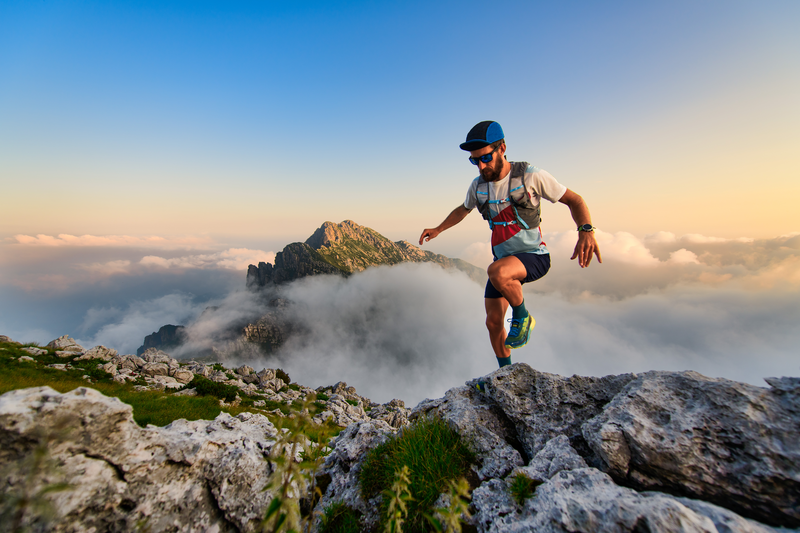
95% of researchers rate our articles as excellent or good
Learn more about the work of our research integrity team to safeguard the quality of each article we publish.
Find out more
MINI REVIEW article
Front. Immunol. , 13 February 2025
Sec. Viral Immunology
Volume 16 - 2025 | https://doi.org/10.3389/fimmu.2025.1541532
This article is part of the Research Topic HIV-1 Tat, An Enhancer of Virus Infectivity and Disease Promoter: Target for Preventive and Therapeutic Interventions View all 4 articles
Human immunodeficiency virus (HIV-1) transactivator Tat is a unique multi-functional viral protein secreted by infected cells. Although its primary function is to promote HIV-1 transcription, secreted Tat interacts with neighboring cells and induces numerous disease-associated pathological changes. Despite the substantial reduction of viral load and disease burden, Tat expression and secretion persist in people living with HIV who are undergoing treatment with highly effective combination antiretroviral therapy (cART). Tat interacts with both oral and genital epithelial cells and impairs their mucosal barrier functions, which facilitates the entry of other pathogenic viruses. Tat-mediated interactions with both human papillomavirus (HPV) -infected and HPV-negative neoplastic epithelial cells lead to epithelial-mesenchymal transition and increased invasiveness of malignant cells. Likewise, Tat-induced disruption of oral epithelial cell junctions leads to herpes simplex virus-1 (HSV-1) infection and spread via exposure of its receptor, nectin-1. HIV-1 Tat facilitates infection and spread of human cytomegalovirus (HCMV) by activating mitogen-activated protein kinases (MAPK) and promoting NF-κB signaling, both critical for the replication and production of progeny virions. HIV extracellular Tat also plays a critical role in human herpesvirus 8 (HHV8) -caused Kaposi sarcoma (KS) pathogenesis by synergizing with HHV-8 lytic proteins and promoting the proliferation, angiogenesis, and migration of endothelial cells. Collectively, these findings emphasize the critical impact of HIV-1 Tat on HIV/AIDS pathogenesis during the cART era and highlight the need for further research on the molecular mechanisms underlying Tat-mediated interactions with oral and genital mucosal epithelial cells.
The HIV-1 trans-activator of transcription protein, Tat, regulates the viral promoter and is responsible for transcriptional elongation, which is critical for HIV replication. Tat is also involved in regulating the expression of cellular genes and key signaling pathways, which are critical for the production and spread of HIV. Moreover, HIV-1 Tat is continuously secreted into the blood circulation of HIV-1-suppressed individuals and may reach various tissues and organs leading to their dysfunction.
HIV coinfection is common with several pathogenic human viruses, including high-risk HPV (1–4), HSV-1 and -2 (5–9), HCMV (10–12), Kaposi’s sarcoma-associated herpesvirus (KSHV) (13–15) and hepatitis C virus (HCV) (16–19).
Accumulating evidence supports that interactions of Tat with oral and genital mucosal epithelial cells disrupt their intercellular junctions and facilitate the spread of pathogenic human viruses, including HPV, HSV, and HCMV. Moreover, Tat may induce epithelial-mesenchymal transition (EMT), which is critical for the invasion of HPV-infected neoplastic epithelial cells. Tat plays a critical role in the development of Kaposi’s sarcoma (KS) by promoting proliferation, angiogenesis, and/or migration of target endothelial cells. The focus of this mini-review is to discuss the interaction of Tat with oral and genital mucosal epithelial and endothelial cells and to elucidate its role in the impairment of the mucosa barrier and induction of EMT, factors that are critical for the spread of viruses and acceleration of neoplastic processes, respectively.
HIV-1 Tat is a small (14-16 kDa) protein encoded by the viral genome that includes several important functional domains (20–23). The two forms of Tat generated by alternative splicing include one generated from a two-exon transcript that varies in length from 86 to 101 amino acids (aa) depending on the specific viral isolate. The second form of Tat is translated from a singly spliced one-exon transcript and is 72 aa long. The Tat amino-terminal domain (aa 1-48) contains a cysteine-rich motif that is responsible for activating HIV-1 transcription via its binding to a transactivation of response (TAR) element of the newly transcribed viral genomic RNA (24). The arginine-rich basic domain of Tat (aa 49-58) plays a critical role in its nuclear localization and binding to the TAR element (25). The basic domain of Tat also contains a protein transduction domain (PTD) which is a specific motif, YGRKKRRQRRR that mediates Tat internalization by bystander cells via interactions with heparan sulfate proteoglycans (HSPGs) (26–33). The Tat glutamine (Q)-rich domain (aa 60-72) also contributes to its interaction with the TAR element and mediates Tat-induced apoptosis (34, 35). HIV-1 Tat also contains a carboxy-terminal (aa 65-80) arginine, glycine, and aspartic acid (RGD) motif that facilitates its binding to various integrins, including αvβ1, αvβ3, and α5β1 (36–40).
One of the important features of HIV-1 Tat is its efficient secretion from virus-infected cells (30, 41–46) Tat crosses the plasma membrane via unconventional pathways that do not involve the endoplasmic reticulum and/or the Golgi/trans-Golgi network (43). Although the molecular mechanisms underlying Tat secretion remain unclear, secretion involves Tat binding to phosphatidylinositol-4,5-bisphosphate (PtdIns(4,5)P2) located in the inner leaflet of the plasma membrane, an interaction mediated by the basic domain of Tat (aa 48–57) and a conserved tryptophan (aa 11) (47). This interaction initiates oligomerization-mediated pore formation in the membrane, followed by Tat translocation and incorporation into exosomes, which mediate Tat’s secretion (22, 43, 44, 47–50). Secretion may also require Tat interactions with three short, non-consecutive cytoplasmic loops at the carboxy-terminus of the cellular Na+, K+-ATPase pump alpha subunit (51).
HIV-1-infected lymphocytes, macrophages, and dendritic cells/Langerhans cells (DC/LCs), as well as cell-free virus and viral RNA/DNA, have all been detected in oropharyngeal and genital mucosal epithelia and in salivary, and cervicovaginal secretions of people living with HIV (PLWH) who were undergoing combination antiretroviral therapy (cART) as well as those who were not (42, 52–66). HIV-1 Tat-positive CD4+ lymphocytes, macrophages, and DC/LCs were detected in the oropharyngeal and anogenital mucosal epithelia of PLWH, including those undergoing treatment with cART (42).
Westendorp and colleagues (67) were the first to demonstrate HIV-1 Tat secretion into the bloodstream; this finding has since been confirmed by others (68, 69). In pre-cART era, serum Tat concentrations in PLWH typically ranged from 0.1-40 ng/mL, sometimes reaching levels as high as 250-550 ng/mL (70–74). HIV-1 Tat was also detected in the serum of PLWH who achieved viral suppression with cART, where Tat concentration ranged from 0 to 14 ng/ml (46). Tat was detected in 25% of cART-treated individuals (46). The serum concentrations of Tat during early cART and late cART were 2-40 ng/ml and 0.2-9 ng/ml, respectively (68, 70).
HIV-1 Tat was also detected in the blood-cerebrospinal fluid of 37% of PLWH maintained on a long cART regimen (75, 76) at concentrations in the 0.2-6.5 ng/mL range. Functional Tat was detected in exosomes from 34.4% of these individuals (75). Secretion of Tat was detected in the saliva of ART-treated and -untreated individuals (42).
Tat was detected in brain and cerebrospinal fluid in the absence of virus replication (73). Furthermore, in experiments performed in vitro, Tat was expressed and secreted from HIV-infected cells treated with protease inhibitors that inhibited viral replication, indicating that Tat production and secretion requires no cleavage events catalyzed by the viral protease (73, 77). Thus, Tat may continue to be expressed and released in the presence of a protease inhibitor, which is among the critical components of cART (73, 75, 77). Tat/TAR-containing exosomes can be endocytosed by HIV-1-infected cells that contain an inactive (latent) provirus (75). Internalized Tat/TAR may then reactivate the latent provirus via induction of host signaling pathways such as those involving NF-κB and transactivation of the HIV-1 long terminal repeat (LTR), which may explain Tat expression detected in PLWH maintained on cART (75). Tat expression in PLWH treated with cART may also result from ongoing abortive and/or spontaneous viral transcription that promotes expression of viral early genes, including Tat (78, 79). Collectively, these findings suggest that HIV-1 Tat is produced continuously by infected cells and released into circulation despite ongoing cART and that circulating Tat may be delivered directly to tissues and organs and internalized into epithelial and other host cells.
Furthermore, cART drugs have only a limited capacity to penetrate solid tissues, including lymph nodes and epithelial tissues (80–83), Thus, HIV-1 replication may persist in these drug-inaccessible environments, leading to the expression and release of Tat and its spread within the surrounding tissues and organs. Cervicovaginal secretion of HIV-1 in ART-treated women with low to undetectable plasma viral loads suggested that there may be a cohort of local intramucosal HIV-1-infected cells that remained capable of producing virus due to lack of drug accessibility (64, 84–88). This hypothesis might also explain the persistent detection of HIV-1 in the semen of ART-treated infected men with undetectable viral loads in circulation (89). Collectively, these findings suggest that ART-inaccessible tissues may serve as a reservoir for HIV-1 and that these cells may release Tat continuously into the surrounding environment (45, 80–83).
The oropharyngeal, ectocervical, vaginal, and foreskin epithelia consist of a multilayered, stratified squamous epithelium; the endocervical and intestinal mucosa is also covered with a monostratified simple epithelium. These epithelial cells form numerous intercellular junctions (42, 90–100). Among these, tight and adherens junctions are critical for maintaining the morphological and physiological features of mucosal epithelia (Figure 1). Tight junctions within the mucosal epithelium form an intramembranous “fence” that links neighboring cells and maintains a physical barrier that protects against external environment (101). Tight junctions are established by the transmembrane proteins known as occludin and claudins in association with the cytoplasmic proteins zonula occludens-1 (ZO-1), ZO-2, and ZO-3 (Figure 1B, left panel), which linked to the actin cytoskeleton (102). Junctional adhesion molecule 1 (JAM-1) is specifically localized at the tight junctions of epithelial cells and is involved in the regulation of junctional integrity and paracellular permeability (103). Intercellular adherens junctions are formed by homotypic interactions of the transmembrane protein, E-cadherin, which is connected to intracellular proteins p120 and α- and β- catenins and the actin cytoskeleton (104).
Figure 1. Model of HIV-1 Tat-induced disruption of tight junctions of mucosal epithelium. (A) Oropharyngeal and genital mucosal epithelial cells have a polarized organization with well-developed tight and adherens junctions and distinct apical and basolateral membranes. (B, left panel) Tight junctions are formed between epithelial cells via the lateral interaction of the transmembrane proteins, occludin and claudins, which are associated with cytoplasmic proteins ZO-1, ZO-2, and ZO-3, which provide links to the cortical actin cytoskeleton. Junctional adhesion molecule 1 (JAM-1) is also localized at the tight junctions of epithelial cells. The cell adhesion protein nectin-1 is sequestered within the adhesion junctions. (B, right panel) Disruption of the tight and adherens junctions of mucosal epithelium. (C, left panel) Buccal biopsy samples from HIV-1-infected ART-treated and HIV-1-negative individuals were immunostained for occludin (red). EP, epithelium; BM, basement membrane; LP, lamina propria. The continuous ring-shaped pattern of occludin localization on the membrane of buccal epithelial cells from HIV-1-uninfected individuals indicates the intact tight junctions of buccal mucosal epithelium. (C, right panel) By contrast, the absence or discontinuous/fragmented ring-shaped pattern of occludin immunostaining in buccal epithelial cells from HIV-1-infected individuals shows disrupted tight junctions of buccal mucosal epithelium. The models generated for this and all other figures to follow were created using Adobe® Illustrator.
Tight junctions in oral, intestinal, and genital mucosal epithelia are disrupted in PLWH, leading to barrier impairment (Figure 1B, right panel, and C) (42, 105–107). Interactions of HIV-1 Tat with oral epithelial cells lead to activation of MAPK and substantial disruption of complexes containing ZO-1, occludin, and claudin-1 (42). HIV-1 glycoprotein (gp)120 also disrupts epithelial junctions; combinations of Tat and gp120 amplify HIV-1-induced impairment of mucosal epithelial barrier functions (42, 108, 109). In oral epithelial cells, HIV-1 Tat activates MAPK and NF-κB signaling, leading to the upregulation of matrix metalloproteinase (MMP)-9 expression and activity (109), and the degradation of epithelial junctions.
Human papillomavirus (HPV) is an oncogenic virus (110, 111). The incidence of HPV-associated oropharyngeal, cervical, and anal cancer is approximately 6-, 22-, and 80-times higher, respectively, in PLWH compared to those who remain uninfected (112–119). Although a highly effective anti-HPV vaccine is available, its value is limited in PLWH because most of these individuals have already been infected with high-risk HPVs, including HPV-16 and HPV-18.
Although HIV-1 may increase the incidence of HPV-associated cancers by attenuating immune responses, accumulating evidence has indicated that the direct interaction of HIV proteins with HPV proteins and HPV-infected epithelial cells may also play a critical role in the progression of HPV-associated malignancy (119–121).
Tat (and/or gp120) proteins disrupt tight junctions of oral and genital mucosal epithelium, thereby reducing the barrier function of mucosal epithelium and facilitating paracellular penetration of HPV-16 (42). This facilitated HPV paracellular spread through strata spinosum and granulosum layers, leading to the infection of basal/parabasal cells, the site of initiation of the HPV life cycle (122).
Tat-induced activation of MAPK and transforming growth factor (TGF)-β signaling in normal and neoplastic oral and genital epithelia led to epithelial-mesenchymal transition (EMT) (120, 121), which is a physiologic process that provides critical contributions to embryonic development (123). However, EMT also promotes epithelial neoplasia, including the invasion and spread of cancer cells (124–132). EMT results in the loss of polarity of epithelial cells, which is followed by loss of adherens (E-cadherin) and tight junctions as well as critical cell-adhesive properties (Figure 2). While in the intermediate stages of EMT, cells may express both mesenchymal (i.e., vimentin) and epithelial (i.e., E-cadherin) markers. Cells with this hybrid phenotype may be highly invasive and contribute to the formation of cancer metastases (Figure 2B) (133–138). Cells displaying the hybrid phenotype will then develop a spindle cell-type shape and express additional mesenchymal markers, including vimentin, fibronectin and N-cadherin and lost E-cadherin; these cells are also highly invasive (Figure 2C) (133–138).
Figure 2. HIV-1 Tat-induced EMT in neoplastic oral and genital epithelial cells. (A) HIV-infected CD4+ lymphocytes, macrophages, and DC/LCs can migrate into the epithelium and secrete HIV-1 Tat in the HPV-infected or HPV-negative neoplastic mucosal environment (42). This will lead to the activation of MAPK and TGF-β signaling pathways followed by induction of the EMT phenotype in which epithelial cells lose intact tight and adherens junctions and (B) coexpress E-cadherin and vimentin. Cells displaying this hybrid EMT phenotype also express stem cell markers and become CSCs with the potential to migrate through the basement membrane and initiate invasive cancer. (C) Further progression of EMT may lead to the loss of E-cadherin expression and a parallel upregulation of N-cadherin and vimentin as cells acquire a mesenchymal spindle-like morphology. These cells may also be highly invasive and capable of migration.
Neoplastic cells with an EMT phenotype may also express stem cell markers and become cancer stem cells (CSCs) with undifferentiated stem cell properties with the capacity for self-renewal and uncontrolled proliferation (134–143). CSCs detected in solid oropharyngeal and genital tumors express numerous stem cell markers, including CD44, CD24, CD133, aldehyde dehydrogenase 1A, CD10, CD147, CD117, CD24, podoplanin, laminin subunit beta 3, and laminin subunit gamma 2 (134–140, 142–172). Recent results suggest that the expression of one or more of these CSC markers may significantly increase resistance to drugs and apoptosis and promote survival, growth, and expansion of invasive cancers (129–131).
Activation of the TGF-β signaling pathway is critical for the induction of EMT in neoplastic cells (173, 174). The binding of the mature form of TGF-β to its receptor TGFBR2 initiates activation of Smad family transcription factors and other specific transcriptional regulators, including Slug, Twist1, and Snail. These events lead to the upregulation of N-cadherin, fibronectin, and vimentin and the downregulation of E-cadherin (175, 176) following induction of EMT.
HIV-1 Tat binding to α5β1 and αvβ3 integrins via its RGD domain increases Ras-induced ERK phosphorylation and activation of MAPK signaling (177), which is critical for upregulation of the TGF-β signaling pathway and induction of EMT (120, 121). HSPG activates TGF-β signaling by depositing latent TGF-β -binding protein-1 in the extracellular matrix, thereby enhancing the TGF-β binding to its primary receptors (178), which is also critical for EMT induction. Tat binding to HSPG may modulate the HSPG-enhanced interaction of TGF-β with its receptors (179). Although TGF-β signaling is critical for EMT induction, additional signaling pathways also contribute to this process depending on the cell type and cellular microenvironment. For example, introduction of HIV-1 Tat and gp120 to cells of the HPV-16-infected cervical cancer SiHa cell line led to the induction of EMT and cell migration and invasion secondary to the activation of the Wnt/β-catenin pathway (132), indicating that the Tat/gp120-activated Wnt/β-catenin signaling pathway also contributes to EMT progression (132). Transcriptome analysis revealed that Tat/gp120-treated cells responded by upregulating keratin 17 and downregulating glycogen synthase kinase 3β, which promote the increase in vimentin and decrease in E-cadherin expression, respectively (i.e., EMT induction) (132).
Prolonged interactions (five days) of HIV-1 Tat and/or gp120 with HPV-16-immortalized oral (UM-SCC-47), cervical (CaSki), and anal (AKC-2) epithelial cells induce EMT and increased invasiveness of neoplastic cells (120). Furthermore, cells with Tat and/or gp120-induced EMT cells that co-expressed vimentin and E-cadherin and presented an intermediate/hybrid stage of EMT became poorly-adherent and easily detached (120). These cells also expressed CSC markers CD44 and CD133 and were more invasive, suggesting a specific role for hybrid EMT cells in cancer-associated metastasis.
HIV-1 Tat exposure also led to reductions in p53 expression, transcription of cell cycle inhibitors, and increased proliferation of HPV-18 infected cervical cancer HeLa cells (180). HIV-1 Tat also transactivated the HPV long control region and increased HPV18 E7 expression in HeLa cells (181).
Consistent with in vitro data, analysis of tissue samples from patients with cervical intraepithelial neoplasia (CIN) and cervical cancer who were infected with high-risk HPVs revealed significantly lower levels of E-cadherin and cytokeratin and higher levels of N-cadherin and vimentin expression in HIV-infected patients than those who were HIV-negative (132).
HIV-1 Tat inhibited epithelial differentiation and apoptosis of HPV-negative colorectal cancer cells (LIM1215 and LIM2537), increasing their invasiveness and tumorigenicity (182). HIV-1 Tat protein also induced EMT and increased invasiveness of HPV-negative lung epithelial (A549, H358) (183) and oral carcinoma (HSC-3) (120) cancer cell lines. These results suggest that HIV-1 Tat may promote the development of malignancy in both HPV-negative and HPV-infected neoplastic epithelial cells.
HIV-1 Tat-induced EMT may also promote epithelial neoplasia associated with other oncogenic viruses. For example, hepatitis C virus (HCV) coinfection with HIV-1 is detected quite frequently; approximately 30% of HIV-infected individuals may be coinfected with HCV (184–187). Primary liver cancer is more aggressive in patients with HIV-HCV coinfection than in those with HCV infection alone (188). HIV-1 Tat-induced EMT may develop in liver epithelial cells, thus accelerating HCV malignancy in coinfected individuals.
TGF-β expression remains persistently elevated in the blood of PLWH undergoing cART as well as those who are not (189–198). HIV-1-associated elevations of TGF-β contribute to the activation of EMT-associated profibrotic processes in various epithelial organs, including kidney, liver, and lung (199–208) (69, 209–214). It is possible that the elevated levels of TGF-β expression may induce EMT and ultimately increase invasiveness in precancer or cancer cells found in PLWH.
HIV-1 Tat induces TGF-β expression in epithelial cells, macrophages, and other cells (120, 121, 196, 215–218), which may contribute to its persistent elevation observed in PLWH. Persistent elevation of circulating TGF-β in PLWH undergoing cART may result from the continuous expression and secretion of HIV-1 Tat. Circulating TGF-β may reach the neoplastic tissues where it induces EMT and increased invasiveness. This may be one of the mechanisms underlying the persistence of HPV malignancy in PLWH treated with cART.
Taken together, the results of these studies suggest that direct interactions of extracellular HIV-1 Tat with neoplastic mucosal and other epithelial cells may lead to induction of EMT and increased invasiveness, leading to the acceleration of HPV-associated and HPV-negative malignancies (Figure 2). HIV-1 Tat-induced EMT may have a more rapid effect on the acceleration of HPV-infected neoplasia, given that oncogenic HPVs also induce EMT. For example, several groups reported that HPV-16 E6 and E7 oncoproteins induced the increased expression of MMP-2 (219–222) which is an enzyme that cleaves E-cadherin (223–225) and leads to the loss of adherens junctions and the induction of EMT (226). Both HIV- and HPV-induced EMT may have synergistic effects in the progression of HPV-associated neoplasia in PLWH.
HSV-1 and 2 may reactivate and replicate in the oral and genital epithelium of PLWH and can lead to ulcers and necrotic lesions (227–229). HSV-1 reactivation may occur despite ongoing cART (227, 230, 231). Although the increased risk of developing an HSV infection may be mediated in part by HIV-1-induced immune dysfunction, it may also be associated with direct and/or indirect molecular interactions between the two viruses.
As discussed in Section 4., interactions of HIV-1 Tat with oral epithelial cells can lead to the disruption of both adherens and tight junctions via the activation of the MAPK and NF-κB signaling pathways and the upregulation of MMP-9 (108, 109). HIV-1 Tat-mediated disruption of oral epithelial junctions facilitates the paracellular spread of HSV-1 (108).
The HSV-1 envelope gD binds to the cell adhesion protein nectin-1 (232), which is sequestered within intercellular junctions (Figure 1B); this localization plays a critical role in limiting HSV access to epithelial cells (233). HIV-1 Tat-mediated disruption of adherens junctions liberates nectin-1, thereby promoting HSV-1 gD binding to nectin-1. Thus, the extent of HSV-1 infection is substantially increased in disrupted compared to intact epithelial cells (108). Disrupted adherens junctions expose sequestered nectin-1 and thus accelerate the cell-to-cell spread of HSV-1. The introduction of anti-nectin-1 and anti-HSV-1 gD antibodies substantially blocked HSV-1 infection and cell-to-cell spread of the virus, indicating a critical role played by HIV-1 Tat-induced disruption of epithelial junctions and the liberation of nectin-1 in HSV infection and spread (108).
HSV-1 is latent in sensory neurons in healthy individuals with normal immune surveillance mechanisms (234). However, HIV-1 infection/acquired immunodeficiency syndrome (AIDS)-induced immune dysfunction leads to HSV-1 reactivation in neurons and the development of mucosal disorders (230). As noted above, HIV-1 Tat-mediated liberation of nectin-1 from cells with disrupted adherens junctions also promotes the spread of HSV-1 from neurons to epithelial cells. Thus, HIV-1 Tat-mediated disruption of intercellular junctions potentiates HSV-1 infection by facilitating paracellular and cell-to-cell spread of the virus within the mucosal epithelium and between epithelial cells and neurons. This mechanism may provide a mechanism to explain the rapid development of HSV-associated oral lesions in HIV-1-infected individuals.
HCMV can promote the development of oral mucosal lesions, retinitis, hepatitis, esophagitis, pneumonia, encephalopathy, and/or gastrointestinal inflammation (235–239). Nearly all HIV-1-infected individuals are co-infected with HCMV (10, 240). HCMV activation has also been reported in HIV-1-associated/AIDS disease (241).
HIV-1 Tat-induced disruption of tonsil epithelial junctions impaired their barrier function and promoted the paracellular spread of HCMV (242). Moreover, HIV-1 Tat-induced activation of NF-κB and MAPK signaling in these cells increases the extent of HCMV infection. NF-κB activation is critical for transactivation of the major immediate early HCMV promoter and the initiation of viral replication (243–245). MAPK activation also plays an important role in HCMV replication and the production of viral progeny (246).
Thus, HIV-1 Tat-induced disruption of the integrity of the oral mucosal epithelia may promote HCMV paracellular spread, which is critical for the initial entry of the virus in HCMV-negative individuals and the development of a systemic viral infection. Tat-induced HCMV paracellular spread is also important for transmitting the virus within the mucosal epithelium and the infection of intraepithelial and submucosal monocytes and macrophages, which may play an important role in promoting HCMV persistence (247–251). HIV-1 Tat-mediated induction of HCMV replication in epithelial cells through activation of NF-κB and MAPK signaling may contribute to viral amplification and dissemination, which lead to the development of mucosal lesions and other diseases.
HIV-1 Tat-induced disruption of epithelial junctions may promote infection and spread of other viruses that use tight junction proteins as receptors. For example, Hepatitis C Virus (HCV) uses occludin and claudins 1, 6, and 9 for entry (252–255). Reovirus entry requires JAM-1, occludin, and ZO-1 (256, 257); occludin is critical for coxsackievirus infection (258). HIV-1 Tat-induced disruption of epithelial tight junctions may expose these sequestered tight junction proteins to their cognate viruses, leading to their more rapid entry and spread.
Kaposi’s sarcoma-associated herpesvirus (KSHV), known as human herpesvirus 8 (HHV8), is an etiological agent of Kaposi sarcoma (KS) (259), which is an AIDS-defining tumor with uncontrolled growth of endothelial cells with abnormal angiogenesis (260). KS may develop on the skin and/or oral/intestinal mucosa and can spread to lymph nodes and lungs (260).
HIV extracellular Tat plays a critical role in KS pathogenesis by synergizing with KSHV lytic proteins and thus promoting the proliferation of endothelial cells, their angiogenesis, and migration (30, 72, 261–271). HIV Tat interaction with endothelial cells leads to increased expression of the KSHV G protein-coupled receptor (vGPCR) and its signaling, which promotes viral infection and tumorigenicity (272–275). HIV Tat also promotes KSHV interleukin -6 (vIL-6)- and K1-induced angiogenesis (271, 276). HIV Tat-promoted increase of the oncogenic activity of KSHV proteins mostly occurs by Tat-associated regulation/modulation of the phosphatidylinositol 3-kinase/protein kinase B (PI3K/Akt) signaling pathway (271, 274, 277). Tat may also be involved in the expression of miRNAs, which increase angiogenesis, and thus accelerate KSHV-associated tumorigenesis (276).
In the pre-cART era, KS was approximately 20 – 50 fold more common in persons with HIV/AIDS than in the general population (278, 279). In PLWH, cART treatment markedly reduced the incidence of KS (280, 281). However, recent findings suggest that in PLWH the risk of KS is increasing despite the normalization of CD4 count and lack of HIV viremia (282–285). Increasing KS in PLWH with cART has been suggested as a wake-up call for research on HHV-8 (286).
The secretion of Tat in PLWH undergoing cART may contribute to the development of KS via Tat-induced proliferation, angiogenesis, and/or migration of HHV-8-infected endothelial cells. Moreover, results presented in other studies revealed that extracellular Tat promotes decreased expression of the tight junction proteins, claudin-1, claudin-5, and ZO-1 and/or ZO-2 in endothelial cells, leading to disruption of their intercellular junctions (189, 287–298). These changes may damage endothelial cells and increase the permeability of blood vessels, leading to endothelial barrier dysfunction, inflammation, and related disorders in the surrounding tissues (299, 300). This has been well characterized in studies of HIV-1 Tat-induced disruption of brain microvascular endothelial cells, which leads to impairment of the blood-brain barrier (BBB) and ultimately HIV-associated neurocognitive disorders (300–303). Tat-induced disruption of BBB may also result from Tat-mediated apoptosis of brain endothelial cells (304), as well as Tat-facilitated elevations in MMPs (297), endoplasmic reticulum (ER) stress, and mitochondrial dysfunction (304, 305).
KSHV-infected microvascular endothelial cells lose the capacity to express vascular endothelial cell markers (e.g., VE-cadherin, CD31, CD34, CD36, vascular endothelial growth factor receptor-3, and vascular endothelial growth factor C) and acquire expression of mesenchymal markers (fibroblast-specific protein-1, alpha 2 smooth muscle actin, type I/III collagen, vimentin, and N-cadherin), displaying endothelial-mesenchymal transition (EndMT) and invasive and migratory properties along with increased survival (306–313). KSHV-activated Notch-induced transcription factors Slug and ZEB1 were identified as critical for KSHV-induced EndMT (306, 307). TGFβ signaling pathway is also required for EndMT induction (314). However, KSHV did not activate the TGFβ signaling pathway in endothelial cells (306, 307). In persons diagnosed with HIV-KSHV coinfection, HIV-1 Tat-induced activation of the TGF-β signaling pathway might synergize with KHSV-induced Notch signaling, thereby accelerating migration and invasion of neoplastic endothelial cells.
HIV-1 Tat is expressed and secreted from HIV-infected cells of both ART-treated and untreated individuals. HIV-infected CD4+ lymphocytes, macrophages, and DC/LCs can migrate into the oral and cervical epithelium and secrete HIV-1 Tat protein in both neoplastic and non-neoplastic epithelial environments. HIV-1 Tat – epithelial cell interactions lead to activation of MAPK/NF-kB signaling followed by the disruption of tight and adherens junctions of epithelial cells and impaired barrier and immune/innate immune cell functions within the mucosal epithelium. HIV-1 Tat-activated MAPK and TGFβ-signaling in neoplastic epithelial cells induce EMT accompanied by the expression of the CSC markers CD133 and CD44, which amplifies the invasiveness of both HPV-infected and HPV-negative premalignant and malignant cells. HIV-1 Tat-induced disruption of epithelial junctions facilitates infection and spread of HSV-1 by liberating its otherwise sequestered gD receptor, nectin-1. HIV-1 Tat-activated NF-kB and MAPK signaling in epithelial cells promote the replication of HCMV and increase the production of viral progeny, respectively, leading to the spread of the virus within the oral mucosal epithelium. HIV Tat also plays a critical role in HHV-8-caused KS malignancy by promoting the proliferation, angiogenesis, and migration of neoplastic endothelial cells. Thus, HIV-1 Tat clearly contributes to the pathogenesis of HIV/AIDS despite the availability of cART. These findings emphasize the need for further investigation focused on the molecular mechanisms of Tat action in non-HIV-infected cells, including normal and neoplastic epithelial and endothelial cells. The development of a therapeutic vaccine targeting HIV-1 Tat may address some of these critical complications, including Tat-mediated disruption of epithelial junctions and induction of EMT.
ST: Writing – original draft, Writing – review & editing.
The author(s) declare that financial support was received for the research, authorship, and/or publication of this article. This project was supported by the National Institute of Dental and Craniofacial Research (NIDCR) R01DE028129 and National Cancer Institute (NCI) R01CA232887 grants (to ST).
The author declares that the research was conducted in the absence of any commercial or financial relationships that could be construed as a potential conflict of interest.
The author(s) declare that no Generative AI was used in the creation of this manuscript.
All claims expressed in this article are solely those of the authors and do not necessarily represent those of their affiliated organizations, or those of the publisher, the editors and the reviewers. Any product that may be evaluated in this article, or claim that may be made by its manufacturer, is not guaranteed or endorsed by the publisher.
1. Ferenczy A, Coutlee F, Franco E, Hankins C. Human papillomavirus and HIV coinfection and the risk of neoplasias of the lower genital tract: a review of recent developments. CMAJ. (2003) 169:431–4.
2. Fontaine J, Hankins C, Mayrand MH, Lefevre J, Money D, Gagnon S, et al. High levels of HPV-16 DNA are associated with high-grade cervical lesions in women at risk or infected with HIV. AIDS. (2005) 19:785–94. doi: 10.1097/01.aids.0000168972.65304.6b
3. Fontaine J, Hankins C, Money D, Rachlis A, Pourreaux K, Ferenczy A, et al. Human papillomavirus type 16 (HPV-16) viral load and persistence of HPV-16 infection in women infected or at risk for HIV. J Clin Virol. (2008) 43:307–12. doi: 10.1016/j.jcv.2008.07.013
4. Perez-Gonzalez A, Cachay E, Ocampo A, Poveda E. Update on the epidemiological features and clinical implications of human papillomavirus infection (HPV) and human immunodeficiency virus (HIV) coinfection. Microorganisms. (2022) 10. doi: 10.3390/microorganisms10051047
5. Tan DH, Kaul R, Walsmley S. Left out but not forgotten: Should closer attention be paid to coinfection with herpes simplex virus type 1 and HIV? Can J Infect Dis Med Microbiol. (2009) 20:e1–7.
6. Bohl DD, Katz KA, Bernstein K, Wong E, Raymond HF, Klausner JD, et al. Prevalence and correlates of herpes simplex virus type-2 infection among men who have sex with men, san francisco, 2008. Sex Transm Dis. (2011) 38:617–21. doi: 10.1097/OLQ.0b013e31820a8c10
7. Turner KR, McFarland W, Kellogg TA, Wong E, Page-Shafer K, Louie B, et al. Incidence and prevalence of herpes simplex virus type 2 infection in persons seeking repeat HIV counseling and testing. Sex Transm Dis. (2003) 30:331–4. doi: 10.1097/00007435-200304000-00011
8. Lama JR, Lucchetti A, Suarez L, Laguna-Torres VA, Guanira JV, Pun M, et al. Association of herpes simplex virus type 2 infection and syphilis with human immunodeficiency virus infection among men who have sex with men in Peru. J Infect Dis. (2006) 194:1459–66. doi: 10.1086/jid.2006.194.issue-10
9. Suligoi B, Dorrucci M, Volpi A, Andreoni M, Zerboni R, Rezza G, et al. Prevalence and determinants of herpes simplex virus type 2 infection in a cohort of HIV-positive individuals in Italy. Sex Transm Dis. (2002) 29:665–7. doi: 10.1097/00007435-200211000-00008
10. Gianella S, Massanella M, Wertheim JO, Smith DM. The sordid affair between human herpesvirus and HIV. J Infect Dis. (2015) 212:845–52. doi: 10.1093/infdis/jiv148
11. Gianella S, Letendre S. Cytomegalovirus and HIV: A dangerous pas de deux. J Infect Dis. (2016) 214 Suppl 2:S67–74. doi: 10.1093/infdis/jiw217
12. Adland E, Klenerman P, Goulder P, Matthews PC. Ongoing burden of disease and mortality from HIV/CMV coinfection in Africa in the antiretroviral therapy era. Front Microbiol. (2015) 6:1016. doi: 10.3389/fmicb.2015.01016
13. Labo N, Miley W, Benson CA, Campbell TB, Whitby D. Epidemiology of Kaposi’s sarcoma-associated herpesvirus in HIV-1-infected US persons in the era of combination antiretroviral therapy. AIDS. (2015) 29:1217–25. doi: 10.1097/QAD.0000000000000682
14. Woodberry T, Suscovich TJ, Henry LM, Martin JN, Dollard S, O’Connor PG, et al. Impact of Kaposi sarcoma-associated herpesvirus (KSHV) burden and HIV coinfection on the detection of T cell responses to KSHV ORF73 and ORF65 proteins. J Infect Dis. (2005) 192:622–9. doi: 10.1086/jid.2005.192.issue-4
15. Pyakurel P, Pak F, Mwakigonja AR, Kaaya E, Biberfeld P. KSHV/HHV-8 and HIV infection in Kaposi’s sarcoma development. Infect Agent Cancer. (2007) 2:4. doi: 10.1186/1750-9378-2-4
16. Bosh KA, Coyle JR, Hansen V, Kim EM, Speers S, Comer M, et al. HIV and viral hepatitis coinfection analysis using surveillance data from 15 US states and two cities. Epidemiol Infect. (2018) 146:920–30. doi: 10.1017/S0950268818000766
17. Bosh KA, Coyle JR, Muriithi NW, Ramaswamy C, Zhou W, Brantley AD, et al. Linking HIV and viral hepatitis surveillance data: evaluating a standard, deterministic matching algorithm using data from 6 US health jurisdictions. Am J Epidemiol. (2018) 187:2415–22. doi: 10.1093/aje/kwy161
18. Sherman KE, Rouster SD, Chung RT, Rajicic N. Hepatitis C Virus prevalence among patients infected with Human Immunodeficiency Virus: a cross-sectional analysis of the US adult AIDS Clinical Trials Group. Clin Infect Dis. (2002) 34:831–7. doi: 10.1086/339042
19. Platt L, Easterbrook P, Gower E, McDonald B, Sabin K, McGowan C, et al. Prevalence and burden of HCV co-infection in people living with HIV: a global systematic review and meta-analysis. Lancet Infect Dis. (2016) 16:797–808. doi: 10.1016/S1473-3099(15)00485-5
20. Dayton AI, Sodroski JG, Rosen CA, Goh WC, Haseltine WA. The trans-activator gene of the human T cell lymphotropic virus type III is required for replication. Cell. (1986) 44:941–7. doi: 10.1016/0092-8674(86)90017-6
21. Bohan CA, Kashanchi F, Ensoli B, Buonaguro L, Boris-Lawrie KA, Brady JN. Analysis of Tat transactivation of human immunodeficiency virus transcription in vitro. Gene Expr. (1992) 2:391–407.
22. Debaisieux S, Rayne F, Yezid H, Beaumelle B. The ins and outs of HIV-1 Tat. Traffic. (2012) 13:355–63. doi: 10.1111/j.1600-0854.2011.01286.x
23. Clark E, Nava B, Caputi M. Tat is a multifunctional viral protein that modulates cellular gene expression and functions. Oncotarget. (2017) 8:27569–81. doi: 10.18632/oncotarget.15174
24. Chiozzini C, Toschi E. HIV-1 TAT and IMMUNE DYSREGULATION in AIDS PATHOGENESIS: a THERAPEUTIC TARGET. Curr Drug Targets. (2016) 17:33–45. doi: 10.2174/1389450116666150825110658
25. Gotora PT, van der Sluis R, Williams ME. HIV-1 Tat amino acid residues that influence Tat-TAR binding affinity: a scoping review. BMC Infect Dis. (2023) 23:164. doi: 10.1186/s12879-023-08123-0
26. Frankel AD, Pabo CO. Cellular uptake of the tat protein from human immunodeficiency virus. Cell. (1988) 55:1189–93. doi: 10.1016/0092-8674(88)90263-2
27. Cashman SM, Sadowski SL, Morris DJ, Frederick J, Kumar-Singh R. Intercellular trafficking of adenovirus-delivered HSV VP22 from the retinal pigment epithelium to the photoreceptors–implications for gene therapy. Mol Ther. (2002) 6:813–23. doi: 10.1006/mthe.2002.0806
28. Nagahara H, Vocero-Akbani AM, Snyder EL, Ho A, Latham DG, Lissy NA, et al. Transduction of full-length TAT fusion proteins into mammalian cells: TAT-p27Kip1 induces cell migration. Nat Med. (1998) 4:1449–52. doi: 10.1038/4042
29. Vives E, Brodin P, Lebleu B. A truncated HIV-1 Tat protein basic domain rapidly translocates through the plasma membrane and accumulates in the cell nucleus. J Biol Chem. (1997) 272:16010–7. doi: 10.1074/jbc.272.25.16010
30. Ensoli B, Buonaguro L, Barillari G, Fiorelli V, Gendelman R, Morgan RA, et al. Release, uptake, and effects of extracellular human immunodeficiency virus type 1 Tat protein on cell growth and viral transactivation. J Virol. (1993) 67:277–87. doi: 10.1128/jvi.67.1.277-287.1993
31. Ruiz AP, Ajasin DO, Ramasamy S, DesMarais V, Eugenin EA, Prasad VR. A naturally occurring polymorphism in the HIV-1 tat basic domain inhibits uptake by bystander cells and leads to reduced neuroinflammation. Sci Rep. (2019) 9:3308. doi: 10.1038/s41598-019-39531-5
32. Ruiz AP, Prasad VR. Measuring the uptake and transactivation function of HIV-1 tat protein in a trans-cellular cocultivation setup. Methods Mol Biol. (2016) 1354:353–66. doi: 10.1007/978-1-4939-3046-3_24
33. Tyagi M, Rusnati M, Presta M, Giacca M. Internalization of HIV-1 tat requires cell surface heparan sulfate proteoglycans. J Biol Chem. (2001) 276:3254–61. doi: 10.1074/jbc.M006701200
34. King JE, Eugenin EA, Buckner CM, Berman JW. and neurotoxicity. Microbes Infect. (2006) 8:1347–57. doi: 10.1016/j.micinf.2005.11.014
35. Loret E. HIV extracellular Tat: myth or reality? Curr HIV Res. (2015) 13:90–7. doi: 10.2174/1570162x12666141202125643
36. Barillari G, Sgadari C, Fiorelli V, Samaniego F, Colombini S, Manzari V, et al. The Tat protein of human immunodeficiency virus type-1 promotes vascular cell growth and locomotion by engaging the alpha5beta1 and alphavbeta3 integrins and by mobilizing sequestered basic fibroblast growth factor. Blood. (1999) 94:663–72.
37. Barillari G, Sgadari C, Palladino C, Gendelman R, Caputo A, Morris CB, et al. Inflammatory cytokines synergize with the HIV-1 Tat protein to promote angiogenesis and Kaposi’s sarcoma via induction of basic fibroblast growth factor and the alpha v beta 3 integrin. J Immunol. (1999) 163:1929–35. doi: 10.4049/jimmunol.163.4.1929
38. Urbinati C, Mitola S, Tanghetti E, Kumar C, Waltenberger J, Ribatti D, et al. Integrin alphavbeta3 as a target for blocking HIV-1 Tat-induced endothelial cell activation in vitro and angiogenesis in vivo. Arterioscler Thromb Vasc Biol. (2005) 25:2315–20. doi: 10.1161/01.ATV.0000186182.14908.7b
39. Vogel BE, Lee SJ, Hildebrand A, Craig W, Pierschbacher MD, Wong-Staal F, et al. A novel integrin specificity exemplified by binding of the alpha v beta 5 integrin to the basic domain of the HIV Tat protein and vitronectin. J Cell Biol. (1993) 121:461–8. doi: 10.1083/jcb.121.2.461
40. Brake DA, Debouck C, Biesecker G. Identification of an Arg-Gly-Asp (RGD) cell adhesion site in human immunodeficiency virus type 1 transactivation protein, tat. J Cell Biol. (1990) 111:1275–81. doi: 10.1083/jcb.111.3.1275
41. Dickens AM, Yoo SW, Chin AC, Xu J, Johnson TP, Trout AL, et al. Chronic low-level expression of HIV-1 Tat promotes a neurodegenerative phenotype with aging. Sci Rep. (2017) 7:7748. doi: 10.1038/s41598-017-07570-5
42. Tugizov SM, Herrera R, Chin-Hong P, Veluppillai P, Greenspan D, Michael Berry J, et al. HIV-associated disruption of mucosal epithelium facilitates paracellular penetration by human papillomavirus. Virology. (2013) 446:378–88. doi: 10.1016/j.virol.2013.08.018
43. Rayne F, Debaisieux S, Bonhoure A, Beaumelle B. HIV-1 Tat is unconventionally secreted through the plasma membrane. Cell Biol Int. (2010) 34:409–13. doi: 10.1042/CBI20090376
44. Rayne F, Debaisieux S, Yezid H, Lin YL, Mettling C, Konate K, et al. Phosphatidylinositol-(4,5)-bisphosphate enables efficient secretion of HIV-1 Tat by infected T-cells. EMBO J. (2010) 29:1348–62. doi: 10.1038/emboj.2010.32
45. Lorenzo-Redondo R, Fryer HR, Bedford T, Kim EY, Archer J, Pond SLK, et al. Persistent HIV-1 replication maintains the tissue reservoir during therapy. Nature. (2016) 530:51–6. doi: 10.1038/nature16933
46. Shmakova A, Tsimailo I, Kozhevnikova Y, Gerard L, Boutboul D, Oksenhendler E, et al. HIV-1 Tat is present in the serum of people living with HIV-1 despite viral suppression. Int J Infect Dis. (2024) 142:106994. doi: 10.1016/j.ijid.2024.106994
47. Mele AR, Marino J, Chen K, Pirrone V, Janetopoulos C, Wigdahl B, et al. Defining the molecular mechanisms of HIV-1 Tat secretion: PtdIns(4,5)P(2) at the epicenter. Traffic. (2018). doi: 10.1111/tra.2018.19.issue-9
48. Rayne F, Debaisieux S, Tu A, Chopard C, Tryoen-Toth P, Beaumelle B. Detecting HIV-1 tat in cell culture supernatants by ELISA or western blot. Methods Mol Biol. (2016) 1354:329–42. doi: 10.1007/978-1-4939-3046-3_22
49. Rayne F, Vendeville A, Bonhoure A, Beaumelle B. The ability of chloroquine to prevent tat-induced cytokine secretion by monocytes is implicated in its in vivo anti-human immunodeficiency virus type 1 activity. J Virol. (2004) 78:12054–7. doi: 10.1128/JVI.78.21.12054-12057.2004
50. Vendeville A, Rayne F, Bonhoure A, Bettache N, Montcourrier P, Beaumelle B. HIV-1 Tat enters T cells using coated pits before translocating from acidified endosomes and eliciting biological responses. Mol Biol Cell. (2004) 15:2347–60. doi: 10.1091/mbc.e03-12-0921
51. Agostini S, Ali H, Vardabasso C, Fittipaldi A, Tasciotti E, Cereseto A, et al. Inhibition of non canonical HIV-1 tat secretion through the cellular na(+),K(+)-ATPase blocks HIV-1 infection. EBioMedicine. (2017) 21:170–81. doi: 10.1016/j.ebiom.2017.06.011
52. Rodriguez-Inigo E, Jimenez E, Bartolome J, Ortiz-Movilla N, Bartolome Villar B, Jose Arrieta J, et al. Detection of human immunodeficiency virus type 1 RNA by in situ hybridization in oral mucosa epithelial cells from anti-HIV-1 positive patients. J Med Virol. (2005) 77:17–22. doi: 10.1002/jmv.20409
53. Chou LL, Epstein J, Cassol SA, West DM, He W, Firth JD. Oral mucosal Langerhans’ cells as target, effector and vector in HIV infection. J Oral Pathol Med. (2000) 29:394–402. doi: 10.1034/j.1600-0714.2000.290805.x
54. Goto Y, Yeh CK, Notkins AL, Prabhakar BS. Detection of proviral sequences in saliva of patients infected with human immunodeficiency virus type 1. AIDS Res Hum Retroviruses. (1991) 7:343–7. doi: 10.1089/aid.1991.7.343
55. Kakizawa J, Ushijima H, Oka S, Ikeda Y, Schroder HC, Muller WE. Detection of human immunodeficiency virus-1 DNA, RNA and antibody, and occult blood in inactivated saliva: availability of the filter paper disk method. Acta Paediatr Jpn. (1996) 38:218–23. doi: 10.1111/j.1442-200X.1996.tb03473.x
56. Liuzzi G, Chirianni A, Clementi M, Bagnarelli P, Valenza A, Cataldo PT, et al. Analysis of HIV-1 load in blood, semen and saliva: evidence for different viral compartments in a cross-sectional and longitudinal study. Aids. (1996) 10:F51–6. doi: 10.1097/00002030-199612000-00001
57. Maticic M, Poljak M, Kramar B, Tomazic J, Vidmar L, Zakotnik B, et al. Proviral HIV-1 DNA in gingival crevicular fluid of HIV-1-infected patients in various stages of HIV disease. J Dent Res. (2000) 79:1496–501. doi: 10.1177/00220345000790071101
58. Qureshi MN, Barr CE, Hewlitt I, Boorstein R, Kong F, Bagasra O, et al. Detection of HIV in oral mucosal cells. Oral Dis. (1997) 3 Suppl 1:S73–8. doi: 10.1111/j.1601-0825.1997.tb00380.x
59. Qureshi MN, Barr CE, Seshamma T, Reidy J, Pomerantz RJ, Bagasra O. Infection of oral mucosal cells by human immunodeficiency virus type 1 in seropositive persons. J Infect Dis. (1995) 171:190–3. doi: 10.1093/infdis/171.1.190
60. Zuckerman RA, Whittington WL, Celum CL, Collis T, Lucchetti A, Sanchez JL, et al. Factors associated with oropharyngeal human immunodeficiency virus shedding. J Infect Dis. (2003) 188:142–5. doi: 10.1086/jid.2003.188.issue-1
61. Nuovo GJ, Forde A, MacConnell P, Fahrenwald R. In situ detection of PCR-amplified HIV-1 nucleic acids and tumor necrosis factor cDNA in cervical tissues. Am J Pathol. (1993) 143:40–8.
62. Clemetson DB, Moss GB, Willerford DM, Hensel M, Emonyi W, Holmes KK, et al. Detection of HIV DNA in cervical and vaginal secretions. Prevalence and correlates among women in Nairobi, Kenya. JAMA: J Am Med Assoc. (1993) 269:2860–4. doi: 10.1001/jama.1993.03500220046024
63. Sonza S, Mutimer HP, Oelrichs R, Jardine D, Harvey K, Dunne A, et al. Monocytes harbour replication-competent, non-latent HIV-1 in patients on highly active antiretroviral therapy. Aids. (2001) 15:17–22. doi: 10.1097/00002030-200101050-00005
64. Henning TR, Kissinger P, Lacour N, Meyaski-Schluter M, Clark R, Amedee AM. Elevated cervical white blood cell infiltrate is associated with genital HIV detection in a longitudinal cohort of antiretroviral therapy-adherent women. J Infect dis. (2010) 202:1543–52. doi: 10.1086/656720
65. Crowe SM, Sonza S. HIV-1 can be recovered from a variety of cells including peripheral blood monocytes of patients receiving highly active antiretroviral therapy: a further obstacle to eradication. J leukocyte Biol. (2000) 68:345–50. doi: 10.1189/jlb.68.3.345
66. Jayakumar P, Berger I, Autschbach F, Weinstein M, Funke B, Verdin E, et al. Tissue-resident macrophages are productively infected ex vivo by primary X4 isolates of human immunodeficiency virus type 1. J Virol. (2005) 79:5220–6. doi: 10.1128/JVI.79.8.5220-5226.2005
67. Westendorp MO, Frank R, Ochsenbauer C, Stricker K, Dhein J, Walczak H, et al. Sensitization of T cells to CD95-mediated apoptosis by HIV-1 Tat and gp120. Nature. (1995) 375:497–500. doi: 10.1038/375497a0
68. Xiao H, Neuveut C, Tiffany HL, Benkirane M, Rich EA, Murphy PM, et al. Selective CXCR4 antagonism by Tat: implications for in vivo expansion of coreceptor use by HIV-1. Proc Natl Acad Sci U S A. (2000) 97:11466–71. doi: 10.1073/pnas.97.21.11466
69. Poggi A, Zocchi MR. HIV-1 Tat triggers TGF-beta production and NK cell apoptosis that is prevented by pertussis toxin B. Clin Dev Immunol. (2006) 13:369–72. doi: 10.1080/17402520600645712
70. Germini D, Tsfasman T, Klibi M, El-Amine R, Pichugin A, Iarovaia OV, et al. HIV Tat induces a prolonged MYC relocalization next to IGH in circulating B-cells. Leukemia. (2017) 31:2515–22. doi: 10.1038/leu.2017.106
71. Poggi A, Carosio R, Fenoglio D, Brenci S, Murdaca G, Setti M, et al. Migration of V delta 1 and V delta 2 T cells in response to CXCR3 and CXCR4 ligands in healthy donors and HIV-1-infected patients: competition by HIV-1 Tat. Blood. (2004) 103:2205–13. doi: 10.1182/blood-2003-08-2928
72. Ensoli B, Barillari G, Salahuddin SZ, Gallo RC, Wong-Staal F. Tat protein of HIV-1 stimulates growth of cells derived from Kaposi’s sarcoma lesions of AIDS patients. Nature. (1990) 345:84–6. doi: 10.1038/345084a0
73. Johnson TP, Patel K, Johnson KR, Maric D, Calabresi PA, Hasbun R, et al. Induction of IL-17 and nonclassical T-cell activation by HIV-Tat protein. Proc Natl Acad Sci U S A. (2013) 110:13588–93. doi: 10.1073/pnas.1308673110
74. Marchio S, Alfano M, Primo L, Gramaglia D, Butini L, Gennero L, et al. Cell surface-associated Tat modulates HIV-1 infection and spreading through a specific interaction with gp120 viral envelope protein. Blood. (2005) 105:2802–11. doi: 10.1182/blood-2004-06-2212
75. Henderson LJ, Johnson TP, Smith BR, Reoma LB, Santamaria UA, Bachani M, et al. Presence of Tat and transactivation response element in spinal fluid despite antiretroviral therapy. AIDS. (2019) 33 Suppl 2:S145–S57. doi: 10.1097/QAD.0000000000002268
76. Choi JY, Hightower GK, Wong JK, Heaton R, Woods S, Grant I, et al. Genetic features of cerebrospinal fluid-derived subtype B HIV-1 tat. J Neurovirol. (2012) 18:81–90. doi: 10.1007/s13365-011-0059-9
77. Arts EJ, Hazuda DJ. HIV-1 antiretroviral drug therapy. Cold Spring Harb Perspect Med. (2012) 2:a007161. doi: 10.1101/cshperspect.a007161
78. Bachani M, Sacktor N, McArthur JC, Nath A, Rumbaugh J. Detection of anti-tat antibodies in CSF of individuals with HIV-associated neurocognitive disorders. J Neurovirol. (2013) 19:82–8. doi: 10.1007/s13365-012-0144-8
79. Dube M, Tastet O, Dufour C, Sannier G, Brassard N, Delgado GG, et al. Spontaneous HIV expression during suppressive ART is associated with the magnitude and function of HIV-specific CD4(+) and CD8(+) T cells. Cell Host Microbe. (2023) 31:1507–22 e5. doi: 10.1016/j.chom.2023.08.006
80. Fletcher CV, Staskus K, Wietgrefe SW, Rothenberger M, Reilly C, Chipman JG, et al. Persistent HIV-1 replication is associated with lower antiretroviral drug concentrations in lymphatic tissues. Proc Natl Acad Sci U S A. (2014) 111:2307–12. doi: 10.1073/pnas.1318249111
81. Huang Y, Hoque MT, Jenabian MA, Vyboh K, Whyte SK, Sheehan NL, et al. Antiretroviral drug transporters and metabolic enzymes in human testicular tissue: potential contribution to HIV-1 sanctuary site. J Antimicrob Chemother. (2016) 71:1954–65. doi: 10.1093/jac/dkw046
82. Lorenzo-Redondo R, Fryer HR, Bedford T, Kim EY, Archer J, Kosakovsky Pond SL, et al. Persistent HIV-1 replication maintains the tissue reservoir during therapy. Nature. (2016) 530:51–6. doi: 10.1038/nature16933
83. Di Mascio M, Srinivasula S, Bhattacharjee A, Cheng L, Martiniova L, Herscovitch P, et al. Antiretroviral tissue kinetics: in vivo imaging using positron emission tomography. Antimicrob Agents Chemother. (2009) 53:4086–95. doi: 10.1128/AAC.00419-09
84. Cummins JE, Christensen L, Lennox JL, Bush TJ, Wu Z, Malamud D, et al. Mucosal innate immune factors in the female genital tract are associated with vaginal HIV-1 shedding independent of plasma viral load. AIDS Res Hum Retroviruses. (2006) 22:788–95. doi: 10.1089/aid.2006.22.788
85. Debiaggi M, Zara F, Spinillo A, De Santolo A, Maserati R, Bruno R, et al. Viral excretion in cervicovaginal secretions of HIV-1-infected women receiving antiretroviral therapy. Eur J Clin Microbiol Infect Dis. (2001) 20:91–6. doi: 10.1007/s100960000442
86. Graham SM, Holte SE, Peshu NM, Richardson BA, Panteleeff DD, Jaoko WG, et al. Initiation of antiretroviral therapy leads to a rapid decline in cervical and vaginal HIV-1 shedding. AIDS. (2007) 21:501–7. doi: 10.1097/QAD.0b013e32801424bd
87. Neely MN, Benning L, Xu J, Strickler HD, Greenblatt RM, Minkoff H, et al. Cervical shedding of HIV-1 RNA among women with low levels of viremia while receiving highly active antiretroviral therapy. J Acquir Immune Defic Syndr. (2007) 44:38–42. doi: 10.1097/01.qai.0000248352.18007.1f
88. Vernazza PL. Genital shedding of HIV-1 despite successful antiretroviral therapy. Lancet. (2001) 358:1564. doi: 10.1016/S0140-6736(01)06665-X
89. Sheth PM, Kovacs C, Kemal KS, Jones RB, Raboud JM, Pilon R, et al. Persistent HIV RNA shedding in semen despite effective antiretroviral therapy. AIDS. (2009) 23:2050–4. doi: 10.1097/QAD.0b013e3283303e04
90. Schluter H, Wepf R, Moll I, Franke WW. Sealing the live part of the skin: the integrated meshwork of desmosomes, tight junctions and curvilinear ridge structures in the cells of the uppermost granular layer of the human epidermis. Eur J Cell Biol. (2004) 83:655–65. doi: 10.1078/0171-9335-00434
91. Langbein L, Pape UF, Grund C, Kuhn C, Praetzel S, Moll I, et al. Tight junction-related structures in the absence of a lumen: occludin, claudins and tight junction plaque proteins in densely packed cell formations of stratified epithelia and squamous cell carcinomas. Eur J Cell Biol. (2003) 82:385–400. doi: 10.1078/0171-9335-00330
92. Langbein L, Grund C, Kuhn C, Praetzel S, Kartenbeck J, Brandner JM, et al. Tight junctions and compositionally related junctional structures in mammalian stratified epithelia and cell cultures derived therefrom. Eur J Cell Biol. (2002) 81:419–35. doi: 10.1078/0171-9335-00270
93. Brandner JM, Kief S, Grund C, Rendl M, Houdek P, Kuhn C, et al. Organization and formation of the tight junction system in human epidermis and cultured keratinocytes. Eur J Cell Biol. (2002) 81:253–63. doi: 10.1078/0171-9335-00244
94. Takano K, Kojima T, Go M, Murata M, Ichimiya S, Himi T, et al. HLA-DR- and CD11c-positive dendritic cells penetrate beyond well-developed epithelial tight junctions in human nasal mucosa of allergic rhinitis. J Histochem Cytochem. (2005) 53:611–9. doi: 10.1369/jhc.4A6539.2005
95. Blaskewicz CD, Pudney J, Anderson DJ. Structure and function of intercellular junctions in human cervical and vaginal mucosal epithelia. Biol reprod. (2011) 85:97–104. doi: 10.1095/biolreprod.110.090423
96. Tugizov SM, Herrera R, Veluppillai P, Greenspan D, Soros V, Greene WC, et al. HIV is inactivated after transepithelial migration via adult oral epithelial cells but not fetal epithelial cells. Virology. (2011) 409:211–22. doi: 10.1016/j.virol.2010.10.004
97. Tugizov SM, Herrera R, Veluppillai P, Greenspan D, Soros V, Greene WC, et al. Differential transmission of HIV traversing fetal oral/intestinal epithelia and adult oral epithelia. J Virol. (2012) 86:2556–70. doi: 10.1128/JVI.06578-11
98. Go M, Kojima T, Takano K, Murata M, Ichimiya S, Tsubota H, et al. Expression and function of tight junctions in the crypt epithelium of human palatine tonsils. J Histochem Cytochem. (2004) 52:1627–38. doi: 10.1369/jhc.4A6339.2004
99. Tugizov S. Virus-associated disruption of mucosal epithelial tight junctions and its role in viral transmission and spread. Tissue Barriers. (2021) 9:1943274. doi: 10.1080/21688370.2021.1943274
100. Tugizov S. Human immunodeficiency virus-associated disruption of mucosal barriers and its role in HIV transmission and pathogenesis of HIV/AIDS disease. Tissue Barriers. (2016) 4:e1159276. doi: 10.1080/21688370.2016.1159276
101. Sawada N, Murata M, Kikuchi K, Osanai M, Tobioka H, Kojima T, et al. Tight junctions and human diseases. Med Electron Microsc. (2003) 36:147–56. doi: 10.1007/s00795-003-0219-y
102. Niessen CM. Tight junctions/adherens junctions: basic structure and function. J Invest Dermatol. (2007) 127:2525–32. doi: 10.1038/sj.jid.5700865
103. Naik UP, Eckfeld K. Junctional adhesion molecule 1 (JAM-1). J Biol Regul Homeost Agents. (2003) 17:341–7.
104. Hartsock A, Nelson WJ. Adherens and tight junctions: structure, function and connections to the actin cytoskeleton. Biochim Biophys Acta. (2008) 1778:660–9. doi: 10.1016/j.bbamem.2007.07.012
105. Epple HJ, Allers K, Troger H, Kuhl A, Erben U, Fromm M, et al. Acute HIV infection induces mucosal infiltration with CD4+ and CD8+ T cells, epithelial apoptosis, and a mucosal barrier defect. Gastroenterology. (2010) 139:1289–300. doi: 10.1053/j.gastro.2010.06.065
106. Epple HJ, Schneider T, Troeger H, Kunkel D, Allers K, Moos V, et al. Impairment of the intestinal barrier is evident in untreated but absent in suppressively treated HIV-infected patients. Gut. (2009) 58:220–7. doi: 10.1136/gut.2008.150425
107. Assimakopoulos SF, Dimitropoulou D, Marangos M, Gogos CA. Intestinal barrier dysfunction in HIV infection: pathophysiology, clinical implications and potential therapies. Infection. (2014) 42:951–9. doi: 10.1007/s15010-014-0666-5
108. Sufiawati I, Tugizov SM. HIV-associated disruption of tight and adherens junctions of oral epithelial cells facilitates HSV-1 infection and spread. PloS One. (2014) 9:e88803. doi: 10.1371/journal.pone.0088803
109. Sufiawati I, Tugizov SM. HIV-induced matrix metalloproteinase-9 activation through mitogen-activated protein kinase signalling promotes HSV-1 cell-to-cell spread in oral epithelial cells. J Gen Virol. (2018) 99:937–47. doi: 10.1099/jgv.0.001075
110. Schiffman M, Doorbar J, Wentzensen N, de Sanjose S, Fakhry C, Monk BJ, et al. Carcinogenic human papillomavirus infection. Nat Rev Dis Primers. (2016) 2:16086. doi: 10.1038/nrdp.2016.86
111. Sathish N, Wang X, Yuan Y. Human papillomavirus (HPV)-associated oral cancers and treatment strategies. J Dent Res. (2014) 93:29S–36S. doi: 10.1177/0022034514527969
112. Bushara O, Krogh K, Weinberg SE, Finkelman BS, Sun L, Liao J, et al. Human immunodeficiency virus infection promotes human papillomavirus-mediated anal squamous carcinogenesis: an immunologic and pathobiologic review. Pathobiology. (2022) 89:1–12. doi: 10.1159/000518758
113. Liu G, Sharma M, Tan N, Barnabas RV. HIV-positive women have higher risk of human papilloma virus infection, precancerous lesions, and cervical cancer. AIDS. (2018) 32:795–808. doi: 10.1097/QAD.0000000000001765
114. Palefsky JM. Human papillomavirus-associated anal and cervical cancers in HIV-infected individuals: incidence and prevention in the antiretroviral therapy era. Curr Opin HIV AIDS. (2017) 12:26–30. doi: 10.1097/COH.0000000000000336
115. Zhu Z, Zhang Y, Wang H, Jiang T, Zhang M, Zhang Y, et al. Renal cell carcinoma associated with HIV/AIDS: A review of the epidemiology, risk factors, diagnosis, and treatment. Front Oncol. (2022) 12:872438. doi: 10.3389/fonc.2022.872438
116. McLemore MS, Haigentz M Jr., Smith RV, Nuovo GJ, Alos L, Cardesa A, et al. Head and neck squamous cell carcinomas in HIV-positive patients: a preliminary investigation of viral associations. Head Neck Pathol. (2010) 4:97–105. doi: 10.1007/s12105-010-0171-9
117. Lee SO, Lee JE, Lee S, Lee SH, Kang JS, Son H, et al. Nationwide population-based incidence of cancer among patients with HIV/AIDS in South Korea. Sci Rep. (2022) 12:9974. doi: 10.1038/s41598-022-14170-5
118. Negri F, Missale G, Antoni AD, Porta C. Hepatocellular cancer therapy in patients with HIV infection: Disparities in cancer care, trials enrolment, and cancer-related research. Transl Oncol. (2021) 14:101153. doi: 10.1016/j.tranon.2021.101153
119. Isaguliants M, Bayurova E, Avdoshina D, Kondrashova A, Chiodi F, Palefsky JM. Oncogenic effects of HIV-1 proteins, mechanisms behind. Cancers (Basel). (2021) 13. doi: 10.3390/cancers13020305
120. Lien K, Mayer W, Herrera R, Padilla NT, Cai X, Lin V, et al. HIV-1 proteins gp120 and tat promote epithelial-mesenchymal transition and invasiveness of HPV-positive and HPV-negative neoplastic genital and oral epithelial cells. Microbiol Spectr. (2022) 10:e0362222. doi: 10.1128/spectrum.03622-22
121. Lien K, Mayer W, Herrera R, Rosbe K, Tugizov SM. HIV-1 proteins gp120 and tat induce the epithelial-mesenchymal transition in oral and genital mucosal epithelial cells. PloS One. (2019) 14:e0226343. doi: 10.1371/journal.pone.0226343
122. Doorbar J. The papillomavirus life cycle. J Clin Virol. (2005) 32 Suppl 1:S7–15. doi: 10.1016/j.jcv.2004.12.006
123. Ocana OH, Nieto MA. Epithelial plasticity, stemness and pluripotency. Cell Res. (2010) 20:1086–8. doi: 10.1038/cr.2010.127
124. Lamouille S, Xu J, Derynck R. Molecular mechanisms of epithelial-mesenchymal transition. Nat Rev Mol Cell Biol. (2014) 15:178–96. doi: 10.1038/nrm3758
125. Lee MY, Shen MR. Epithelial-mesenchymal transition in cervical carcinoma. Am J Transl Res. (2012) 4:1–13.
126. Hsu YM, Chen YF, Chou CY, Tang MJ, Chen JH, Wilkins RJ, et al. KCl cotransporter-3 down-regulates E-cadherin/beta-catenin complex to promote epithelial-mesenchymal transition. Cancer Res. (2007) 67:11064–73. doi: 10.1158/0008-5472.CAN-07-2443
127. Lee MY, Chou CY, Tang MJ, Shen MR. Epithelial-mesenchymal transition in cervical cancer: correlation with tumor progression, epidermal growth factor receptor overexpression, and snail up-regulation. Clin Cancer Res. (2008) 14:4743–50. doi: 10.1158/1078-0432.CCR-08-0234
128. Gilles C, Polette M, Piette J, Delvigne AC, Thompson EW, Foidart JM, et al. Vimentin expression in cervical carcinomas: association with invasive and migratory potential. J pathol. (1996) 180:175–80. doi: 10.1002/(SICI)1096-9896(199610)180:2<175::AID-PATH630>3.0.CO;2-G
129. Ma Z, Zhang C, Liu X, Fang F, Liu S, Liao X, et al. Characterisation of a subpopulation of CD133(+) cancer stem cells from Chinese patients with oral squamous cell carcinoma. Sci Rep. (2020) 10:8875. doi: 10.1038/s41598-020-64947-9
130. Chinn SB, Darr OA, Owen JH, Bellile E, McHugh JB, Spector ME, et al. Cancer stem cells: mediators of tumorigenesis and metastasis in head and neck squamous cell carcinoma. Head Neck. (2015) 37:317–26. doi: 10.1002/hed.23600
131. Caspa Gokulan R, Devaraj H. Stem cell markers CXCR-4 and CD133 predict aggressive phenotype and their double positivity indicates poor prognosis of oral squamous cell carcinoma. Cancers (Basel). (2021) 13. doi: 10.3390/cancers13235895
132. Wang P, Yang B, Huang H, Liang P, Long B, Chen L, et al. HIV gp120/Tat protein-induced epithelial-mesenchymal transition promotes the progression of cervical lesions. AIDS Res Ther. (2023) 20:82. doi: 10.1186/s12981-023-00577-1
133. Barillari G, Bei R, Manzari V, Modesti A. Infection by high-risk human papillomaviruses, epithelial-to-mesenchymal transition and squamous pre-malignant or Malignant lesions of the uterine cervix: A series of chained events? Int J Mol Sci. (2021) 22. doi: 10.3390/ijms222413543
134. Gupta PB, Pastushenko I, Skibinski A, Blanpain C, Kuperwasser C. Phenotypic plasticity: driver of cancer initiation, progression, and therapy resistance. Cell Stem Cell. (2019) 24:65–78. doi: 10.1016/j.stem.2018.11.011
135. Jolly MK, Boareto M, Huang B, Jia D, Lu M, Ben-Jacob E, et al. Implications of the hybrid epithelial/mesenchymal phenotype in metastasis. Front Oncol. (2015) 5:155. doi: 10.3389/fonc.2015.00155
136. Jolly MK, Murphy RJ, Bhatia S, Whitfield HJ, Redfern A, Davis MJ, et al. Measuring and modelling the epithelial- mesenchymal hybrid state in cancer: clinical implications. Cells Tissues Organs. (2022) 211:110–33. doi: 10.1159/000515289
137. Grosse-Wilde A, Fouquier d’Herouel A, McIntosh E, Ertaylan G, Skupin A, Kuestner RE, et al. Stemness of the hybrid epithelial/mesenchymal state in breast cancer and its association with poor survival. PloS One. (2015) 10:e0126522. doi: 10.1371/journal.pone.0126522
138. Ruscetti M, Quach B, Dadashian EL, Mulholland DJ, Wu H. Tracking and functional characterization of epithelial-mesenchymal transition and mesenchymal tumor cells during prostate cancer metastasis. Cancer Res. (2015) 75:2749–59. doi: 10.1158/0008-5472.CAN-14-3476
139. Strauss R, Li ZY, Liu Y, Beyer I, Persson J, Sova P, et al. Analysis of epithelial and mesenchymal markers in ovarian cancer reveals phenotypic heterogeneity and plasticity. PloS One. (2011) 6:e16186. doi: 10.1371/journal.pone.0016186
140. Jolly MK, Huang B, Lu M, Mani SA, Levine H, Ben-Jacob E. Towards elucidating the connection between epithelial-mesenchymal transitions and stemness. J R Soc Interf. (2014) 11:20140962. doi: 10.1098/rsif.2014.0962
141. Celia-Terrassa T, Jolly MK. Cancer stem cells and epithelial-to-mesenchymal transition in cancer metastasis. Cold Spring Harb Perspect Med. (2020) 10. doi: 10.1101/cshperspect.a036905
142. Yan Y, Zuo X, Wei D. Concise review: emerging role of CD44 in cancer stem cells: A promising biomarker and therapeutic target. Stem Cells Transl Med. (2015) 4:1033–43. doi: 10.5966/sctm.2015-0048
143. Ju F, Atyah MM, Horstmann N, Gul S, Vago R, Bruns CJ, et al. Characteristics of the cancer stem cell niche and therapeutic strategies. Stem Cell Res Ther. (2022) 13:233. doi: 10.1186/s13287-022-02904-1
144. Ghazi N, Saghravanian N, Taghi Shakeri M, Jamali M. Evaluation of CD44 and TGF-B expression in oral carcinogenesis. J Dent (Shiraz). (2021) 22:33–40.
145. Mirhashemi M, Ghazi N, Saghravanian N, Taghipour A, Mohajertehran F. Evaluation of CD24 and CD44 as cancer stem cell markers in squamous cell carcinoma and epithelial dysplasia of the oral cavity by q- RT-PCR. Dent Res J (Isfahan). (2020) 17:208–12. doi: 10.4103/1735-3327.284727
146. Saghravanian N, Anvari K, Ghazi N, Memar B, Shahsavari M, Aghaee MA. Expression of p63 and CD44 in oral squamous cell carcinoma and correlation with clinicopathological parameters. Arch Oral Biol. (2017) 82:160–5. doi: 10.1016/j.archoralbio.2017.06.011
147. Bose B, Nihad M, Sudheer SP. Pluripotent stem cells: Basic biology or else differentiations aimed at translational research and the role of flow cytometry. Cytom A. (2023) 103:368–77. doi: 10.1002/cyto.a.v103.5
148. Marcato P, Dean CA, Giacomantonio CA, Lee PW. Aldehyde dehydrogenase: its role as a cancer stem cell marker comes down to the specific isoform. Cell Cycle. (2011) 10:1378–84. doi: 10.4161/cc.10.9.15486
149. Marcato P, Dean CA, Pan D, Araslanova R, Gillis M, Joshi M, et al. Aldehyde dehydrogenase activity of breast cancer stem cells is primarily due to isoform ALDH1A3 and its expression is predictive of metastasis. Stem Cells. (2011) 29:32–45. doi: 10.1002/stem.563
150. Emich H, Chapireau D, Hutchison I, Mackenzie I. The potential of CD44 as a diagnostic and prognostic tool in oral cancer. J Oral Pathol Med. (2015) 44:393–400. doi: 10.1111/jop.2015.44.issue-6
151. Tong D, Sun J, Huang P, Li M, Zhang F. p75 neurotrophin receptor: A potential surface marker of tongue squamous cell carcinoma stem cells. Mol Med Rep. (2017) 15:2521–9. doi: 10.3892/mmr.2017.6291
152. Barzegar Behrooz A, Syahir A, Ahmad S. CD133: beyond a cancer stem cell biomarker. J Drug Targeting. (2019) 27:257–69. doi: 10.1080/1061186X.2018.1479756
153. Miyata Y, Kumagai K, Nagaoka T, Kitaura K, Kaneda G, Kanazawa H, et al. Clinicopathological significance and prognostic value of Wilms’ tumor gene expression in colorectal cancer. Cancer biomark. (2015) 15:789–97. doi: 10.3233/CBM-150521
154. Amini S, Fathi F, Mobalegi J, Sofimajidpour H, Ghadimi T. The expressions of stem cell markers: Oct4, Nanog, Sox2, nucleostemin, Bmi, Zfx, Tcl1, Tbx3, Dppa4, and Esrrb in bladder, colon, and prostate cancer, and certain cancer cell lines. Anat Cell Biol. (2014) 47:1–11. doi: 10.5115/acb.2014.47.1.1
155. Herreros-Pomares A. Identification, culture and targeting of cancer stem cells. Life (Basel). (2022) 12. doi: 10.3390/life12020184
156. Herreros-Pomares A, de-Maya-Girones JD, Calabuig-Farinas S, Lucas R, Martinez A, Pardo-Sanchez JM, et al. Lung tumorspheres reveal cancer stem cell-like properties and a score with prognostic impact in resected non-small-cell lung cancer. Cell Death Dis. (2019) 10:660. doi: 10.1038/s41419-019-1898-1
157. Baillie R, Itinteang T, Yu HH, Brasch HD, Davis PF, Tan ST. Cancer stem cells in moderately differentiated oral tongue squamous cell carcinoma. J Clin Pathol. (2016) 69:742–4. doi: 10.1136/jclinpath-2015-203599
158. Baillie R, Tan ST, Itinteang T. Cancer stem cells in oral cavity squamous cell carcinoma: A review. Front Oncol. (2017) 7:112. doi: 10.3389/fonc.2017.00112
159. Amor NG, Buzo RF, Ortiz RC, Lopes NM, Saito LM, Mackenzie IC, et al. In vitro and in vivo characterization of cancer stem cell subpopulations in oral squamous cell carcinoma. J Oral Pathol Med. (2021) 50:52–9. doi: 10.1111/jop.13101
160. de Andrade NP, Rodrigues MF, Rodini CO, Nunes FD. Cancer stem cell, cytokeratins and epithelial to mesenchymal transition markers expression in oral squamous cell carcinoma derived from ortothopic xenoimplantation of CD44(high) cells. Pathol Res Pract. (2017) 213:235–44. doi: 10.1016/j.prp.2016.12.009
161. de Camargo MR, Frazon TF, Inacio KK, Smiderle FR, Amor NG, Dionisio TJ, et al. Ganoderma lucidum polysaccharides inhibit in vitro tumorigenesis, cancer stem cell properties and epithelial-mesenchymal transition in oral squamous cell carcinoma. J Ethnopharmacol. (2022) 286:114891. doi: 10.1016/j.jep.2021.114891
162. Ortiz RC, Lopes NM, Amor NG, Ponce JB, Schmerling CK, Lara VS, et al. CD44 and ALDH1 immunoexpression as prognostic indicators of invasion and metastasis in oral squamous cell carcinoma. J Oral Pathol Med. (2018) 47:740–7. doi: 10.1111/jop.2018.47.issue-8
163. Rodini CO, Lopes NM, Lara VS, Mackenzie IC. Oral cancer stem cells - properties and consequences. J Appl Oral Sci. (2017) 25:708–15. doi: 10.1590/1678-7757-2016-0665
164. Rodrigues M, Miguita L, De Andrade NP, Heguedusch D, Rodini CO, Moyses RA, et al. GLI3 knockdown decreases stemness, cell proliferation and invasion in oral squamous cell carcinoma. Int J Oncol. (2018) 53:2458–72. doi: 10.3892/ijo.2018.4572
165. Shigeishi H, Biddle A, Gammon L, Emich H, Rodini CO, Gemenetzidis E, et al. Maintenance of stem cell self-renewal in head and neck cancers requires actions of GSK3beta influenced by CD44 and RHAMM. Stem Cells. (2013) 31:2073–83. doi: 10.1002/stem.1418
166. Shigeishi H, Biddle A, Gammon L, Rodini CO, Yamasaki M, Seino S, et al. Elevation in 5-FU-induced apoptosis in head and neck cancer stem cells by a combination of CDHP and GSK3beta inhibitors. J Oral Pathol Med. (2015) 44:201–7. doi: 10.1111/jop.2015.44.issue-3
167. Siqueira JM, Heguedusch D, Rodini CO, Nunes FD, Rodrigues M. Mechanisms involved in cancer stem cell resistance in head and neck squamous cell carcinoma. Cancer Drug Resist. (2023) 6:116–37. doi: 10.20517/cdr.2022.107
168. Xavier FCA, Silva JC, Rodini CO, Rodrigues M. Mechanisms of immune evasion by head and neck cancer stem cells. Front Oral Health. (2022) 3:957310. doi: 10.3389/froh.2022.957310
169. Pal A, Barrett TF, Paolini R, Parikh A, Puram SV. Partial EMT in head and neck cancer biology: a spectrum instead of a switch. Oncogene. (2021) 40:5049–65. doi: 10.1038/s41388-021-01868-5
170. Krishnan H, Rayes J, Miyashita T, Ishii G, Retzbach EP, Sheehan SA, et al. Podoplanin: An emerging cancer biomarker and therapeutic target. Cancer Sci. (2018) 109:1292–9. doi: 10.1111/cas.2018.109.issue-5
171. Liao C, Wang Q, An J, Long Q, Wang H, Xiang M, et al. Partial EMT in squamous cell carcinoma: A snapshot. Int J Biol Sci. (2021) 17:3036–47. doi: 10.7150/ijbs.61566
172. Kisoda S, Mouri Y, Kitamura N, Yamamoto T, Miyoshi K, Kudo Y. The role of partial-EMT in the progression of head and neck squamous cell carcinoma. J Oral Biosci. (2022) 64:176–82. doi: 10.1016/j.job.2022.02.004
173. Gordon KJ, Blobe GC. Role of transforming growth factor-beta superfamily signaling pathways in human disease. Biochim Biophys Acta. (2008) 1782:197–228. doi: 10.1016/j.bbadis.2008.01.006
174. Blobe GC, Schiemann WP, Lodish HF. Role of transforming growth factor beta in human disease. New Engl J Med. (2000) 342:1350–8. doi: 10.1056/NEJM200005043421807
175. Wendt MK, Tian M, Schiemann WP. Deconstructing the mechanisms and consequences of TGF-beta-induced EMT during cancer progression. Cell Tissue Res. (2012) 347:85–101. doi: 10.1007/s00441-011-1199-1
176. Barrallo-Gimeno A, Nieto MA. The Snail genes as inducers of cell movement and survival: implications in development and cancer. Development. (2005) 132:3151–61. doi: 10.1242/dev.01907
177. Toschi E, Bacigalupo I, Strippoli R, Chiozzini C, Cereseto A, Falchi M, et al. HIV-1 Tat regulates endothelial cell cycle progression via activation of the Ras/ERK MAPK signaling pathway. Mol Biol Cell. (2006) 17:1985–94. doi: 10.1091/mbc.e05-08-0717
178. Chen Q, Sivakumar P, Barley C, Peters DM, Gomes RR, Farach-Carson MC, et al. Potential role for heparan sulfate proteoglycans in regulation of transforming growth factor-beta (TGF-beta) by modulating assembly of latent TGF-beta-binding protein-1. J Biol Chem. (2007) 282:26418–30. doi: 10.1074/jbc.M703341200
179. Urbinati C, Milanesi M, Lauro N, Bertelli C, David G, D’Ursi P, et al. HIV-1 tat and heparan sulfate proteoglycans orchestrate the setup of in cis and in trans cell-surface interactions functional to lymphocyte trans-endothelial migration. Molecules. (2021) 26. doi: 10.3390/molecules26247488
180. Nyagol J, Leucci E, Onnis A, De Falco G, Tigli C, Sanseverino F, et al. The effects of HIV-1 Tat protein on cell cycle during cervical carcinogenesis. Cancer Biol Ther. (2006) 5:684–90. doi: 10.4161/cbt.5.6.2907
181. Tornesello ML, Buonaguro FM, Beth-Giraldo E, Giraldo G. Human immunodeficiency virus type 1 tat gene enhances human papillomavirus early gene expression. Intervirology. (1993) 36:57–64. doi: 10.1159/000150322
182. Huynh D, Vincan E, Mantamadiotis T, Purcell D, Chan CK, Ramsay R. Oncogenic properties of HIV-Tat in colorectal cancer cells. Curr HIV Res. (2007) 5:403–9. doi: 10.2174/157016207781023974
183. Liu YP, Chen CH, Yen CH, Tung CW, Chen CJ, Chen YA, et al. Human immunodeficiency virus Tat-TIP30 interaction promotes metastasis by enhancing the nuclear translocation of Snail in lung cancer cell lines. Cancer Sci. (2018) 109:3105–14. doi: 10.1111/cas.2018.109.issue-10
184. Rubino C, Stinco M, Indolfi G. Hepatitis co-infection in paediatric HIV: progressing treatment and prevention. Curr Opin HIV AIDS. (2024) 19(6):338–47. doi: 10.1097/COH.0000000000000882
185. Sultana C, Falanga C, Chicin G, Ion L, Grancea C, Chiriac D, et al. HIV, HCV and HIV-HCV coinfections in the general population versus inmates from Romania. Viruses. (2024) 16. doi: 10.3390/v16081279
186. Gobran ST, Ancuta P, Shoukry NH. A tale of two viruses: immunological insights into HCV/HIV coinfection. Front Immunol. (2021) 12:726419. doi: 10.3389/fimmu.2021.726419
187. Gobran ST, Pagliuzza A, Khedr O, Fert A, Chomont N, Bruneau J, et al. DAA-mediated HCV cure reduces HIV DNA levels in HCV/HIV coinfected people. J Virol. (2023) 97:e0110523. doi: 10.1128/jvi.01105-23
188. Bourcier V, Winnock M, Ait Ahmed M, Sogni P, Pambrun E, Poizot-Martin I, et al. Primary liver cancer is more aggressive in HIV-HCV coinfection than in HCV infection. A prospective study (ANRS CO13 Hepavih and CO12 Cirvir). Clin Res Hepatol Gastroenterol. (2012) 36:214–21. doi: 10.1016/j.clinre.2011.11.002
189. Theron AJ, Anderson R, Rossouw TM, Steel HC. The role of transforming growth factor beta-1 in the progression of HIV/AIDS and development of non-AIDS-defining fibrotic disorders. Front Immunol. (2017) 8:1461. doi: 10.3389/fimmu.2017.01461
190. Wiercinska-Drapalo A, Flisiak R, Jaroszewicz J, Prokopowicz D. Increased plasma transforming growth factor-beta1 is associated with disease progression in HIV-1-infected patients. Viral Immunol. (2004) 17:109–13. doi: 10.1089/088282404322875502
191. Maina EK, Abana CZ, Bukusi EA, Sedegah M, Lartey M, Ampofo WK. Plasma concentrations of transforming growth factor beta 1 in non-progressive HIV-1 infection correlates with markers of disease progression. Cytokine. (2016) 81:109–16. doi: 10.1016/j.cyto.2016.02.009
192. Dickinson M, Kliszczak AE, Giannoulatou E, Peppa D, Pellegrino P, Williams I, et al. Dynamics of transforming growth factor (TGF)-beta superfamily cytokine induction during HIV-1 infection are distinct from other innate cytokines. Front Immunol. (2020) 11:596841. doi: 10.3389/fimmu.2020.596841
193. Yim LY, Lam KS, Luk TY, Mo Y, Lu X, Wang J, et al. Transforming growth factor beta signaling promotes HIV-1 infection in activated and resting memory CD4(+) T cells. J Virol. (2023) 97:e0027023. doi: 10.1128/jvi.00270-23
194. Chinnapaiyan S, Dutta RK, Nair M, Chand HS, Rahman I, Unwalla HJ. TGF-beta1 increases viral burden and promotes HIV-1 latency in primary differentiated human bronchial epithelial cells. Sci Rep. (2019) 9:12552. doi: 10.1038/s41598-019-49056-6
195. Reinhold D, Wrenger S, Kahne T, Ansorge S. HIV-1 Tat: immunosuppression via TGF-beta1 induction. Immunol Today. (1999) 20:384–5. doi: 10.1016/S0167-5699(99)01497-8
196. Zauli G, Davis BR, Re MC, Visani G, Furlini G, La Placa M. tat protein stimulates production of transforming growth factor-beta 1 by marrow macrophages: a potential mechanism for human immunodeficiency virus-1-induced hematopoietic suppression. Blood. (1992) 80:3036–43. doi: 10.1182/blood.V80.12.3036.3036
197. Aboushala A, Kugel G, Hurley E. Class II composite resin restorations using glass-ionomer liners: microleakage studies. J Clin Pediatr Dent. (1996) 21:67–70. doi: 10.1128/jvi.00270-23
198. Hu R, Oyaizu N, Kalyanaraman VS, Pahwa S. HIV-1 gp160 as a modifier of Th1 and Th2 cytokine response: gp160 suppresses interferon-gamma and interleukin-2 production concomitantly with enhanced interleukin-4 production in vitro. Clin Immunol Immunopathol. (1994) 73:245–51. doi: 10.1006/clin.1994.1194
199. Lijnen PJ, Petrov VV, Fagard RH. Induction of cardiac fibrosis by transforming growth factor-beta(1). Mol Genet Metab. (2000) 71:418–35. doi: 10.1006/mgme.2000.3032
200. Mehta T, Buzkova P, Kizer JR, Djousse L, Chonchol M, Mukamal KJ, et al. Higher plasma transforming growth factor (TGF)-beta is associated with kidney disease in older community dwelling adults. BMC Nephrol. (2017) 18:98. doi: 10.1186/s12882-017-0509-6
201. Hernandez-Gea V, Friedman SL. Pathogenesis of liver fibrosis. Annu Rev Pathol. (2011) 6:425–56. doi: 10.1146/annurev-pathol-011110-130246
202. Barisoni L, Bruggeman LA, Mundel P, D’Agati VD, Klotman PE. HIV-1 induces renal epithelial dedifferentiation in a transgenic model of HIV-associated nephropathy. Kidney Int. (2000) 58:173–81. doi: 10.1046/j.1523-1755.2000.00152.x
203. Schwartz EJ, Cara A, Snoeck H, Ross MD, Sunamoto M, Reiser J, et al. Human immunodeficiency virus-1 induces loss of contact inhibition in podocytes. J Am Soc Nephrol. (2001) 12:1677–84. doi: 10.1681/ASN.V1281677
204. Medapalli RK, He JC, Klotman PE. HIV-associated nephropathy: pathogenesis. Curr Opin Nephrol Hypertens. (2011) 20:306–11. doi: 10.1097/MNH.0b013e328345359a
205. Bruggeman LA, Ross MD, Tanji N, Cara A, Dikman S, Gordon RE, et al. Renal epithelium is a previously unrecognized site of HIV-1 infection. J Am Soc Nephrol. (2000) 11:2079–87. doi: 10.1681/ASN.V11112079
206. Lu TC, He JC, Wang ZH, Feng X, Fukumi-Tominaga T, Chen N, et al. HIV-1 Nef disrupts the podocyte actin cytoskeleton by interacting with diaphanous interacting protein. J Biol Chem. (2008) 283:8173–82. doi: 10.1074/jbc.M708920200
207. Kopp JB, Klotman ME, Adler SH, Bruggeman LA, Dickie P, Marinos NJ, et al. Progressive glomerulosclerosis and enhanced renal accumulation of basement membrane components in mice transgenic for human immunodeficiency virus type 1 genes. Proc Natl Acad Sci United States America. (1992) 89:1577–81. doi: 10.1073/pnas.89.5.1577
208. Zhong J, Zuo Y, Ma J, Fogo AB, Jolicoeur P, Ichikawa I, et al. Expression of HIV-1 genes in podocytes alone can lead to the full spectrum of HIV-1-associated nephropathy. Kidney Int. (2005) 68:1048–60. doi: 10.1111/j.1523-1755.2005.00497.x
209. Lotz M, Clark-Lewis I, Ganu V. HIV-1 transactivator protein Tat induces proliferation and TGF beta expression in human articular chondrocytes. J Cell Biol. (1994) 124:365–71. doi: 10.1083/jcb.124.3.365
210. Stettner MR, Nance JA, Wright CA, Kinoshita Y, Kim WK, Morgello S, et al. SMAD proteins of oligodendroglial cells regulate transcription of JC virus early and late genes coordinately with the Tat protein of human immunodeficiency virus type 1. J Gen Virol. (2009) 90:2005–14. doi: 10.1099/vir.0.011072-0
211. Ratnam KK, He CJ, Klotman P. Nef as a proliferative factor for kidney epithelial cells in HIV-associated nephropathy. Clin Med Oncol. (2008) 2:539–45. doi: 10.4137/CMO.S661
212. Husain M, D’Agati VD, He JC, Klotman ME, Klotman PE. HIV-1 Nef induces dedifferentiation of podocytes in vivo: a characteristic feature of HIVAN. AIDS. (2005) 19:1975–80. doi: 10.1097/01.aids.0000191918.42110.27
213. Farrow N, Cmielewski P, Donnelley M, Rout-Pitt N, Moodley Y, Bertoncello I, et al. Epithelial disruption: a new paradigm enabling human airway stem cell transplantation. Stem Cell Res Ther. (2018) 9:153. doi: 10.1186/s13287-018-0911-4
214. Rout-Pitt N, Farrow N, Parsons D, Donnelley M. Epithelial mesenchymal transition (EMT): a universal process in lung diseases with implications for cystic fibrosis pathophysiology. Respir Res. (2018) 19:136. doi: 10.1186/s12931-018-0834-8
215. Lotz M, Clark-Lewis I, Ganu V. HIV-1 transactivator protein Tat induces proliferation and TGF beta expression in human articular chondrocytes. J Cell Biol. (1994) 124:365–71. doi: 10.1083/jcb.124.3.365
216. Jiang Y, Chai L, Wang H, Shen X, Fasae MB, Jiao J, et al. HIV tat protein induces myocardial fibrosis through TGF-beta1-CTGF signaling cascade: A potential mechanism of HIV infection-related cardiac manifestations. Cardiovasc Toxicol. (2021) 21:965–72. doi: 10.1007/s12012-021-09687-6
217. Bettaccini AA, Baj A, Accolla RS, Basolo F, Toniolo AQ. Proliferative activity of extracellular HIV-1 Tat protein in human epithelial cells: expression profile of pathogenetically relevant genes. BMC Microbiol. (2005) 5:20. doi: 10.1186/1471-2180-5-20
218. Yamamoto T, Noble NA, Miller DE, Gold LI, Hishida A, Nagase M, et al. Increased levels of transforming growth factor-beta in HIV-associated nephropathy. Kidney Int. (1999) 55:579–92. doi: 10.1046/j.1523-1755.1999.00296.x
219. Pim D, Banks L. Interaction of viral oncoproteins with cellular target molecules: infection with high-risk vs low-risk human papillomaviruses. APMIS. (2010) 118:471–93. doi: 10.1111/j.1600-0463.2010.02618.x
220. Shiau MY, Fan LC, Yang SC, Tsao CH, Lee H, Cheng YW, et al. Human papillomavirus up-regulates MMP-2 and MMP-9 expression and activity by inducing interleukin-8 in lung adenocarcinomas. PloS One. (2013) 8:e54423. doi: 10.1371/journal.pone.0054423
221. Smola-Hess S, Pahne J, Mauch C, Zigrino P, Smola H, Pfister HJ. Expression of membrane type 1 matrix metalloproteinase in papillomavirus-positive cells: role of the human papillomavirus (HPV) 16 and HPV8 E7 gene products. J Gen virol. (2005) 86:1291–6. doi: 10.1099/vir.0.80551-0
222. Cardeal LB, Boccardo E, Termini L, Rabachini T, Andreoli MA, di Loreto C, et al. HPV16 oncoproteins induce MMPs/RECK-TIMP-2 imbalance in primary keratinocytes: possible implications in cervical carcinogenesis. PloS One. (2012) 7:e33585. doi: 10.1371/journal.pone.0033585
223. Noe V, Fingleton B, Jacobs K, Crawford HC, Vermeulen S, Steelant W, et al. Release of an invasion promoter E-cadherin fragment by matrilysin and stromelysin-1. J Cell Sci. (2001) 114:111–8. doi: 10.1242/jcs.114.1.111
224. Symowicz J, Adley BP, Gleason KJ, Johnson JJ, Ghosh S, Fishman DA, et al. Engagement of collagen-binding integrins promotes matrix metalloproteinase-9-dependent E-cadherin ectodomain shedding in ovarian carcinoma cells. Cancer Res. (2007) 67:2030–9. doi: 10.1158/0008-5472.CAN-06-2808
225. You TK, Kim KM, Noh SJ, Bae JS, Jang KY, Chung MJ, et al. Expressions of E-cadherin, cortactin and MMP-9 in pseudoepitheliomatous hyperplasia and squamous cell carcinoma of the head and neck: their relationships with clinicopathologic factors and prognostic implication. Korean J Pathol. (2012) 46:331–40. doi: 10.4132/KoreanJPathol.2012.46.4.331
226. David JM, Rajasekaran AK. Dishonorable discharge: the oncogenic roles of cleaved E-cadherin fragments. Cancer Res. (2012) 72:2917–23. doi: 10.1158/0008-5472.CAN-11-3498
227. Tobian AA, Grabowski MK, Serwadda D, Newell K, Ssebbowa P, Franco V, et al. Reactivation of herpes simplex virus type 2 after initiation of antiretroviral therapy. J Infect Dis. (2013) 208:839–46. doi: 10.1093/infdis/jit252
228. Lomeli-Martinez SM, Gonzalez-Hernandez LA, Ruiz-Anaya AJ, Lomeli-Martinez MA, Martinez-Salazar SY, Mercado Gonzalez AE, et al. Oral manifestations associated with HIV/AIDS patients. Medicina (Kaunas). (2022) 58. doi: 10.3390/medicina58091214
229. Wald A, Ericsson M, Krantz E, Selke S, Corey L. Oral shedding of herpes simplex virus type 2. Sex Transm Infect. (2004) 80:272–6. doi: 10.1136/sti.2003.007823
230. Posavad CM, Wald A, Kuntz S, Huang ML, Selke S, Krantz E, et al. Frequent reactivation of herpes simplex virus among HIV-1-infected patients treated with highly active antiretroviral therapy. J Infect Dis. (2004) 190:693–6. doi: 10.1086/jid.2004.190.issue-4
231. Tan DH, Raboud JM, Kaul R, Walmsley SL. Antiretroviral therapy is not associated with reduced herpes simplex virus shedding in HIV coinfected adults: an observational cohort study. BMJ Open. (2014) 4:e004210. doi: 10.1136/bmjopen-2013-004210
232. Krummenacher C, Nicola AV, Whitbeck JC, Lou H, Hou W, Lambris JD, et al. Herpes simplex virus glycoprotein D can bind to poliovirus receptor-related protein 1 or herpesvirus entry mediator, two structurally unrelated mediators of virus entry. J Virol. (1998) 72:7064–74. doi: 10.1128/JVI.72.9.7064-7074.1998
233. Yoon M, Spear PG. Disruption of adherens junctions liberates nectin-1 to serve as receptor for herpes simplex virus and pseudorabies virus entry. J Virol. (2002) 76:7203–8. doi: 10.1128/JVI.76.14.7203-7208.2002
234. Grinde B. Herpesviruses: latency and reactivation - viral strategies and host response. J Oral Microbiol. (2013) 5. doi: 10.3402/jom.v5i0.22766
235. Drew WL. Cytomegalovirus infection in patients with AIDS. Clin Infect Dis. (1992) 14:608–15. doi: 10.1093/clinids/14.2.608-a
236. Kovacs A, Schluchter M, Easley K, Demmler G, Shearer W, La Russa P, et al. Cytomegalovirus infection and HIV-1 disease progression in infants born to HIV-1-infected women. Pediatric Pulmonary and Cardiovascular Complications of Vertically Transmitted HIV Infection Study Group. N Engl J Med. (1999) 341:77–84. doi: 10.1056/NEJM199907083410203
237. Nigro G, Krzysztofiak A, Gattinara GC, Mango T, Mazzocco M, Porcaro MA, et al. Rapid progression of HIV disease in children with cytomegalovirus DNAemia. AIDS. (1996) 10:1127–33.
238. Chandwani S, Kaul A, Bebenroth D, Kim M, John DD, Fidelia A, et al. Cytomegalovirus infection in human immunodeficiency virus type 1-infected children. Pediatr Infect Dis J. (1996) 15:310–4. doi: 10.1097/00006454-199604000-00006
239. Gie RP, Goussard P. CMV pneumonia in HIV-infected and HIV-uninfected infants: a neglected disease? Int J Tuberc Lung Dis. (2017) 21:1209–10. doi: 10.5588/ijtld.17.0714
240. Gianella S, Letendre S. Cytomegalovirus and HIV: A dangerous pas de deux. J Infect Dis. (2016) 214 Suppl 2:S67–74. doi: 10.1093/infdis/jiw217
241. Griffiths P, Baraniak I, Reeves M. The pathogenesis of human cytomegalovirus. J Pathol. (2015) 235:288–97. doi: 10.1002/path.2015.235.issue-2
242. Sufiawati I, Herrera R, Mayer W, Cai X, Borkakoti J, Lin V, et al. Human immunodeficiency virus (HIV) and human cytomegalovirus (HCMV) coinfection of infant tonsil epithelium may synergistically promote both HIV-1 and HCMV spread and infection. J Virol. (2021) 95:e0092121. doi: 10.1128/JVI.00921-21
243. DeMeritt IB, Milford LE, Yurochko AD. Activation of the NF-kappaB pathway in human cytomegalovirus-infected cells is necessary for efficient transactivation of the major immediate-early promoter. J Virol. (2004) 78:4498–507. doi: 10.1128/JVI.78.9.4498-4507.2004
244. Chan G, Bivins-Smith ER, Smith MS, Yurochko AD. Transcriptome analysis of NF-kappaB- and phosphatidylinositol 3-kinase-regulated genes in human cytomegalovirus-infected monocytes. J Virol. (2008) 82:1040–6. doi: 10.1128/JVI.00864-07
245. Yurochko AD, Kowalik TF, Huong SM, Huang ES. Human cytomegalovirus upregulates NF-kappa B activity by transactivating the NF-kappa B p105/p50 and p65 promoters. J Virol. (1995) 69:5391–400. doi: 10.1128/jvi.69.9.5391-5400.1995
246. Johnson RA, Ma XL, Yurochko AD, Huang ES. The role of MKK1/2 kinase activity in human cytomegalovirus infection. J Gen Virol. (2001) 82:493–7. doi: 10.1099/0022-1317-82-3-493
247. Min CK, Shakya AK, Lee BJ, Streblow DN, Caposio P, Yurochko AD. The differentiation of human cytomegalovirus infected-monocytes is required for viral replication. Front Cell Infect Microbiol. (2020) 10:368. doi: 10.3389/fcimb.2020.00368
248. Rodgers BC, Scott DM, Mundin J, Sissons JG. Monocyte-derived inhibitor of interleukin 1 induced by human cytomegalovirus. J Virol. (1985) 55:527–32. doi: 10.1128/jvi.55.3.527-532.1985
249. Rodgers BC, Scott DM, Sissons JG. A monocyte-derived inhibitor of interleukin 1 induced by human cytomegalovirus. Trans Assoc Am Phys. (1985) 98:334–43. doi: 10.1128/jvi.55.3.527-532.1985
250. Scott DM, Rodgers BC, Freeke C, Buiter J, Sissons JG. Human cytomegalovirus and monocytes: limited infection and negligible immunosuppression in normal mononuclear cells infected in vitro with mycoplasma-free virus strains. J Gen Virol. (1989) 70:685–94. doi: 10.1099/0022-1317-70-3-685
251. Taylor-Wiedeman J, Sissons JG, Borysiewicz LK, Sinclair JH. Monocytes are a major site of persistence of human cytomegalovirus in peripheral blood mononuclear cells. J Gen Virol. (1991) 72:2059–64. doi: 10.1099/0022-1317-72-9-2059
252. Zheng A, Yuan F, Li Y, Zhu F, Hou P, Li J, et al. Claudin-6 and claudin-9 function as additional coreceptors for hepatitis C virus. J Virol. (2007) 81:12465–71. doi: 10.1128/JVI.01457-07
253. Ploss A, Evans MJ, Gaysinskaya VA, Panis M, You H, de Jong YP, et al. Human occludin is a hepatitis C virus entry factor required for infection of mouse cells. Nature. (2009) 457:882–6. doi: 10.1038/nature07684
254. Mailly L, Baumert TF. Hepatitis C virus infection and tight junction proteins: The ties that bind. Biochim Biophys Acta Biomembr. (2020) 1862:183296. doi: 10.1016/j.bbamem.2020.183296
255. So CW, Sourisseau M, Sarwar S, Evans MJ, Randall G. Roles of epidermal growth factor receptor, claudin-1 and occludin in multi-step entry of hepatitis C virus into polarized hepatoma spheroids. PloS Pathog. (2023) 19:e1011887. doi: 10.1371/journal.ppat.1011887
256. Barton ES, Forrest JC, Connolly JL, Chappell JD, Liu Y, Schnell FJ, et al. Junction adhesion molecule is a receptor for reovirus. Cell. (2001) 104:441–51. doi: 10.1016/S0092-8674(01)00231-8
257. Torres-Flores JM, Silva-Ayala D, Espinoza MA, Lopez S, Arias CF. The tight junction protein JAM-A functions as coreceptor for rotavirus entry into MA104 cells. Virology. (2015) 475:172–8. doi: 10.1016/j.virol.2014.11.016
258. Coyne CB, Shen L, Turner JR, Bergelson JM. Coxsackievirus entry across epithelial tight junctions requires occludin and the small GTPases Rab34 and Rab5. Cell Host Microbe. (2007) 2:181–92. doi: 10.1016/j.chom.2007.07.003
259. Chang Y, Cesarman E, Pessin MS, Lee F, Culpepper J, Knowles DM, et al. Identification of herpesvirus-like DNA sequences in AIDS-associated Kaposi’s sarcoma. Science. (1994) 266:1865–9. doi: 10.1126/science.7997879
260. Sternbach G, Varon J. Moritz Kaposi: idiopathic pigmented sarcoma of the skin. J Emerg Med. (1995) 13:671–4. doi: 10.1016/0736-4679(95)00077-N
261. Ensoli B, Barillari G, Buonaguro L, Gallo RC. Molecular mechanisms in the pathogenesis of AIDS-associated Kaposi’s sarcoma. Adv Exp Med Biol. (1991) 303:27–38. doi: 10.1007/978-1-4684-6000-1_4
262. Ensoli B, Barillari G, Gallo RC. Pathogenesis of AIDS-associated kaposi’s sarcoma. Hematol Oncol Clin North Am. (1991) 5:281–95. doi: 10.1016/S0889-8588(18)30441-6
263. Ensoli B, Gendelman R, Markham P, Fiorelli V, Colombini S, Raffeld M, et al. Synergy between basic fibroblast growth factor and HIV-1 Tat protein in induction of Kaposi’s sarcoma. Nature. (1994) 371:674–80. doi: 10.1038/371674a0
264. Ensoli B, Sturzl M, Monini P. Reactivation and role of HHV-8 in Kaposi’s sarcoma initiation. Adv Cancer Res. (2001) 81:161–200. doi: 10.1016/S0065-230X(01)81005-8
265. Albini A, Barillari G, Benelli R, Gallo RC, Ensoli B. Angiogenic properties of human immunodeficiency virus type 1 Tat protein. Proc Natl Acad Sci U S A. (1995) 92:4838–42. doi: 10.1073/pnas.92.11.4838
266. Albini A, Benelli R, Presta M, Rusnati M, Ziche M, Rubartelli A, et al. HIV-tat protein is a heparin-binding angiogenic growth factor. Oncogene. (1996) 12:289–97.
267. Albini A, Fontanini G, Masiello L, Tacchetti C, Bigini D, Luzzi P, et al. Angiogenic potential in vivo by Kaposi’s sarcoma cell-free supernatants and HIV-1 tat product: inhibition of KS-like lesions by tissue inhibitor of metalloproteinase-2. AIDS. (1994) 8:1237–44. doi: 10.1097/00002030-199409000-00004
268. Albini A, Soldi R, Giunciuglio D, Giraudo E, Benelli R, Primo L, et al. The angiogenesis induced by HIV-1 tat protein is mediated by the Flk-1/KDR receptor on vascular endothelial cells. Nat Med. (1996) 2:1371–5. doi: 10.1038/nm1296-1371
269. Corallini A, Campioni D, Rossi C, Albini A, Possati L, Rusnati M, et al. Promotion of tumour metastases and induction of angiogenesis by native HIV-1 Tat protein from BK virus/tat transgenic mice. AIDS. (1996) 10:701–10. doi: 10.1097/00002030-199606001-00003
270. Vogel J, Hinrichs SH, Reynolds RK, Luciw PA, Jay G. The HIV tat gene induces dermal lesions resembling Kaposi’s sarcoma in transgenic mice. Nature. (1988) 335:606–11. doi: 10.1038/335606a0
271. Zhou F, Xue M, Qin D, Zhu X, Wang C, Zhu J, et al. HIV-1 Tat promotes Kaposi’s sarcoma-associated herpesvirus (KSHV) vIL-6-induced angiogenesis and tumorigenesis by regulating PI3K/PTEN/AKT/GSK-3beta signaling pathway. PloS One. (2013) 8:e53145. doi: 10.1371/journal.pone.0053145
272. Aoki Y, Tosato G. HIV-1 Tat enhances Kaposi sarcoma-associated herpesvirus (KSHV) infectivity. Blood. (2004) 104:810–4. doi: 10.1182/blood-2003-07-2533
273. Yen-Moore A, Hudnall SD, Rady PL, Wagner RF Jr., Moore TO, Memar O, et al. Differential expression of the HHV-8 vGCR cellular homolog gene in AIDS-associated and classic Kaposi’s sarcoma: potential role of HIV-1 Tat. Virology. (2000) 267:247–51. doi: 10.1006/viro.1999.0125
274. Pati S, Foulke JS Jr., Barabitskaya O, Kim J, Nair BC, Hone D, et al. Human herpesvirus 8-encoded vGPCR activates nuclear factor of activated T cells and collaborates with human immunodeficiency virus type 1 Tat. J Virol. (2003) 77:5759–73. doi: 10.1128/JVI.77.10.5759-5773.2003
275. Guo HG, Pati S, Sadowska M, Charurat M, Reitz M. Tumorigenesis by human herpesvirus 8 vGPCR is accelerated by human immunodeficiency virus type 1 Tat. J Virol. (2004) 78:9336–42. doi: 10.1128/JVI.78.17.9336-9342.2004
276. Yao S, Hu M, Hao T, Li W, Xue X, Xue M, et al. MiRNA-891a-5p mediates HIV-1 Tat and KSHV Orf-K1 synergistic induction of angiogenesis by activating NF-kappaB signaling. Nucleic Acids Res. (2015) 43:9362–78. doi: 10.1093/nar/gkv988
277. Chen X, Cheng L, Jia X, Zeng Y, Yao S, Lv Z, et al. Human immunodeficiency virus type 1 Tat accelerates Kaposi sarcoma-associated herpesvirus Kaposin A-mediated tumorigenesis of transformed fibroblasts in vitro as well as in nude and immunocompetent mice. Neoplasia. (2009) 11:1272–84. doi: 10.1593/neo.09494
278. Beral V, Peterman TA, Berkelman RL, Jaffe HW. Kaposi’s sarcoma among persons with AIDS: a sexually transmitted infection? Lancet. (1990) 335:123–8. doi: 10.1016/0140-6736(90)90001-L
279. Gallo RC. The enigmas of Kaposi’s sarcoma. Science. (1998) 282:1837–9. doi: 10.1126/science.282.5395.1837
280. La Ferla L, Pinzone MR, Nunnari G, Martellotta F, Lleshi A, Tirelli U, et al. Kaposi’ s sarcoma in HIV-positive patients: the state of art in the HAART-era. Eur Rev Med Pharmacol Sci. (2013) 17:2354–65.
281. Robey RC, Bower M. Facing up to the ongoing challenge of Kaposi’s sarcoma. Curr Opin Infect Dis. (2015) 28:31–40. doi: 10.1097/QCO.0000000000000122
282. Silverberg MJ, Chao C, Leyden WA, Xu L, Horberg MA, Klein D, et al. HIV infection, immunodeficiency, viral replication, and the risk of cancer. Cancer Epidemiol Biomarkers Prev. (2011) 20:2551–9. doi: 10.1158/1055-9965.EPI-11-0777
283. Poizot-Martin I, Lions C, Cheret A, Rey D, Duvivier C, Jacomet C, et al. Kaposi sarcoma in people living with HIV: incidence and associated factors in a French cohort between 2010 and 2015. AIDS. (2020) 34:569–77. doi: 10.1097/QAD.0000000000002450
284. Poizot-Martin I, Obry-Roguet V, Duvivier C, Lions C, Huleux T, Jacomet C, et al. Kaposi sarcoma among people living with HIV in the French DAT’AIDS cohort between 2010 and 2015. J Eur Acad Dermatol Venereol. (2020) 34:1065–73. doi: 10.1111/jdv.16204
285. Palich R, Veyri M, Valantin MA, Marcelin AG, Guihot A, Pourcher V, et al. Recurrence and occurrence of kaposi’s sarcoma in patients living with human immunodeficiency virus (HIV) and on antiretroviral therapy, despite suppressed HIV viremia. Clin Infect Dis. (2020) 70:2435–8. doi: 10.1093/cid/ciz762
286. Royston L, Isnard S, Calmy A, Routy JP. Kaposi sarcoma in antiretroviral therapy-treated people with HIV: a wake-up call for research on human herpesvirus-8. AIDS. (2021) 35:1695–9. doi: 10.1097/QAD.0000000000002933
287. Mishra R, Singh SK. HIV-1 Tat C modulates expression of miRNA-101 to suppress VE-cadherin in human brain microvascular endothelial cells. J Neurosci. (2013) 33:5992–6000. doi: 10.1523/JNEUROSCI.4796-12.2013
288. Mishra R, Singh SK. HIV-1 Tat C phosphorylates VE-cadherin complex and increases human brain microvascular endothelial cell permeability. BMC Neurosci. (2014) 15:80. doi: 10.1186/1471-2202-15-80
289. Kim TA, Avraham HK, Koh YH, Jiang S, Park IW, Avraham S. HIV-1 Tat-mediated apoptosis in human brain microvascular endothelial cells. J Immunol. (2003) 170:2629–37. doi: 10.4049/jimmunol.170.5.2629
290. Zhong Y, Smart EJ, Weksler B, Couraud PO, Hennig B, Toborek M. Caveolin-1 regulates human immunodeficiency virus-1 Tat-induced alterations of tight junction protein expression via modulation of the Ras signaling. J Neurosci. (2008) 28:7788–96. doi: 10.1523/JNEUROSCI.0061-08.2008
291. Zhong Y, Zhang B, Eum SY, Toborek M. HIV-1 Tat triggers nuclear localization of ZO-1 via Rho signaling and cAMP response element-binding protein activation. J Neurosci. (2012) 32:143–50. doi: 10.1523/JNEUROSCI.4266-11.2012
292. Zhou F, Liu X, Gao L, Zhou X, Cao Q, Niu L, et al. HIV-1 Tat enhances purinergic P2Y4 receptor signaling to mediate inflammatory cytokine production and neuronal damage via PI3K/Akt and ERK MAPK pathways. J Neuroinflamm. (2019) 16:71. doi: 10.1186/s12974-019-1466-8
293. Bayer C, Varani S, Wang L, Walther P, Zhou S, Straschewski S, et al. Human cytomegalovirus infection of M1 and M2 macrophages triggers inflammation and autologous T-cell proliferation. J Virol. (2013) 87:67–79. doi: 10.1128/JVI.01585-12
294. Xu R, Feng X, Xie X, Zhang J, Wu D, Xu L. HIV-1 Tat protein increases the permeability of brain endothelial cells by both inhibiting occludin expression and cleaving occludin via matrix metalloproteinase-9. Brain Res. (2012) 1436:13–9. doi: 10.1016/j.brainres.2011.11.052
295. Nottet HS. Interactions between macrophages and brain microvascular endothelial cells: role in pathogenesis of HIV-1 infection and blood - brain barrier function. J Neurovirol. (1999) 5:659–69. doi: 10.3109/13550289909021294
296. Andras IE, Pu H, Deli MA, Nath A, Hennig B, Toborek M. HIV-1 Tat protein alters tight junction protein expression and distribution in cultured brain endothelial cells. J Neurosci Res. (2003) 74:255–65. doi: 10.1002/jnr.10762
297. Mahajan SD, Aalinkeel R, Sykes DE, Reynolds JL, Bindukumar B, Fernandez SF, et al. Tight junction regulation by morphine and HIV-1 tat modulates blood-brain barrier permeability. J Clin Immunol. (2008) 28:528–41. doi: 10.1007/s10875-008-9208-1
298. Pu H, Tian J, Andras IE, Hayashi K, Flora G, Hennig B, et al. HIV-1 Tat protein-induced alterations of ZO-1 expression are mediated by redox-regulated ERK 1/2 activation. J Cereb Blood Flow Metab. (2005) 25:1325–35. doi: 10.1038/sj.jcbfm.9600125
299. Claesson-Welsh L, Dejana E, McDonald DM. Permeability of the endothelial barrier: identifying and reconciling controversies. Trends Mol Med. (2021) 27:314–31. doi: 10.1016/j.molmed.2020.11.006
300. Leibrand CR, Paris JJ, Ghandour MS, Knapp PE, Kim WK, Hauser KF, et al. HIV-1 Tat disrupts blood-brain barrier integrity and increases phagocytic perivascular macrophages and microglia in the dorsal striatum of transgenic mice. Neurosci Lett. (2017) 640:136–43. doi: 10.1016/j.neulet.2016.12.073
301. Jadhav S, Nema V. HIV-associated neurotoxicity: the interplay of host and viral proteins. Mediators Inflamm. (2021) 2021:1267041. doi: 10.1155/2021/1267041
302. Debalkie Animut M, Sorrie MB, Birhanu YW, Teshale MY. High prevalence of neurocognitive disorders observed among adult people living with HIV/AIDS in Southern Ethiopia: A cross-sectional study. PloS One. (2019) 14:e0204636. doi: 10.1371/journal.pone.0204636
303. Sun Y, Cai M, Liang Y, Zhang Y. Disruption of blood-brain barrier: effects of HIV Tat on brain microvascular endothelial cells and tight junction proteins. J Neurovirol. (2023) 29:658–68. doi: 10.1007/s13365-023-01179-3
304. Ma R, Yang L, Niu F, Buch S. HIV tat-mediated induction of human brain microvascular endothelial cell apoptosis involves endoplasmic reticulum stress and mitochondrial dysfunction. Mol Neurobiol. (2016) 53:132–42. doi: 10.1007/s12035-014-8991-3
305. Chandra PK, Rutkai I, Kim H, Braun SE, Abdel-Mageed AB, Mondal D, et al. Latent HIV-exosomes induce mitochondrial hyperfusion due to loss of phosphorylated dynamin-related protein 1 in brain endothelium. Mol Neurobiol. (2021) 58:2974–89. doi: 10.1007/s12035-021-02319-8
306. Gasperini P, Espigol-Frigole G, McCormick PJ, Salvucci O, Maric D, Uldrick TS, et al. Kaposi sarcoma herpesvirus promotes endothelial-to-mesenchymal transition through Notch-dependent signaling. Cancer Res. (2012) 72:1157–69. doi: 10.1158/0008-5472.CAN-11-3067
307. Ding Y, Chen W, Lu Z, Wang Y, Yuan Y. Kaposi’s sarcoma-associated herpesvirus promotes mesenchymal-to-endothelial transition by resolving the bivalent chromatin of PROX1 gene. PloS Pathog. (2021) 17:e1009847. doi: 10.1371/journal.ppat.1009847
308. Xu-Dubois YC, Peltier J, Brocheriou I, Suberbielle-Boissel C, Djamali A, Reese S, et al. Markers of endothelial-to-mesenchymal transition: evidence for antibody-endothelium interaction during antibody-mediated rejection in kidney recipients. J Am Soc Nephrol. (2016) 27:324–32. doi: 10.1681/ASN.2014070679
309. Lacunza E, Ahuja A, Coso OA, Abba M, Ramos JC, Cesarman E, et al. Unveiling the role of KSHV-infected human mesenchymal stem cells in Kaposi’s sarcoma initiation. J Med Virol. (2024) 96:e29684. doi: 10.1002/jmv.29684
310. Piera-Velazquez S, Jimenez SA. Endothelial to mesenchymal transition: role in physiology and in the pathogenesis of human diseases. Physiol Rev. (2019) 99:1281–324. doi: 10.1152/physrev.00021.2018
311. Piera-Velazquez S, Mendoza FA, Jimenez SA. Endothelial to mesenchymal transition (EndoMT) in the pathogenesis of human fibrotic diseases. J Clin Med. (2016) 5. doi: 10.3390/jcm5040045
312. Wermuth PJ, Carney KR, Mendoza FA, Piera-Velazquez S, Jimenez SA. Endothelial cell-specific activation of transforming growth factor-beta signaling in mice induces cutaneous, visceral, and microvascular fibrosis. Lab Invest. (2017) 97:806–18. doi: 10.1038/labinvest.2017.23
313. Wermuth PJ, Li Z, Mendoza FA, Jimenez SA. Stimulation of transforming growth factor-beta1-induced endothelial-to-mesenchymal transition and tissue fibrosis by endothelin-1 (ET-1): A novel profibrotic effect of ET-1. PloS One. (2016) 11:e0161988. doi: 10.1371/journal.pone.0161988
Keywords: human immunodeficiency virus, Tat, mucosal epithelium, disruption of epithelial junctions, epithelial-mesenchymal transition
Citation: Tugizov S (2025) HIV-1 Tat-induced disruption of epithelial junctions and epithelial-mesenchymal transition of oral and genital epithelial cells lead to increased invasiveness of neoplastic cells and the spread of herpes simplex virus and cytomegalovirus. Front. Immunol. 16:1541532. doi: 10.3389/fimmu.2025.1541532
Received: 08 December 2024; Accepted: 28 January 2025;
Published: 13 February 2025.
Edited by:
Aurelio Cafaro, National Institute of Health (ISS), ItalyReviewed by:
Guochun Jiang, University of North Carolina at Chapel Hill, United StatesCopyright © 2025 Tugizov. This is an open-access article distributed under the terms of the Creative Commons Attribution License (CC BY). The use, distribution or reproduction in other forums is permitted, provided the original author(s) and the copyright owner(s) are credited and that the original publication in this journal is cited, in accordance with accepted academic practice. No use, distribution or reproduction is permitted which does not comply with these terms.
*Correspondence: Sharof Tugizov, c2hhcm9mLnR1Z2l6b3ZAdWNzZi5lZHU=
Disclaimer: All claims expressed in this article are solely those of the authors and do not necessarily represent those of their affiliated organizations, or those of the publisher, the editors and the reviewers. Any product that may be evaluated in this article or claim that may be made by its manufacturer is not guaranteed or endorsed by the publisher.
Research integrity at Frontiers
Learn more about the work of our research integrity team to safeguard the quality of each article we publish.