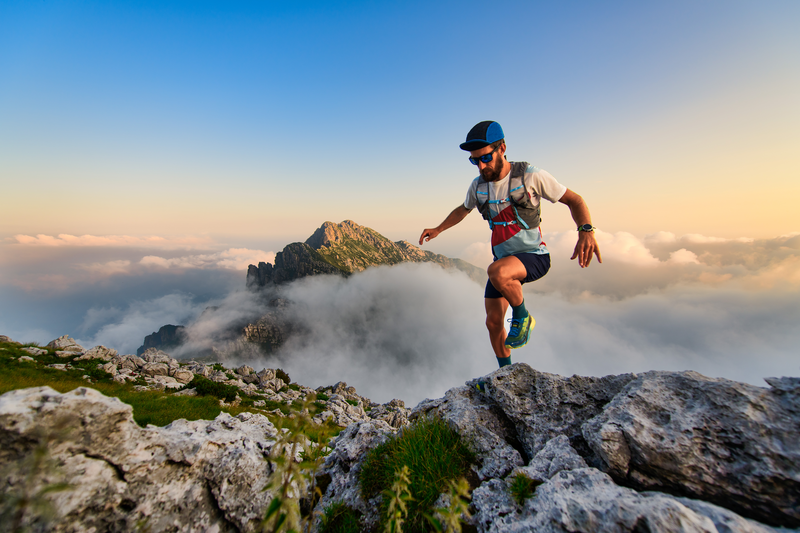
94% of researchers rate our articles as excellent or good
Learn more about the work of our research integrity team to safeguard the quality of each article we publish.
Find out more
ORIGINAL RESEARCH article
Front. Immunol.
Sec. Nutritional Immunology
Volume 16 - 2025 | doi: 10.3389/fimmu.2025.1544299
The final, formatted version of the article will be published soon.
You have multiple emails registered with Frontiers:
Please enter your email address:
If you already have an account, please login
You don't have a Frontiers account ? You can register here
The importance of small bioactive peptides derived from pig spleen have been used to enhance immune responses and support intestinal health. However, there is a lack of information regarding the conformational relationship and their effects on immune function of pig spleen proteins (PSPs).The objective of this study was to prepare and assess the immunomodulatory characteristics of immunopeptides from PSP. Firstly, enzymatic hydrolysates from PSP were prepared using alkaline protease and aminopeptidase, and small hydrolysate fractions with a <3 kDa were separated by SDS-PAGE and GPC. The bioactive peptides were then identified at peaks 5 to 7 (PSP-5, 6 and 7) by HPLC and TOF-MS, which were mainly composed of Pro-Glu-Leu by LC-MS. The PSP-5 and PSP-6 pronounced greater beneficial effects on cell viability and nitric oxide (NO) production than PSP-7 in macrophage, and PSP-5 exhibited a higher immunomodulatory ability than PSP-6. In vivo, the oral administration of 25-50 mg PSP-5/kg body weight (BW) protected against cyclophosphamide (CTX)induced immunosuppression in spleen and intestine of mouse, as evidenced by increased cytokine and sIgA productions. In conclusion, a novel set of bioactive immunopeptides derived from PSP through enzymatic hydrolysis could enhance immunomodulatory properties.
Keywords: pig spleen protein, immunopeptides, Enzymatic hydrolysis, Immunosuppression, Bioactive peptides
Received: 12 Dec 2024; Accepted: 19 Feb 2025.
Copyright: © 2025 Xia, Ling, Ye, Liang, Cao, Wang, Zhang, Dong, Tian, Jianjun and Zhu. This is an open-access article distributed under the terms of the Creative Commons Attribution License (CC BY). The use, distribution or reproduction in other forums is permitted, provided the original author(s) or licensor are credited and that the original publication in this journal is cited, in accordance with accepted academic practice. No use, distribution or reproduction is permitted which does not comply with these terms.
* Correspondence:
Zuo Jianjun, South China Agricultural University, Guangzhou, China
Yongwen Zhu, South China Agricultural University, Guangzhou, China
Disclaimer: All claims expressed in this article are solely those of the authors and do not necessarily represent those of their affiliated organizations, or those of the publisher, the editors and the reviewers. Any product that may be evaluated in this article or claim that may be made by its manufacturer is not guaranteed or endorsed by the publisher.
Research integrity at Frontiers
Learn more about the work of our research integrity team to safeguard the quality of each article we publish.