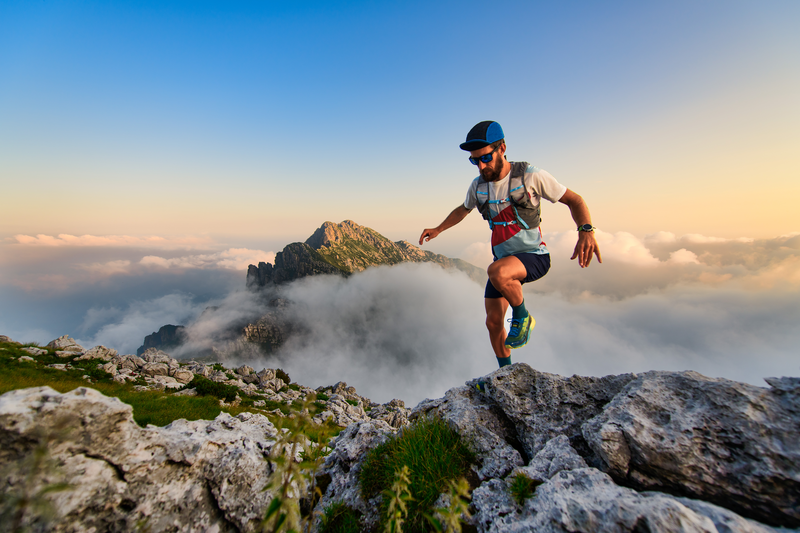
94% of researchers rate our articles as excellent or good
Learn more about the work of our research integrity team to safeguard the quality of each article we publish.
Find out more
REVIEW article
Front. Immunol.
Sec. Cancer Immunity and Immunotherapy
Volume 16 - 2025 | doi: 10.3389/fimmu.2025.1543096
The final, formatted version of the article will be published soon.
You have multiple emails registered with Frontiers:
Please enter your email address:
If you already have an account, please login
You don't have a Frontiers account ? You can register here
Ovarian cancer (OC) remains the most lethal gynecological malignancy, primarily due to its late-stage diagnosis, frequent recurrence, and resistance to conventional chemotherapy. A critical factor contributing to OC's aggressiveness is the tumor microenvironment (TME), particularly the presence and polarization of tumor-associated macrophages (TAMs). TAMs, often skewed towards an immunosuppressive M2-like phenotype, facilitate tumor growth, angiogenesis, metastasis, and resistance to therapy. This comprehensive review delves into the multifaceted regulation of macrophage polarization in OC, highlighting key molecular pathways such as PTEN loss, Wnt/β-catenin signaling, NF-κB, Myc, STAT3, and JNK, among others. Additionally, it explores the role of chemokines, non-coding RNAs, and various proteins in modulating TAM phenotypes. Emerging evidence underscores the significance of extracellular vesicles (EVs) and ovarian cancer stem cells (CSCs) in promoting M2 polarization, thereby enhancing tumor progression and therapy resistance. The review also identifies critical biomarkers associated with macrophage polarization, including CD163, LILRB1, MUC2, and others, which hold prognostic and therapeutic potential. Therapeutic strategies targeting TAMs are extensively discussed, encompassing oncolytic viruses, engineered EVs, immunotherapies, nanoparticles, targeted therapies, and natural products. These approaches aim to reprogram TAMs from a pro-tumorigenic M2 state to an antitumorigenic M1 phenotype, thereby enhancing immune responses and overcoming resistance to treatments such as chemotherapy and immune checkpoint inhibitors. Furthermore, the review addresses the interplay between macrophage polarization and therapy resistance, emphasizing the need for novel interventions to modulate the TME effectively. By synthesizing current knowledge on macrophage polarization in ovarian cancer, this study underscores the potential of targeting TAMs to improve clinical outcomes and personalize treatment strategies for OC patients. Continued research in this domain is essential to develop robust therapeutic frameworks that can mitigate the immunosuppressive TME and enhance the efficacy of existing and novel cancer therapies.
Keywords: ovarian cancer, Tumor-associated macrophages, Macrophage polarization, Tumor Microenvironment, therapy resistance, biomarkers, Immunotherapy, extracellular vesicles
Received: 10 Dec 2024; Accepted: 21 Mar 2025.
Copyright: © 2025 Xu, Chen, Tan and Tan. This is an open-access article distributed under the terms of the Creative Commons Attribution License (CC BY). The use, distribution or reproduction in other forums is permitted, provided the original author(s) or licensor are credited and that the original publication in this journal is cited, in accordance with accepted academic practice. No use, distribution or reproduction is permitted which does not comply with these terms.
* Correspondence:
Qingqing Tan, Nanjing Medical University, Nanjing, China
Disclaimer: All claims expressed in this article are solely those of the authors and do not necessarily represent those of their affiliated organizations, or those of the publisher, the editors and the reviewers. Any product that may be evaluated in this article or claim that may be made by its manufacturer is not guaranteed or endorsed by the publisher.
Research integrity at Frontiers
Learn more about the work of our research integrity team to safeguard the quality of each article we publish.