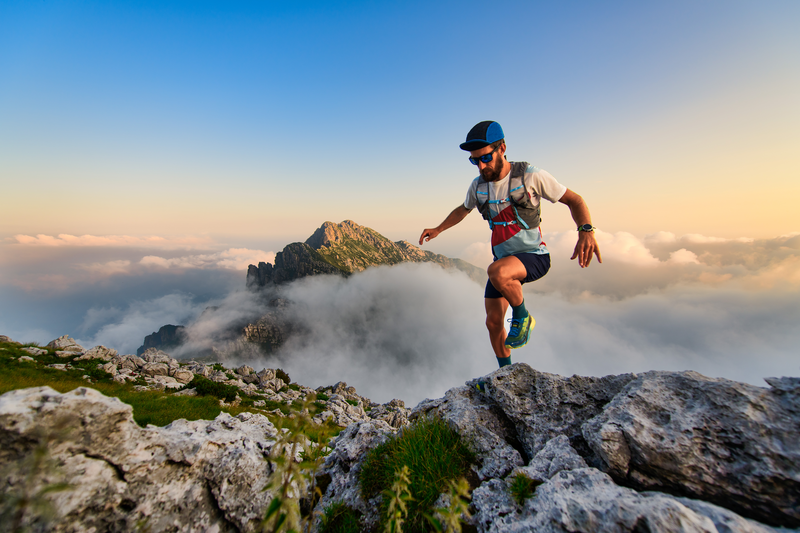
94% of researchers rate our articles as excellent or good
Learn more about the work of our research integrity team to safeguard the quality of each article we publish.
Find out more
ORIGINAL RESEARCH article
Front. Immunol.
Sec. Molecular Innate Immunity
Volume 16 - 2025 | doi: 10.3389/fimmu.2025.1542191
This article is part of the Research Topic Innovative Insights into Pattern Recognition and Signaling in Innate Immunity View all 4 articles
The final, formatted version of the article will be published soon.
You have multiple emails registered with Frontiers:
Please enter your email address:
If you already have an account, please login
You don't have a Frontiers account ? You can register here
Background: In addition to serving as the primary effector cells against infections, neutrophils have been implicated in the regulation of both innate and adaptive immunity. In this study, we aimed to investigate the role of neutrophils in the regulation of the immune system under physiological conditions.The in vivo effect of neutrophils on the immune system was examined using neutropenic mice. The interaction between neutrophils and γδ T cells was investigated using an in vitro co-culture system.Findings: Unexpectedly, we observed an accumulation of γδ T cells in the cervical lymph nodes of neutropenic mice. Transcriptomic analysis revealed that these γδ T cells exhibited unique expression profiles of cell surface molecules and genes involved in defense responses. Further characterization indicated that the accumulated γδ T cells were IL-17 producing CD44 + CD62L -CD27 -memory cells. Additionally, in vitro experiments demonstrated that neutrophils could inhibit the function of IL-17A producing γδ T cells by inducing cell death in a contact-dependent manner.This present study demonstrates that neutrophils negatively regulate IL-17 producing γδ T cells under physiological conditions. Given that IL-17A is a critical cytokine for the recruitment of neutrophils to peripheral tissues, our study suggests that the crosstalk between neutrophils and IL-17A producing γδ T cells is a crucial mechanism for maintaining immune homeostasis under physiological conditions.
Keywords: Neutrophils, γδ T cells, IL-17A, Cell Death, Physiological condition, Innate immunity
Received: 09 Dec 2024; Accepted: 07 Mar 2025.
Copyright: © 2025 Yu, Yue, Tchudjin Magatsin, Marwitz, Behrends, Goldmann, Opferman, Kasper and Petersen. This is an open-access article distributed under the terms of the Creative Commons Attribution License (CC BY). The use, distribution or reproduction in other forums is permitted, provided the original author(s) or licensor are credited and that the original publication in this journal is cited, in accordance with accepted academic practice. No use, distribution or reproduction is permitted which does not comply with these terms.
* Correspondence:
Xinhua Yu, Research Center Borstel (LG), Borstel, Germany
Disclaimer: All claims expressed in this article are solely those of the authors and do not necessarily represent those of their affiliated organizations, or those of the publisher, the editors and the reviewers. Any product that may be evaluated in this article or claim that may be made by its manufacturer is not guaranteed or endorsed by the publisher.
Research integrity at Frontiers
Learn more about the work of our research integrity team to safeguard the quality of each article we publish.