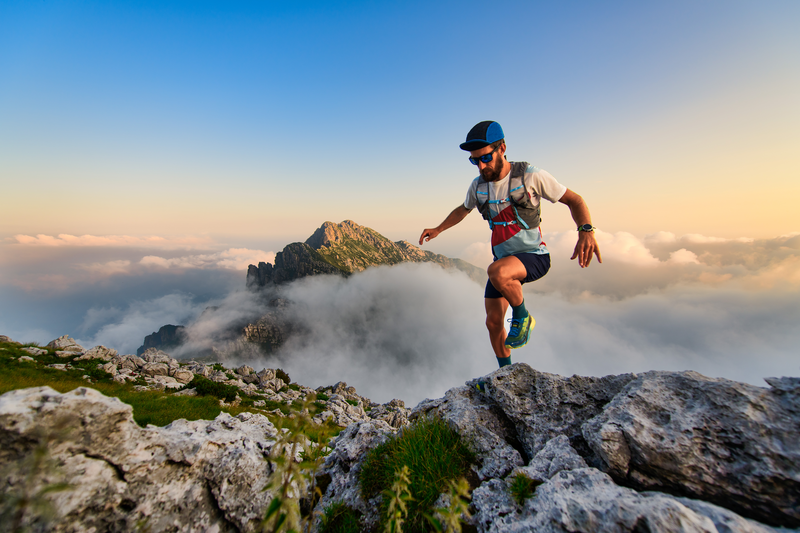
94% of researchers rate our articles as excellent or good
Learn more about the work of our research integrity team to safeguard the quality of each article we publish.
Find out more
REVIEW article
Front. Immunol. , 17 February 2025
Sec. Cancer Immunity and Immunotherapy
Volume 16 - 2025 | https://doi.org/10.3389/fimmu.2025.1542197
Metastasis is a hallmark of advanced cancer, and the liver is a common site for secondary metastasis of many tumor cells, including colorectal, pancreatic, gastric, and prostate cancers. Macrophages in the tumor microenvironment (TME) promote tumor cell metastasis through various mechanisms, including angiogenesis and immunosuppression, and play a unique role in the development of liver metastasis. Macrophages are affected by a variety of factors. Under conditions of hypoxia and increased acidity in the TME, more factors are now found to promote the polarization of macrophages to the M2 type, including exosomes and amino acids. M2-type macrophages promote tumor cell angiogenesis through a variety of mechanisms, including the secretion of factors such as VEGF, IL-1β, and TGF-β1. M2-type macrophages are subjected to multiple regulatory mechanisms. They also interact with various cells within the tumor microenvironment to co-regulate certain conditions, including the creation of an immunosuppressive microenvironment. This interaction promotes tumor cell metastasis, drug resistance, and immune escape. Based on the advent of single-cell sequencing technology, further insights into macrophage subpopulations in the tumor microenvironment may help in exploring new therapeutic targets in the future. In this paper, we will focus on how macrophages affect the TME, how tumor cells and macrophages as well as other immune cells interact with each other, and further investigate the mechanisms involved in liver metastasis of tumor cells and their potential as therapeutic targets.
Metastasis is the leading cause of cancer-related death, and the liver is the most common site of cancer metastasis. Liver metastases are highly invasive and refractory. They are a major cause of cancer morbidity and mortality (1). As shown in Figure 1, primary tumors of different tissues and organs can metastasize to different sites, and liver metastases occur at advanced stages in a variety of tumor types, including colorectal cancer (CRC), pancreatic cancer (PDAC), breast cancer, gallbladder cancer (GBC), gastric cancer (GC), endometrial mesenchymal sarcoma (ESS), and lung adenocarcinoma (LUAD). However, liver metastases still only benefit from immunotherapy in a small number of patients despite the emergence of emerging therapeutic techniques such as immunotherapy, which may be due to macrophage-mediated factors and affects the patients’ prognosis. Macrophages are a kind of immune cells of the organism, accounting for a large proportion in the tumor microenvironment, and macrophages in the tumor microenvironment are inextricably linked to the emergence of liver metastasis of tumor cells. Tumor cells secrete a variety of factors, such as cytokines and vascular endothelial generating factor, to recruit macrophages and promote macrophages to secrete certain factors to promote the formation of the pre-metastatic ecological niche (2). In the tumor microenvironment, macrophages interact with tumor cells and other immune cells to form an immunosuppressive microenvironment that promotes tumor cell survival and liver metastasis.
Figure 1. Primary and metastatic sites of different tumor cells. The liver serves as a common site of metastasis for tumor cells, for example pancreatic and colorectal cancers are more common. Created with BioRender.com.
Nowadays, emerging therapeutic techniques for liver metastasis are constantly developing. Stereotactic radiation therapy (SBRT) and some immunotherapy are used for the treatment of liver metastasis. However, due to the complexity of the tumor microenvironment, the effectiveness of immunotherapy has been limited. With the emergence of single-cell sequencing technology and in-depth understanding of the molecular mechanisms of cancer occurrence and progression, the development of a more specific and effective therapeutic approach will become the focus of future research. Tumor cell liver metastasis is not a simple disease, and a series of changes occur during the metastatic process. Through in-depth study of the correlation of macrophages in the process of tumor cell liver metastasis and the discovery of new targets, a reference basis is provided for the development of personalized therapeutic methods and new strategies. This provides a broad future for the patient’s quality of life and the patient’s prognosis through the use of new medications and new personalized interventions for treatment.
Macrophages are widely distributed innate immune cells. They play different roles in a variety of diseases, such as autoimmune diseases, tumors, and parasitic infections. Macrophages were described by Elie Metchnikoff in 1905. They are found in several parts of the body and have different names depending on their location, such as microglia, Kupffer cells, osteoclasts, bronchoalveolar macrophages, intestinal tissue macrophages, and epidermal Langerhans cells, among others. They have three main sources: macrophages/dendritic cell progenitors (MDPs) derived from prenatal embryonic precursors (yolk sacs or fetal livers) or from bone marrow that give rise to monocytes. They play an irreplaceable role in the maintenance of collective homeostasis (3–5). They have three basic functions: phagocytosis, exogenous antigen presentation, and immunomodulation through cytokine and growth factor secretion (6).Macrophages are highly plastic cells that undergo various forms of functional activation in response to different signals. As shown in Figure 2, macrophage classification is usually divided into classically activated macrophages (M1 macrophages) and selectively activated macrophages (M2 macrophages). However, this classification is relatively simplistic. Some studies have mentioned that there are also tumor-associated macrophages (TAMs) and CD169 macrophages. The functions of M1 and M2 macrophages are diametrically opposed. M1-type macrophages have an antitumor effect, while M2-type macrophages promote tumorigenesis and progression. They act on two major lymphocyte subpopulations: Th1 and Th2 cells. M1-type macrophages are mainly activated by interferon (IFN)-γ, tumor necrosis factor (TNF)-α, or lipopolysaccharide (LPS). M2-type macrophages are mainly activated by Th2-associated cytokines (e.g., IL-4, IL-10, and IL-13) and lactate, among others (7). HIF1α is indispensable in the activation induction process (8). M1 macrophages are mainly dependent on aerobic glycolysis, whereas M2 macrophages rely on oxidative metabolism (9). Macrophages can be polarized into M2a, M2b, and M2c subtypes by IL-4, LPS, IL-1β, and IL-10, respectively (10). They contain large amounts of intracellular S100A4, which enhances pre-tumor macrophage polarization by controlling PPAR-γ-dependent fatty acid oxidation induction (11).
Figure 2. Classification of macrophages and the role of different cell types. Monocytes become M0 macrophages upon M-CSF stimulation, and M0 macrophages can become M2a, M2b, M2c, M2d and M1 types under different conditions, different types of macrophages due to stimulation by different factors and thus have different functions. Created with BioRender.com.
M1 macrophages are typically induced by stimulation with lipopolysaccharide (LPS), interferon-gamma (IFN-γ), tumor necrosis factor-alpha (TNF-α), or granulocyte-macrophage colony-stimulating factor (GM-CSF). They are characterized by high expression of MHC class II molecules, which are involved in antigen presentation, and CD86 molecules, which play a role in T cell co-stimulation. M1 macrophages can secrete TNF-α, interleukin-6 (IL-6), interleukin-1β (IL-1β), IFN-γ, interleukin-12 (IL-12), nitric oxide (NO), and reactive oxygen species (ROS) (12). They promote inflammation and sterilization, block ongoing damage processes, and prevent over-repair (13). Additionally, M1 macrophages have an oncogenic function by releasing large amounts of IL-12, thereby impeding tumor growth (14). The M1 phenotype has been associated with various diseases, including chronic obstructive pulmonary disease (COPD) (15), sepsis (16), diabetic foot ulcers (DFUs) (17), and atherosclerotic lesions (18). M1 macrophages induce TLR4/AP1 signaling in pre-osteoblasts, and their paracrine secretion inhibits osteoclastogenesis, contributing to alveolar bone destruction in periodontitis (19). Exosomes from M1 macrophages transfer miR-628-5p to hepatocellular carcinoma (HCC) cells, inhibiting the expression of human methyltransferase-like 14 (METTL14). This, in turn, suppresses the m6A modification of circFUT8 and the development of HCC (20). Phospholipase D4 (PLD4) has been shown to enhance the anti-tumor effects of M1 macrophages in colon cancer cells (21). M1 macrophages carrying miR-16-5p-derived exosomes inhibit gastric cancer (GC) progression by activating T-cell immune responses through PD-L1 (22). Selective class IIa HDAC inhibitors exert anti-tumor effects on colorectal cancer by promoting M1 macrophage polarization and enhancing the efficacy of PD-1 blockade (23). Macroporous hydrogel (M1LMHA) can secrete various factors, including TNF-α, IFN-γ, and IL-12, and it exerts anti-tumor effects on melanoma by repolarizing M2-type to M1-type macrophages through the NF-κB pathway (24). Chemokine ligand 13 is more highly upregulated in M1 macrophages than in M0 macrophages (25). M1 macrophage-derived exosomes, engineered to promote M1 polarization and target IL4R, inhibit tumor growth by reprogramming tumor-associated macrophages (TAMs) into M1-like macrophages (26). miR-18a inhibits liver metastasis by inducing M1 macrophages (27).
IL-4 and IL-13 are the primary inducers of M2a macrophages. These macrophages secrete TGF-β, IGF, and fibronectin, which facilitate wound healing and fibrosis. M2a macrophages are predominantly found in the renal tubular interstitium, particularly in cases of renal pathologies such as segmental glomerulosclerosis and tubular atrophy/interstitial fibrosis (28). They have also been identified as a significant source of early fibrosis following cerebral ischemia (29). Moreover, M2a macrophages possess bactericidal capabilities (30). CCL22 can polarize cervical cancer-associated tumor-associated macrophages (TAMs) into M2a macrophages (10). However, the elevated expression of chitinase 3-like 1 protein (CHI3L1), a secreted glycoprotein, in M2a macrophages promotes tumor cell invasion and metastasis by upregulating matrix metalloproteinases (MMPs) in various tumor cells. In addition to its role in cancer, CHI3L1 also plays an anti-inflammatory role in several other inflammatory diseases. CHI3L1 secreted by M2a macrophages contributes to the metabolic imbalance of the extracellular matrix in rat intervertebral disc degeneration by activating the IL-13Rα2/MAPK pathway (31).
M2b macrophages are a subtype of M2 macrophages that have garnered attention due to their potent immunomodulatory and anti-inflammatory effects. Also known as regulatory macrophages, they are activated by immune complexes, lipopolysaccharide (LPS), and toll-like receptor (TLR) ligands. Additionally, it has been shown that activated lymphocyte-derived DNA (ALD-DNA) can also promote macrophage polarization towards the M2b phenotype (32). M2b macrophages are characterized by the expression of markers such as IL-6, tumor necrosis factor-alpha (TNF-α), CD86, TNFSF14, IL-10, CCL1, and SPHK1 (33). These macrophages secrete factors like IL-10, IL-6, and IL-1, which can exert pro-tumorigenic effects. Notably, IL-10 possesses strong anti-inflammatory properties. M2b macrophage exosomes mediate the response to glucagon by modulating the CCL1/CCR8 axis. This exocytosis also exerts a protective effect against dextran sulfate sodium (DSS)-induced colitis through the same CCL1/CCR8 axis (34). Furthermore, alcohol and LPS have been found to induce macrophage M2b polarization by activating the MAPK/P38, MAPK/ERK, and NF-κB signaling pathways. This activation leads to an increased expression of inflammatory cytokines such as TNF, IL-1β, and IL-10 (35).
M2c macrophages are characterized by their role in tissue remodeling and regeneration (36). They also perform several other functions, including angiogenesis, matrix maturation, and phagocytosis (37). M2c macrophages are primarily activated by glucocorticoids or IL-10. They promote the epithelial-mesenchymal transition (EMT) of human renal proximal tubular epithelial cells (HK-2) (38). M2c macrophages secrete TGF-β and IL-10, and they are involved in phagocytosis, immunosuppression, angiogenesis, and the development of tissue fibrosis.
M2d macrophages, also known as tumor-associated macrophages (TAMs), are primarily activated by adenosine or IL-6. These macrophages secrete IL-10, TGF-β, and VEGF, which promote angiogenesis and cancer metastasis. They are recruited from circulating monocytes into tumors and are influenced by the presence of cancer to facilitate tumor malignancy and progression. M2d macrophages are often referred to as tumor-associated macrophages (TAMs) (5). In the tumor microenvironment, various secreted factors and exosomes from tumor cells can promote macrophage polarization towards the M2 type. For instance, circPOLQ can enhance M2 macrophage polarization via CRC cell-derived exosomes (39). Similarly, the cancer-associated fibroblast (CAF)-related gene insulin-like growth factor-binding protein 2 (IGFBP2) plays a role in this process, as mentioned in gliomas (40). The exosome miR-106a-5p from colorectal cancer is also involved in M2-type polarization (41). In cervical cancer, IL-17A has been found to promote M2 macrophage polarization, thereby facilitating metastasis. Additionally, lactate secreted by cervical cancer promotes M2-type polarization by upregulating GPD2 through histone lactylation (42, 43). In lung cancer, tumor-derived γ-aminobutyric acid (GABA) promotes macrophage M2 polarization by activating the JAK1/STAT6 pathway and inhibits M1 polarization by suppressing the JAK2/STAT3 pathway (44). Nowadays, M2 macrophage polarization is not only mediated by IL-6 and other factors, but an increasing number of exosomes, secreted factors, and even amino acids have been discovered to play a role. TAMs can promote tumor progression, metastasis, and treatment resistance. Macrophages infiltrating tumor tissues are driven by tumor-derived and T-cell-derived cytokines (45). TAMs, as a specific phenotype of M2-like macrophages, are phagocytes of unknown origin (4). They typically exist in an M2-like phenotype with anti-inflammatory functions and shape the tumor microenvironment by secreting various immunosuppressive cytokines and modulating cytotoxic T lymphocyte (CTL) activity (46). Hypoxia, a hallmark of the tumor microenvironment, can promote macrophage polarization into TAMs to a greater extent (47). TAMs are the most abundant cell type in the tumor microenvironment (TME) and are mainly involved in angiogenesis, immunosuppression, tumor cell invasion, stromal remodeling, and tumor resistance to chemotherapy. They also promote immune escape of tumor cells (48). TAMs can promote tumor cell initiation and metastasis, inhibit T cell-mediated anti-tumor immune responses, and stimulate tumor angiogenesis and subsequent tumor progression (49). They contribute to the hematogenous spread of cancer cells, especially liver metastasis (50). Increased TAM recruitment and M2 polarization are observed in liver metastatic lesions compared to primary sites of human CRC tissue (51). Kupffer cells enhance metastasis formation in the liver (52) and also induce hepatic metastasis formation through AT1a signaling by inducing TGF-β1 (53). Fatty Acid Binding Protein 4 (FABP4) in TAMs may contribute to hepatic metastasis formation by inactivating the NF-κB-IL1α pathway through ubiquitination of ATPB, thereby promoting the proliferation and migration of neuroblastoma cells (NB) (54). STAT1, STAT3, STAT5, and STAT6 have all been associated with TAM function. The release of sphingosine 1-phosphate (S1P) from apoptotic tumor cells stimulates lipid-cotransporting protein 2 (LCN2) production in TAMs and is associated with metastasis (55). Lung cancer particles (L-MPs) induce the release of a key pro-inflammatory cytokine, IL-1β, from M2-like macrophages, which promotes lung cancer development (56). Interstitial flow in the tumor environment, a mechanical stimulus, promotes M2 polarization of macrophages. These polarized macrophages are recruited to the tumor mass, thereby promoting cancer cell invasion and tumor progression (57). Co-culture of TAMs with tumor-associated neutrophils (TANs) results in altered expression of cytokines and chemokines, producing interleukin (IL-11) and oncostatin M (OSM). These factors activate STAT3 signaling and promote intrahepatic cholangiocarcinoma (ICC) cell proliferation, invasion, and colony formation (58). Collagen Triple Helix Repeat Sequence 1 (CTHRC1) in primary CRC promotes CRC liver metastasis by remodeling infiltrating macrophages through TGF-β signaling (59). Depletion of mitogen-activated protein kinase 4 (MAPK4) in gastric cancer cells is associated with macrophage polarization to tumor-associated macrophages (TAMs). This induces the secretion of macrophage migration inhibitory factor (MIF), thereby promoting macrophage polarization. TAMs activate the epithelial-mesenchymal transition of gastric cancer cells and inhibit the expression of MAPK4, creating a positive feedback loop that induces the secretion of MIF and contributes to TAM polarization. This process can promote gastric cancer liver metastasis (60). CC-chemokine ligand 20 (CCL20) expression is abnormally elevated in TAMs of pancreatic cancer tissues. CC-chemokine receptor 6 (CCR6) acts as a receptor for CCL20 in pancreatic cancer cells, mediating the promotion of pancreatic cancer growth and liver metastasis by CCL20 in a mouse model (61). CRC with liver metastasis has an elevated proportion of infiltrating M2 macrophages compared to CRC without liver metastasis. A positive feedback loop exists in which CRC promotes macrophage M2 polarization through the activation of the JAK2/STAT3 pathway by releasing the exosome miR-106a-5p and inhibiting SOCS6. This, in turn, promotes the occurrence of liver metastasis in CRC (41). Macrophages in the TME microenvironment have a strong lipid uptake capacity. Tumor cell-derived fatty acids, such as monounsaturated long-chain fatty acids (LCFAs), play a role in inducing macrophage polarization to the M2 type. This process is mainly mediated by upregulated CD36 in tumor-infiltrating macrophages, which play a pro-tumorigenic role after phagocytosis of lipids in the tumor cells via a CD36-dependent mechanism (62). As shown in Figure 3, in the tumor microenvironment, tumor-associated macrophages play a crucial role in promoting the progression of liver metastasis,therefore, the occurrence of liver metastasis may be suppressed to some extent by inhibiting TAM.
Figure 3. The crucial role of tumor-associated macrophages in promoting the progression of liver metastases in the tumor microenvironment and therapeutic strategies to target them. Created with BioRender.com.
CD169 macrophages are a subpopulation of macrophages primarily found in the lymph node peritoneal sinusoids and the splenic border (10). However, a few are also present in the intestine, liver, and bone marrow. The density of CD169-positive macrophages in lymph node (LN) sinusoids is positively correlated with the density of infiltrating T cells and NK cells in tumor tissues. This suggests that CD169-positive macrophages play a significant role in the anti-tumor immune response of patients with tumors (63). Depletion of CD169 macrophages in tumor-draining LNs leads to increased lung metastasis (64). Radiofrequency ablation (RFA) is a therapeutic approach for treating primary or metastatic hepatocellular carcinoma with small foci. RFA induces limited distant effects in hepatocellular carcinoma. However, the transfer of CD169 macrophages effectively improves RFA-induced distant effects and contributes to the regression of liver tumors (65).
With the advent of single-cell sequencing technology, macrophage classification is no longer limited to traditional M subtypes. In the tumor microenvironment (TME), macrophages are divided into two main subsets: C1Q+ and SPP1+ tumor-associated macrophages (TAMs), as well as two secondary subsets: FCN1+ and CCL18+ TAMs. The SPP1+ and C1Q+ TAM subsets can be further divided into distinct populations with varying functions (66). As shown in Figure 4, in the tumor microenvironment, each of these subsets express different molecular functions, including immunosuppression and promotion of angiogenesis, with SPP1+ TAMs being particularly associated with malignancy. They are also linked to liver metastases and promote angiogenesis (67). C1Q+ TAMs and CCL18+ TAMs mainly exert immunosuppressive effects. The interaction of SPP1+ TAMs with fibroblasts establishes an immunosuppressive metastatic niche, fostering the growth of colorectal cancer cells within this niche (68). Liang et al. have reported similar findings: in oral squamous cell carcinoma, TAMs may potentially activate fibroblasts and promote T cell exhaustion through SPP1-CD44 and CD155-CD226 ligand-receptor interactions. This reshapes the metastatic lymph node microenvironment, thereby facilitating the colonization and proliferation of disseminated tumor cells (69). Xiangxiang Liu et al. (70) reported that SPP1+ TAMs enhance the invasion and metastasis of colorectal cancer cells and also create an immunosuppressive microenvironment for hepatocellular carcinoma (71). In non-small cell lung cancer, single-cell RNA sequencing has identified SPP1+ macrophages, and another immunosuppressive TAM, CCL18+ TAM, has been discovered. CCL18+ TAMs exert immunosuppressive effects by inhibiting the production of inflammatory cytokines through the suppression of oxidative phosphorylation as their primary metabolic mode. At the same time, CCL18+ TAMs can promote proliferation, migration, and epithelial-mesenchymal transition (EMT) in oral squamous cell carcinoma (72). Current research on the relationship between CCL18+ TAMs and liver metastasis is limited, and future studies will primarily focus on developing treatments targeting CCL18+ TAMs. For SPP1+ TAMs, they can upregulate PD-L1 expression while promoting TME matrix remodeling to accelerate tumor progression (73). C1Q+ TAMs appear to be associated with T cell exhaustion in the tumor microenvironment, facilitating this process by expressing immune checkpoints, interacting with T cell immune checkpoints, and also directly interacting with endothelial cells to promote the neoangiogenesis required for tumor growth (74). The discovery of the interaction between CD3+C1Q+ TAMs and CD8+CCL4+ T cells in hepatocellular carcinoma presents a novel therapeutic target for reversing the immunosuppressive microenvironment in this cancer type. FCN1+ TAMs are thought to represent an intermediate stage in the maturation of monocytes into tumor macrophages and are associated with angiogenesis (75). These TAM subsets are more specific than traditional M subsets. Different subsets focus on distinct functions, making them more detailed and precise targets for therapeutic research.
Figure 4. Different subpopulations of macrophages play different roles in the tumor microenvironment. Created with BioRender.com.
Tumor cells metastasize through a variety of mechanisms, including angiogenesis, immunosuppression, and establishment of a pre-metastatic ecological niche, etc. Macrophages can mediate these mechanisms. The environment in which tumor cells live is a state of hypoxia, and under hypoxia, macrophages promote the emergence of hepatic metastasis by affecting angiogenesis and immunosuppression of tumor cells, as shown in Figure 5.
Figure 5. M2 macrophages mediate tumor cell liver metastasis. M2-type macrophages mediate tumor cell liver metastasis Lack of oxygen in the tumor microenvironment promotes monocyte polarization to M2 type, and M2 type macrophages further promote angiogenesis, EMT, and immunosuppressive state of tumor cells through IL-10, TGF-β, and IL-6, and further liver metastasis. Created with BioRender.com.
Tumor cells can metastasize through various mechanisms, including increased angiogenesis to facilitate metastasis. Blood vessels are crucial for providing nutrients, maintaining cells, and supporting further growth in new metastases. The tumor microenvironment, where tumor cells reside, is often hypoxic. In this hypoxic state, macrophages are also oxygen-deprived, and HIF-1α stimulates tumor-associated macrophages (TAMs) to produce various factors, such as VEGF, IL-1β, TGF-β1, tumor necrosis factor (TNF)-α, basic fibroblast growth factor (bFGF), and IL-10. These factors induce angiogenesis, thereby promoting tumor cell metastasis (76, 77). CRC cells that overexpress regenerative hepatic protein phosphatase 3 (PRL-3) exhibit increased angiogenesis when co-cultured with TAMs. PRL-3 promotes angiogenesis by activating the NF-κB pathway in CRC cells (78). Additionally, TGF-β and pro-angiogenic growth factor PDGF contribute to solid tumor angiogenesis (79, 80). Studies have shown that M2 macrophage-derived exosomes (MDEs) can promote angiogenesis in mouse aortic endothelial cells (MAECs) in vitro. Moreover, M2 MDEs can enhance subcutaneous tumor growth and increase vascular density in mice. Exosomal miR-155-5p and miR-221-5p target E2F2 to promote angiogenesis and growth of pancreatic ductal adenocarcinoma (PDAC) in vivo (81). Upregulated miR-25-3p, miR-130b-3p, and miR-425-5p in CRC cells regulate PTEN through activation of the PI3K/Akt signaling pathway, inducing M2 polarization of macrophages. In turn, M2-polarized macrophages promote cancer metastasis by enhancing epithelial-mesenchymal transition (EMT) and secreting vascular endothelial growth factor (VEGF) (82). A similar situation exists in liver metastasis of gastric cancer cells. Hepatic macrophage KC secretes IL-6, hepatocyte growth factor (HGF), VEGF, and matrix metalloproteinases (MMP) 9 and 14. These factors accelerate tumor invasion into the parenchymal and interstitial spaces, promote tumor cell proliferation and angiogenesis, and thus enhance liver metastasis (83).
Macrophage-mediated immunosuppression contributes to liver metastasis and a poor prognosis. It can exert its immunosuppressive effects in various ways, such as recruiting cancer-associated fibroblasts (CAFs) and promoting mutual interactions to further create an immunosuppressive environment. Additionally, it induces Treg differentiation, inhibits natural killer (NK) cell activation, and impairs dendritic cell (DC) function (84). Tumor-resident microbiota, such as E. coli, enhances lactic acid production. Lactic acid-cultured Kupffer cells secrete cytokines that promote PD-1 expression in regulatory T cells (Tregs) and reduce CD8 T cell activation (85). In the hypoxic tumor microenvironment, macrophages express PD-L1 in response to HIF-1α secretion. PD-L1 acts as a ligand for the inhibitory receptor programmed cell death protein 1 (PD-1) and suppresses T cell activity, creating a state of immunosuppression. Prostaglandin E2 (PGE2), an immunosuppressant produced by cancer cells and their surrounding macrophages, and high concentrations of PGE2 in the portal vein inhibit liver-associated immunity and promote the formation of hepatic metastases (86). Tumor-associated macrophages (TAMs) may promote gastric cancer (GC) angiogenesis by enhancing VEGF and VEGF-C expression and lymphangiogenesis (87). In colorectal cancer, mucin 1 (ORM1) partially promotes colorectal liver metastasis (CRLM) by inducing macrophage M2 polarization. It also promotes macrophage production of IL-10, which is implicated in regulating the immune response, thereby inducing the formation of an immunosuppressive microenvironment (88). Various complex interactions between myofibroblasts-metastasis-associated fibroblasts (myMAFs) can promote tumor cell metastasis. Macrophage-derived granulin precursors can induce myMAFs through a STAT3-dependent mechanism. Further, myMAF secretion of bone-bridging proteins promotes an immunosuppressive macrophage phenotype, which inhibits cytotoxic T cell function. This, in turn, causes immunosuppression and promotes hepatic metastasis of pancreatic cancer cells (89). Tumor TCF4-induced recruitment and polarization of TAMs may produce an immunosuppressive, pro-metastatic milieu by preventing CD8 T cell infiltration (51).
Tumor cells create a susceptible metastatic microenvironment, known as the pre-metastatic niche (PMN), before reaching the metastatic site. In this PMN, CRC exosomes promote the polarization of M2 macrophages. These macrophages, in turn, secrete CXCL12, which increases cancer metastasis through the CXCL12/CXCR4/NF-κB signaling pathway, leading to colorectal cancer liver metastasis (CRLM) (90). Triple-negative breast cancer (TNBC) develops a pre-metastatic niche in the liver during the development of liver metastases. This process is associated with CX3CL1 levels, which promote macrophage migration and breast cancer cell invasion by recruiting CX3CR1-expressing macrophages. Consequently, CX3CL1-CX3CR1 signaling leads to the upregulation of MMP9 (91). The exosome miR-4488 secreted by pancreatic neuroendocrine tumors (pNENs) promotes M2-like polarization by directly targeting and inhibiting RTN3 in macrophages. M2-polarized macrophages then promote pre-metastatic ecological niche formation and advance pNENs liver metastasis by releasing MMP2 (92). CRC-sEVs induce an inflammatory pre-metastatic ecological niche through the miR-21-TLR7-IL-6 axis, playing a key role in promoting liver metastasis (93). Liver metastasis of pancreatic ductal adenocarcinoma (PDAC) has also been linked to the hepatic pre-metastatic niche. Here, exosomal macrophage migration inhibitory factor (MIF) induces the release of transforming growth factor β (TGFβ) from Kupffer cells, which in turn promotes the production of fibronectin (FN) by hepatic stellate cells (hStCs). FN deposits facilitate the colonization of the liver by bone marrow-derived macrophages and neutrophils, resulting in the formation of a pre-metastatic ecological niche (94). Tumor-derived extracellular vesicles (TEVs) enriched with Circ-0034880 promote strong interactions between primary tumor cells and SPP1 high CD206 tumor-promoting macrophages, fostering PMN formation and CRLM (95).
Tumor cells metastasize through several steps. Initially, they invade the adjacent parenchyma from the confined primary tumor. Subsequently, they enter the circulation and ultimately reach the metastatic site. At this site, they are exposed to certain factors, such as transforming growth factor β (TGFβ) and fibronectin, which enable them to survive.
The process of tumor invasion and metastasis is often associated with the activation of epithelial-mesenchymal transition (EMT), and the link between EMT and tumor-associated macrophages (TAMs) is quite close. The activation of EMT typically requires crosstalk between cancer cells and components of the local tumor microenvironment, including TAMs. TAMs can promote EMT in tumor cells by secreting various factors. For instance, TGF-β and PRL-3 upregulate the expression of CCL26. CCL26 induces TAMs infiltration and increases the expression of IL-6 and IL-8 in tumor cells, which promotes EMT. Fibroblast growth factor 9 (FGF9) may contribute to macrophage recruitment and M2 polarization, and TAMs are involved in regulating the EMT process, further contributing to hepatic metastasis (96). M2-polarized TAMs are further promoted, in part, via TLR4/IL-10 signaling, which enhances EMT in pancreatic cancer cells (97). Additionally, their secretion of CXCL2 promotes EMT in gastrointestinal mesenchymal stromal tumor (GIST) cells (98). In pancreatic cancer, ALOX5 is highly expressed, primarily in macrophages. ALOX5 upregulates the expression of vimentin and downregulates the expression of E-cadherin to promote EMT in pancreatic cancer. It also promotes the M2-like phenotype of human macrophages through the JAK/STAT pathway, and the occurrence of liver metastasis is more pronounced with high ALOX5 expression, as observed in in vivo experiments (99).
Autophagy is a crucial protective mechanism that enables tumor cells to withstand harsh living conditions, such as hypoxia and nutrient deprivation. Tumor-associated macrophages (TAMs) can induce autophagy in tumor cells, thereby helping them resist these adverse environments. In particular, TAMs that home around metastatic nests can induce autophagic fluxes in gastric cancer (GC) cells and promote hepatic metastasis through GDNF-GFRA1 signaling. In vivo experiments have shown that downregulating GFRA1 significantly inhibits the colonization and formation of metastatic GC ecosystems in the liver. Conversely, this suggests that GFRA1 promotes the formation of hepatic GC metastatic ecosystems. Additionally, it has been found that TAMs act on GFRA1 expressed by GC cells, enhancing their growth ability under poor nutritional conditions through secreted proteins, which may include GDNF from TAMs (100).
In recent years, research on extracellular vesicles (EVs) promoting tumor cell liver metastasis by affecting macrophages has garnered attention. The tumor microenvironment (TME) is a dynamic system where non-tumor cells and cancer cells interact through soluble factors and EVs, releasing a variety of EVs (including exosomes, microvesicles, and many other vesicles) into the environment (101). Within the TME, both tumor cells and macrophages can promote metastasis, drug resistance, and immune escape by secreting microvesicles. The TME often exhibits a hypoxic profile, leading to an increase in exosome production in response to hypoxia-inducible factor (HIF)-1α (102). In colorectal cancer, CRC-EVs activate NOD1 in macrophages, initiating the secretion of inflammatory cytokines and chemokines (IL-6, CCL1, and CCL2) to promote tumor cell migration, while also promoting CRC liver metastasis (CRC-LM) by activating NOD1 (103). Tumor-derived extracellular vesicles (TEVs) can contribute to liver metastasis by fostering the formation of pre-metastatic niches (PMNs), such as small extracellular vesicles (sEVs) enriched with miR-151a-3p (104), and by accelerating GC-LM generation through macrophage polarization and its impact on angiogenesis (105). Tumor cells can also undergo hepatic metastasis via tumor-associated macrophage (TAM)-derived extracellular vesicles (EVs). TAM-EVs can influence tumor cells to undergo epithelial-mesenchymal transition (EMT), characterized by upregulation of N-cadherin and vimentin proteins and downregulation of E-cadherin in CRC cells incubated with TAM-EVs. TAM-EVs primarily affect tumor cells to undergo EMT by upregulating ABCA1 expression in MC-38 and CT-26 cells, decreasing cell membrane and intracellular cholesterol content, and potentially increasing membrane fluidity, which is closely associated with tumor cell metastasis (106). These extracellular vesicles regulate macrophage polarization and differentiation through various mechanisms and signaling pathways, providing a conducive environment for tumor cell survival and growth in the liver (Table 1).
Immune checkpoints play a crucial role in the immune response process. Previously identified immune checkpoints include PD-1/PD-L1 and cytotoxic T-lymphocyte antigen 4 (CTLA4). By interfering with the binding of immune checkpoints to their ligands, the anti-tumor ability of immune cells can be restored. Nowadays, the study of newly identified immune checkpoints, such as TIM3, LAG3, TIGIT, NKG2D, and CD4, as well as their respective ligands, is on the rise. These checkpoints and ligands are being intensively studied and may become new therapeutic targets in the future (117). The lymphotoxin β-receptor (LTBR) has been identified as a potential immune checkpoint in tumor-associated macrophages (TAMs). Its high expression, duplication, and hypomethylation are associated with a poor prognosis. LTBR maintains the immunosuppressive activity and M2 phenotype of TAMs through the NF-κB and Wnt/β-catenin signaling pathways, making it a promising target for overcoming immune checkpoint resistance (118). In the tumor microenvironment (TME), the expression of PD-L1 and CTLA-4 on TAM surfaces, as well as their ligands, is increased. This is often linked to a poorer clinical prognosis in patients with liver metastatic cancers (6). PD-L1 is upregulated and induces M2 polarization in TAMs (119). The binding of TAM surface PD-L1 and CTLA-4 to the corresponding receptors on T cells can inhibit T cell activation and proliferation, thereby enhancing the immunosuppressive effect (120). Studies have shown that overexpression of PAARH in hepatocellular carcinoma significantly promotes M2 macrophage polarization, increases PD-L1 levels, and enhances cell proliferation, migration, and invasion. It also inhibits M1 macrophage polarization, decreases PD-L2 levels, and suppresses apoptosis (121). TGF-β secreted by M2-TAMs increases glycolysis levels in bladder cancer (BLCA) and inhibits cell proliferation and migration via the pyruvate kinase isoenzyme (122). The pyruvate kinase isoform M2 (PKM2) plays a significant role in PD-L1-mediated immune evasion (122). Macrophages exert immunosuppressive effects in the tumor microenvironment, and their presence can inhibit the action of immune checkpoint inhibitors (123). This may contribute to the poor therapeutic efficacy of immune checkpoint inhibitors in patients with liver metastases and the development of drug resistance. Therefore, targeting macrophages in combination with immune checkpoint inhibitors can synergistically enhance the anti-tumor immune response and help overcome immune resistance.
Macrophages in the tumor microenvironment promote hepatic metastasis by enhancing immunosuppression and tumor cell proliferation, influencing the formation of the tumor microenvironment, and interacting with tumor cells. Specifically, they facilitate liver metastasis through tumor cell angiogenesis, the establishment of immunosuppression within the tumor microenvironment, and the production of pre-metastatic niches (PMNs), which involve multiple mechanisms and are associated with various secreted factors. Within the tumor microenvironment, macrophages can promote liver metastasis in several ways. For instance, M2-type macrophages can facilitate colorectal liver metastasis (CRLM) by secreting CXCL13, which subsequently activates the CXCL13/CXCR5/NF-κB/p65/miR-934 pathway in colorectal cancer (CRC) cells (111). TGF-β, a pro-fibroblastic cytokine, is secreted by intrahepatic macrophages, which in turn activate hepatic stellate cells (HSCs) and hepatic stromal cells, promoting hepatic PMN formation. Exosomes from tumor-associated macrophages (TAMs) also impact tumor cell liver metastasis. miR-142-5p and miR-202-5p promote pancreatic ductal adenocarcinoma (PDAC) cell invasion and metastasis by inhibiting PTEN-mediated mesenchymal-epithelial transition (MET) (124), and TAMs also suppress tumor suppressor genes to facilitate PDAC cell metastasis. In addition, macrophages affect the tumor microenvironment through a variety of secreted factors and signaling pathways that enhance tumor cell survival in the liver. These signaling pathways work synergistically to regulate the immunosuppressive function of macrophages in the liver, creating a favorable environment for cancer cell dissemination by suppressing immune responses, promoting angiogenesis, and modulating the tumor microenvironment (Tables 2, 3).
As research into the tumor microenvironment (TME) deepens, an increasing number of therapeutic approaches are emerging. A wide variety of emerging technologies and personalized therapies are now on the agenda. Whether targeting macrophage polarization towards the M2 type or addressing macrophage-promoted tumor cell angiogenesis, epithelial-mesenchymal transition (EMT), and immunosuppression for hepatic metastasis, numerous novel approaches have been developed. Targeting the metabolic interactions between tumor cells and tumor-associated macrophages (TAMs) within the TME is a potential strategy for treating tumor cell metastasis. Tumor cell-associated glucose metabolism, such as hypoxia and metabolite secretion, promotes macrophage recruitment and M2-type macrophage polarization. Meanwhile, TAM-related metabolism facilitates tumor cell EMT, invasion, and metastasis (141). Arginine metabolism in the TME also affects TAM differentiation, promoting an increased M2 phenotype. Natural compounds like leucovorin 3’-O-glucoside and indocyanine green, encapsulated in tumor cell-derived particles, target and promote TAM repolarization towards the M1 phenotype (142). A novel class of extracellular vesicle (EV)-like ginseng-derived nanoparticles (GDNPs) isolated from ginseng C. A. Mey plays a role in M1-type and M2-type macrophage polarization. GDNPs can alter M2-type macrophages, inducing M1-type macrophage polarization through Toll-like receptor (TLR)-4 and myeloid differentiation antigen 88 (MyD88)-mediated signaling. GDNPs significantly promote M2 polarization towards the M1 phenotype and generate reactive oxygen species, leading to increased apoptosis in mouse melanoma cells (143). It has been shown that delivering type I interferon (IFNα) to liver metastases via macrophages such as Kupffer cells and tumor-associated macrophages (TAMs) can delay the growth of colorectal and pancreatic ductal adenocarcinoma liver metastases in mice. This may be related to TAM immune activation, enhanced MHC-II-restricted antigen presentation, and reduced depletion of CD8 T cells (120). In one study, it was mentioned that the anti-programmed cell death protein 1 (aPD1) antibody alone had no effect on macrophage recruitment and infiltration into primary colon tumors. However, in tumors treated with regorafenib (REG) and aPD1, there was a significant reduction in intracellularly infiltrated macrophages, and it significantly affected liver metastases. The REG-induced remission of immunosuppression, along with the reactivation of cytotoxic T cells by aPD1, could be related to TAM immune activation, enhanced MHC-II-restricted antigen presentation, and CD8 T cell depletion (120). The reactivation of aPD1-toxic T-cells may explain these occurrences (144). Nanotechnology is now a relatively new field in antitumor research and can solve numerous problems, including the challenge of drug delivery barriers that prevent drugs from accurately reaching their target locations due to multiple barriers within the body. Albumin-conjugated paclitaxel (nAb-PTX) nanoparticles loaded onto nanoporous solid multistage nanovehicles (MSVs) can transport larger amounts to the liver for the treatment of liver metastases. A larger amount of nAb-PTX can be transported to the liver, allowing liver macrophages to retain nAb-PTX, remain viable, and release drugs to inhibit the proliferation of liver metastatic tumor cells and induce apoptosis (145). A novel nanomedicine, composed of KIRA6 (an endoplasmic reticulum stress inhibitor), α-tocopherol nanoemulsion, and anti-PD1 antibody, has been identified. It can transform the hepatic immune microenvironment into a “hot” phenotype by targeting hepatic macrophages to restore the number of CD8+ T-cells and promote a “hot” phenotype in immunosuppressed hepatic macrophages. This nanomedicine inhibits tumor cell liver metastasis by targeting hepatic macrophages to restore CD8+ T cell numbers, transforming the hepatic immune microenvironment into a “hot” phenotype, and promoting the repolarization of immunosuppressed hepatic macrophages to restore immune responses (146). Similar results were reported in another study, where TGF β-targeted inhibitory nanomedicines for sonodynamic therapy (SDT) reversed the immunosuppressive tumor microenvironment (147). 25-Hydroxycholesterol (25HC) exhibits a high concentration of aggregates in the TME, and targeting CH25H to disrupt this metabolic checkpoint can manipulate macrophage function to turn cold tumors into hot tumors. This can shape the immunological landscape and overall anti-tumor immune response, offering a promising therapeutic intervention (148). Iron oxide nanoparticles inhibit tumor growth by inducing pro-inflammatory macrophage polarization in tumor tissues (149).In pancreatic cancer, where low drug accumulation rates lead to poor responses to conventional chemotherapy, a technique called ultrasound-targeted microbubble destruction (UTMD) has emerged. UTMD improves chemotherapeutic outcomes by redirecting M2 macrophages to tumor-suppressing M1 macrophages, remodeling vascular normalization, and inducing an anti-tumor immune response, thereby enhancing the chemotherapeutic response (150).
As shown in Table 4, there are multiple pathways associated with TAMs during tumor cell metastasis.
As shown in Table 5, drugs targeting TAMs to inhibit tumor cell metastasis.
Clinical trials of drugs targeting TAMs to inhibit tumor cell metastasis, as shown in Table 6.
Chinese medicine possesses the characteristics and advantages of “multi-target, multi-pathway” in tumor treatment. It can exert anti-angiogenic effects by acting on multiple targets, such as HIF-1α, TGF-β1, VEGF, and VEGFR2, and influencing related pathways, including catenin/TCF3/LEF1, mTOR/p70S6K/4E-BP1, Akt/MAPKs/mTOR, p-Src/p-STAT3/VEGF/MMP-2,9, and so on (164). Relevant studies have shown that traditional Chinese medicine (TCM) can regulate the tumor microenvironment (TME) and affect the polarization of M2-type macrophages, thereby inhibiting tumor progression. TCM can exert anti-tumor effects through three pathways: first, by inhibiting macrophage polarization to the M2 phenotype; second, by reversing the M2 type to the M1 macrophage; and third, by inhibiting the role of M2-type macrophages in promoting tumor cell metastasis (165). Dandelion extract mediates the third of these phenotypes by polarizing macrophages from the M2 phenotype to the M1 phenotype (166), and iron in superparamagnetic iron oxide nanoparticles (SPION) achieves a similar effect (167). Macelignan inhibits macrophage M2 polarization via the ROS-mediated PI3K/AKT signaling pathway, thereby blocking IL-1β/NF-κB-dependent CRC metastasis (168). Gambogic acid (GA) inhibits macrophage M2 polarization by reducing tumor cell-derived EV-shuttle miR-21, thereby attenuating CRC liver metastasis (169). Secretion of IL-6 by highly metastatic colon cancer cells induces Kupffer cells to polarize to the M2 type, and KCs M2 polarization is essential for promoting the metastatic ability of colon cancer cells. Toadflax (BU) regulates KCs M2-type polarization and inhibits colon cancer liver metastasis through the SRC-3/IL-6 pathway (170). Astragaloside IV remodels tumor-associated macrophage (TAM) polarization by decreasing M2-type polarization and increasing M1-type polarization, and it inhibits CRC liver metastasis, reduces M2-type macrophage infiltration, and decreases nSMase2 and Rab27a expression in liver metastasis (171). Exosomal CC chemokine ligand-2 (CCL2) promotes the formation of metastatic ecotopes by activating macrophage recruitment and shifting the M1/M2 paradigm to the M2 phenotype. The classic formula in the “Compendium of Golden Chamber Medicinal Formulas” has been used for the treatment of abdominal masses (including tumor diseases), Rhubarb stinging pill, which is mainly used in the clinical treatment of liver fibrosis to reduce hepatic collagen levels, ECM production, and TGF-β1 levels. This formula inhibits the expression of CCL2 in the liver, thereby attenuating macrophage recruitment and polarization to improve the pre-metastatic niche, and then inhibit the liver metastasis of CRC (172). Atractylenolide II (AT-II) inhibits IL-4/IL-13-induced activation of the STAT6 signaling pathway to reduce M2-type macrophage polarization, resulting in fewer lung metastatic nodules (173). Macrophages modified by CAR (CAR-M) have also been initiated for the treatment of solid tumors (174).
Currently, using immunotherapy against liver metastases presents a challenge, often associated with macrophage-mediated factors (175). Therapeutic strategies targeting macrophages include tumor-associated macrophage (TAM) depletion, CAR-M series, and M2-type macrophage repolarization (46). For TAM depletion, some studies have suggested using CAR-T cells targeting FRβ+ TAMs to promote their depletion, thereby enhancing endogenous anti-tumor immunity. This offers a promising direction for future immunotherapeutic approaches (176). Regarding macrophage-promoted liver metastasis, with the advent of single-cell sequencing technology and our deeper understanding of TAM subpopulations, we will be able to further explore the relationships between different subpopulations and liver metastasis, facilitating the discovery of new therapeutic targets. In the tumor microenvironment, there is a correlation between TAM heterogeneity and the mechanisms of liver metastasis. Currently, there are limited studies on TAM-related subpopulations and liver metastasis. In the future, we can continue to use single-cell technology and multi-omics analysis to gain a deeper understanding of TAM subpopulations. However, due to the inconsistency in criteria and methods for defining and evaluating TAM heterogeneity, in-depth study of standard TAM subpopulation classification faces challenges. As for current single-cell sequencing, I believe that in the future, SPP1+ TAMs may be an ideal target for adjuvant immunotherapy and improving immunotherapeutic efficacy against C1q+ TAMs, which primarily contribute to the formation of an immunosuppressive microenvironment. However, the intracellular role of C1q+ TAMs within TAMs has not yet been extensively studied. Whether C1q+ TAMs can be used as more specific therapeutic targets in anticancer therapy in the future, or in combination with immune checkpoint inhibitors in cases of resistance, these are promising areas for future exploration. I believe that future research should focus more on how specific subpopulations of tumor-associated macrophages (TAMs) contribute to tumor cell metastasis. Immunotherapy has emerged as a major cancer treatment, but its benefits are limited in patients with liver metastases due to the development of immunotherapeutic resistance. This resistance is often attributed to macrophages, which can affect the efficacy of immune checkpoint blockade. The liver tumor environment is particularly influenced by macrophages, which can impair the recruitment of CD8+ T cells by immunotherapy, leading to immunosuppression in the tumor microenvironment. Therefore, before initiating immunotherapy, it is crucial to identify appropriate treatments to alter the immunosuppressive microenvironment of liver metastases. Research is ongoing to improve drug delivery technologies, such as nanotechnology. The use of nanocarriers may enhance drug accumulation in the liver while minimizing potential negative effects on healthy cells. One study demonstrated that the synergistic treatment of nano-agents with immune checkpoint inhibitors (ICIs) can repolarize macrophages and rejuvenate the liver by remodeling the immunosuppressive microenvironment (146). Another study showed that co-treatment of nano-agents with ICIs could improve the efficacy of immunotherapy by repolarizing macrophages (146). Zhaoting Li et al. (177) utilized a biocompatible alginate-based hydrogel to target Pexidartinib (PLX) to the tumor site and release PLX to block the colony-stimulating factor 1 receptor (CSF1R), thereby depleting TAMs. TAM depletion creates a favorable environment for the local and systemic delivery of anti-programmed cell death protein 1 (aPD-1) antibody-bound platelets to inhibit post-surgical tumor recurrence. The use of nano-agents to induce repolarization of M2-type macrophages improves immunotherapy, offering a better option for overcoming immunotherapy resistance. A lentiviral vector (LV) has also been proposed to deliver interferon alpha (IFNα) to liver metastases via liver macrophages, inhibiting the growth of liver metastases through the activation of antigen presentation and CD8+ T-cell effector function. The use of IFNα in combination with CTLA-4 antibody resolved some resistance mechanisms, such as increased IL-10 signaling, decreased MHC-II restricted antigen presentation in antigen-presenting cells (APCs), and enhanced CTLA-4 expression in T cells (120). In the future, more combination therapies can be explored to target macrophages to release immunosuppression and overcome immunoresistance, including the inhibition of M2-type macrophage polarization and immunotherapy combination therapy. In the tumor microenvironment, TAMs account for a large proportion, and there are other immune cells present, so how to target TAM-mediated liver metastasis is a question to consider. Constructing M2-type macrophage-targeting nanoparticles or using TAM-specific surface markers to design drugs targeting TAM may be viable strategies. With the emergence of new TAM subpopulations identified by single-cell sequencing, this could represent a novel therapeutic approach. It may also be possible to induce the conversion of M2-type macrophages to M1-type by targeting specific signaling pathways associated with the infiltration and polarization of M2-type macrophages, such as the CCL2-CCR2 axis and the STAT3 signaling pathway. Inducing the conversion of M2-type macrophages to M1-type may be a strategy that does not affect other cells, potentially achievable through the use of CpG-targeted liposomes or activation of the TLR signaling pathway. Gene editing techniques may also enable targeting of M2-type macrophages in the future. As new macrophage subpopulations are discovered through single-cell sequencing, drug design studies could involve multiple and more specific targets for drug action. Currently, the identification of macrophage subpopulations needs to be more in-depth to determine the unique functional and phenotypic markers of different subpopulations in order to more accurately target TAMs, which will be more beneficial for highlighting the anticancer therapeutic effects. In the future, TAM subpopulations will become more specific with technologies such as NanoString digital spatial analysis, mass RNA sequencing, or single-cell sequencing, and the newly discovered TAM subpopulations can be used as novel targets. Combination therapies can be developed to inhibit liver metastasis of tumor cells by breaking tumor cell growth or reversing drug resistance. Continued refinement of the delivery system has the potential to improve drug delivery to the tumor microenvironment (TME) and overcome resistance mechanisms. Additionally, emphasis should be placed on the design and rigorous execution of clinical trials, including the promotion of multicenter study designs to improve reliability and the optimization of randomized controlled trial designs to accurately assess the efficacy of targeted macrophage therapy.
In this paper, it is mentioned that tumor-associated macrophages (TAMs) promote tumor cell growth and liver metastasis through angiogenesis, immunosuppression, and the promotion of epithelial-mesenchymal transition, among other mechanisms. Previously, macrophage classification was limited to the M subpopulations, and research on the influence of the M2 subpopulation on tumor cell development was more restricted. TAMs are heterogeneous, making it challenging to define their subpopulations and functions. However, with the advent of single-cell sequencing technology, macrophage subpopulations have become more specific and detailed. This advancement provides more targets for targeting TAMs to inhibit tumor cell metastasis and offers a deeper understanding of strategies to address resistance to immune checkpoint inhibitors. It enhances the prospects for improving therapeutic efficacy in cancer patients through the in-depth study of macrophage subpopulations and innovative therapeutic approaches. Despite the many hurdles ahead, continued exploration of TAMs is essential. TAMs represent a novel and attractive target that may transform the future landscape of cancer treatment and warrant further research efforts.
QY: Writing – original draft. LJ: Writing – review & editing. JY: Writing – review & editing. WL: Funding acquisition, Writing – review & editing.
The author(s) declare financial support was received for the research, authorship, and/or publication of this article. This study was sponsored by the One Hundred Talents Project of Putuo Hospital, Shanghai University of Traditional Chinese Medicine (2022-RCLH-03). Shanghai Health System Key Disciplines - General Surgery (2024ZDXK0046). Shanghai Putuo District Health and Wellness System Science and Technology Innovation Project Plan(ptkwws202308).
The authors declare that the research was conducted in the absence of any commercial or financial relationships that could be construed as a potential conflict of interest.
The author(s) declare that no Generative AI was used in the creation of this manuscript.
All claims expressed in this article are solely those of the authors and do not necessarily represent those of their affiliated organizations, or those of the publisher, the editors and the reviewers. Any product that may be evaluated in this article, or claim that may be made by its manufacturer, is not guaranteed or endorsed by the publisher.
1. Sendi H, Yazdimamaghani M, Hu M, Sultanpuram N, Wang J, Moody AS, et al. Nanoparticle delivery of miR-122 inhibits colorectal cancer liver metastasis. Cancer Res. (2022) 82:105–13. doi: 10.1158/0008-5472.CAN-21-2269
2. Wang D, Sun H, Wei J, Cen B, DuBois RN. CXCL1 is critical for premetastatic niche formation and metastasis in colorectal cancer. Cancer Res. (2017) 77:3655–65. doi: 10.1158/0008-5472.CAN-16-3199
3. Hao NB, Lü MH, Fan YH, Cao YL, Zhang ZR, Yang SM. Macrophages in tumor microenvironments and the progression of tumors. Clin Dev Immunol. (2012) 2012:948098. doi: 10.1155/2012/948098
4. Li C, Xu X, Wei S, Jiang P, Xue L, Wang J. Tumor-associated macrophages: potential therapeutic strategies and future prospects in cancer. J Immunother Cancer. (2021) 9(1):e001341. doi: 10.1136/jitc-2020-001341
5. Cassetta L, Pollard JW. Tumor-associated macrophages. Curr Biol. (2020) 30:R246–r248. doi: 10.1016/j.cub.2020.01.031
6. Boutilier AJ, Elsawa SF. Macrophage polarization states in the tumor microenvironment. Int J Mol Sci. (2021) 22(13):6995. doi: 10.3390/ijms22136995
7. Zhu S, Yi M, Wu Y, Dong B, Wu K. Roles of tumor-associated macrophages in tumor progression: implications on therapeutic strategies. Exp Hematol Oncol. (2021) 10:60. doi: 10.1186/s40164-021-00252-z
8. Colegio OR. Lactic acid polarizes macrophages to a tumor-promoting state. Oncoimmunology. (2016) 5:e1014774. doi: 10.1080/2162402X.2015.1014774
9. Li M, Yang Y, Xiong L, Jiang P, Wang J, Li C. Metabolism, metabolites, and macrophages in cancer. J Hematol Oncol. (2023) 16:80. doi: 10.1186/s13045-023-01478-6
10. Wang Q, Sudan K, Schmoeckel E, Kost BP, Kuhn C, Vattai A, et al. CCL22-polarized TAMs to M2a macrophages in cervical cancer in vitro model. Cells. (2022) 11(13):2027. doi: 10.3390/cells11132027
11. Liu S, Zhang H, Li Y, Zhang Y, Bian Y, Zeng Y, et al. S100A4 enhances protumor macrophage polarization by control of PPAR-γ-dependent induction of fatty acid oxidation. J Immunother Cancer. (2021) 9(6):e002548. doi: 10.1136/jitc-2021-002548
12. Ishida K, Nagatake T, Saika A, Kawai S, Node E, Hosomi K, et al. Induction of unique macrophage subset by simultaneous stimulation with LPS and IL-4. Front Immunol. (2023) 14:1111729. doi: 10.3389/fimmu.2023.1111729
13. Yang G, Yang Y, Liu Y, Liu X. Regulation of alveolar macrophage death in pulmonary fibrosis: a review. Apoptosis. (2023) 28:1505–19. doi: 10.1007/s10495-023-01888-4
14. Wei Y, Li R, Wang Y, Fu J, Liu J, Ma X. Nanomedicines targeting tumor cells or tumor-associated macrophages for combinatorial cancer photodynamic therapy and immunotherapy: strategies and influencing factors. Int J Nanomedicine. (2024) 19:10129–44. doi: 10.2147/IJN.S466315
15. Hu T, Pang N, Li Z, Xu D, Jing J, Li F, et al. The activation of M1 macrophages is associated with the JNK-m6A-p38 axis in chronic obstructive pulmonary disease. Int J Chron Obstruct Pulmon Dis. (2023) 18:2195–206. doi: 10.2147/COPD.S420471
16. Chen W, Wang Y, Zhou Y, Xu Y, Bo X, Wu J. M1 Macrophages Increase Endothelial Permeability and Enhance p38 Phosphorylation via PPAR-γ/CXCL13-CXCR5 in Sepsis. Int Arch Allergy Immunol. (2022) 183:997–1006. doi: 10.1159/000524272
17. Li Y, Li X, Ju S, Li W, Zhou S, Wang G, et al. Role of M1 macrophages in diabetic foot ulcers and related immune regulatory mechanisms. Front Pharmacol. (2022) 13:1098041. doi: 10.3389/fphar.2022.1098041
18. Kaplan M, Shur A, Tendler Y. M1 Macrophages but Not M2 Macrophages Are Characterized by Upregulation of CRP Expression via Activation of NFκB: a Possible Role for Ox-LDL in Macrophage Polarization. Inflammation. (2018) 41:1477–87. doi: 10.1007/s10753-018-0793-8
19. Zhu LF, Li L, Wang XQ, Pan L, Mei YM, Fu YW, et al. M1 macrophages regulate TLR4/AP1 via paracrine to promote alveolar bone destruction in periodontitis. Oral Dis. (2019) 25:1972–82. doi: 10.1111/odi.13167
20. Wang L, Yi X, Xiao X, Zheng Q, Ma L, Li B. Exosomal miR-628-5p from M1 polarized macrophages hinders m6A modification of circFUT8 to suppress hepatocellular carcinoma progression. Cell Mol Biol Lett. (2022) 27:106. doi: 10.1186/s11658-022-00406-9
21. Gao L, Zhou Y, Zhou SX, Yu XJ, Xu JM, Zuo L, et al. PLD4 promotes M1 macrophages to perform antitumor effects in colon cancer cells. Oncol Rep. (2017) 37:408–16. doi: 10.3892/or.2016.5216
22. Li Z, Suo B, Long G, Gao Y, Song J, Zhang M, et al. Exosomal miRNA-16-5p derived from M1 macrophages enhances T cell-dependent immune response by regulating PD-L1 in gastric cancer. Front Cell Dev Biol. (2020) 8:572689. doi: 10.3389/fcell.2020.572689
23. Han Y, Sun J, Yang Y, Liu Y, Lou J, Pan H, et al. TMP195 exerts antitumor effects on colorectal cancer by promoting M1 macrophages polarization. Int J Biol Sci. (2022) 18:5653–66. doi: 10.7150/ijbs.73264
24. Li W, Ye Q, Jiang Z, Xia D, Yan Z, Wang D, et al. A cross-linked macropore hydrogel based on M1 macrophage lysate and alginate regulates tumor-associated macrophages for the treatment of melanoma. Int J Biol Macromol. (2024) 269:132089. doi: 10.1016/j.ijbiomac.2024.132089
25. Chávez-Galán L, Olleros ML, Vesin D, Garcia I. Much More than M1 and M2 Macrophages, There are also CD169(+) and TCR(+) Macrophages. Front Immunol. (2015) 6:263. doi: 10.3389/fimmu.2015.00263
26. Gunassekaran GR, Poongkavithai Vadevoo SM, Baek MC, Lee B. M1 macrophage exosomes engineered to foster M1 polarization and target the IL-4 receptor inhibit tumor growth by reprogramming tumor-associated macrophages into M1-like macrophages. Biomaterials. (2021) 278:121137. doi: 10.1016/j.biomaterials.2021.121137
27. Teng Y, Mu J, Hu X, Samykutty A, Zhuang X, Deng Z, et al. Grapefruit-derived nanovectors deliver miR-18a for treatment of liver metastasis of colon cancer by induction of M1 macrophages. Oncotarget. (2016) 7:25683–97. doi: 10.18632/oncotarget.8361
28. Hu W, Lin J, Lian X, Yu F, Liu W, Wu Y, et al. M2a and M2b macrophages predominate in kidney tissues and M2 subpopulations were associated with the severity of disease of IgAN patients. Clin Immunol. (2019) 205:8–15. doi: 10.1016/j.clim.2019.05.005
29. Huang J, Chen Y, Zhou L, Ren J, Tian M, Yang Q, et al. M2a macrophages regulate fibrosis and affect the outcome after stroke via PU.1/mTOR pathway in fibroblasts. Neurochem Int. (2024) 173:105674. doi: 10.1016/j.neuint.2024.105674
30. Liu D, Wei Y, Liu Y, Wu T, Hu J, Lu H. The long non-coding RNA NEAT1/miR-224-5p/IL-33 axis modulates macrophage M2a polarization and A1 astrocyte activation. Mol Neurobiol. (2021) 58:4506–19. doi: 10.1007/s12035-021-02405-x
31. Li L, Wei K, Ding Y, Ahati P, Xu H, Fang H, et al. M2a macrophage-secreted CHI3L1 promotes extracellular matrix metabolic imbalances via activation of IL-13Rα2/MAPK pathway in rat intervertebral disc degeneration. Front Immunol. (2021) 12:666361. doi: 10.3389/fimmu.2021.666361
32. Zhang Q, Sioud M. Tumor-associated macrophage subsets: shaping polarization and targeting. Int J Mol Sci. (2023) 24(8):7493. doi: 10.3390/ijms24087493
33. Wang LX, Zhang SX, Wu HJ, Rong XL, Guo J. M2b macrophage polarization and its roles in diseases. J Leukoc Biol. (2019) 106:345–58. doi: 10.1002/JLB.3RU1018-378RR
34. Yang R, Liao Y, Wang L, He P, Hu Y, Yuan D, et al. Exosomes derived from M2b macrophages attenuate DSS-induced colitis. Front Immunol. (2019) 10:2346. doi: 10.3389/fimmu.2019.02346
35. Zhang Z, Leng Z, Kang L, Yan X, Shi J, Ji Y, et al. Alcohol inducing macrophage M2b polarization in colitis by modulating the TRPV1-MAPK/NF-κB pathways. Phytomedicine. (2024) 130:155580. doi: 10.1016/j.phymed.2024.155580
36. Liu Y, Xue M, Han Y, Li Y, Xiao B, Wang W, et al. Exosomes from M2c macrophages alleviate intervertebral disc degeneration by promoting synthesis of the extracellular matrix via MiR-124/CILP/TGF-β. Bioeng Transl Med. (2023) 8:e10500. doi: 10.1002/btm2.10500
37. Bayat M, Sarojini H, Chien S. The role of cluster of differentiation 163-positive macrophages in wound healing: a preliminary study and a systematic review. Arch Dermatol Res. (2023) 315:359–70. doi: 10.1007/s00403-022-02407-2
38. Chen Z, Dong F, Lu J, Wei L, Tian L, Ge H, et al. Polarized M2c macrophages have a promoting effect on the epithelial-to-mesenchymal transition of human renal tubular epithelial cells. Immunobiology. (2018) 223:826–33. doi: 10.1016/j.imbio.2018.08.008
39. Sun Z, Xu Y, Shao B, Dang P, Hu S, Sun H, et al. Exosomal circPOLQ promotes macrophage M2 polarization via activating IL-10/STAT3 axis in a colorectal cancer model. J Immunother Cancer. (2024) 12(5):e008491. doi: 10.1136/jitc-2023-008491
40. Zhang X, Sun X, Guo C, Li J, Liang G. Cancer-associated fibroblast-associated gene IGFBP2 promotes glioma progression through induction of M2 macrophage polarization. Am J Physiol Cell Physiol. (2024) 326:C252–c268. doi: 10.1152/ajpcell.00234.2023
41. Liang Y, Li J, Yuan Y, Ju H, Liao H, Li M, et al. Exosomal miR-106a-5p from highly metastatic colorectal cancer cells drives liver metastasis by inducing macrophage M2 polarization in the tumor microenvironment. J Exp Clin Cancer Res. (2024) 43:281. doi: 10.1186/s13046-024-03204-7
42. Bian Z, Wu X, Chen Q, Gao Q, Xue X, Wang Y. Oct4 activates IL-17A to orchestrate M2 macrophage polarization and cervical cancer metastasis. Cancer Immunol Immunother. (2024) 73:73. doi: 10.1007/s00262-023-03596-z
43. Huang C, Xue L, Lin X, Shen Y, Wang X. Histone lactylation-driven GPD2 mediates M2 macrophage polarization to promote Malignant transformation of cervical cancer progression. DNA Cell Biol. (2024) 43:605–18. doi: 10.1089/dna.2024.0122
44. Dong Y, Wang G, Nie D, Xu Y, Bai X, Lu C, et al. Tumor-derived GABA promotes lung cancer progression by influencing TAMs polarization and neovascularization. Int Immunopharmacol. (2024) 126:111217. doi: 10.1016/j.intimp.2023.111217
45. Mantovani A, Sozzani S, Locati M, Allavena P, Sica A. Macrophage polarization: tumor-associated macrophages as a paradigm for polarized M2 mononuclear phagocytes. Trends Immunol. (2002) 23:549–55. doi: 10.1016/s1471-4906(02)02302-5
46. Wang Y, Barrett A, Hu Q. Targeting macrophages for tumor therapy. AAPS J. (2023) 25:80. doi: 10.1208/s12248-023-00845-y
47. Wu K, Lin K, Li X, Yuan X, Xu P, Ni P, et al. Redefining tumor-associated macrophage subpopulations and functions in the tumor microenvironment. Front Immunol. (2020) 11:1731. doi: 10.3389/fimmu.2020.01731
48. Irey EA, Lassiter CM, Brady NJ, Chuntova P, Wang Y, Knutson TP, et al. JAK/STAT inhibition in macrophages promotes therapeutic resistance by inducing expression of protumorigenic factors. Proc Natl Acad Sci U.S.A. (2019) 116:12442–51. doi: 10.1073/pnas.1816410116
49. Yang L, Zhang Y. Tumor-associated macrophages: from basic research to clinical application. J Hematol Oncol. (2017) 10:58. doi: 10.1186/s13045-017-0430-2
50. Imano M, Okuno K, Itoh T, Ishimaru E, Satou T, Shiozaki H. Increased osteopontin-positive macrophage expression in colorectal cancer stroma with synchronous liver metastasis. World J Surg. (2010) 34:1930–6. doi: 10.1007/s00268-010-0582-5
51. Tu W, Gong J, Zhou Z, Tian D, Wang Z. TCF4 enhances hepatic metastasis of colorectal cancer by regulating tumor-associated macrophage via CCL2/CCR2 signaling. Cell Death Dis. (2021) 12:882. doi: 10.1038/s41419-021-04166-w
52. Kruse J, von Bernstorff W, Evert K, Albers N, Hadlich S, Hagemann S, et al. Macrophages promote tumour growth and liver metastasis in an orthotopic syngeneic mouse model of colon cancer. Int J Colorectal Dis. (2013) 28:1337–49. doi: 10.1007/s00384-013-1703-z
53. Shimizu Y, Amano H, Ito Y, Betto T, Yamane S, Inoue T, et al. Angiotensin II subtype 1a receptor signaling in resident hepatic macrophages induces liver metastasis formation. Cancer Sci. (2017) 108:1757–68. doi: 10.1111/cas.13306
54. Miao L, Zhuo Z, Tang J, Huang X, Liu J, Wang HY, et al. FABP4 deactivates NF-κB-IL1α pathway by ubiquitinating ATPB in tumor-associated macrophages and promotes neuroblastoma progression. Clin Transl Med. (2021) 11:e395. doi: 10.1002/ctm2.395
55. Jung M, Ören B, Mora J, Mertens C, Dziumbla S, Popp R, et al. Lipocalin 2 from macrophages stimulated by tumor cell-derived sphingosine 1-phosphate promotes lymphangiogenesis and tumor metastasis. Sci Signal. (2016) 9:ra64. doi: 10.1126/scisignal.aaf3241
56. Chen J, Sun W, Zhang H, Ma J, Xu P, Yu Y, et al. Macrophages reprogrammed by lung cancer microparticles promote tumor development via release of IL-1β. Cell Mol Immunol. (2020) 17:1233–44. doi: 10.1038/s41423-019-0313-2
57. Li R, Serrano JC, Xing H, Lee TA, Azizgolshani H, Zaman M, et al. Interstitial flow promotes macrophage polarization toward an M2 phenotype. Mol Biol Cell. (2018) 29:1927–40. doi: 10.1091/mbc.E18-03-0164
58. Zhou Z, Wang P, Sun R, Li J, Hu Z, Xin H, et al. Tumor-associated neutrophils and macrophages interaction contributes to intrahepatic cholangiocarcinoma progression by activating STAT3. J Immunother Cancer. (2021) 9(3):e001946. doi: 10.1136/jitc-2020-001946
59. Zhang XL, Hu LP, Yang Q, Qin WT, Wang X, Xu CJ, et al. CTHRC1 promotes liver metastasis by reshaping infiltrated macrophages through physical interactions with TGF-β receptors in colorectal cancer. Oncogene. (2021) 40:3959–73. doi: 10.1038/s41388-021-01827-0
60. Li S, Guo D, Sun Q, Zhang L, Cui Y, Liu M, et al. MAPK4 silencing in gastric cancer drives liver metastasis by positive feedback between cancer cells and macrophages. Exp Mol Med. (2023) 55:457–69. doi: 10.1038/s12276-023-00946-w
61. Liu B, Jia Y, Ma J, Wu S, Jiang H, Cao Y, et al. Tumor-associated macrophage-derived CCL20 enhances the growth and metastasis of pancreatic cancer. Acta Biochim Biophys Sin (Shanghai). (2016) 48:1067–74. doi: 10.1093/abbs/gmw101
62. Yang P, Qin H, Li Y, Xiao A, Zheng E, Zeng H, et al. CD36-mediated metabolic crosstalk between tumor cells and macrophages affects liver metastasis. Nat Commun. (2022) 13:5782. doi: 10.1038/s41467-022-33349-y
63. Komohara Y, Ohnishi K, Takeya M. Possible functions of CD169-positive sinus macrophages in lymph nodes in anti-tumor immune responses. Cancer Sci. (2017) 108:290–5. doi: 10.1111/cas.13137
64. Tacconi C, Commerford CD, Dieterich LC, Schwager S, He Y, Ikenberg K, et al. CD169(+) lymph node macrophages have protective functions in mouse breast cancer metastasis. Cell Rep. (2021) 35:108993. doi: 10.1016/j.celrep.2021.108993
65. Song X, Li N, Liu Y, Wang Z, Wang T, Tan S, et al. CD169-positive macrophages enhance abscopal effect of radiofrequency ablation therapy in liver cancer. Transl Oncol. (2022) 15:101306. doi: 10.1016/j.tranon.2021.101306
66. Wang J, Zhu N, Su X, Gao Y, Yang R. Novel tumor-associated macrophage populations and subpopulations by single cell RNA sequencing. Front Immunol. (2023) 14:1264774. doi: 10.3389/fimmu.2023.1264774
67. Liu Y, Zhang Q, Xing B, Luo N, Gao R, Yu K, et al. Immune phenotypic linkage between colorectal cancer and liver metastasis. Cancer Cell. (2022) 40:424–437.e5. doi: 10.1016/j.ccell.2022.02.013
68. Sathe A, Mason K, Grimes SM, Zhou Z, Lau BT, Bai X, et al. Colorectal cancer metastases in the liver establish immunosuppressive spatial networking between tumor-associated SPP1+ Macrophages and fibroblasts. Clin Cancer Res. (2023) 29:244–60. doi: 10.1158/1078-0432.CCR-22-2041
69. Dong L, Hu S, Li X, Pei S, Jin L, Zhang L, et al. SPP1(+) TAM regulates the metastatic colonization of CXCR4(+) metastasis-associated tumor cells by remodeling the lymph node microenvironment. Adv Sci (Weinh). (2024) 11:e2400524. doi: 10.1002/advs.202400524
70. Liu X, Qin J, Nie J, Gao R, Hu S, Sun H, et al. ANGPTL2+cancer-associated fibroblasts and SPP1+macrophages are metastasis accelerators of colorectal cancer. Front Immunol. (2023) 14:1185208. doi: 10.3389/fimmu.2023.1185208
71. Wang Z, Liu J, Wang X, Wu Q, Peng Q, Yang T, et al. Glycosyltransferase B4GALNT1 promotes immunosuppression in hepatocellular carcinoma via the HES4-SPP1-TAM/Th2 axis. Mol BioMed. (2024) 5:65. doi: 10.1186/s43556-024-00231-w
72. Korbecki J, Olbromski M, Dzięgiel P. CCL18 in the progression of cancer. Int J Mol Sci. (2020) 21(21):7955. doi: 10.3390/ijms21217955
73. Yang Q, Zhang H, Wei T, Lin A, Sun Y, Luo P, et al. Single-cell RNA sequencing reveals the heterogeneity of tumor-associated macrophage in non-small cell lung cancer and differences between sexes. Front Immunol. (2021) 12:756722. doi: 10.3389/fimmu.2021.756722
74. Revel M, Sautès-Fridman C, Fridman WH, Roumenina LT. C1q+ macrophages: passengers or drivers of cancer progression. Trends Cancer. (2022) 8:517–26. doi: 10.1016/j.trecan.2022.02.006
75. Song H, Lou C, Ma J, Gong Q, Tian Z, You Y, et al. Single-cell transcriptome analysis reveals changes of tumor immune microenvironment in oral squamous cell carcinoma after chemotherapy. Front Cell Dev Biol. (2022) 10:914120. doi: 10.3389/fcell.2022.914120
76. Iurca I, Tirpe A, Zimta AA, Moldovan C, Gulei D, Slabý O, et al. Berindan-neagoe, macrophages interaction and microRNA interplay in the modulation of cancer development and metastasis. Front Immunol. (2020) 11:870. doi: 10.3389/fimmu.2020.00870
77. Song W, Mazzieri R, Yang T, Gobe GC. Translational significance for tumor metastasis of tumor-associated macrophages and epithelial-mesenchymal transition. Front Immunol. (2017) 8:1106. doi: 10.3389/fimmu.2017.01106
78. Zhang T, Liu L, Lai W, Zeng Y, Xu H, Lan Q, et al. Interaction with tumor−associated macrophages promotes PRL−3−induced invasion of colorectal cancer cells via MAPK pathway−induced EMT and NF−κB signaling−induced angiogenesis. Oncol Rep. (2019) 41:2790–802. doi: 10.3892/or.2019.7049
79. Wang H, Tian T, Zhang J. Tumor-associated macrophages (TAMs) in colorectal cancer (CRC): from mechanism to therapy and prognosis. Int J Mol Sci. (2021) 22(16):8470. doi: 10.3390/ijms22168470
80. Chen Y, Song Y, Du W, Gong L, Chang H, Zou Z. Tumor-associated macrophages: an accomplice in solid tumor progression. J BioMed Sci. (2019) 26:78. doi: 10.1186/s12929-019-0568-z
81. Yang Y, Guo Z, Chen W, Wang X, Cao M, Han X, et al. M2 macrophage-derived exosomes promote angiogenesis and growth of pancreatic ductal adenocarcinoma by targeting E2F2. Mol Ther. (2021) 29:1226–38. doi: 10.1016/j.ymthe.2020.11.024
82. Wang D, Wang X, Si M, Yang J, Sun S, Wu H, et al. Exosome-encapsulated miRNAs contribute to CXCL12/CXCR4-induced liver metastasis of colorectal cancer by enhancing M2 polarization of macrophages. Cancer Lett. (2020) 474:36–52. doi: 10.1016/j.canlet.2020.01.005
83. Mielgo A, Schmid MC. Liver tropism in cancer: the hepatic metastatic niche. Cold Spring Harb Perspect Med. (2020) 10(3):a037259. doi: 10.1101/cshperspect.a037259
84. Kang X, Huang Y, Wang H, Jadhav S, Yue Z, Tiwari AK, et al. Tumor-associated macrophage targeting of nanomedicines in cancer therapy. Pharmaceutics. (2023) 16(1):61. doi: 10.3390/pharmaceutics16010061
85. Gu J, Xu X, Li X, Yue L, Zhu X, Chen Q, et al. Tumor-resident microbiota contributes to colorectal cancer liver metastasis by lactylation and immune modulation. Oncogene. (2024) 43:2389–404. doi: 10.1038/s41388-024-03080-7
86. Okuno K, Jinnai H, Lee YS, Nakamura K, Hirohata T, Shigeoka H, et al. A high level of prostaglandin E2 (PGE2) in the portal vein suppresses liver-associated immunity and promotes liver metastases. Surg Today. (1995) 25:954–8. doi: 10.1007/BF00312380
87. Wu H, Xu JB, He YL, Peng JJ, Zhang XH, Chen CQ, et al. Tumor-associated macrophages promote angiogenesis and lymphangiogenesis of gastric cancer. J Surg Oncol. (2012) 106:462–8. doi: 10.1002/jso.23110
88. Yue L, Xu X, Dai S, Xu F, Zhao W, Gu J, et al. Orosomucoid 1 promotes colorectal cancer progression and liver metastasis by affecting PI3K/AKT pathway and inducing macrophage M2 polarization. Sci Rep. (2023) 13:14092. doi: 10.1038/s41598-023-40404-1
89. Raymant M, Astuti Y, Alvaro-Espinosa L, Green D, Quaranta V, Bellomo G, et al. Macrophage-fibroblast JAK/STAT dependent crosstalk promotes liver metastatic outgrowth in pancreatic cancer. Nat Commun. (2024) 15:3593. doi: 10.1038/s41467-024-47949-3
90. Pei W, Wei K, Wu Y, Qiu Q, Zhu H, Mao L, et al. Colorectal cancer tumor cell-derived exosomal miR-203a-3p promotes CRC metastasis by targeting PTEN-induced macrophage polarization. Gene. (2023) 885:147692. doi: 10.1016/j.gene.2023.147692
91. Heo W, Lee W, Cheun JH, Lee ES, Li S, Kim HS, et al. Triple-negative breast cancer-derived extracellular vesicles promote a hepatic premetastatic niche via a cascade of microenvironment remodeling. Mol Cancer Res. (2023) 21:726–40. doi: 10.1158/1541-7786.MCR-22-0673
92. Lu F, Ye M, Shen Y, Xu Y, Hu C, Chen J, et al. Hypoxic tumor-derived exosomal miR-4488 induces macrophage M2 polarization to promote liver metastasis of pancreatic neuroendocrine neoplasm through RTN3/FABP5 mediated fatty acid oxidation. Int J Biol Sci. (2024) 20:3201–18. doi: 10.7150/ijbs.96831
93. Shao Y, Chen T, Zheng X, Yang S, Xu K, Chen X, et al. Colorectal cancer-derived small extracellular vesicles establish an inflammatory premetastatic niche in liver metastasis. Carcinogenesis. (2018) 39:1368–79. doi: 10.1093/carcin/bgy115
94. Costa-Silva B, Aiello NM, Ocean AJ, Singh S, Zhang H, Thakur BK, et al. Pancreatic cancer exosomes initiate pre-metastatic niche formation in the liver. Nat Cell Biol. (2015) 17:816–26. doi: 10.1038/ncb3169
95. Zhou J, Song Q, Li H, Han Y, Pu Y, Li L, et al. Targeting circ-0034880-enriched tumor extracellular vesicles to impede SPP1(high)CD206(+) pro-tumor macrophages mediated pre-metastatic niche formation in colorectal cancer liver metastasis. Mol Cancer. (2024) 23:168. doi: 10.1186/s12943-024-02086-9
96. Chang MM, Wu SZ, Yang SH, Wu CC, Wang CY, Huang BM. FGF9/FGFR1 promotes cell proliferation, epithelial-mesenchymal transition, M2 macrophage infiltration and liver metastasis of lung cancer. Transl Oncol. (2021) 14:101208. doi: 10.1016/j.tranon.2021.101208
97. Liu CY, Xu JY, Shi XY, Huang W, Ruan TY, Xie P, et al. M2-polarized tumor-associated macrophages promoted epithelial-mesenchymal transition in pancreatic cancer cells, partially through TLR4/IL-10 signaling pathway. Lab Invest. (2013) 93:844–54. doi: 10.1038/labinvest.2013.69
98. Cai H, Chen Y, Chen X, Sun W, Li Y. Tumor-associated macrophages mediate gastrointestinal stromal tumor cell metastasis through CXCL2/CXCR2. Cell Immunol. (2023) 384:104642. doi: 10.1016/j.cellimm.2022.104642
99. Hu WM, Liu SQ, Zhu KF, Li W, Yang ZJ, Yang Q, et al. The ALOX5 inhibitor Zileuton regulates tumor-associated macrophage M2 polarization by JAK/STAT and inhibits pancreatic cancer invasion and metastasis. Int Immunopharmacol. (2023) 121:110505. doi: 10.1016/j.intimp.2023.110505
100. Ni B, He X, Zhang Y, Wang Z, Dong Z, Xia X, et al. Tumor-associated macrophage-derived GDNF promotes gastric cancer liver metastasis via a GFRA1-modulated autophagy flux. Cell Oncol (Dordr). (2023) 46:315–30. doi: 10.1007/s13402-022-00751-z
101. Cocozza F, Grisard E, Martin-Jaular L, Mathieu M, Théry C. SnapShot: extracellular vesicles. Cell. (2020) 182:262–262.e1. doi: 10.1016/j.cell.2020.04.054
102. Meng W, Hao Y, He C, Li L, Zhu G. Exosome-orchestrated hypoxic tumor microenvironment. Mol Cancer. (2019) 18:57. doi: 10.1186/s12943-019-0982-6
103. Wei X, Ye J, Pei Y, Wang C, Yang H, Tian J, et al. Extracellular vesicles from colorectal cancer cells promote metastasis via the NOD1 signalling pathway. J Extracell Vesicles. (2022) 11:e12264. doi: 10.1002/jev2.12264
104. Li B, Xia Y, Lv J, Wang W, Xuan Z, Chen C, et al. miR-151a-3p-rich small extracellular vesicles derived from gastric cancer accelerate liver metastasis via initiating a hepatic stemness-enhancing niche. Oncogene. (2021) 40:6180–94. doi: 10.1038/s41388-021-02011-0
105. Qiu S, Xie L, Lu C, Gu C, Xia Y, Lv J, et al. Gastric cancer-derived exosomal miR-519a-3p promotes liver metastasis by inducing intrahepatic M2-like macrophage-mediated angiogenesis. J Exp Clin Cancer Res. (2022) 41:296. doi: 10.1186/s13046-022-02499-8
106. Chen W, Zhou M, Guan B, Xie B, Liu Y, He J, et al. Tumour-associated macrophage-derived DOCK7-enriched extracellular vesicles drive tumour metastasis in colorectal cancer via the RAC1/ABCA1 axis. Clin Transl Med. (2024) 14:e1591. doi: 10.1002/ctm2.1591
107. Chang YT, Peng HY, Hu CM, Huang SC, Tien SC, Jeng YM. Pancreatic cancer-derived small extracellular vesical Ezrin regulates macrophage polarization and promotes metastasis. Am J Cancer Res. (2020) 10:12–37. doi: 10.1158/1538-7445.SABCS19-BS1-2
108. Xu D, Chen WQ, Liang MX, Chen X, Liu Z, Fei YJ, et al. Tumor-derived small extracellular vesicles promote breast cancer progression by upregulating PD-L1 expression in macrophages. Cancer Cell Int. (2023) 23:137. doi: 10.1186/s12935-023-02980-0
109. Yin Y, Liu B, Cao Y, Yao S, Liu Y, Jin G, et al. Colorectal cancer-derived small extracellular vesicles promote tumor immune evasion by upregulating PD-L1 expression in tumor-associated macrophages. Adv Sci (Weinh). (2022) 9:2102620. doi: 10.1002/advs.202102620
110. Novizio N, Belvedere R, Pessolano E, Morello S, Tosco A, Campiglia P, et al. ANXA1 contained in EVs regulates macrophage polarization in tumor microenvironment and promotes pancreatic cancer progression and metastasis. Int J Mol Sci. (2021) 22(20):11018. doi: 10.3390/ijms222011018
111. Zhao S, Mi Y, Guan B, Zheng B, Wei P, Gu Y, et al. Tumor-derived exosomal miR-934 induces macrophage M2 polarization to promote liver metastasis of colorectal cancer. J Hematol Oncol. (2020) 13:156. doi: 10.1186/s13045-020-00991-2
112. Dai S, Zhuang H, Li Z, Chen Z, Chai Y, Zhou Q. miR-122/NEGR1 axis contributes colorectal cancer liver metastasis by PI3K/AKT pathway and macrophage modulation. J Transl Med. (2024) 22:1060. doi: 10.1186/s12967-024-05901-5
113. Zhou J, Li X, Wu X, Zhang T, Zhu Q, Wang X, et al. Exosomes released from tumor-associated macrophages transfer miRNAs that induce a treg/th17 cell imbalance in epithelial ovarian cancer. Cancer Immunol Res. (2018) 6:1578–92. doi: 10.1158/2326-6066.CIR-17-0479
114. Chen X, Zhou J, Li X, Wang X, Lin Y, Wang X. Exosomes derived from hypoxic epithelial ovarian cancer cells deliver microRNAs to macrophages and elicit a tumor-promoted phenotype. Cancer Lett. (2018) 435:80–91. doi: 10.1016/j.canlet.2018.08.001
115. Yin Z, Ma T, Huang B, Lin L, Zhou Y, Yan J, et al. Macrophage-derived exosomal microRNA-501-3p promotes progression of pancreatic ductal adenocarcinoma through the TGFBR3-mediated TGF-β signaling pathway. J Exp Clin Cancer Res. (2019) 38:310. doi: 10.1186/s13046-019-1313-x
116. Choi JY, Seok HJ, Lee DH, Lee E, Kim TJ, Bae S, et al. Tumor-derived miR-6794-5p enhances cancer growth by promoting M2 macrophage polarization. Cell Commun Signal. (2024) 22:190. doi: 10.1186/s12964-024-01570-5
117. Wang J, Wang Y, Jiang X, Xu M, Wang M, Wang R, et al. Unleashing the power of immune checkpoints: Post-translational modification of novel molecules and clinical applications. Cancer Lett. (2024) 588:216758. doi: 10.1016/j.canlet.2024.216758
118. Wang L, Fan J, Wu S, Cheng S, Zhao J, Fan F, et al. LTBR acts as a novel immune checkpoint of tumor-associated macrophages for cancer immunotherapy. Imeta. (2024) 3:e233. doi: 10.1002/imt2.233
119. Chen Y, Gong L, Cao Y, Liu Z, Wang Y, Cheng H, et al. Reprogramming tumor-associated macrophages by a dually targeted milk exosome system as a potent monotherapy for cancer. J Control Release. (2024) 366:395–409. doi: 10.1016/j.jconrel.2023.12.058
120. Kerzel T, Giacca G, Beretta S, Bresesti C, Notaro M, Scotti GM, et al. In vivo macrophage engineering reshapes the tumor microenvironment leading to eradication of liver metastases. Cancer Cell. (2023) 41:1892–1910.e10. doi: 10.1016/j.ccell.2023.09.014
121. Lu X, Li L, Lin J, Wu X, Li W, Tan C, et al. PAARH promotes M2 macrophage polarization and immune evasion of liver cancer cells through VEGF protein. Int J Biol Macromol. (2024) 281:136580. doi: 10.1016/j.ijbiomac.2024.136580
122. Yu Y, Liang Y, Xie F, Zhang Z, Zhang P, Zhao X, et al. Tumor-associated macrophage enhances PD-L1-mediated immune escape of bladder cancer through PKM2 dimer-STAT3 complex nuclear translocation. Cancer Lett. (2024) 593:216964. doi: 10.1016/j.canlet.2024.216964
123. Huang HY, Chen YZ, Zhao C, Zheng XN, Yu K, Yue JX, et al. Alternations in inflammatory macrophage niche drive phenotypic and functional plasticity of Kupffer cells. Nat Commun. (2024) 15:9337. doi: 10.1038/s41467-024-53659-7
124. Chen Y, Lei Y, Li J, Wang X, Li G. Macrophage-derived exosomal microRNAs promote metastasis in pancreatic ductal adenocarcinoma. Int Immunopharmacol. (2024) 129:111590. doi: 10.1016/j.intimp.2024.111590
125. Lv J, Liu C, Chen FK, Feng ZP, Jia L, Liu PJ, et al. M2−like tumour−associated macrophage−secreted IGF promotes thyroid cancer stemness and metastasis by activating the PI3K/AKT/mTOR pathway. Mol Med Rep. (2021) 24(2):604. doi: 10.3892/mmr.2021.12249
126. Yang C, Dou R, Wei C, Liu K, Shi D, Zhang C, et al. Tumor-derived exosomal microRNA-106b-5p activates EMT-cancer cell and M2-subtype TAM interaction to facilitate CRC metastasis. Mol Ther. (2021) 29:2088–107. doi: 10.1016/j.ymthe.2021.02.006
127. Xiong C, Zhu Y, Xue M, Jiang Y, Zhong Y, Jiang L, et al. Tumor-associated macrophages promote pancreatic ductal adenocarcinoma progression by inducing epithelial-to-mesenchymal transition. Aging (Albany NY). (2021) 13:3386–404. doi: 10.18632/aging.202264
128. Xie L, Qiu S, Lu C, Gu C, Wang J, Lv J, et al. Gastric cancer-derived LBP promotes liver metastasis by driving intrahepatic fibrotic pre-metastatic niche formation. J Exp Clin Cancer Res. (2023) 42:258. doi: 10.1186/s13046-023-02833-8
129. Wei C, Yang C, Wang S, Shi D, Zhang C, Lin X, et al. Crosstalk between cancer cells and tumor associated macrophages is required for mesenchymal circulating tumor cell-mediated colorectal cancer metastasis. Mol Cancer. (2019) 18:64. doi: 10.1186/s12943-019-0976-4
130. Geng Y, Fan J, Chen L, Zhang C, Qu C, Qian L, et al. Notch-dependent inflammatory feedback circuit between macrophages and cancer cells regulates pancreatic cancer metastasis. Cancer Res. (2021) 81:64–76. doi: 10.1158/0008-5472.CAN-20-0256
131. Li Q, Lin Y, Ni B, Geng H, Wang C, Zhao E, et al. Circadian system disorder induced by aberrantly activated EFNB2-EPHB2 axis leads to facilitated liver metastasis in gastric cancer. Cell Oncol (Dordr). (2024) 47:2113–34. doi: 10.1007/s13402-024-00991-1
132. Liguori M, Digifico E, Vacchini A, Avigni R, Colombo FS, Borroni EM, et al. The soluble glycoprotein NMB (GPNMB) produced by macrophages induces cancer stemness and metastasis via CD44 and IL-33. Cell Mol Immunol. (2021) 18:711–22. doi: 10.1038/s41423-020-0501-0
133. Xu H, Lai W, Zhang Y, Liu L, Luo X, Zeng Y, et al. Tumor-associated macrophage-derived IL-6 and IL-8 enhance invasive activity of LoVo cells induced by PRL-3 in a KCNN4 channel-dependent manner. BMC Cancer. (2014) 14:330. doi: 10.1186/1471-2407-14-330
134. Mantovani A, Allavena P, Marchesi F, Garlanda C. Macrophages as tools and targets in cancer therapy. Nat Rev Drug Discovery. (2022) 21:799–820. doi: 10.1038/s41573-022-00520-5
135. Bai H, Feng L, Schmid F. Macrophage-based cancer immunotherapy: Challenges and opportunities. Exp Cell Res. (2024) 442:114198. doi: 10.1016/j.yexcr.2024.114198
136. Zhang W, Wang M, Ji C, Liu X, Gu B, Dong T. Macrophage polarization in the tumor microenvironment: Emerging roles and therapeutic potentials. BioMed Pharmacother. (2024) 177:116930. doi: 10.1016/j.biopha.2024.116930
137. Wang K, He M, Fan X, Zhou J, Yang J, Wang L, et al. SEMA5A-PLXNB3 axis promotes PDAC liver metastasis outgrowth through enhancing the warburg effect. J Immunol Res. (2023) 2023:3274467. doi: 10.1155/2023/3274467
138. Deng Q, Geng Y, Zhao L, Li R, Zhang Z, Li K, et al. NLRP3 inflammasomes in macrophages drive colorectal cancer metastasis to the liver. Cancer Lett. (2019) 442:21–30. doi: 10.1016/j.canlet.2018.10.030
139. Sun D, Luo T, Dong P, Zhang N, Chen J, Zhang S, et al. M2-polarized tumor-associated macrophages promote epithelial-mesenchymal transition via activation of the AKT3/PRAS40 signaling pathway in intrahepatic cholangiocarcinoma. J Cell Biochem. (2020) 121:2828–38. doi: 10.1002/jcb.29514
140. Kawata M, Koinuma D, Ogami T, Umezawa K, Iwata C, Watabe T, et al. TGF-β-induced epithelial-mesenchymal transition of A549 lung adenocarcinoma cells is enhanced by pro-inflammatory cytokines derived from RAW 264. 7 macrophage cells J Biochem. (2012) 151:205–16. doi: 10.1093/jb/mvr136
141. Zhao X, Ren T, Li S, Wang X, Hou R, Guan Z, et al. A new perspective on the therapeutic potential of tumor metastasis: targeting the metabolic interactions between TAMs and tumor cells. Int J Biol Sci. (2024) 20:5109–26. doi: 10.7150/ijbs.99680
142. Wang J, Deng S, Cheng D, Gu J, Qin L, Mao F, et al. Engineered microparticles modulate arginine metabolism to repolarize tumor-associated macrophages for refractory colorectal cancer treatment. J Transl Med. (2024) 22:908. doi: 10.1186/s12967-024-05652-3
143. Cao M, Yan H, Han X, Weng L, Wei Q, Sun X, et al. Ginseng-derived nanoparticles alter macrophage polarization to inhibit melanoma growth. J Immunother Cancer. (2019) 7:326. doi: 10.1186/s40425-019-0817-4
144. Doleschel D, Hoff S, Koletnik S, Rix A, Zopf D, Kiessling F, et al. Regorafenib enhances anti-PD1 immunotherapy efficacy in murine colorectal cancers and their combination prevents tumor regrowth. J Exp Clin Cancer Res. (2021) 40:288. doi: 10.1186/s13046-021-02043-0
145. Tanei T, Leonard F, Liu X, Alexander JF, Saito Y, Ferrari M, et al. Redirecting transport of nanoparticle albumin-bound paclitaxel to macrophages enhances therapeutic efficacy against liver metastases. Cancer Res. (2016) 76:429–39. doi: 10.1158/0008-5472.CAN-15-1576
146. Luo Z, Jiang M, Cheng N, Zhao X, Liu H, Wang S, et al. Remodeling the hepatic immune microenvironment and demolishing T cell traps to enhance immunotherapy efficacy in liver metastasis. J Control Release. (2024) 373:890–904. doi: 10.1016/j.jconrel.2024.07.057
147. Huang S, Ding D, Lan T, He G, Ren J, Liang R, et al. Multifunctional nanodrug performs sonodynamic therapy and inhibits TGF-β to boost immune response against colorectal cancer and liver metastasis. Acta Biomater. (2023) 164:538–52. doi: 10.1016/j.actbio.2023.04.001
148. Xiao J, Wang S, Chen L, Ding X, Dang Y, Han M, et al. 25-Hydroxycholesterol regulates lysosome AMP kinase activation and metabolic reprogramming to educate immunosuppressive macrophages. Immunity. (2024) 57:1087–1104.e7. doi: 10.1016/j.immuni.2024.03.021
149. Zanganeh S, Hutter G, Spitler R, Lenkov O, Mahmoudi M, Shaw A, et al. Iron oxide nanoparticles inhibit tumour growth by inducing pro-inflammatory macrophage polarization in tumour tissues. Nat Nanotechnol. (2016) 11:986–94. doi: 10.1038/nnano.2016.168
150. Lin L, Du Y, Hao J, Wu R, Du L. UTMD inhibits pancreatic cancer growth and metastasis by inducing macrophage polarization and vessel normalization. BioMed Pharmacother. (2023) 160:114322. doi: 10.1016/j.biopha.2023.114322
151. Li R, Zhou R, Wang H, Li W, Pan M, Yao X, et al. Gut microbiota-stimulated cathepsin K secretion mediates TLR4-dependent M2 macrophage polarization and promotes tumor metastasis in colorectal cancer. Cell Death Differ. (2019) 26:2447–63. doi: 10.1038/s41418-019-0312-y
152. Grossman JG, Nywening TM, Belt BA, Panni RZ, Krasnick BA, DeNardo DG, et al. Recruitment of CCR2(+) tumor associated macrophage to sites of liver metastasis confers a poor prognosis in human colorectal cancer. Oncoimmunology. (2018) 7:e1470729. doi: 10.1080/2162402X.2018.1470729
153. Ren L, Yi J, Yang Y, Li W, Zheng X, Liu J, et al. Systematic pan-cancer analysis identifies APOC1 as an immunological biomarker which regulates macrophage polarization and promotes tumor metastasis. Pharmacol Res. (2022) 183:106376. doi: 10.1016/j.phrs.2022.106376
154. Liu X, Wang Z, Lv X, Tao Z, Lin L, Zhao S, et al. JDF promotes the apoptosis of M2 macrophages and reduces epithelial-mesenchymal transition and migration of liver cancer cells by inhibiting CSF-1/PI3K/AKT signaling pathway. Heliyon. (2024) 10:e34968. doi: 10.1016/j.heliyon.2024.e34968
155. Cheng Y, Zhong X, Nie X, Gu H, Wu X, Li R, et al. Glycyrrhetinic acid suppresses breast cancer metastasis by inhibiting M2-like macrophage polarization via activating JNK1/2 signaling. Phytomedicine. (2023) 114:154757. doi: 10.1016/j.phymed.2023.154757
156. Li J, Wang S, Wang N, Zheng Y, Yang B, Wang X, et al. Aiduqing formula inhibits breast cancer metastasis by suppressing TAM/CXCL1-induced Treg differentiation and infiltration. Cell Commun Signal. (2021) 19:89. doi: 10.1186/s12964-021-00775-2
157. Lv Q, Zhang Y, Gao W, Wang J, Hu Y, Yang H, et al. CSF1R inhibition reprograms tumor-associated macrophages to potentiate anti-PD-1 therapy efficacy against colorectal cancer. Pharmacol Res. (2024) 202:107126. doi: 10.1016/j.phrs.2024.107126
158. Vadevoo SMP, Gunassekaran GR, Yoo JD, Kwon TH, Hur K, Chae S, et al. Epigenetic therapy reprograms M2-type tumor-associated macrophages into an M1-like phenotype by upregulating miR-7083-5p. Front Immunol. (2022) 13:976196. doi: 10.3389/fimmu.2022.976196
159. Chen K, Li X, Dong S, Guo Y, Luo Z, Zhuang SM, et al. Modulating tumor-associated macrophages through CSF1R inhibition: a potential therapeutic strategy for HNSCC. J Transl Med. (2025) 23:27. doi: 10.1186/s12967-024-06036-3
160. Zhou Q, Liang J, Yang T, Liu J, Li B, Li Y, et al. Carfilzomib modulates tumor microenvironment to potentiate immune checkpoint therapy for cancer. EMBO Mol Med. (2022) 14:e14502. doi: 10.15252/emmm.202114502
161. Wang YN, Wang YY, Wang J, Bai WJ, Miao NJ, Wang J. Vinblastine resets tumor-associated macrophages toward M1 phenotype and promotes antitumor immune response. J Immunother Cancer. (2023) 11(8):e007253. doi: 10.1136/jitc-2023-007253
162. Li H, Huang N, Zhu W, Wu J, Yang X, Teng W, et al. Modulation the crosstalk between tumor-associated macrophages and non-small cell lung cancer to inhibit tumor migration and invasion by ginsenoside Rh2. BMC Cancer. (2018) 18:579. doi: 10.1186/s12885-018-4299-4
163. Moisan F, Francisco EB, Brozovic A, Duran GE, Wang YC, Chaturvedi S, et al. Enhancement of paclitaxel and carboplatin therapies by CCL2 blockade in ovarian cancers. Mol Oncol. (2014) 8:1231–9. doi: 10.1016/j.molonc.2014.03.016
164. Zhou J, Wang L, Peng C, Peng F. Co-targeting tumor angiogenesis and immunosuppressive tumor microenvironment: A perspective in ethnopharmacology. Front Pharmacol. (2022) 13:886198. doi: 10.3389/fphar.2022.886198
165. He J, Yin P, Xu K. Effect and molecular mechanisms of traditional chinese medicine on tumor targeting tumor-associated macrophages. Drug Des Devel Ther. (2020) 14:907–19. doi: 10.2147/DDDT.S223646
166. Deng XX, Jiao YN, Hao HF, Xue D, Bai CC, Han SY. Taraxacum mongolicum extract inhibited Malignant phenotype of triple-negative breast cancer cells in tumor-associated macrophages microenvironment through suppressing IL-10 / STAT3 / PD-L1 signaling pathways. J Ethnopharmacol. (2021) 274:113978. doi: 10.1016/j.jep.2021.113978
167. Laskar A, Eilertsen J, Li W, Yuan XM. SPION primes THP1 derived M2 macrophages towards M1-like macrophages. Biochem Biophys Res Commun. (2013) 441:737–42. doi: 10.1016/j.bbrc.2013.10.115
168. Che N, Li M, Liu X, Cui CA, Gong J, Xuan Y. Macelignan prevents colorectal cancer metastasis by inhibiting M2 macrophage polarization. Phytomedicine. (2024) 122:155144. doi: 10.1016/j.phymed.2023.155144
169. Li Y, Liao W, Huang W, Liu F, Ma L, Qian X. Mechanism of gambogic acid repressing invasion and metastasis of colorectal cancer by regulating macrophage polarization via tumor cell-derived extracellular vesicle-shuttled miR-21. Drug Dev Res. (2024) 85:e22141. doi: 10.1002/ddr.22141
170. Tang D, Wang H, Deng W, Wang J, Shen D, Wang L, et al. Mechanism of bufalin inhibition of colon cancer liver metastasis by regulating M2-type polarization of Kupffer cells induced by highly metastatic colon cancer cells. Apoptosis. (2024) 29:635–48. doi: 10.1007/s10495-023-01930-5
171. Zhou J, Li L, Pu Y, Li H, Wu X, Wang Z, et al. Astragaloside IV inhibits colorectal cancer metastasis by reducing extracellular vesicles release and suppressing M2-type TAMs activation. Heliyon. (2024) 10:e31450. doi: 10.1016/j.heliyon.2024.e31450
172. Chen C, Yao X, Xu Y, Zhang Q, Wang H, Zhao L, et al. Dahuang Zhechong Pill suppresses colorectal cancer liver metastasis via ameliorating exosomal CCL2 primed pre-metastatic niche. J Ethnopharmacol. (2019) 238:111878. doi: 10.1016/j.jep.2019.111878
173. Zhang Y, Liu Y, Wang J, Jiang Z, Zhang L, Cui Y, et al. Atractylenolide II inhibits tumor-associated macrophages (TAMs)-induced lung cancer cell metastasis. Immunopharmacol Immunotoxicol. (2022) 44:227–37. doi: 10.1080/08923973.2022.2037629
174. Chen Y, Yu Z, Tan X, Jiang H, Xu Z, Fang Y, et al. CAR-macrophage: A new immunotherapy candidate against solid tumors. BioMed Pharmacother. (2021) 139:111605. doi: 10.1016/j.biopha.2021.111605
175. Yu J, Green MD, Li S, Sun Y, Journey SN, Choi JE, et al. Liver metastasis restrains immunotherapy efficacy via macrophage-mediated T cell elimination. Nat Med. (2021) 27:152–64. doi: 10.1038/s41591-020-1131-x
176. Rodriguez-Garcia A, Lynn RC, Poussin M, Eiva MA, Shaw LC, O'Connor RS, et al. CAR-T cell-mediated depletion of immunosuppressive tumor-associated macrophages promotes endogenous antitumor immunity and augments adoptive immunotherapy. Nat Commun. (2021) 12:877. doi: 10.1038/s41467-021-20893-2
Keywords: macrophages, tumor-associated macrophages, liver metastases, tumor microenvironment, traditional Chinese medicine, therapy
Citation: Yuan Q, Jia L, Yang J and Li W (2025) The role of macrophages in liver metastasis: mechanisms and therapeutic prospects. Front. Immunol. 16:1542197. doi: 10.3389/fimmu.2025.1542197
Received: 09 December 2024; Accepted: 21 January 2025;
Published: 17 February 2025.
Edited by:
Zong Sheng Guo, University at Buffalo, United StatesReviewed by:
Dongya Zhang, Nanjing University of Chinese Medicine, ChinaCopyright © 2025 Yuan, Jia, Yang and Li. This is an open-access article distributed under the terms of the Creative Commons Attribution License (CC BY). The use, distribution or reproduction in other forums is permitted, provided the original author(s) and the copyright owner(s) are credited and that the original publication in this journal is cited, in accordance with accepted academic practice. No use, distribution or reproduction is permitted which does not comply with these terms.
*Correspondence: Jiahua Yang, eWFuZ2ppYWh1YTdAMTYzLmNvbQ==; Wei Li, bGl3ZWkxNTExOTcyQDE2My5jb20=
†These authors have contributed equally to this work and share last authorship
Disclaimer: All claims expressed in this article are solely those of the authors and do not necessarily represent those of their affiliated organizations, or those of the publisher, the editors and the reviewers. Any product that may be evaluated in this article or claim that may be made by its manufacturer is not guaranteed or endorsed by the publisher.
Research integrity at Frontiers
Learn more about the work of our research integrity team to safeguard the quality of each article we publish.