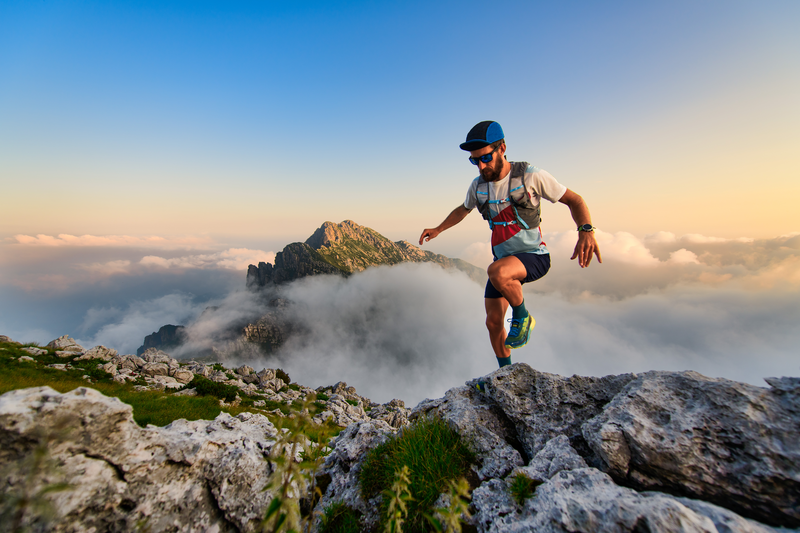
94% of researchers rate our articles as excellent or good
Learn more about the work of our research integrity team to safeguard the quality of each article we publish.
Find out more
SYSTEMATIC REVIEW article
Front. Immunol.
Sec. Cancer Immunity and Immunotherapy
Volume 16 - 2025 | doi: 10.3389/fimmu.2025.1537926
The final, formatted version of the article will be published soon.
You have multiple emails registered with Frontiers:
Please enter your email address:
If you already have an account, please login
You don't have a Frontiers account ? You can register here
Background: The clinical application of immune checkpoint blockade (ICB)-based neoadjuvant therapy has been approved in breast cancer since 2021. However, no studies have evaluated its efficacy and safety in randomized and non-randomized settings. Additionally, there exists controversy about which specific subpopulation can benefit from this management strategy.Methods: We searched MEDLINE and EMBASE databases for prospective clinical trials of ICB-based neoadjuvant therapy in breast cancer. Informations regarding pathological complete response (pCR), event-free survival (EFS), overall survival (OS), and treatment-related adverse event (TRAE) were pooled to estimate the efficacy and safety. Hazard ratio, relative risk (RR) and their 95% confidence intervals (CIs) were calculated.Results: Among 22 eligible trials including 6134 women with resectable breast cancer, there were 11 randomized studies with 5574 patients. Pooled analysis on pCR (RR, 1.38; 95% CI, 1.20-1.58; P<0.001), EFS (hazard ratio, 0.67; 95% CI, 0.54-0.81; P<0.001), and OS (hazard ratio, 0.56; 95% CI, 0.35-0.91; P=0.01) revealed that ICB-based neoadjuvant therapy was associated with favorable outcomes over conventional treatment. Moreover, the benefits of EFS were independent of PD-L1 expression (Pinteraction=0.57) and pCR (Pinteraction=0.37) in neoadjuvant immunotherapy. However, combining ICB with conventional neoadjuvant treatment significantly increased the risk of high-grade TRAE (RR, 1.06; 95% CI, 1.01-1.12; P=0.03), serious TRAE (RR, 1.57; 95% CI, 1.26-1.94; P<0.001), treatment discontinuation due to TRAE (RR, 1.47; 95% CI, 1.14-1.90; P=0.003), and potentially fatal adverse event (RR, 2.25; 95% CI, 0.80-6.31; P=0.12).Conclusion: The combination of ICB with conventional neoadjuvant treatment is associated with favorable clinical outcomes and importantly, increased grade 3+ toxicities. Clinicians should meticulously monitor patients to minimize the risk of treatment discontinuation in individuals with potentially curable breast cancer.
Keywords: Neoadjuvant Therapy, Immune checkpoint blockade, breast cancer, Toxicity, Pathologic complete response, Event free survival
Received: 02 Dec 2024; Accepted: 27 Feb 2025.
Copyright: © 2025 Ye, Zhang, Zhao and Zhao. This is an open-access article distributed under the terms of the Creative Commons Attribution License (CC BY). The use, distribution or reproduction in other forums is permitted, provided the original author(s) or licensor are credited and that the original publication in this journal is cited, in accordance with accepted academic practice. No use, distribution or reproduction is permitted which does not comply with these terms.
* Correspondence:
Bin Zhao, Quanzhou First Hospital, Fujian Medical University, Quanzhou, 362000, Fujian Province, China
Disclaimer: All claims expressed in this article are solely those of the authors and do not necessarily represent those of their affiliated organizations, or those of the publisher, the editors and the reviewers. Any product that may be evaluated in this article or claim that may be made by its manufacturer is not guaranteed or endorsed by the publisher.
Research integrity at Frontiers
Learn more about the work of our research integrity team to safeguard the quality of each article we publish.