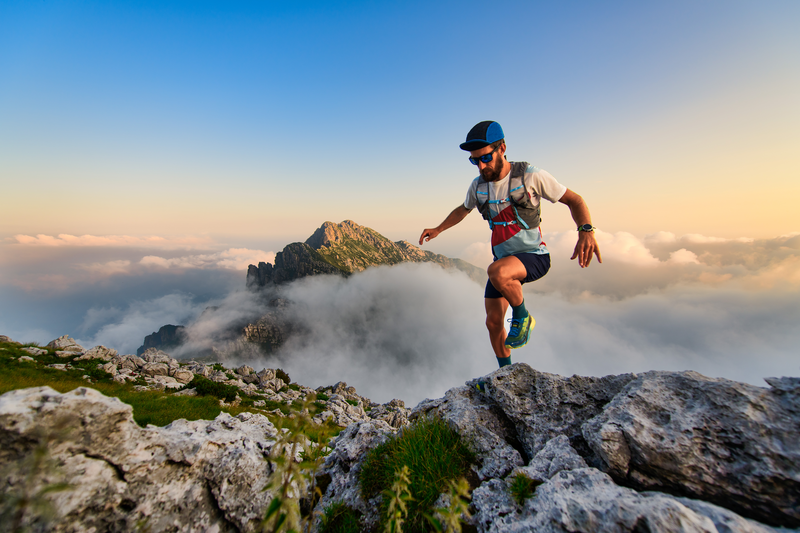
94% of researchers rate our articles as excellent or good
Learn more about the work of our research integrity team to safeguard the quality of each article we publish.
Find out more
SYSTEMATIC REVIEW article
Front. Immunol.
Sec. Cancer Immunity and Immunotherapy
Volume 16 - 2025 | doi: 10.3389/fimmu.2025.1538969
The final, formatted version of the article will be published soon.
You have multiple emails registered with Frontiers:
Please enter your email address:
If you already have an account, please login
You don't have a Frontiers account ? You can register here
Background: Probiotics have been demonstrated to exert a potential clinical enhancing effect in cancer patients receiving immune checkpoint inhibitors (ICIs), while antibiotics exert a detrimental impact. Prior meta-analysis papers have substantial limitations and are devoid of recent published studies. Therefore, this study aimed to perform an updated meta-analysis and, for the first time, assess whether probiotics can restore the damage of antibiotics to immunotherapy. Methods: A comprehensive literature search was conducted in three English databases and three Chinese databases with a cutoff date of August 11, 2024. The methodological quality of the studies was evaluated using the Newcastle-Ottawa Quality Assessment Scale (NOS) or the Revised Cochrane risk-of-bias tool (RoB 2). Engauge Digitizer v12.1 was employed to extract hazard ratios (HRs) with 95% confidence interval (CI) for survival outcomes when these data were not explicitly provided in the manuscripts. Meta-analysis was conducted using Stata 14 software. Results: The study sample comprised eight retrospective and four prospective studies, involving a total of 3,142 participants. The findings indicate that probiotics significantly prolong the overall survival (OS) (I 2 =31.2%; HR=0.58, 95% CI: 0.46-0.73, p < 0.001) and progression-free survival (PFS) (I 2 =65.2%; HR=0.66, 95% CI: 0.54-0.81, p < 0.001) in cancer patients receiving ICIs, enhance the objective response rate (ORR) (I 2 = 33.5%; OR=1.75, 95% CI: 1.27-2.40, p = 0.001) and disease control rate (DCR) (I 2 = 50.0%; OR=1.93, 95% CI: 1.11-3.35, p = 0.002). For non-small cell lung cancer (NSCLC) patients exposed to antibiotics, the use of probiotics was associated with superior OS (I 2 =0.0%; HR=0.45, 95% CI: 0.34-0.59, p < 0.001) and PFS (I 2 =0.0%; HR=0.48, 95% CI: 0.38-0.62, p < 0.001) when compared to non-users. Subgroup differences were observed regarding the cancer type (P=0.006) and ethnic backgrounds (P=0.011) in OS. Conclusions: The meta-analysis findings suggest that probiotics can effectively extend the survival of cancer treated with ICIs. In NSCLC, probiotics appear to mitigate the negative impact of antibiotics on immunotherapy effectiveness, which has profound clinical significance. Nevertheless, additional large-scale, high-quality randomized controlled trials are necessary to further validate these findings.
Keywords: Cancer, Immunotherapy, Probiotics, antibiotics, Meta-analysis, Systematic review
Received: 03 Dec 2024; Accepted: 28 Feb 2025.
Copyright: © 2025 Zhao, Zhao, Lu, Tang, Zhang and Feng. This is an open-access article distributed under the terms of the Creative Commons Attribution License (CC BY). The use, distribution or reproduction in other forums is permitted, provided the original author(s) or licensor are credited and that the original publication in this journal is cited, in accordance with accepted academic practice. No use, distribution or reproduction is permitted which does not comply with these terms.
* Correspondence:
Lishan Zhang, Dongzhimen Hospital, Beijing University of Chinese Medicine, Beijing, China
Cuiling Feng, Dongzhimen Hospital, Beijing University of Chinese Medicine, Beijing, China
Disclaimer: All claims expressed in this article are solely those of the authors and do not necessarily represent those of their affiliated organizations, or those of the publisher, the editors and the reviewers. Any product that may be evaluated in this article or claim that may be made by its manufacturer is not guaranteed or endorsed by the publisher.
Research integrity at Frontiers
Learn more about the work of our research integrity team to safeguard the quality of each article we publish.