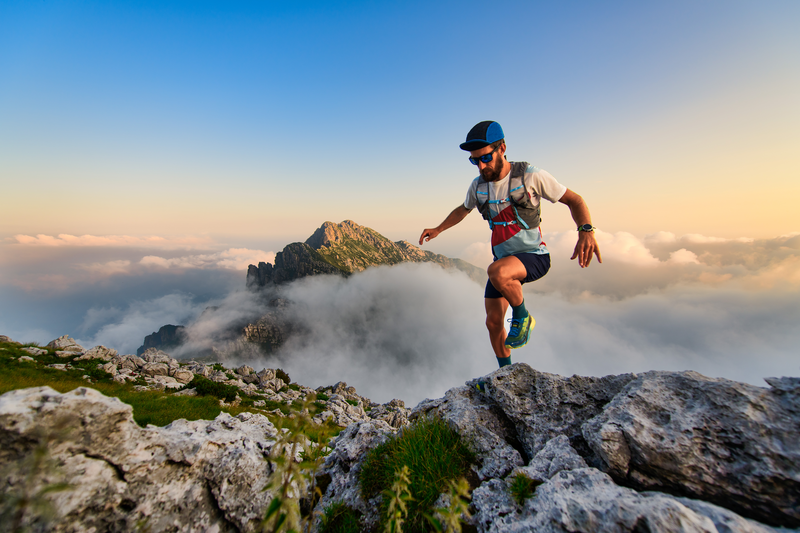
94% of researchers rate our articles as excellent or good
Learn more about the work of our research integrity team to safeguard the quality of each article we publish.
Find out more
REVIEW article
Front. Immunol.
Sec. Autoimmune and Autoinflammatory Disorders : Autoimmune Disorders
Volume 16 - 2025 | doi: 10.3389/fimmu.2025.1533955
This article is part of the Research Topic Biomarkers and Beyond: Predicting Course and Tailoring Treatment in Inflammatory Bowel Diseases View all articles
The final, formatted version of the article will be published soon.
You have multiple emails registered with Frontiers:
Please enter your email address:
If you already have an account, please login
You don't have a Frontiers account ? You can register here
Background: Inflammatory bowel disease (IBD) is an increasingly prevalent condition in developed countries. Alongside the growing number of patients, there is a rising incidence of disease-related complications, including osteoporosis. While well-established risk factors for low bone mineral density in IBD-such as low body mass or steroid therapy-are widely recognized, other contributing factors warrant further investigation. One such factor is visfatin, a proinflammatory adipokine encoded by the NAMPT gene. Objectives: This review aimed to explore the association between visfatin level, bone health and obesity among patients with inflammatory bowel disease. Key findings: Although visfatin is primarily associated with metabolic syndrome, it may also influence bone mineral density by affecting osteoblast and osteoclast differentiation and function. Additionally, some studies have identified a correlation between visfatin levels and bone mineral density. A deeper understanding of visfatin's role in osteoporosis development may contribute to the identification of novel therapeutic strategies. Therefore, lower bone mineral density in inflammatory bowel disease in inflammatory bowel disease may be associated with obesity and visfatin levels. However, visfatin concentrations depend on many factors, including genetics, immunology and nutritional factors, which may affect visfatin levels. Implications: Current research highlights visfatin as both a potential biomarker and a therapeutic target for osteoporosis treatment. Nevertheless, limited studies have specifically examined the relationship between visfatin and bone mineral density in IBD. Further research is required to clarify this association and to explore how variations in visfatin levels impact bone density in IBD patients.
Keywords: Obesity, osteopenia/osteoporosis, inflammatory bowel disease, bone mineral density, visfatin, Crohn disease, ulcerative colitis
Received: 25 Nov 2024; Accepted: 21 Feb 2025.
Copyright: © 2025 Ratajczak-Pawłowska, Szymczak-Tomczak, Hryhorowicz, Zawada, Skoracka, Rychter, Skrzypczak-Zielinska, Słomski, Dobrowolska and Krela-Kaźmierczak. This is an open-access article distributed under the terms of the Creative Commons Attribution License (CC BY). The use, distribution or reproduction in other forums is permitted, provided the original author(s) or licensor are credited and that the original publication in this journal is cited, in accordance with accepted academic practice. No use, distribution or reproduction is permitted which does not comply with these terms.
* Correspondence:
Alicja Ewa Ratajczak-Pawłowska, Department of Gastroenterology, Dietetics and Internal Diseases, Poznan University of Medical Sciences, Poznan, Poland
Disclaimer: All claims expressed in this article are solely those of the authors and do not necessarily represent those of their affiliated organizations, or those of the publisher, the editors and the reviewers. Any product that may be evaluated in this article or claim that may be made by its manufacturer is not guaranteed or endorsed by the publisher.
Research integrity at Frontiers
Learn more about the work of our research integrity team to safeguard the quality of each article we publish.