, Wang J, Tang H, Isah AD, et al. Ang2-targeted combination therapy for cancer treatment. Front Immunol. (2022) 13:949553. doi: 10.3389/fimmu.2022.949553
68. Gengenbacher N, Singhal M, Mogler C, Hai L, Milde L, Pari AAA, et al. Timed ang2-targeted therapy identifies the angiopoietin-tie pathway as key regulator of fatal lymphogenous metastasis. Cancer Discovery. (2021) 11:424–45. doi: 10.1158/2159-8290.CD-20-0122
69. Pyrillou K, Burzynski LC, Clarke MCH. Alternative pathways of IL-1 activation, and its role in health and disease. Front Immunol. (2020) 11:613170. doi: 10.3389/fimmu.2020.613170
70. Tanaka T, Narazaki M, Kishimoto T. IL-6 in inflammation, immunity, and disease. Cold Spring Harb Perspect Biol. (2014) 6:a016295. doi: 10.1101/cshperspect.a016295
71. Serpa Neto A, Campos PP, Hemmes SN, Bos LD, Bluth T, Ferner M, et al. Kinetics of plasma biomarkers of inflammation and lung injury in surgical patients with or without postoperative pulmonary complications. Eur J Anaesthesiol. (2017) 34:229–38. doi: 10.1097/EJA.0000000000000614
72. Jabaudon M, Futier E, Roszyk L, Sapin V, Pereira B, Constantin JM. Association between intraoperative ventilator settings and plasma levels of soluble receptor for advanced glycation end-products in patients without pre-existing lung injury. Respirology. (2015) 20:1131–8. doi: 10.1111/resp.2015.20.issue-7
73. Ljungqvist O, Scott M, Fearon KC. Enhanced recovery after surgery: A review. JAMA Surg. (2017) 152:292–8. doi: 10.1001/jamasurg.2016.4952
74. Zheng Y, Mao M, Li F, Wang L, Zhang X, Zhang X, et al. Effects of enhanced recovery after surgery plus pulmonary rehabilitation on complications after video-assisted lung cancer surgery: a multicentre randomised controlled trial. Thorax. (2022) 78(6):574–86. doi: 10.1136/thoraxjnl-2021-218183
75. Lin XH, Jiang JK, Luo JC, Lin CC, Ting PH, Yang UC, et al. The long term microbiota and metabolic status in patients with colorectal cancer after curative colon surgery. PloS One. (2019) 14:e0218436. doi: 10.1371/journal.pone.0218436
76. Rao SSC, Tan G, Abdulla H, Yu S, Larion S, Leelasinjaroen P. Does colectomy predispose to small intestinal bacterial (SIBO) and fungal overgrowth (SIFO)? Clin Transl Gastroenterol. (2018) 9:146. doi: 10.1038/s41424-018-0011-x
77. Hope C, Reilly J, Lund J, Andreyev H. Systematic review: the effect of right hemicolectomy for cancer on postoperative bowel function. Support Care Cancer. (2020) 28:4549–59. doi: 10.1007/s00520-020-05519-5
78. McAlister FA, Bertsch K, Man J, Bradley J, Jacka M. Incidence of and risk factors for pulmonary complications after nonthoracic surgery. Am J Respir Crit Care Med. (2005) 171:514–7. doi: 10.1164/rccm.200408-1069OC
79. Robinson TN, Wu DS, Pointer L, Dunn CL, Cleveland JC Jr., Moss M. Simple frailty score predicts postoperative complications across surgical specialties. Am J Surg. (2013) 206:544–50. doi: 10.1016/j.amjsurg.2013.03.012
80. Hao J, Pang P, Liu X, Chi W, Luo Z, Cai W, et al. Can the lung ultrasound score predict pulmonary complications after non-thoracic surgery in patients with blunt thoracic trauma: A single-center observational study. J Clin Anesth. (2024) 99:111675. doi: 10.1016/j.jclinane.2024.111675
81. Raphael J, Chae A, Feng X, Shotwell MS, Mazzeffi MA, Bollen BA, et al. Red blood cell transfusion and pulmonary complications: the society of thoracic surgeons adult cardiac surgery database analysis. Ann Thorac Surg. (2024) 117:839–46. doi: 10.1016/j.athoracsur.2023.12.012
82. Yokota S, Koizumi M, Togashi K, Morimoto M, Yasuda Y, Sata N, et al. Preoperative pulmonary function tests do not predict the development of pulmonary complications after elective major abdominal surgery: A prospective cohort study. Int J Surg. (2020) 73:65–71. doi: 10.1016/j.ijsu.2019.11.032
83. Lukannek C, Shaefi S, Platzbecker K, Raub D, Santer P, Nabel S, et al. The development and validation of the Score for the Prediction of Postoperative Respiratory Complications (SPORC-2) to predict the requirement for early postoperative tracheal re-intubation: a hospital registry study. Anaesthesia. (2019) 74:1165–74. doi: 10.1111/anae.14742
84. Schmitt M, Greten FR. The inflammatory pathogenesis of colorectal cancer. Nat Rev Immunol. (2021) 21:653–67. doi: 10.1038/s41577-021-00534-x
Keywords: colorectal cancer, Trendelenburg position, inflammatory biomarker, lung injury, postoperative pulmonary complications
Citation: Li H-x, Che L, Li Y, Wang T-h, Min F-d, Xu L, Wang M, Zheng Z-x, Qu S-n, Wang F, Tang W, Wei S-j, Sun Y-l, Zheng H and Yan T (2025) Correlations between primary tumour location, biomarkers of inflammation and lung injury, and postoperative pulmonary complications in patients underwent laparoscopic colorectomy: a propensity score matched analysis of 300 patients. Front. Immunol. 16:1546167. doi: 10.3389/fimmu.2025.1546167
Received: 16 December 2024; Accepted: 06 January 2025;
Published: 30 January 2025.
Edited by:
Xiaosheng Tan, Rutgers, United StatesReviewed by:
Xiaowei Wu, Dana–Farber Cancer Institute, United StatesCopyright © 2025 Li, Che, Li, Wang, Min, Xu, Wang, Zheng, Qu, Wang, Tang, Wei, Sun, Zheng and Yan. This is an open-access article distributed under the terms of the Creative Commons Attribution License (CC BY). The use, distribution or reproduction in other forums is permitted, provided the original author(s) and the copyright owner(s) are credited and that the original publication in this journal is cited, in accordance with accepted academic practice. No use, distribution or reproduction is permitted which does not comply with these terms.
*Correspondence: Tao Yan, eWFudGFvQGNpY2Ftcy5hYy5jbg==; YmxpenphcmR5dEAxNjMuY29t; Hui Zheng, emhlbmdodWlAY2ljYW1zLmFjLmNu; Yu-lin Sun, eWxzdW5AY2ljYW1zLmFjLmNu
†These authors have contributed equally to this work and share first authorship
Disclaimer: All claims expressed in this article are solely those of the authors and do not necessarily represent those of their affiliated organizations, or those of the publisher, the editors and the reviewers. Any product that may be evaluated in this article or claim that may be made by its manufacturer is not guaranteed or endorsed by the publisher.
Research integrity at Frontiers
Learn more about the work of our research integrity team to safeguard the quality of each article we publish.