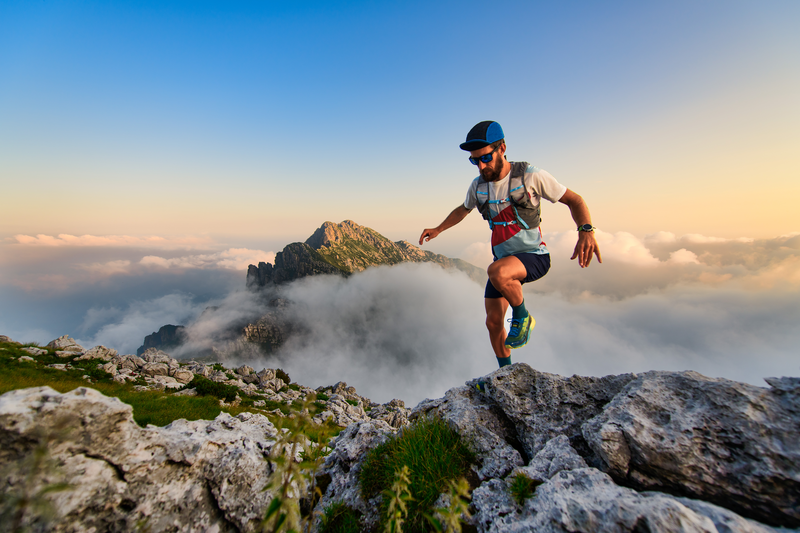
94% of researchers rate our articles as excellent or good
Learn more about the work of our research integrity team to safeguard the quality of each article we publish.
Find out more
ORIGINAL RESEARCH article
Front. Immunol.
Sec. Immunological Memory
Volume 16 - 2025 | doi: 10.3389/fimmu.2025.1531059
This article is part of the Research Topic Exploring Immune Memory Dynamics in Chronic Antigen Exposure and Disease Progression: Implications for Immunotherapy View all 8 articles
The final, formatted version of the article will be published soon.
You have multiple emails registered with Frontiers:
Please enter your email address:
If you already have an account, please login
You don't have a Frontiers account ? You can register here
Chronic lymphocytic leukaemia (CLL) is an uncurable haematological malignancy, associated with significant infection morbidity. Bruton's tyrosine-kinase inhibitors (e.g., ibrutinib) have improved disease outcomes, but severe infections and poor immunization responses afflict patients. Recently, carriage of the endemic Staphylococcus aureus (SA) was associated with lymphocytosis and decreased survival in CLL patients. We then hypothesized that exposure to staphylococcal superantigens (SAgs), known to promote hyper-inflammatory responses, impairs immunity and increases severe infection risk in CLL patients. Herein, we evaluate the reactivity of T cells and CLL cells to SA SAgs, in cultures derived from ibrutinib-treated and untreated CLL patients. We found that ibrutinib-treated patients had less naive CD8+ T cells (p=0.0348), more checkpoint receptor (TIM-3) expression in memory T cells (p<0.0001), and lower IFNγ/cytokine responses in patient T cells (p≤0.0298). Exposure to SA SAg further increased the accumulation of memory T cells with an exhaustion-phenotype, preferentially in cultures derived from ibrutinib-treated patients (p≤0.0350). Nevertheless, staphylococcal SAgs could not induce regulatory T cells from CLL patients inasmuch as healthy donors (p≤0.0461) and this was associated with accumulation of inflammatory T cells. Significantly, SAg-exposure enhanced inflammatory activation of CLL tumour cells, which acquired CD38, CD40, CD86, while downregulating CD27 (p≤0.005), even in cultures from ibrutinib-treated CLL patients. Thus, we suggest that environmental SAg-exposure promotes the accumulation of pseudo-exhausted T cells, which induce/sustain tumour cell activation, not counteracted by ibrutinib. Our study critically helps understand the chronic inflammatory milieu in CLL patients, with implications for infection morbidity, disease aetiology and future interventions.
Keywords: chronic lymphocytic leukaemia, Superantigens, Staphylococcus aureus, pseudoexhaustion, chronic inflammation, Ibrutinib, TSST-1, SEB
Received: 19 Nov 2024; Accepted: 25 Feb 2025.
Copyright: © 2025 Tantoush, Allsup, Naylor-Adamson, Voncken and Caserta. This is an open-access article distributed under the terms of the Creative Commons Attribution License (CC BY). The use, distribution or reproduction in other forums is permitted, provided the original author(s) or licensor are credited and that the original publication in this journal is cited, in accordance with accepted academic practice. No use, distribution or reproduction is permitted which does not comply with these terms.
* Correspondence:
Stefano Caserta, Biomedical Institute for Multimorbidity, Centre for Biomedicine, Hull York Medical School, Faculty of Health Sciences, University of Hull, Hull, United Kingdom
Disclaimer: All claims expressed in this article are solely those of the authors and do not necessarily represent those of their affiliated organizations, or those of the publisher, the editors and the reviewers. Any product that may be evaluated in this article or claim that may be made by its manufacturer is not guaranteed or endorsed by the publisher.
Research integrity at Frontiers
Learn more about the work of our research integrity team to safeguard the quality of each article we publish.