- 1Department of Gynecology and Obstetrics, West China Second University Hospital, Sichuan University, Chengdu, Sichuan, China
- 2Key Laboratory of Birth Defects and Related Diseases of Women and Children, Ministry of Education, Chengdu, Sichuan, China
- 3Joint Laboratory of Reproductive Medicine (SCU-CUHK), West China Second University Hospital, Sichuan University, Chengdu, Sichuan, China
- 4West China School of Medicine, Sichuan University, Chengdu, Sichuan, China
- 5Department of Pathology, West China Second University Hospital, Sichuan University, Chengdu, Sichuan, China
Gestational trophoblastic neoplasia (GTN) is a rare but aggressive malignancy that follows normal or aberrant pregnancies. Until the advent of immunotherapy in 2017, surgery and chemotherapy were the standard treatment modalities, with chemotherapy remaining the cornerstone. However, chemoresistance and high-risk disease present significant challenges in managing GTN. Recent advancements in immunotherapy, particularly immune checkpoint inhibitors (ICIs), have offered new hope for managing these difficult cases. This review provides the comprehensive overview of the mechanisms underlying ICIs in GTN, and explores the potential synergy of combining ICIs with targeted therapies, such as vascular endothelial growth factor and epidermal growth factor receptor inhibitors. We also provide an overview of the latest evidence on the use of ICIs in treating GTN, focusing on their effectiveness in both low- and high-risk cases, as well as in chemorefractory settings. In addition, we discuss ongoing clinical trials, immune-related adverse events associated with ICIs, biomarker-driven approaches, immunosuppressive tumor microenvironments, and the challenges posed with ICIs resistance. The review also explores future directions, including the integration of ICIs into standard regimens, the potential for personalized treatment based on tumor biology, and the importance of fertility preservation in young patients with GTN. In conclusion, while challenges remain, immunotherapy represents a promising frontier in GTN treatment, with the potential to improve outcomes and provide a more personalized approach to care
1 Introduction
Gestational trophoblastic neoplasia (GTN) represents a rare yet distinct category of malignancies originating from the abnormal transformation of placental trophoblastic tissues following normal or aberrant fertilization. It encompasses various tumor types, including invasive mole, choriocarcinoma, placental site trophoblastic tumor (PSTT), and epithelioid trophoblastic tumor (ETT) (1). According to the International Federation of Gynecology and Obstetrics (FIGO) 2000 risk scoring system, GTN is stratified into low-risk (FIGO score <7), high-risk (FIGO score ≥7), and ultra-high-risk (FIGO score ≥13) groups. However, PSTT and ETT are not managed based on the FIGO score (2). While low-risk GTN typically responds well to single-agent chemotherapy, high-risk GTN requires multi-agent chemotherapy (1). However, approximately 5% of patients experience chemotherapy resistance or relapse, highlighting an unmet need for novel therapeutic strategies (3–5). Immunotherapy has emerged as a promising option, especially for chemoresistant GTN. In particular, immune checkpoint inhibitors (ICIs) targeting the programmed cell death protein 1/programmed cell death ligand 1(PD-1/PD-L1) axis have demonstrated notable efficacy in cases where conventional chemotherapy fails. This review examines advances in immunotherapy for GTN, focusing on its mechanisms, clinical efficacy, Immune-related adverse events (irAEs) with ICIs, impact on fertility, and future research directions (Figure 1).
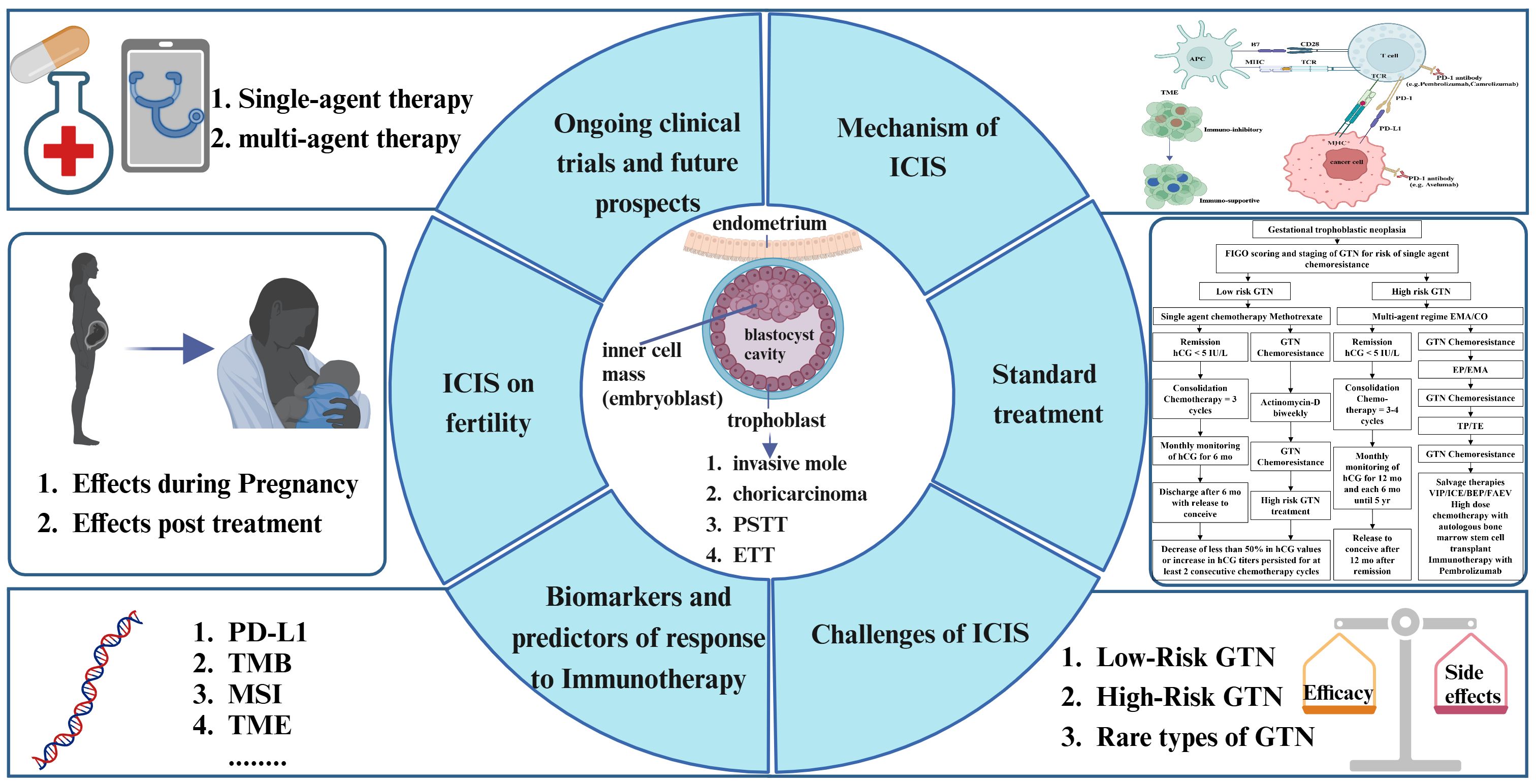
Figure 1. Conceptual framework of our study. This figure outlines the comprehensive approach to investigating immune checkpoint inhibitors (ICIs) in gestational trophoblastic neoplasia (GTN). It encompasses the mechanisms of ICIs in immune regulation, therapeutic efficacy, integration with standard treatments, exploration of predictive biomarkers, fertility-related considerations, and forward-looking perspectives on the future of GTN immunotherapy.
2 Standard treatment in GTN
2.1 Treatment in low-risk GTN
The standard treatment of GTN is stratified into low-risk and high-risk categories based on the FIGO scoring system, which informs the choice of therapeutic strategy (6). (Figure 2) As above, ultra-high risk and PSTT/ETT are treated separately. Low-risk GTN (FIGO score <7) typically responds well to single-agent chemotherapy. For women who have completed childbearing and do not have metastatic disease, then a hysterectomy could be considered (7). Commonly used agents include methotrexate and folinic acid (MTX/FA) or actinomycin-D (ActD), both showing high remission rates with minimal toxicity. MTX, which can be administered as a weekly intramuscular injection or in multi-day protocols, is often chosen for its high efficacy and manageable toxicity profile. ActD serves as an alternative, especially for patients who do not respond well to MTX or experience unacceptable side effects (8). Patients scoring 0–1 on the FIGO scale have a high cure rate (90%) with single-agent therapy, but resistance risk rises in patients with scores of 5–6, with only one-third achieving a cure with initial single-agent treatment (9). Resistance to MTX/FA can often be managed successfully by switching to ActD. Multi-agent regimens like EMA/CO (etoposide, methotrexate, and actinomycin-D/cyclophosphamide and vincristine) are reserved for patients with higher hCG levels or when initial single-agent therapies fail. Recent practices have adjusted the hCG threshold for transitioning to multi-agent treatments, with centers like Charing Cross hospital using a threshold up to 3000 IU/l to reduce the need for EMA/CO, minimizing toxicity (10).
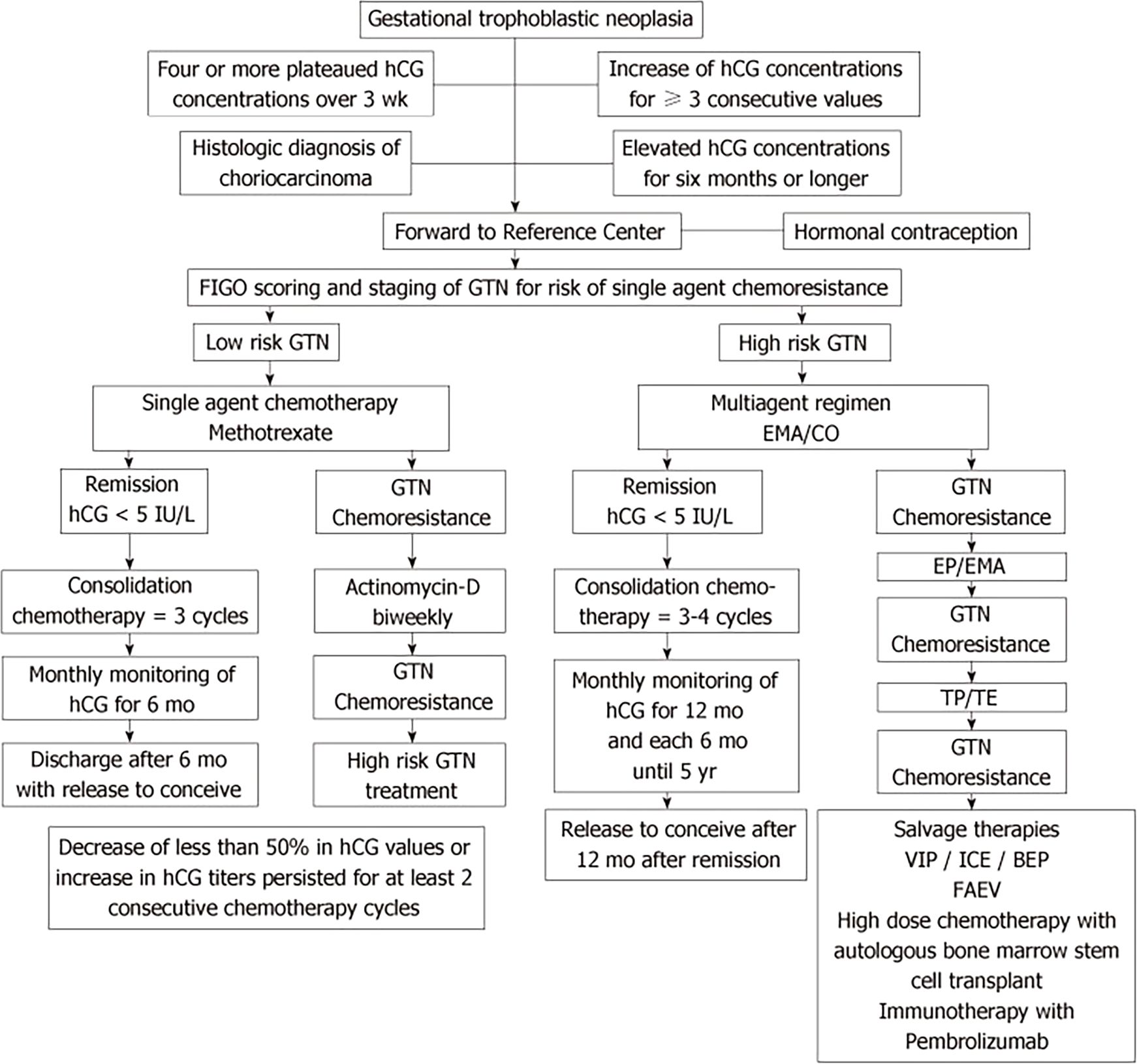
Figure 2. Algorithm for standard treatment of gestational trophoblastic neoplasia [adapted from Braga et al., (6)].
2.2 Treatment in high-risk GTN
Women with a FIGO score of 7 or higher receive multi-agent chemotherapy, typically using the EMA/CO regimen. For patients with a score of 13 or higher, considered “ultra-high risk,” there is a significant risk of early and late mortality due to complications like hemorrhage or metabolic issues from a heavy tumor burden. Low-dose etoposide and cisplatin induction therapy can mitigate early death (11, 12). For those with liver metastases, EP/EMA regimen (etoposide, cisplatin/etoposide, methotrexate, actinomycin-D) may reduce the risk of late deaths from drug resistance. Treatment continues with EMA/CO until hCG normalization, followed by consolidation therapy for 6–8 weeks, particularly if high-risk features (e.g., brain or liver metastases) are present. Studies evaluating alternative regimens like 4-day MEA (etoposide, methotrexate, actinomycin-D) or FAEV(5-fluorouracil, actinomycin D, etoposide, vincristine) have shown activity but without clear superiority over EMA/CO (13, 14).
2.3 Treatment in chemoresistant or relapsed GTN
For patients with chemoresistant or relapsed GTN, there are no randomized trials specifically evaluating treatment options for GTN relapse, and existing studies often do not differentiate between resistance and relapse, indicating that the same chemotherapy regimens used for chemoresistant cases may also apply to relapsed disease. Prior to treatment, all patients should be re-staged to identify new metastatic sites. Surgical resection of isolated metastatic lesions is advised, and Positron Emission Tomography/Computed Tomography (PET/CT) can help differentiate viable tumor from necrotic tissue. For high-risk relapsed cases, sequential multi-agent regimens such as EMA-EP or TP-TE (paclitaxel, cisplatin/paclitaxel, etoposide) have shown better outcomes, but if resistance occurs, options like BEP (bleomycin, etoposide, cisplatin) or ICE (ifosfamide, carboplatin, cisplatin) regimens may be used (6, 15, 16). Immunotherapy, particularly ICIs, offers a promising alternative for chemoresistant GTN, with emerging evidence supporting its efficacy.
2.4 Treatment in rare type GTN
PSTT and ETT present unique challenges as they typically show less responsiveness to conventional chemotherapy. These subtypes often require surgical intervention, such as hysterectomy, especially for localized stage I disease, which can achieve high long-term survival rates (17). In cases of advanced or metastatic disease, platinum-based multi-agent regimens, such as EP/EMA and TP/TE are commonly used, although the prognosis remains challenging with overall survival rates between 50% and 60% (17, 18). It is important to note that an interval ≥48 months from their last known pregnancy and stage IV disease are important predictors of chemoresistance (17, 19). For patients with chemotherapy-resistant disease, newer treatments like ICIs have shown promise in achieving complete remission (20). Careful monitoring of prognostic factors, including FIGO stage, hCG levels, and time from the antecedent pregnancy, is crucial in guiding treatment decisions. The use of adjuvant chemotherapy is also an important consideration in these cases. Referral to specialized centers is recommended for high-risk cases to ensure optimal management.
In summary, the standard treatment for GTN involves a tailored approach based on the patient’s risk classification, ranging from single-agent chemotherapy for low-risk cases to multi-agent regimens and surgical interventions for high-risk and chemoresistant forms. Emerging targeted therapies and immunotherapies hold promise for improving outcomes, especially in cases where traditional treatments fail.
3 Mechanisms related to GTN treatment
3.1 Different subtypes of GTN
GTN encompasses four tumor types: invasive mole, choriocarcinoma, PSTT, and ETT, all of which originate from abnormal trophoblastic proliferation. These subtypes exhibit distinct genetic, epigenetic, and clinical characteristics that influence their behavior and treatment responses. Invasive moles, characterized by the penetration of molar villi into the myometrium, originate from complete or partial hydatidiform moles. Partial hydatidiform moles are typically triploid, containing both maternal and paternal genetic material, while complete hydatidiform moles are usually diploid, consisting only of paternal genes due to the absence of maternal DNA (16). Choriocarcinoma is a highly malignant tumor arising from any gestational event, characterized by abnormal trophoblastic hyperplasia and anaplasia, the absence of chorionic villi and vascular invasion. Its aggressiveness is often linked to epigenetic modifications, such as hypermethylation of tumor suppressor genes like DNA methyltransferase 3 beta (DNMT3B), which is the enzyme involved in de novo methylation of DNA during development (21). The process of hypermethylation significantly influences key molecular pathways, including phosphatidylinositol 3-hydroxy kinase-Akt (PI3K-Akt), human epidermal growth factor receptor-2 (ERBB2)/ERBB2 receptor tyrosine kinase 3 (ERBB3), and Janus kinase- signal transducer and activator of transcription (JAK-STAT), by promoting cellular proliferation, enhancing migration, and enabling the evasion of apoptotic signals (21). However, the pathways altered in choriocarcinoma remain poorly understood, and available data are limited.
Based on limited data, PSTT and ETT are thought to originate from different subtyped of trophoblast (22). The precise cell of origin remains speculative and poorly understood. While different trophoblast subtypes, including villous cytotrophoblasts, syncytiotrophoblasts, and intermediate trophoblasts, play distinct roles during normal placental development (23), the relationship between these cell types and the malignancy of GTN remains unclear. Disease biology may be related to the cell of origin, but this requires further investigation and clarification. Compared to choriocarcinoma, PSTT and ETT exhibit slower growth and lower hCG levels, along with chemotherapy resistance. These tumors are generally diploid with biparental contributions. The presence of both maternal and paternal genetic material, or in the case of a complete hydatidiform mole, exclusively paternal DNA, renders GTN highly immunogenic and particularly susceptible to immunotherapeutic interventions.
3.2 Mechanism of ICIs in GTN
The immune microenvironment in GTN shares similarities with the placental environment, characterized by the presence of regulatory T cells (Tregs) and immunosuppressive cytokines like Interleukin-10 (IL-10) and transforming growth factor-β (TGF-β), which create an anti-inflammatory state that supports tumor survival (24). These factors inhibit cytotoxic immune responses, aiding in tumor persistence. The immunosuppressive environment in GTN is further reinforced by the expression of indoleamine 2,3-dioxygenase (IDO), which modulates tryptophan metabolism, leading to T cell suppression and heightened immune tolerance (24).
Tumor immune escape plays a critical role in the malignancy of GTN, with immune checkpoints serving as key mechanisms that prevent apoptosis of cancer cells. PD-1, expressed predominantly on activated cytotoxic T lymphocytes (CTLs), is upregulated during T cell activation, not just under chronic antigen stimulation, such as cancer. The upregulation is asscociated with reduced T cell function, which impairs the immune system’s ability to effectively target and eliminate tumor cells (25–27). Tumor cells exploit this by expressing co-inhibitory molecules like members of the B7 family, suppressing CTLs and evading immune detection (26, 28). Immunohistochemistry studies show that PD-L1 is strongly expressed in various GTN cell types, including syncytiotrophoblasts and cytotrophoblasts, indicating that GTN employs immune evasion strategies similar to the placenta (29). The high levels of PD-L1 expression in GTN make PD-1/PD-L1 inhibitors a logical therapeutic approach. Studies have shown that blocking PD-1/PD-L1 signaling with drugs like Pembrolizumab can restore immune surveillance, leading to tumor regression in patients with chemoresistant GTN (30, 31). Additionally, other B7 family members, such as B7-H3 (CD276) and VISTA (PD-H1), are also highly expressed in both normal placental tissue and GTN. While VISTA’s exact receptor remains unknown, other B7 family members expression, particularly when occurring alongside PD-L1, further supports the rationale for targeting the PD-1/PD-L1 pathway with ICIs as a potential treatment not only for chemo-resistant GTN but also for patients with newly diagnosed disease who are at high risk of developing resistance (29, 32). Figure 3 shows the pathogenesis of PD-1/PD-L1 blockade in GTN.
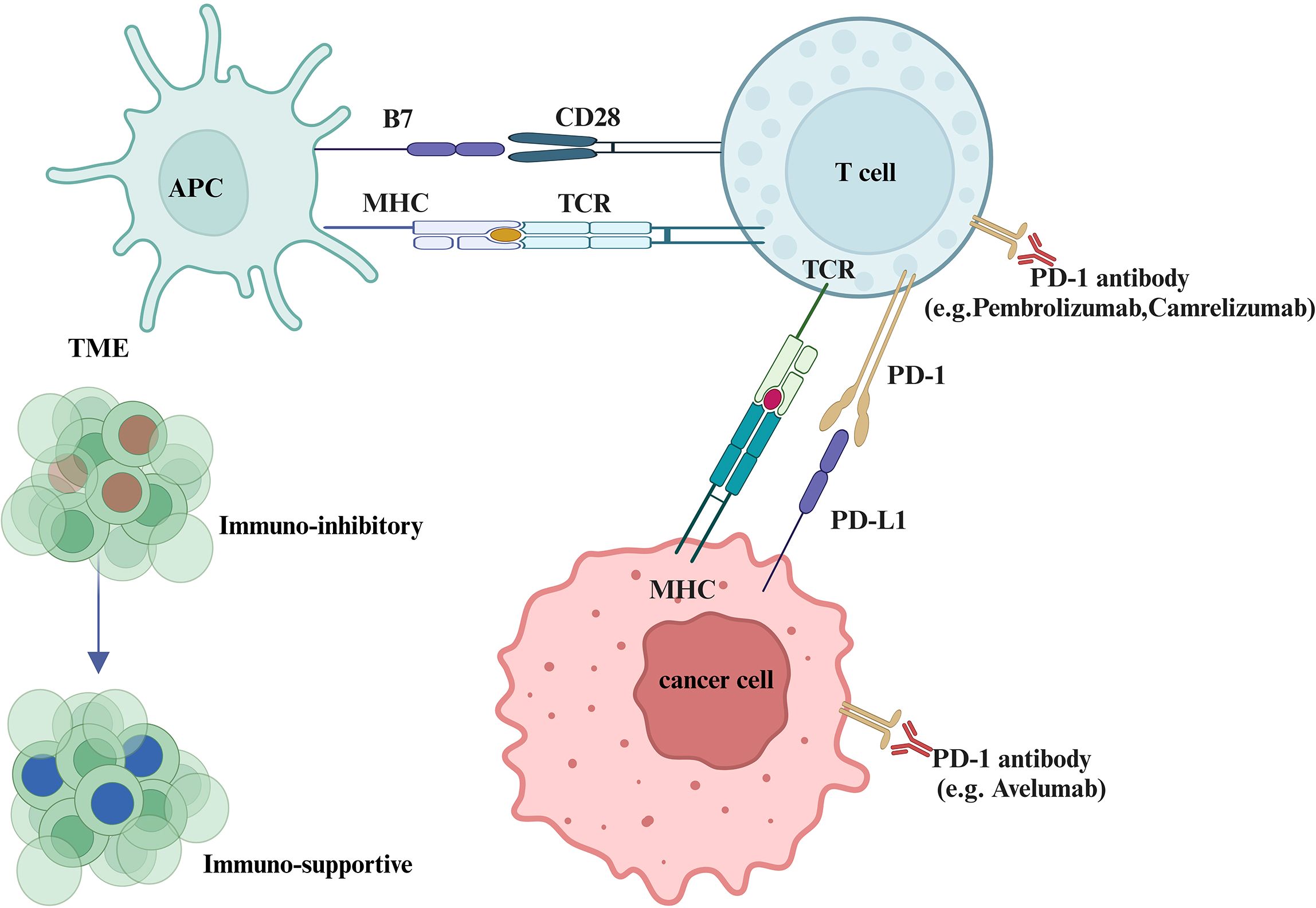
Figure 3. Pathogenesis of PD-1/PD-L1 blockade in malignant tumors. This figure illustrates how PD-1/PD-L1 interactions facilitate tumor immune evasion. Tumor cells suppress cytotoxic T lymphocytes (CTLs) by expressing co-inhibitory molecules such as PD-L1, leading to immune escape. When PD-1 antibodies (e.g., pembrolizumab, camrelizumab) or PD-L1 inhibitors (e.g., avelumab) are administered, CTLs regain their tumor-killing capabilities.The figure also highlights how the tumor immune microenvironment (TME) from an immunosuppressive to an activated state, which is enriched with immunosuppressive factors like regulatory T cells (Tregs) and cytokines such as TGF-β and IL-10, can inhibit immune responses and support tumor survival. ICIs can shift the TME toward activation by promoting the activity of tumor-infiltrating lymphocytes (TILs), especially CD8+ cytotoxic T cells.
3.3 Mechanisms of combined therapy in GTN
Combining ICIs with targeted therapies that inhibit pathways like vascular endothelial growth factor (VEGF) and epidermal growth factor receptor (EGFR) may further enhance efficacy by addressing tumor angiogenesis and proliferation. VEGF promotes tumor angiogenesis, supporting tumor growth and metastasis, and contributes to an immunosuppressive tumor microenvironment by recruiting regulatory Tregs and inhibiting dendritic cell maturation, which impairs antigen presentation and reduces T cell activation (33). Combining VEGF-targeted therapies with immune ICIs, such as those blocking the PD-1/PD-L1 pathway, has shown potential to enhance anti-tumor responses (34). VEGF inhibition can decrease the immunosuppressive properties of the tumor microenvironment, thereby supporting T cell activity and improving the efficacy of ICIs (33). This combined approach reduces tumor vascular support while boosting immune system engagement, providing a potential strategy for treating aggressive and chemoresistant GTN. EGFR, part of the ERBB receptor family, is also overexpressed in GTN and is associated with increased cell proliferation and survival (35). Research indicates that drugs such as lapatinib can inhibit GTN cell growth by modulating EGFR and ERBB2 activity (36). These pathways underscore the potential of integrating targeted therapies with immunotherapy to improve GTN treatment outcomes.
4 Clinical efficacy of ICIs
4.1 Application of ICIs in low-risk GTN
Low-risk GTN generally responds well to single-agent chemotherapy, with cure rates exceeding 90%. For this reason, ICIs have not been widely utilized in low-risk GTN, as standard treatments are highly effective. However, in cases where patients are intolerant to chemotherapy, or when chemotherapy poses significant risk due to toxicity, the exploration of alternative therapies like ICIs has emerged as a valuable avenue of research. One significant investigation into the role of ICIs in GTN, particularly low-risk cases, is cohort A of the TROPHIMMUN Phase II Trial (37). Table 1 shows the key phase II clinical trials of immunotherapy for GTN.
The TROPHIMMUN Phase II Trial is a pivotal study evaluating the efficacy and safety of Avelumab, an anti-PD-L1 immune checkpoint inhibitor, in GTN patients. The trial includes two cohorts: Cohort A, focused on low-risk GTN patients first-line treatment resistant to MTX, and Cohort B, targeting high-risk or chemoresistant GTN (37, 38). In Cohort A, 15 patients were treated with avelumab at a dose of 10 mg/kg bi-weekly until normalization of serum hCG levels, followed by three consolidation cycles. Results showed that 8 patients (53.3%) achieved complete remission with normalized hCG levels after a median of 9 treatment cycles, and none experienced relapse after a median follow-up of 29 months. The remaining 7 patients required further chemotherapy or surgery to achieve remission. Avelumab demonstrated a favorable safety profile, with most treatment-related adverse events (TRAEs) being mild (grade 1-2), including fatigue and infusion-related reactions (37). The findings suggest that avelumab could be an alternative for chemotherapy-resistant GTN, especially for patients preferring to avoid traditional side effects or those intolerant to MTX or ActD.
However, other scholars have pointed out Avelumab achieved a 53% remission rate, which falls short compared to the 75% remission rate observed with second-line ActD (39, 40). ActD remains the preferred option due to its proven efficacy. Additionally, while ICIs are more costly and long-term safety data are lacking, chemotherapy continues to demonstrate high success rates with manageable adverse effects. This underscores that, although ICIs provide an alternative therapy for patients intolerant to standard treatments, they have not yet replaced chemotherapy as the optimal approach for low-risk GTN. Future studies might explore ICIs as first-line treatments for selected low-risk GTN patients. This trial marks a significant step in expanding immunotherapy options for GTN beyond high-risk cases.
4.2 Challenges of ICIs in high-risk and chemoresistant GTN
Patients with multidrug-resistant GTN historically face a poor prognosis, but pembrolizumab has shown potential for achieving complete remission in this challenging group. The initial report in 2017 highlighted four patients with high-risk GTN treated after multiple chemotherapy regimens, resulting in three complete responses and one death (30). These promising outcomes led to the development of UK guidelines recommending pembrolizumab for high-risk GTN patients who have failed at least two lines of multi-agent chemotherapy, including EMA/CO, and as an alternative for PSTT/ETT cases post-platinum-based chemotherapy (41). During the COVID-19 pandemic, the guidelines were adjusted to permit pembrolizumab use after first-line EMA/CO. Treatment generally continues until hCG normalization or radiological disease resolution, followed by consolidation therapy. Some case reports further support pembrolizumab’s efficacy, showing hCG normalization with multidrug-resistant GTN (42–45).
Despite the potential benefits of ICIs, challenges remain, particularly in high-risk and chemoresistant GTN cases. Cohort B of the TROPHIMMUN Phase II Trial evaluated Avelumab in such patients, revealing mixed results. In this cohort, seven patients with polychemotherapy-resistant GTN received Avelumab at 10 mg/kg bi-weekly until hCG normalization, followed by three consolidation cycles. Only one patient (14.3%) achieved hCG normalization, highlighting limited efficacy, with a median resistance-free survival of 1.4 months and 57.1% of patients showing resistance to Avelumab (38). Although there are no head-to-head comparisons of anti-PD-1 and anti-PD-L1 agents, a meta-analysis showed that anti-PD-1 is more effective than anti-PD-L1 (46). This underscores the difficulty in overcoming the aggressive nature and chemoresistance of advanced GTN, pointing to the need for continued research and combination therapy approaches to enhance treatment outcomes.
The CAP01 study provided further insights into the application of PD-1 inhibitors and antiangiogenic agents in high-risk GTN. In the trial, 20 patients with high-risk, chemorefractory, or relapsed GTN were treated with camrelizumab (200 mg bi-weekly) plus apatinib (250 mg daily). The primary endpoint, objective response rate (ORR), was met by 55% of participants (95% CI 32–77), with 10 patients (50%) achieving complete response (CR) and no relapses after treatment discontinuation. Among the 10 patients who did not achieve CR, 7 responded effectively to salvage chemotherapy. The median time to CR was 3 cycles. The safety profile was considered manageable, with grade 3 TRAEs such as hypertension (25%), rash (20%), and neutropenia (10%). One patient discontinued treatment due to a serious adverse event (47). These results suggest that the combination of camrelizumab and apatinib demonstrates promising antitumor activity and could be explored as a salvage therapy for this challenging patient group. These findings indicate that while ICIs can contribute to disease control, they may not be sufficient as standalone treatments for high-risk or chemoresistant GTN.
A retrospective multicenter study assessed the safety and efficacy of combining anti-PD-1 therapy with chemotherapy versus anti-PD-1 monotherapy in patients with high-risk, chemorefractory, or relapsed GTN (48). Conducted across three hospitals in China, the study enrolled 66 patients, with 35 receiving anti-PD-1 therapy alone and 31 undergoing combination treatment. The ORR in the combined treatment group was significantly higher at 96.8% compared to 62.9% in the monotherapy group (p < 0.001). The CR rate was also greater in the combination group (87.1%) than in the monotherapy cohort (54.3%) (p = 0.007). The median time to achieve CR was 2.8 months for the combined group and 2.2 months for monotherapy. Progression-free survival (PFS) significantly favored the combined therapy group [HR 0.06 (95% CI 0.02–0.16), p < 0.001], while overall survival (OS) did not show a significant difference between groups [HR 0.50 (95% CI 0.07–3.24), p = 0.499]. TRAEs were comparable between groups, occurring in 77.1% of monotherapy and 80.6% of combination therapy patients, with the most common grade 3–4 events including leukopenia and elevated alanine aminotransferase levels. These results suggest that combining anti-PD-1 therapy with chemotherapy provides a significant benefit in ORR and PFS, offering an effective treatment option for high-risk GTN cases.
The ongoing exploration of combination therapies that integrate ICIs with conventional chemotherapy or targeted agents may hold promise for improving ORR. Current studies and anecdotal evidence suggest that such approaches might enhance the immune response and overcome resistance mechanisms inherent in high-risk GTN. However, these strategies require further validation through larger, prospective clinical trials to establish efficacy, safety, and optimal treatment protocols.
4.3 Role of ICIs in rare types of GTN
The role of ICIs in rare types of GTN, such as PSTT and ETT, has gained attention due to the unique challenges these tumors present. PSTT and ETT are known for their chemoresistance and slower growth, producing lower hCG levels relative to tumor volume. This profile limits the effectiveness of conventional chemotherapy and complicates treatment strategies. While the FIGO scoring system is not applicable to PSTT and ETT, treatment decisions are guided by specific prognostic factors, including an interval of over 48 months from the antecedent pregnancy and advanced stage IV disease, which are associated with poorer outcomes (17, 49).
Pembrolizumab has shown promise in treating chemo-resistant PSTT and ETT, as evidenced by multiple case reports (30, 50–52). For instance, the above case reports in 2017, three out of four patients with high-risk GTN, including 2 cases of PSTT and ETT, achieved complete remission following pembrolizumab treatment after failure of multi-agent chemotherapy (30). The TROPHIMMUN Phase II trial’s Cohort B, due to their pathological subtypes (4 patients with choriocarcinomas, 1 with ETT, 1 with PSTT, 1with an unknown subtype) and their FIGO scores at inclusion (≥ 10 for 4 of them), showed that while Avelumab led to hCG normalization in one out of seven patients (14.3%), its limited success underscores the complexity of treating chemoresistant GTN with monotherapy (38). Nonetheless, challenges remain, as the treatment of rare types like PSTT and ETT often requires aggressive multimodal approaches, including surgery and tailored combination regimens. These findings stress the need for further research to optimize the use of ICIs and combination therapies in rare GTN subtypes to improve patient outcomes.
4.4 Biomarkers and predictors of response to immunotherapy
The clinical studies highlighted above demonstrate variability in the response of GTN patients to ICIs. The success of ICIs in treating GTN largely hinges on the identification of reliable biomarkers to predict patient outcomes. This is particularly crucial given the heterogeneous nature of GTN. Recent advancements in genomic, transcriptomic, and tumor microenvironment analyses have shed light on potential biomarkers that could enhance the predictive accuracy for ICIs efficacy in GTN.
PD-L1 expression has been widely studied as a potential biomarker for immunotherapy, although its predictive value remains limited. Immunohistochemical studies reveal that PD-L1 is highly expressed in trophoblastic cells of GTN, including syncytiotrophoblasts and cytotrophoblasts, akin to its role in placental immune tolerance (29). Studies, such as the KEYNOTE-001 and KEYNOTE-052, have demonstrated a correlation between higher PD-L1 expression and improved clinical outcomes (53, 54). However, the heterogeneity of PD-L1 expression across tumor subtypes and the lack of standardized testing protocols complicate its application. Emerging evidence suggests that PD-L1 expression alone may not suffice as a standalone predictor, underscoring the need forcomplementary biomarkers.
Tumor mutational burden (TMB), defined as the number of somatic mutations per megabase, has been associated with responses to ICIs in various cancers. Studies, such as the KEYNOTE-158 trial, have shown that patients with high TMB (≥10 mutations/megabase) exhibit improved outcomes with pembrolizumab, leading to Food and Drug Administration (FDA) approval for its use in TMB-high solid tumors (55). However, the utility of TMB is limited by technical and biological factors. Technical limitations include variability in sequencing platforms and methodologies, while biologically, the predictive accuracy of TMB is affected by spatial and temporal tumor heterogeneity, subclonal variations, and the differing immunogenicity of individual mutations (56). In the case of GTN, however, TMB is generally low. A study analyzing 30 cases of GTN, including choriocarcinoma, ETT, PSTT, found that 92.8% of GTN samples exhibited low TMB. Despite this, some GTN subtypes, such as choriocarcinoma, showed changes in the homologous recombination repair (HRR) genes (57). These findings highlight that although TMB may not be a robust predictive biomarker for immunotherapy in GTN, other genetic and molecular features may provide useful insights for identifying patients who could benefit from ICIs.
Microsatellite Instability (MSI) and Mismatch Repair Deficiency (dMMR) are well-established biomarkers for predicting response to ICIs in multiple cancers. MSI, characterized by insertions or deletions in microsatellite sequences, emerges due to deficiencies in the MMR pathway (58, 59). These deficiencies result in genomic hypermutability, increased neoantigen production, and an immune-rich tumor microenvironment (TME), making MSI-high (MSI-H) tumors responsive to ICIs (60–62). The KEYNOTE-016 trial demonstrated an ORR of 40% for pembrolizumab in dMMR/MSI tumors, leading to FDA approval (63). Subsequent studies, like KEYNOTE-177 and CheckMate-142, evaluated pembrolizumab and nivolumab in metastatic colorectal cancer, reporting median PFS improvements and durable responses (64, 65). Furthermore, dual-agent approaches combining PD-1 and cytotoxic T lymphocyte-associated antigen 4 (CTLA-4) inhibitors show potential, as seen in CheckMate-142, which reported a 55% ORR in dMMR/MSI metastatic colorectal cancer (65). However, the prevalence of MSI-H or dMMR in GTN subtypes is not well-characterized, and further research is needed to explore their role in guiding immunotherapy.
TME plays a pivotal role in determining the response to ICIs. The presence of tumor-infiltrating lymphocytes (TILs), particularly CD8+ cytotoxic T cells, correlates with better responses to ICIs (66). In the context of GTN, a study demonstrated that TILs are associated with improved responses to pembrolizumab, further emphasizing the importance of TILs in immune response modulation (30). Conversely, immunosuppressive cells like Tregs and myeloid-derived suppressor cells (MDSCs) may limit the efficacy of ICIs by creating an anti-inflammatory TME (67, 68). Additionally, T-cell receptor (TCR) diversity and clonality measured through sequencing offer insights into immune activation and responsiveness to ICIs (69). High TCR clonality has been associated with better outcomes in head and neck squamous cell carcinoma and may hold promise in GTN (69). Furthermore, HLA-G expression has been identified as a potential biomarker for predicting response to ICIs in GTN, as it plays a role in immune evasion by modulating NK and T cell activity (30). This highlights the complexity of immune interactions within the TME and the potential for targeting multiple immune pathways to enhance therapeutic responses in GTN.
Despite promising biomarkers, resistance to ICIs remains a challenge in GTN. Mechanisms such as PD-L1 loss, TME immunosuppression, and alternative immune checkpoint activation (e.g., B7-H3 and VISTA) contribute to primary and acquired resistance (70). Understanding these resistance pathways is crucial for developing combination therapies that enhance ICIs efficacy. Integrating multiple biomarkers, such as PD-L1, TMB, MSI, and TME, could improve patient stratification and treatment personalization in GTN. Prospective studies combining ICIs with targeted agents like VEGF inhibitors may offer synergistic effects, overcoming resistance mechanisms. Further, advancements in single-cell sequencing and spatial transcriptomics will provide deeper insights into the interplay between tumor genetics and the immune microenvironment, paving the way for precision immunotherapy in GTN.
5 IrAE of ICIs
IrAEs are a critical consideration when using ICIs for cancer treatment, including in the context of GTN. While ICIs have revolutionized cancer treatment by enhancing immune responses against tumors, they also lead to unintended activation of the immune system, which can result in damage to normal tissues and organs (71–79). These immune-mediated side effects present a significant challenge in the clinical use of ICIs, especially in diseases like GTN, where the patient population is often young and reproductive health is a major concern.
5.1 Variation in irAE across ICI types and cancers
The types of irAEs vary depending on the specific ICIs used. A meta-analysis of 48 trials found that PD-1 blockade is associated with a higher frequency of pneumonitis, myalgia, hypothyroidism, arthralgia, and vitiligo, whereas CTLA-4 blockade tends to result in more colitis, hypophysitis, and skin-related irAEs (80). Additionally, even when the same ICI is used, the incidence of irAEs differs slightly across different diseases. When comparing melanoma, non-small cell lung cancer (NSCLC), and renal cell carcinoma, melanoma patients tend to experience more gastrointestinal and skin-related irAEs, whereas pneumonitis is more common in those with NSCLC and renal cell carcinoma (80). Based on clinical trial data of ICIs in GTN, the occurrence of irAEs has also shown to affect various systems (37, 47).
5.2 Spectrum of irAE across organ systems
The spectrum of irAEs associated with ICIs is broad and can involve virtually any organ system (80). Some of the most common irAEs are dermatologic, including pruritus, rashes, and vitiligo, as well as more severe manifestations like blistering or erythema multiforme (81). Gastrointestinal toxicity, particularly diarrhea, colitis, and abdominal pain, can occur and, in severe cases, may result in life-threatening conditions like colonic perforation (82, 83). Endocrinopathies are also common, with thyroiditis (hypo- or hyperthyroidism) being the most frequently reported, though adrenalitis, hypophysitis, and diabetes have also been observed (84–86). Hepatic toxicity, characterized by elevated liver enzymes (AST, ALT), may progress to immune-mediated hepatitis and, in rare cases, liver failure (76). Pulmonary complications, such as pneumonitis, and cardiovascular effects, including myocarditis, are less common but can be fatal if not promptly recognized and treated (73, 79).
As more patients achieve long-term survival with ICIs, the significance of chronic irAEs is increasingly recognized. Chronic irAEs, defined as lasting beyond 3 months after treatment discontinuation, affect 43% of patients in certain studies, with mild cases being most common (87, 88). These chronic events include endocrinopathies, arthritis, xerostomia, neurotoxicities, and ocular issues, and are often irreversible (88). Though these irAEs can impact quality of life, particularly in long-term survivors, they are underreported in clinical trials.
Fatal irAEs, though rare, occur in up to 2% of patients, with severe cases like myocarditis and pneumonitis being most lethal (89). Additionally, long-term cardiovascular risks, such as increased aortic plaque volume and higher incidence of myocardial infarction, are emerging as significant concerns (90). Studies suggest T-cell mediated inflammation may drive ICI-related atherosclerosis, highlighting the need for proactive cardiovascular surveillance and management strategies for ICI-treated patients (91, 92).
5.3 Management of irAE
While ICIs offer promising benefits in treating GTN, the potential for irAEs presents a significant challenge to treatment. Grade 3 or higher irAEs generally require immunosuppressive treatment. The first-line treatment is usually discontinuation of the ICI therapy, followed by the administration of corticosteroids (93–95). High-dose corticosteroids are generally effective and are typically initiated at doses ranging from 0.5 to 2 mg/kg of prednisolone (93–95). In cases of severe irAEs, such as myocarditis or pneumonitis, higher doses of corticosteroids, including methylprednisolone (1,000 mg/day), may be required (93–95). Steroid treatment usually leads to symptom improvement within days, and after resolution, steroids are gradually tapered to minimize long-term side effects (96). However, the prolonged use of corticosteroids carries its own risks, such as increased susceptibility to infections and potential effects on tumor immunity. For patients who do not respond to corticosteroids or experience relapse of symptoms, second-line immunosuppressive agents may be required. These include biologics such as infliximab (anti-TNF-α) for gastrointestinal irAEs like colitis (96), and other immunosuppressive agents like methotrexate for managing severe autoimmune reactions (97). The choice of second-line therapy is often guided by the severity of the irAE and requires careful monitoring.
When these treatments effectively manage irAEs, they also pose a risk of impairing the anti-tumor immune response. Some studies indicate that low-grade irAEs may provide a more pronounced survival benefit, while high-grade irAEs, often requiring immunosuppressive treatment, might compromise the efficacy of ICIs (98, 99). The effects of combined immunosuppression and ICIs are inconsistent due to study design variations. For example, tumor necrosis factor (TNF) blockade may enhance ICI efficacy by supporting tumor-infiltrating lymphocyte survival (100, 101), while IL-6 receptor blockade could induce remission without impairing anti-tumor immunity (100, 102, 103). However, corticosteroids and second-line immunosuppressants might reduce ICI effectiveness (104). While ongoing clinical studies aim to provide clarity, current research is often limited by insufficient statistical power to accurately assess ICI responses. To optimize outcomes, strategies involving meticulous monitoring, personalized treatment plans, and regular organ function evaluations are essential for maintaining patient safety and maximizing therapeutic efficacy.
6 ICIs on fertility
As GTN primarily affects patients of childbearing age, and hysterectomy is generally not required for types other than PSTT and ETT, preserving fertility should be considered before planning treatment. Therefore, understanding the potential impact of immunotherapy on patients’ fertility and fetal health is particularly important.
6.1 Impact of ICIs on reproductive health
ICIs alter immune responses by reactivating T cells to target cancer cells. However, this broad immune activation can have unintended consequences on gonadal function. Preclinical studies have shown that ICIs increase immune cell infiltration and cytokine levels, such as TNF-α and IL-1β, within ovarian tissues, leading to follicular atresia in mouse models (105). Furthermore, ICIs disrupt the hypothalamic-pituitary-gonadal axis through endocrine irAEs such as hypophysitis, which can result in reduced gonadotropin levels and secondary hypogonadism (106). The PD-1/PD-L1 and CTLA-4 pathways, essential for maternal-fetal tolerance, are also implicated in reproductive health. By blocking these checkpoints, ICIs interfere with immune-regulatory mechanisms essential for implantation and pregnancy maintenance, thereby increasing risks of implantation failure and pregnancy complications (107). Animal studies reinforce these findings, showing that treatment with anti-PD-L1 antibodies or nivolumab in pregnant mice and cynomolgus monkeys led to significantly higher abortion rates and premature neonatal deaths, primarily through the depletion of regulatory T cells (108).
6.2 Outcomes of ICIs use during pregnancy
Clinical data further illustrate the complexities of using ICIs during pregnancy. Seven case reports document outcomes of women who either conceived while on ICIs or initiated treatment during pregnancy, including two twin pregnancies (52, 109–114). These cases predominantly involved patients with metastatic melanoma, with one exception being a PSTT case treated with pembrolizumab. Treatments included nivolumab (alone or with ipilimumab), pembrolizumab, and ipilimumab with intralesional IL-2. Pregnancy complications were notable, with four cases of intrauterine growth restriction or placental insufficiency leading to preterm caesarean sections (109–112). Among nine neonates, one exhibited transient congenital hypothyroidism, and another was born without a left hand, because of strangulation by the amniotic cord unrelated to the immunotherapy (110). At follow-up (6 weeks to 2.75 years), all children were healthy with normal developmental milestones. Maternal outcomes varied; while three mothers experienced disease progression (111, 113, 114), the remaining four patients achieved remission or stable disease during pregnancy. These cases underscore the challenges of balancing cancer treatment with pregnancy management, showing generally favorable neonatal health but variable maternal outcomes.
6.3 Post-treatment fertility and pregnancy outcomes after ICIs
Building on these findings, a recent retrospective cohort study further examined the post-treatment impact of ICIs on fertility and pregnancy outcomes in 53 reproductive-age patients treated for various cancers (115). Among these, 15% (8 patients) successfully conceived post-treatment after a median interval of 10.5 months (range 2-30 months), highlighting the possibility of fertility preservation despite prior immunotherapy. Of these pregnancies, five were carried to term, with minimal complications; however, one patient experienced a first-trimester miscarriage followed by a successful pregnancy. Maternal complications included cases of gestational diabetes and preeclampsia, attributed to pre-existing risk factors rather than ICIs. Importantly, all neonates were born healthy, with normal growth and developmental milestones reported during follow-ups. Beyond pregnancy outcomes, the study also explored ICI-induced endocrine disruptions, noting a significant decline in anti-Müllerian hormone (AMH) levels in one patient, suggesting potential long-term impacts on ovarian reserve. Preclinical evidence aligns with these findings, demonstrating ICI-associated gonadotoxicity, including immune cell infiltration, cytokine-mediated ovarian damage, and diminished follicular reserves in animal models (105, 116, 117).
6.4 Fertility preservation strategies in patients receiving ICIs
Given the potential risks to fertility associated with ICI therapy, fertility preservation has become an essential consideration for reproductive-age patients. Cryopreservation remains the cornerstone of fertility preservation strategies (118–120). Oocyte and embryo freezing, typically performed after controlled ovarian stimulation, are the most established methods. For patients who require immediate cancer treatment and cannot delay therapy, ovarian tissue cryopreservation offers a viable alternative (118–120). Pharmacological approaches, such as gonadotropin-releasing hormone agonists (GnRHa), have been employed to suppress ovarian function and potentially reduce gonadotoxicity during chemotherapy. However, their efficacy in the context of ICI therapy is not yet well established, requiring further research to determine their protective role. Emerging techniques, such as in vitro follicle maturation (IVFM) and regenerative therapies like mesenchymal stem cell-derived exosomes, show promise for restoring ovarian function and repairing gonadal damage caused by immunotherapy (120). These advancements, alongside comprehensive fertility counseling and a multidisciplinary approach involving oncologists, endocrinologists, and reproductive specialists, are essential for optimizing fertility preservation strategies and aligning treatment plans with patients’ reproductive goals.
7 Summary and future prospects
Immunotherapy has emerged as a transformative approach in the treatment of GTN, particularly for patients with chemoresistant or high-risk disease. Recent trials, such as the TROPHIMMUN study, have demonstrated the potential of ICIs like avelumab in low-risk GTN patients resistant to MTX, with promising outcomes including a 53% CR rate. Similarly, the CAP01 study highlighted the efficacy of combining camrelizumab with apatinib, achieving a CR rate of 50% in patients with chemorefractory GTN. These results underscore the potential of ICIs, both as monotherapies and it combining with other therapeutic agents, to overcome the challenges of chemoresistance in GTN.
Looking forward, several ongoing clinical trials are further investigating the role of ICIs in GTN (Table 2), with a particular focus on combination therapies. The RESOLVE study (NCT05635344) warrants further discussion as an example of attempts to use immunotherapy earlier in the disease course and limit exposure to chemotherapy. The TROPHAMET Phase I/II trial (NCT04396223) is examining the combination of avelumab with MTX as a first-line treatment for low-risk GTN. This study aims to determine the effectiveness of this combination in treating chemotherapy-resistant cases of GTN. The study, reported at ESMO 2024, has shown promising early results, with a high rate of successful hCG normalization, indicating that the combination of avelumab and MTX might improve treatment outcomes compared to single-agent chemotherapy. With a median follow-up of 24.8 months, the trial reports a 96.2% success rate in achieving hCG normalization and suggests that this combination therapy may provide long-term remission for low-risk GTN patients. Similarly, the CR-GTP trial (NCT04303884) is assessing the clinical efficacy of pembrolizumab in patients with GTN resistant to multi-agent chemotherapy.
Two other studies are evaluating the combination of Toripalimab and ActD in GTN patients with FIGO scores of 5-6(NCT06028672) and 7(NCT06020755), respectively, offering new possibilities for integrating ICIs into standard regimens. Another combination therapy study (NCT04812002) focuses on patients with relapsed or refractory high-risk GTN who have previously undergone second-line or higher chemotherapy. The study aims to evaluate the PFS in patients receiving PD-1 inhibitors in combination with bevacizumab. Additionally, the combination of camrelizumab and apatinib is currently being tested in a CAP01for patients with ultra-high-risk or chemorefractory GTN, following encouraging results from earlier studies. This trial (NCT05139095), which started in January 2022, is expected to provide valuable insights into the potential of combining ICIs with anti-angiogenic therapies in managing aggressive forms of GTN.
Biomarker-driven approaches are also gaining traction. Advances in molecular profiling have identified potential predictive biomarkers such as PD-L1, TMB, MSI expression, and TILs. Furthermore, next-generation sequencing of GTN subtypes like choriocarcinoma, PSTT and ETT is uncovering specific genetic mutations that may guide personalized immunotherapy. Challenges remain, including the need for more robust clinical evidence and the management of irAEs. The high cost of ICIs and accessibility issues also limit their widespread adoption. Future clinical trials must address these barriers while assessing long-term outcomes such as PFS, OS, and fertility preservation.
In conclusion, while immunotherapy, particularly ICIs, shows great promise in treating GTN, there is still much to learn. Ongoing clinical trials and international collaborations will be critical in advancing our understanding of how to optimize immunotherapy for GTN, overcoming current limitations, and ensuring that these therapies are accessible and effective for a broader range of patients. As more data emerges, it is likely that immunotherapy will become an integral part of the treatment paradigm for GTN, alongside conventional chemotherapy and surgery, improving survival and quality of life for patients with this rare and challenging disease.
Author contributions
JZe: Conceptualization, Funding acquisition, Validation, Writing – review & editing, Data curation, Investigation, Methodology, Project administration, Software, Writing – original draft. JZh: Data curation, Project administration, Software, Visualization, Writing – original draft, Writing – review & editing. JW: Data curation, Project administration, Writing – original draft, Writing – review & editing. LX: Data curation, Project administration, Writing – original draft, Writing – review & editing. CW: Conceptualization, Supervision, Validation, Visualization, Writing – original draft, Writing – review & editing. RY: Conceptualization, Funding acquisition, Supervision, Validation, Writing – original draft, Writing – review & editing.
Funding
The author(s) declare that financial support was received for the research and/or publication of this article. This study was supported by the National Natural Science Foundation of China (No. 81902649) and the Horizontal Science and Technology Project of Sichuan University (22H1536, 23H1223).
Conflict of interest
The authors declare that the research was conducted in the absence of any commercial or financial relationships that could be construed as a potential conflict of interest.
Generative AI statement
The author(s) declare that no Generative AI was used in the creation of this manuscript.
Publisher’s note
All claims expressed in this article are solely those of the authors and do not necessarily represent those of their affiliated organizations, or those of the publisher, the editors and the reviewers. Any product that may be evaluated in this article, or claim that may be made by its manufacturer, is not guaranteed or endorsed by the publisher.
References
1. Ngan HYS, Seckl MJ, Berkowitz RS, Xiang Y, Golfier F, Sekharan PK, et al. Diagnosis and management of gestational trophoblastic disease: 2021 update. Int J Gynecology Obstetrics. (2021) 155:86–93. doi: 10.1002/ijgo.13877
2. Kohorn EI. The new FIGO 2000 staging and risk factor scoring system for gestational trophoblastic disease: Description and critical assessment. Int J Gynecological Cancer. (2001) 11:73–7. doi: 10.1046/j.1525-1438.2001.011001073.x
3. Kong Y, Yang J, Jiang F, Zhao J, Ren T, Li J, et al. Clinical characteristics and prognosis of ultra high-risk gestational trophoblastic neoplasia patients: A retrospective cohort study. Gynecologic Oncol. (2017) 146:81–6. doi: 10.1016/j.ygyno.2017.04.010
4. Balachandran K, Salawu A, Ghorani E, Kaur B, Sebire NJ, Short D, et al. When to stop human chorionic gonadotrophin (hCG) surveillance after treatment with chemotherapy for gestational trophoblastic neoplasia (GTN): A national analysis on over 4,000 patients. Gynecologic Oncol. (2019) 155:8–12. doi: 10.1016/j.ygyno.2019.07.024
5. Kong Y, Zong L, Cheng H, Jiang F, Wan X, Feng F, et al. Management and risk factors of recurrent gestational trophoblastic neoplasia: An update from 2004 to 2017. Cancer Med. (2020) 9:2590–9. doi: 10.1002/cam4.2901
6. Braga A, Mora P, Melo ACD, Nogueira-Rodrigues A, Amim-Junior J, Rezende-Filho J, et al. Challenges in the diagnosis and treatment of gestational trophoblastic neoplasia worldwide. World J Clin Oncol. (2019) 10:28–37. doi: 10.5306/wjco.v10.i2.28
7. Bolze PA, Mathe M, Hajri T, You B, Dabi Y, Schott AM, et al. First-line hysterectomy for women with low-risk non-metastatic gestational trophoblastic neoplasia no longer wishing to conceive. Gynecologic Oncol. (2018) 150:282–7. doi: 10.1016/j.ygyno.2018.05.030
8. Lok C, Van Trommel N, Massuger L, Golfier F, Seckl M, Abreu MH, et al. Practical clinical guidelines of the EOTTD for treatment and referral of gestational trophoblastic disease. Eur J Cancer. (2020) 130:228–40. doi: 10.1016/j.ejca.2020.02.011
9. Braga A, Paiva G, Ghorani E, Freitas F, Velarde LGC, Kaur B, et al. Predictors for single-agent resistance in FIGO score 5 or 6 gestational trophoblastic neoplasia: a multicentre, retrospective, cohort study. Lancet Oncol. (2021) 22:1188–98. doi: 10.1016/S1470-2045(21)00262-X
10. Sita-Lumsden A, Short D, Lindsay I, Sebire NJ, Adjogatse D, Seckl MJ, et al. Treatment outcomes for 618 women with gestational trophoblastic tumours following a molar pregnancy at the Charing Cross Hospital, 2000–2009. Br J Cancer. (2012) 107:1810–4. doi: 10.1038/bjc.2012.462
11. Alifrangis C, Agarwal R, Short D, Fisher RA, Sebire NJ, Harvey R, et al. EMA/CO for high-risk gestational trophoblastic neoplasia: good outcomes with induction low-dose etoposide-cisplatin and genetic analysis. J Clin Oncol. (2013) 31:280–6. doi: 10.1200/JCO.2012.43.1817
12. Chan Wah Hak C, Coyle C, Kocache A, Short D, Sarwar N, Seckl MJ, et al. Emergency Etoposide-Cisplatin (Em-EP) for patients with germ cell tumours (GCT) and trophoblastic neoplasia (TN). BMC Cancer. (2019) 19:770. doi: 10.1186/s12885-019-5968-7
13. Ngan HYS, Seckl MJ, Berkowitz RS, Xiang Y, Golfier F, Sekharan PK, et al. Update on the diagnosis and management of gestational trophoblastic disease. Int J Gynecology Obstetrics. (2018) 143:79–85. doi: 10.1002/ijgo.12615
14. Sato S, Yamamoto E, Niimi K, Ino K, Nishino K, Suzuki S, et al. The efficacy and toxicity of 4-day chemotherapy with methotrexate, etoposide and actinomycin D in patients with choriocarcinoma and high-risk gestational trophoblastic neoplasia. Int J Clin Oncol. (2020) 25:203–9. doi: 10.1007/s10147-019-01540-9
15. Lurain JR. Gestational trophoblastic disease II: classification and management of gestational trophoblastic neoplasia. Am J Obstetrics Gynecology. (2011) 204:11–8. doi: 10.1016/j.ajog.2010.06.072
16. Seckl MJ, Sebire NJ, Berkowitz RS. Gestational trophoblastic disease. Lancet. (2010) 376:717–29. doi: 10.1016/S0140-6736(10)60280-2
17. Schmid P, Nagai Y, Agarwal R, Hancock B, Savage PM, Sebire NJ, et al. Prognostic markers and long-term outcome of placental-site trophoblastic tumours: a retrospective observational study. Lancet. (2009) 374:48–55. doi: 10.1016/S0140-6736(09)60618-8
18. Horowitz NS, Goldstein DP, Berkowitz RS. Placental site trophoblastic tumors and epithelioid trophoblastic tumors: Biology, natural history, and treatment modalities. Gynecologic Oncol. (2017) 144:208–14. doi: 10.1016/j.ygyno.2016.10.024
19. Froeling FEM, Ramaswami R, Papanastasopoulos P, Kaur B, Sebire NJ, Short D, et al. Intensified therapies improve survival and identification of novel prognostic factors for placental-site and epithelioid trophoblastic tumours. Br J Cancer. (2019) 120:587–94. doi: 10.1038/s41416-019-0402-0
20. Matthews BJ, Froehlich A, Goicochea L, Levinson K. Postmenopausal mixed gestational trophoblastic neoplasia with complete response to immunotherapy – A case report. Gynecologic Oncol Rep. (2023) 46:101168. doi: 10.1016/j.gore.2023.101168
21. Szabolcsi Z, Demeter A, Kiraly P, Balogh A, Wilson ML, King JR, et al. Epigenetic dysregulation of trophoblastic gene expression in gestational trophoblastic disease. Biomedicines. (2021) 9:1935. doi: 10.3390/biomedicines9121935
22. Burkett WC, Soper JT. A review of current management of placental site trophoblastic tumor and epithelioid trophoblastic tumor. Obstetrical Gynecological Survey. (2022) 77:101–10. doi: 10.1097/OGX.0000000000000978
23. Shih IM, Kurman RJ. The pathology of intermediate trophoblastic tumors and tumor-like lesions. Int J Gynecological Pathology. (2001) 20:31–47. doi: 10.1097/00004347-200101000-00004
24. Lala PK, Nandi P, Hadi A, Halari C. A crossroad between placental and tumor biology: What have we learnt? Placenta. (2021) 116:12–30. doi: 10.1016/j.placenta.2021.03.003
25. Fanoni D, Tavecchio S, Recalcati S, Balice Y, Venegoni L, Fiorani R, et al. New monoclonal antibodies against B-cell antigens: Possible new strategies for diagnosis of primary cutaneous B-cell lymphomas. Immunol Letters. (2011) 134:157–60. doi: 10.1016/j.imlet.2010.09.022
26. Inaguma S, Wang Z, Lasota J, Sarlomo-Rikala M, McCue PA, Ikeda H, et al. Comprehensive immunohistochemical study of programmed cell death ligand 1 (PD-L1): analysis in 5536 cases revealed consistent expression in trophoblastic tumors. Am J Surg Pathology. (2016) 40:1133–42. doi: 10.1097/PAS.0000000000000653
27. Terme M, Ullrich E, Aymeric L, Meinhardt K, Desbois M, Delahaye N, et al. IL-18 induces PD-1–dependent immunosuppression in cancer. Cancer Res. (2011) 71:5393–9. doi: 10.1158/0008-5472.CAN-11-0993
28. Ni L, Dong C. New B7 family checkpoints in human cancers. Mol Cancer Ther. (2017) 16:1203–11. doi: 10.1158/1535-7163.MCT-16-0761
29. Veras E, Kurman RJ, Wang TL, Shih IM. PD-L1 expression in human placentas and gestational trophoblastic diseases. Int J Gynecological Pathology. (2017) 36:146–53. doi: 10.1097/PGP.0000000000000305
30. Ghorani E, Kaur B, Fisher RA, Short D, Joneborg U, Carlson JW, et al. Pembrolizumab is effective for drug-resistant gestational trophoblastic neoplasia. Lancet. (2017) 390:2343–5. doi: 10.1016/S0140-6736(17)32894-5
31. Choi MC, Oh J, Lee C. Effective anti-programmed cell death 1 treatment for chemoresistant gestational trophoblastic neoplasia. Eur J Cancer. (2019) 121:94–7. doi: 10.1016/j.ejca.2019.08.024
32. Lu B, Teng X, Fu G, Bao L, Tang J, Shi H, et al. Analysis of PD-L1 expression in trophoblastic tissues and tumors. Hum Pathology. (2019) 84:202–12. doi: 10.1016/j.humpath.2018.10.001
33. Fukumura D, Kloepper J, Amoozgar Z, Duda DG, Jain RK. Enhancing cancer immunotherapy using antiangiogenics: opportunities and challenges. Nat Rev Clin Oncol. (2018) 15:325–40. doi: 10.1038/nrclinonc.2018.29
34. Song Y, Fu Y, Xie Q, Zhu B, Wang J, Zhang B. Anti-angiogenic agents in combination with immune checkpoint inhibitors: A promising strategy for cancer treatment. Front Immunol. (2020) 11:1956. doi: 10.3389/fimmu.2020.01956
35. Hatanaka K, Higashi M, Fujibayashi M, Hori S, Yoshioka T, Umekita Y, et al. A case of complete hydatidiform mole in a perimenopausal woman with diagnostic usefulness of p57kip2 immunohistochemistry and HER2 fluorescent in situ hybridization. Pathol - Res Practice. (2012) 208:118–20. doi: 10.1016/j.prp.2011.11.005
36. Pires LV, Yi Y, Cheng JC, Pizzolato LS, Cordero E, Leung PCK, et al. Lapatinib inhibits amphiregulin-induced beWo choriocarcinoma cell proliferation by reducing ERK1/2 and AKT signaling pathways. Anticancer Res. (2019) 39:2377–83. doi: 10.21873/anticanres.13355
37. You B, Bolze PA, Lotz JP, Massardier J, Gladieff L, Joly F, et al. Avelumab in patients with gestational trophoblastic tumors with resistance to single-agent chemotherapy: cohort A of the TROPHIMMUN phase II trial. J Clin Oncol. (2020) 38:3129–37. doi: 10.1200/JCO.20.00803
38. You B, Bolze PA, Lotz JP, Massardier J, Gladieff L, Floquet A, et al. cohortB GO Avelumab in patients with gestational trophoblastic tumors with resistance to polychemotherapy: Cohort B of the TROPHIMMUN phase 2 trial. Gynecologic Oncol. (2023) 168:62–7. doi: 10.1016/j.ygyno.2022.11.005
39. Lurain JR, Chapman-Davis E, Hoekstra AV, Schink JC. Actinomycin D for methotrexate-failed low-risk gestational trophoblastic neoplasia. J Reprod Med. (2012) 57:283–7.
40. Prouvot C, Golfier F, Massardier J, You B, Lotz JP, Patrier S, et al. Efficacy and safety of second-line 5-day dactinomycin in case of methotrexate failure for gestational trophoblastic neoplasia. Int J Gynecologic Cancer. (2018) 28:1038–44. doi: 10.1097/IGC.0000000000001248
41. Hennah L, Seckl M, Ghorani E. Novel approaches to managing gestational trophoblastic tumors in the age of immunotherapy. Int J Gynecological Cancer. (2023) 33:414–9. doi: 10.1136/ijgc-2022-003771
42. Huang M, Pinto A, Castillo RP, Slomovitz BM. Complete serologic response to pembrolizumab in a woman with chemoresistant metastatic choriocarcinoma. J Clin Oncol. (2017) 35:3172–4. doi: 10.1200/JCO.2017.74.4052
43. Clair KH, Gallegos N, Bristow RE. Successful treatment of metastatic refractory gestational choriocarcinoma with pembrolizumab: A case for immune checkpoint salvage therapy in trophoblastic tumors. Gynecologic Oncol Rep. (2020) 34:100625. doi: 10.1016/j.gore.2020.100625
44. Paspalj V, Polterauer S, Poetsch N, Reinthaller A, Grimm C, Bartl T. Long-term survival in multiresistant metastatic choriocarcinoma after pembrolizumab treatment: A case report. Gynecologic Oncol Rep. (2021) 37:100817. doi: 10.1016/j.gore.2021.100817
45. Baas IO, Westermann AM, You B, Bolze PA, Seckl M, Ghorani E. Immunotherapy for gestational trophoblastic neoplasia: A new paradigm. Gynecol Obstet Invest. (2024) 89:230–8. doi: 10.1159/000533972
46. Duan J, Cui L, Zhao X, Bai H, Cai S, Wang G, et al. Use of immunotherapy with programmed cell death 1 vs programmed cell death ligand 1 inhibitors in patients with cancer: A systematic review and meta-analysis. JAMA Oncol. (2020) 6:375. doi: 10.1001/jamaoncol.2019.5367
47. Cheng H, Zong L, Kong Y, Wang X, Gu Y, Cang W, et al. Camrelizumab plus apatinib in patients with high-risk chemorefractory or relapsed gestational trophoblastic neoplasia (CAP 01): a single-arm, open-label, phase 2 trial. Lancet Oncol. (2021) 22:1609–17. doi: 10.1016/S1470-2045(21)00460-5
48. Wang X, Cang W, Liu X, Cheng Y, Wan X, Feng F, et al. Anti-PD-1 therapy plus chemotherapy versus anti-PD-1 therapy alone in patients with high-risk chemorefractory or relapsed gestational trophoblastic neoplasia: a multicenter, retrospective study. eClinicalMedicine. (2023) 59:101974. doi: 10.1016/j.eclinm.2023.101974
49. Frijstein MM, Lok CAR, Van Trommel NE, Ten Kate-Booij MJ, Massuger LFAG, Van Werkhoven E, et al. Management and prognostic factors of epithelioid trophoblastic tumors: Results from the International Society for the Study of Trophoblastic Diseases database. Gynecologic Oncol. (2019) 152:361–7. doi: 10.1016/j.ygyno.2018.11.015
50. Bell SG, Uppal S, Sakala MD, Sciallis AP, Rolston A. An extrauterine extensively metastatic epithelioid trophoblastic tumor responsive to pembrolizumab. Gynecologic Oncol Rep. (2021) 37:100819. doi: 10.1016/j.gore.2021.100819
51. Pisani D, Calleja-Agius J, Di Fiore R, O’Leary JJ, Beirne JP, O’Toole SA, et al. Epithelioid trophoblastic tumour: A case with genetic linkage to a child born over seventeen years prior, successfully treated with surgery and pembrolizumab. Curr Oncol. (2021) 28:5346–55. doi: 10.3390/curroncol28060446
52. Polnaszek B, Mullen M, Bligard K, Raghuraman N, Massad LS. Term pregnancy after complete response of placental site trophoblastic tumor to immunotherapy. Obstetrics Gynecology. (2021) 138:115–8. doi: 10.1097/AOG.0000000000004434
53. Balar AV, Castellano D, O’Donnell PH, Grivas P, Vuky J, Powles T, et al. First-line pembrolizumab in cisplatin-ineligible patients with locally advanced and unresectable or metastatic urothelial cancer (KEYNOTE-052): a multicentre, single-arm, phase 2 study. Lancet Oncol. (2017) 18:1483–92. doi: 10.1016/S1470-2045(17)30616-2
54. Leighl NB, Hellmann MD, Hui R, Carcereny E, Felip E, Ahn MJ, et al. Pembrolizumab in patients with advanced non-small-cell lung cancer (KEYNOTE-001): 3-year results from an open-label, phase 1 study. Lancet Respir Med. (2019) 7:347–57. doi: 10.1016/S2213-2600(18)30500-9
55. Marabelle A, Fakih M, Lopez J, Shah M, Shapira-Frommer R, Nakagawa K, et al. Association of tumour mutational burden with outcomes in patients with advanced solid tumours treated with pembrolizumab: prospective biomarker analysis of the multicohort, open-label, phase 2 KEYNOTE-158 study. Lancet Oncol. (2020) 21:1353–65. doi: 10.1016/S1470-2045(20)30445-9
56. Wang X, Lamberti G, Di Federico A, Alessi J, Ferrara R, Sholl ML, et al. Tumor mutational burden for the prediction of PD-(L)1 blockade efficacy in cancer: challenges and opportunities. Ann Oncol. (2024) 35:508–22. doi: 10.1016/j.annonc.2024.03.007
57. McNally L, Wu S, Hodges K, Oberley M, Wallbillich JJ, Jones NL, et al. Molecular profiling of gestational trophoblastic neoplasia: Identifying therapeutic targets. Gynecologic Oncol. (2024) 184:111–6. doi: 10.1016/j.ygyno.2024.01.033
58. Boland CR, Goel A. Microsatellite instability in colorectal cancer. Gastroenterology. (2010) 138:2073–2087.e3. doi: 10.1053/j.gastro.2009.12.064
59. Findeisen P, Kloor M, Merx S, Sutter C, Woerner SM, Dostmann N, et al. T25 repeat in the 3′ Untranslated region of the CASP2 gene: A sensitive and specific marker for microsatellite instability in colorectal cancer. Cancer Res. (2005) 65:8072–8. doi: 10.1158/0008-5472.CAN-04-4146
60. Maby P, Tougeron D, Hamieh M, Mlecnik B, Kora H, Bindea G, et al. Correlation between density of CD8+ T-cell infiltrate in microsatellite unstable colorectal cancers and frameshift mutations: A rationale for personalized immunotherapy. Cancer Res. (2015) 75:3446–55. doi: 10.1158/0008-5472.CAN-14-3051
61. Phillips SM, Banerjea A, Feakins R, Li SR, Bustin SA, Dorudi S. Tumour-infiltrating lymphocytes in colorectal cancer with microsatellite instability are activated and cytotoxic. Br J Surgery. (2004) 91:469–75. doi: 10.1002/bjs.4472
62. Schwitalle Y, Kloor M, Eiermann S, Linnebacher M, Kienle P, Knaebel HP, et al. Immune response against frameshift-induced neopeptides in HNPCC patients and healthy HNPCC mutation carriers. Gastroenterology. (2008) 134:988–97. doi: 10.1053/j.gastro.2008.01.015
63. Le DT, Uram JN, Wang H, Bartlett BR, Kemberling H, Eyring AD, et al. PD-1 blockade in tumors with mismatch-repair deficiency. New Engl J Med. (2015) 372:2509–20. doi: 10.1056/NEJMoa1500596
64. Diaz LA, Shiu KK, Kim TW, Jensen BV, Jensen LH, Punt C, et al. Pembrolizumab versus chemotherapy for microsatellite instability-high or mismatch repair-deficient metastatic colorectal cancer (KEYNOTE-177): final analysis of a randomised, open-label, phase 3 study. Lancet Oncol. (2022) 23:659–70. doi: 10.1016/S1470-2045(22)00197-8
65. Overman MJ, McDermott R, Leach JL, Lonardi S, Lenz HJ, Morse MA, et al. Nivolumab in patients with metastatic DNA mismatch repair-deficient or microsatellite instability-high colorectal cancer (CheckMate 142): an open-label, multicentre, phase 2 study. Lancet Oncol. (2017) 18:1182–91. doi: 10.1016/S1470-2045(17)30422-9
66. Zou XL, Li XB, Ke H, Zhang GY, Tang Q, Yuan J, et al. Prognostic value of neoantigen load in immune checkpoint inhibitor therapy for cancer. Front Immunol. (2021) 12:689076. doi: 10.3389/fimmu.2021.689076
67. Chen DS, Mellman I. Oncology meets immunology: the cancer-immunity cycle. Immunity. (2013) 39:1–10. doi: 10.1016/j.immuni.2013.07.012
68. Hackl H, Charoentong P, Finotello F, Trajanoski Z. Computational genomics tools for dissecting tumour–immune cell interactions. Nat Rev Genet. (2016) 17:441–58. doi: 10.1038/nrg.2016.67
69. Wang X, Muzaffar J, Kirtane K, Song F, Johnson M, Schell MJ, et al. T cell repertoire in peripheral blood as a potential biomarker for predicting response to concurrent cetuximab and nivolumab in head and neck squamous cell carcinoma. J ImmunoTherapy Cancer. (2022) 10:e004512. doi: 10.1136/jitc-2022-004512
70. Sharma P, Hu-Lieskovan S, Wargo JA, Ribas A. Primary, adaptive, and acquired resistance to cancer immunotherapy. Cell. (2017) 168:707–23. doi: 10.1016/j.cell.2017.01.017
71. Chan SWS, Shukla R, Ramsay J, McWhirter E, Barnfield P, Juergens RA. Immune-checkpoint induced skin toxicity masked as squamous cell carcinoma: case report on mimickers of dermatological toxicity with PD-1 inhibition. Curr Oncol. (2023) 30:4527–37. doi: 10.3390/curroncol30050342
72. Cheng S, Yang Y, Yu J, Chen W, Li X. Immune-related colitis induced by camrelizumab: A case report. J Inflammation Res. (2023) 16:1727–31. doi: 10.2147/JIR.S405023
73. Altan M, Soto F, Xu T, Wilson N, Franco-Vega MC, Simbaqueba Clavijo CA, et al. Pneumonitis after concurrent chemoradiation and immune checkpoint inhibition in patients with locally advanced non-small cell lung cancer. Clin Oncol. (2023) 35:630–9. doi: 10.1016/j.clon.2023.07.003
74. De Filette JMK, André S, De Mey L, Aspeslagh S, Karmali R, van der Auwera BJ, et al. Durvalumab-induced thyroiditis in a patient with non-small cell lung carcinoma: a case report and review of pathogenic mechanisms. BMC Endocrine Disord. (2022) 22:291. doi: 10.1186/s12902-022-01190-5
75. Johnson DB, Manouchehri A, Haugh AM, Quach HT, Balko JM, Lebrun-Vignes B, et al. Neurologic toxicity associated with immune checkpoint inhibitors: a pharmacovigilance study. J ImmunoTherapy Cancer. (2019) 7:134. doi: 10.1186/s40425-019-0617-x
76. Hountondji L, Ferreira De Matos C, Lebossé F, Quantin X, Lesage C, Palassin P, et al. Clinical pattern of checkpoint inhibitor-induced liver injury in a multicentre cohort. JHEP Rep. (2023) 5:100719. doi: 10.1016/j.jhepr.2023.100719
77. Bobircă A, Bobircă F, Ancuta I, Florescu A, Pădureanu V, Florescu DN, et al. Rheumatic immune-related adverse events—A consequence of immune checkpoint inhibitor therapy. Biology. (2021) 10:561. doi: 10.3390/biology10060561
78. Ratanasrimetha P, Reddy VD, Kala J, Tchakarov A, Glass WF, Msaouel P, et al. Case Report: Successful treatment of late-onset immune checkpoint inhibitor-associated membranous nephropathy in a patient with advanced renal cell carcinoma. Front Immunol. (2022) 13:898811. doi: 10.3389/fimmu.2022.898811
79. Pi JK, Chen XT, Zhang YJ, Chen XM, Wang YC, Xu JY, et al. Insight of immune checkpoint inhibitor related myocarditis. Int Immunopharmacology. (2024) 143:113559. doi: 10.1016/j.intimp.2024.113559
80. Khoja L, Day D, Wei-Wu Chen T, Siu LL, Hansen AR. Tumour- and class-specific patterns of immune-related adverse events of immune checkpoint inhibitors: a systematic review. Ann Oncol. (2017) 28:2377–85. doi: 10.1093/annonc/mdx286
81. Curry JL, Tetzlaff MT, Nagarajan P, Drucker C, Diab A, Hymes SR, et al. Diverse types of dermatologic toxicities from immune checkpoint blockade therapy. J Cutaneous Pathology. (2017) 44:158–76. doi: 10.1111/cup.12858
82. Bamias G, Delladetsima I, Perdiki M, Siakavellas SI, Goukos D, Papatheodoridis GV, et al. Immunological characteristics of colitis associated with anti-CTLA-4 antibody therapy. Cancer Invest. (2017) 35:443–55. doi: 10.1080/07357907.2017.1324032
83. Wang Y, Abu-Sbeih H, Mao E, Ali N, Ali FS, Qiao W, et al. Immune-checkpoint inhibitor-induced diarrhea and colitis in patients with advanced Malignancies: retrospective review at MD Anderson. J ImmunoTherapy Cancer. (2018) 6:37. doi: 10.1186/s40425-018-0346-6
84. Chang LS, Barroso-Sousa R, Tolaney SM, Hodi FS, Kaiser UB, Min L. Endocrine toxicity of cancer immunotherapy targeting immune checkpoints. Endocrine Rev. (2019) 40:17–65. doi: 10.1210/er.2018-00006
85. Mizukoshi T, Fukuoka H, Takahashi Y. Immune checkpoint inhibitor-related hypophysitis. Best Pract Res Clin Endocrinol Metab. (2022) 36:101668. doi: 10.1016/j.beem.2022.101668
86. Jeun R, Iyer PC, Best C, Lavis V, Varghese JM, Yedururi S, et al. Clinical outcomes of immune checkpoint inhibitor diabetes mellitus at a comprehensive cancer center. Immunotherapy. (2023) 15:417–28. doi: 10.2217/imt-2021-0316
87. Naidoo J, Murphy C, Atkins MB, Brahmer JR, Champiat S, Feltquate D, et al. Society for Immunotherapy of Cancer (SITC) consensus definitions for immune checkpoint inhibitor-associated immune-related adverse events (irAEs) terminology. J ImmunoTherapy Cancer. (2023) 11:e006398. doi: 10.1136/jitc-2022-006398
88. Patrinely JR, Johnson R, Lawless AR, Bhave P, Sawyers A, Dimitrova M, et al. Chronic immune-related adverse events following adjuvant anti–PD-1 therapy for high-risk resected melanoma. JAMA Oncol. (2021) 7:744. doi: 10.1001/jamaoncol.2021.0051
89. Martins F, Sofiya L, Sykiotis GP, Lamine F, Maillard M, Fraga M, et al. Adverse effects of immune-checkpoint inhibitors: epidemiology, management and surveillance. Nat Rev Clin Oncol. (2019) 16:563–80. doi: 10.1038/s41571-019-0218-0
90. Drobni ZD, Alvi RM, Taron J, Zafar A, Murphy SP, Rambarat PK, et al. Association between immune checkpoint inhibitors with cardiovascular events and atherosclerotic plaque. Circulation. (2020) 142:2299–311. doi: 10.1161/CIRCULATIONAHA.120.049981
91. Suero-Abreu GA, Zanni MV, Neilan TG. Atherosclerosis with immune checkpoint inhibitor therapy. JACC: CardioOncology. (2022) 4:598–615. doi: 10.1016/j.jaccao.2022.11.011
92. Poels K, Van Leent MMT, Boutros C, Tissot H, Roy S, Meerwaldt AE, et al. Immune checkpoint inhibitor therapy aggravates T cell–driven plaque inflammation in atherosclerosis. JACC: CardioOncology. (2020) 2:599–610. doi: 10.1016/j.jaccao.2020.08.007
93. Haanen J, Obeid M, Spain L, Carbonnel F, Wang Y, Robert C, et al. Management of toxicities from immunotherapy: ESMO Clinical Practice Guideline for diagnosis, treatment and follow-up. Ann Oncol. (2022) 33:1217–38. doi: 10.1016/j.annonc.2022.10.001
94. Brahmer JR, Abu-Sbeih H, Ascierto PA, Brufsky J, Cappelli LC, Cortazar FB, et al. Society for Immunotherapy of Cancer (SITC) clinical practice guideline on immune checkpoint inhibitor-related adverse events. J ImmunoTherapy Cancer. (2021) 9:e002435. doi: 10.1136/jitc-2021-002435
95. Schneider BJ, Naidoo J, Santomasso BD, Lacchetti C, Adkins S, Anadkat M, et al. Management of immune-related adverse events in patients treated with immune checkpoint inhibitor therapy: ASCO guideline update. J Clin Oncol. (2021) 39:4073–126. doi: 10.1200/JCO.21.01440
96. Zou F, Faleck D, Thomas A, Harris J, Satish D, Wang X, et al. Efficacy and safety of vedolizumab and infliximab treatment for immune-mediated diarrhea and colitis in patients with cancer: a two-center observational study. J ImmunoTherapy Cancer. (2021) 9:e003277. doi: 10.1136/jitc-2021-003277
97. Bass AR, Abdel-Wahab N, Reid PD, Sparks JA, Calabrese C, Jannat-Khah DP, et al. Comparative safety and effectiveness of TNF inhibitors, IL6 inhibitors and methotrexate for the treatment of immune checkpoint inhibitor-associated arthritis. Ann Rheumatic Diseases. (2023) 82:920–6. doi: 10.1136/ard-2023-223885
98. Hussaini S, Chehade R, Boldt RG, Raphael J, Blanchette P, Maleki Vareki S, et al. Association between immune-related side effects and efficacy and benefit of immune checkpoint inhibitors – A systematic review and meta-analysis. Cancer Treat Rev. (2021) 92:102134. doi: 10.1016/j.ctrv.2020.102134
99. Verheijden RJ, Van Eijs MJM, May AM, Van Wijk F, Suijkerbuijk KPM. Immunosuppression for immune-related adverse events during checkpoint inhibition: an intricate balance. NPJ Precis Oncol. (2023) 7:41. doi: 10.1038/s41698-023-00380-1
100. Walsh MJ, Ali LR, Lenehan P, Kureshi CT, Kureshi R, Dougan M, et al. Blockade of innate inflammatory cytokines TNF α, IL-1 β, or IL-6 overcomes virotherapy-induced cancer equilibrium to promote tumor regression. Immunotherapy Adv. (2023) 3:ltad011. doi: 10.1093/immadv/ltad011
101. Perez-Ruiz E, Minute L, Otano I, Alvarez M, Ochoa MC, Belsue V, et al. Prophylactic TNF blockade uncouples efficacy and toxicity in dual CTLA-4 and PD-1 immunotherapy. Nature. (2019) 569:428–32. doi: 10.1038/s41586-019-1162-y
102. Fa’ak F, Buni M, Falohun A, Lu H, Song J, Johnson DH, et al. Selective immune suppression using interleukin-6 receptor inhibitors for management of immune-related adverse events. J ImmunoTherapy Cancer. (2023) 11:e006814. doi: 10.1136/jitc-2023-006814
103. Hailemichael Y, Johnson DH, Abdel-Wahab N, Foo WC, Bentebibel SE, Daher M, et al. Interleukin-6 blockade abrogates immunotherapy toxicity and promotes tumor immunity. Cancer Cell. (2022) 40:509–523.e6. doi: 10.1016/j.ccell.2022.04.004
104. Keam S, Turner N, Kugeratski FG, Rico R, Colunga-Minutti J, Poojary R, et al. Toxicity in the era of immune checkpoint inhibitor therapy. Front Immunol. (2024) 15:1447021. doi: 10.3389/fimmu.2024.1447021
105. Winship AL, Alesi LR, Sant S, Stringer JM, Cantavenera A, Hegarty T, et al. Checkpoint inhibitor immunotherapy diminishes oocyte number and quality in mice. Nat Cancer. (2022) 3:1–13. doi: 10.1038/s43018-022-00413-x
106. Garutti M, Lambertini M, Puglisi F. Checkpoint inhibitors, fertility, pregnancy, and sexual life: a systematic review. ESMO Open. (2021) 6:100276. doi: 10.1016/j.esmoop.2021.100276
107. Chen Z, Huang J, Kwak-Kim J, Wang W. Immune checkpoint inhibitors and reproductive failures. J Reprod Immunol. (2023) 156:103799. doi: 10.1016/j.jri.2023.103799
108. Özdemir BC. Immune checkpoint inhibitor-related hypogonadism and infertility: a neglected issue in immuno-oncology. J ImmunoTherapy Cancer. (2021) 9:e002220. doi: 10.1136/jitc-2020-002220
109. Xu W, Moor RJ, Walpole ET, Atkinson VG. Pregnancy with successful foetal and maternal outcome in a melanoma patient treated with nivolumab in the first trimester: case report and review of the literature. Melanoma Res. (2019) 29:333–7. doi: 10.1097/CMR.0000000000000586
110. Haiduk J, Ziemer M. Pregnancy in a patient with metastatic uveal melanoma treated with nivolumab. JDDG: J der Deutschen Dermatologischen Gesellschaft. (2021) 19:762–5. doi: 10.1111/ddg.14463
111. Burotto M, Gormaz JG, Samtani S, Valls N, Silva R, Rojas C, et al. Viable Pregnancy in a patient with metastatic melanoma treated with double checkpoint immunotherapy. Semin Oncol. (2018) 45:164–9. doi: 10.1053/j.seminoncol.2018.03.003
112. Bucheit AD, Hardy JT, Szender JB, Glitza Oliva IC. Conception and viable twin pregnancy in a patient with metastatic melanoma while treated with CTLA-4 and PD-1 checkpoint inhibition. Melanoma Res. (2020) 30:423–5. doi: 10.1097/CMR.0000000000000657
113. Mehta A, Kim KB, Minor DR. Case report of a pregnancy during ipilimumab therapy. J Global Oncol. (2018) 4):1–3. doi: 10.1200/JGO.17.00019
114. Menzer C, Beedgen B, Rom J, Duffert CM, Volckmar AL, Sedlaczek O, et al. Immunotherapy with ipilimumab plus nivolumab in a stage IV melanoma patient during pregnancy. Eur J Cancer. (2018) 104:239–42. doi: 10.1016/j.ejca.2018.09.008
115. Harrold EC, Ogle M, O’Brien E, Wilde C, Sinopoli J, Weiss J, et al. Fertility outcomes post immune checkpoint inhibitor exposure. Cancer Cell. (2024) 42:1808–10. doi: 10.1016/j.ccell.2024.10.005
116. Meggyes M, Miko E, Szigeti B, Farkas N, Szereday L. The importance of the PD-1/PD-L1 pathway at the maternal-fetal interface. BMC Pregnancy Childbirth. (2019) 19:74. doi: 10.1186/s12884-019-2218-6
117. Xu PC, Luan Y, Yu SY, Xu J, Coulter DW, Kim SY. Effects of PD-1 blockade on ovarian follicles in a prepubertal female mouse. J Endocrinology. (2022) 252:15–30. doi: 10.1530/JOE-21-0209
118. Angarita AM, Johnson CA, Fader AN, Christianson MS. Fertility preservation: A key survivorship issue for young women with cancer. Front Oncol. (2016) 6:102. doi: 10.3389/fonc.2016.00102
119. Antonouli S, Di Nisio V, Messini C, Daponte A, Rajender S, Anifandis G. A comprehensive review and update on human fertility cryopreservation methods and tools. Front Veterinary Science. (2023) 10:1151254. doi: 10.3389/fvets.2023.1151254
Keywords: gestational trophoblastic neoplasia, immune checkpoint inhibitors, chemoresistance, clinical trials, fertility preservation, immune-related adverse events
Citation: Zeng J, Zhang J, Wang J, Xu L, Wang C and Yin R (2025) Immunotherapy in gestational trophoblastic neoplasia: advances and future directions. Front. Immunol. 16:1544585. doi: 10.3389/fimmu.2025.1544585
Received: 13 December 2024; Accepted: 26 March 2025;
Published: 11 April 2025.
Edited by:
Kanjoormana Aryan Manu, Amala Cancer Research Centre, IndiaReviewed by:
Zhen Dong, Southwest University, ChinaEhsan Ghorani, Imperial College London, United Kingdom
Copyright © 2025 Zeng, Zhang, Wang, Xu, Wang and Yin. This is an open-access article distributed under the terms of the Creative Commons Attribution License (CC BY). The use, distribution or reproduction in other forums is permitted, provided the original author(s) and the copyright owner(s) are credited and that the original publication in this journal is cited, in accordance with accepted academic practice. No use, distribution or reproduction is permitted which does not comply with these terms.
*Correspondence: Cheng Wang, aHhleWNjQDEyNi5jb20=; Rutie Yin, eWlucnV0aWVAc2N1LmVkdS5jbg==