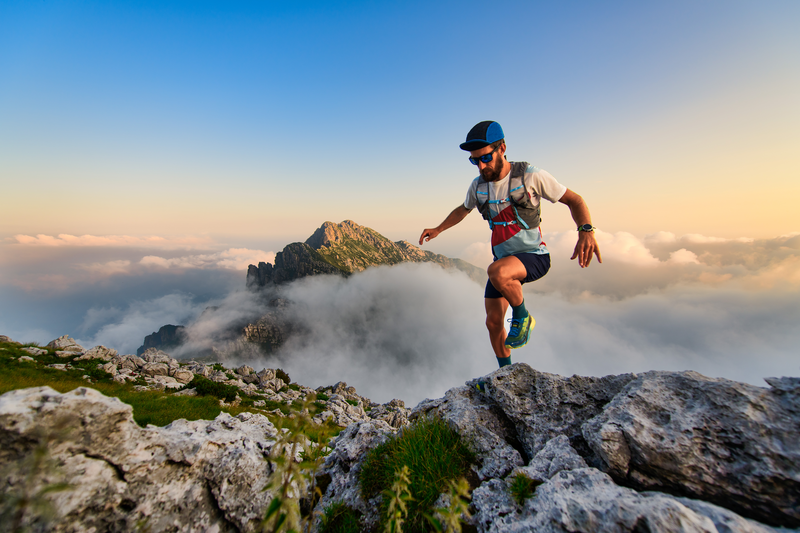
94% of researchers rate our articles as excellent or good
Learn more about the work of our research integrity team to safeguard the quality of each article we publish.
Find out more
ORIGINAL RESEARCH article
Front. Immunol.
Sec. Microbial Immunology
Volume 16 - 2025 | doi: 10.3389/fimmu.2025.1533213
This article is part of the Research Topic Immune Response in Tuberculosis with Comorbidities or Coinfections View all 9 articles
The final, formatted version of the article will be published soon.
You have multiple emails registered with Frontiers:
Please enter your email address:
If you already have an account, please login
You don't have a Frontiers account ? You can register here
SARS-CoV-2 and Mycobacterium tuberculosis (Mtb) share similarities in their modes of transmission, pathophysiological symptoms, and clinical manifestations. An imbalance in the immune response characterised by elevated levels of some inflammatory cytokines caused by tuberculosis (TB) and COVID-19 may increase the risk of developing a severe disease-like condition. It has been reported that TB increases the expression levels of Ace2 (angiotensin converting enzyme 2) and Tmprss2 (transmembrane protease serine 2) proteins, which are essential for COVID-19 pathogenesis. Mutations in single nucleotide polymorphisms (SNPs) of ace2 and tmprss2 genes can impact virus and host-cell interactions and alter immune response by modulating cytokine production. This may modify the susceptibility and/or severity in COVID-19-infected participants. The role of SNPs in ace2 and tmprss2 in relation to Mtb and SARS-CoV-2 co-infection is relatively underexplored. In this study, genotype frequency of 13 SNPs of ace2 and tmprss2 genes in a Cameroonian cohort consisting of COVID-19-positive (n = 31), TB-positive (n = 43), TB-COVID-19 co-infected (n = 21), and a control group (n = 24) were studied. The immune response was estimated by quantitating inflammatory cytokine levels alongside self-reported and clinically diagnosed symptoms. We identified wild-type, heterozygous, and double-mutant genotypes in seven SNPs (rs2285666, rs6632677, rs4646116, rs4646140, rs147311723, rs2074192 and rs4646142) in ace2 gene, which showed significant variations in distribution across the study groups. Our most significant findings include the association of double mutant alleles of rs4646140 and rs2074192 in the ace2 gene with decreased IL-6 and IL-2 expression levels respectively in TB-COVID-19 participants. Also, the double mutant alleles (AA) of rs4646116 were responsible for increased expression level of IL-2 in TB-COVID-19 patients. Additionally, elevated serum levels of AST, urea, and D-dimer, as well as increased plasma concentrations of IL-10, IFN-γ, and TNF-α, have been associated with co-infections involving Mtb and SARS-CoV-2. These biomarkers may reflect the complex interplay between the two pathogens and their impact on host immune responses and disease progression. This study highlights the critical role of genetic and immunological factors in shaping altered immune responses during co-infections involving Mtb and SARS-CoV-2.
Keywords: ACE2, tmprss2, snps, COVID - 19, Tuberculosis
Received: 23 Nov 2024; Accepted: 27 Feb 2025.
Copyright: © 2025 Ngongang Kameni, Berenger Tchoupe, Kamdem, Bhalla, Jean Paul, Njiguet Arnaud, Roger Neba, NANDA, Afum-Adjei Awuah, Amuasi and Masumbe Netongo. This is an open-access article distributed under the terms of the Creative Commons Attribution License (CC BY). The use, distribution or reproduction in other forums is permitted, provided the original author(s) or licensor are credited and that the original publication in this journal is cited, in accordance with accepted academic practice. No use, distribution or reproduction is permitted which does not comply with these terms.
* Correspondence:
Palmer Masumbe Netongo, University of Yaounde I, Yaounde, Centre, Cameroon
Disclaimer: All claims expressed in this article are solely those of the authors and do not necessarily represent those of their affiliated organizations, or those of the publisher, the editors and the reviewers. Any product that may be evaluated in this article or claim that may be made by its manufacturer is not guaranteed or endorsed by the publisher.
Research integrity at Frontiers
Learn more about the work of our research integrity team to safeguard the quality of each article we publish.