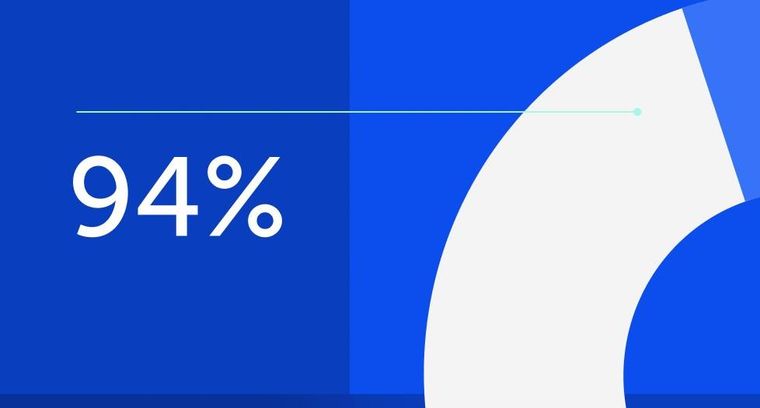
94% of researchers rate our articles as excellent or good
Learn more about the work of our research integrity team to safeguard the quality of each article we publish.
Find out more
SYSTEMATIC REVIEW article
Front. Immunol., 31 March 2025
Sec. Autoimmune and Autoinflammatory Disorders: Autoinflammatory Disorders
Volume 16 - 2025 | https://doi.org/10.3389/fimmu.2025.1526095
This article is part of the Research TopicClinical and Immunological Phenotypic Characterization to better understand Pathogenesis and Response to Therapies in Systemic Autoimmune DiseasesView all articles
Objective: The association of B lymphocytes and B lymphocyte subsets and Parkinson’s disease (PD) is increasingly acknowledged. However, there is inconsistence in the alterations of B lymphocytes or B lymphocyte subsets in peripheral blood of PD patients. To comprehensively understand its changes in PD patients,it is necessary to conduct a systematic review on this subject.
Methods: PubMed, Cochrane Library, and MEDLINE databases were searched until 3rd February 2024.
Results: We included 20 studies (n=2658) to conduct this systematic review. We conducted a qualitative analysis to assess the alterations of B lymphocytes and B lymphocyte subsets in the peripheral blood of individuals with PD. And studies reviewed demonstrated a significant decrease in the number of B cells, as well as immune dysregulation in the B lymphocyte subsets of these patients’ peripheral blood.
Conclusion: Studies reviewed demonstrated that PD is linked to abnormalities in B lymphocytes and/or B lymphocytes subsets in peripheral blood. This study provides a novel perspective into the pathogenesis of PD, and future investigations into the B lymphocytes and/or B lymphocyte subsets as biomarkers and therapeutic targets for PD is warranted.
Parkinson’s Disease(PD), the second most common progressive neurodegenerative disorder, causes motor and non-motor symptoms. PD is not common among individuals younger than 50 years and increases in prevalence with age (1). One data estimated by The Global Burden of Disease Study has shown that the prevalence of PD has reached 11.8 million in 2021, with a percentage change of 273.9%, which may bring a tremendous burden to society (2).
The characteristic of PD is the death of dopaminergic neurons in the substantia nigra. The pathological feature of PD is the Lewy body, which is a neuronal inclusion consistinglargely of α-synuclein protein aggregations (3). Although decades of research and the development of a large group of animal models, our understanding of the mechanisms responsible for the progressive loss of dopamine neurons in PD is still unclear (4). Research has shown that IgG antibodies deposit on dopaminergic neurons in Parkinson’s disease patients, and the Lewy bodies were also coated by IgG (5). Although B lymphocytes were not detected in the brains of PD patients (6), these results were later supported by animal model studies of PD, revealing long-term infiltration of B cells in the midbrain of rodents (7–9) and non-human primates (10, 11). Therefore, the role of B lymphatic system in the occurrence and development of PD is receiving increasing attention (12–16).
B lymphocytes, as a part of the adaptive immune system, play multiple roles and have complex interactions with other branches of the innate and adaptive immune systems (17). A simple definition of B lymphocytes is a population of cells that express clonally diverse cell surface immunoglobulin (Ig) receptors which can recognize specific antigenic epitopes (18). B lymphocytes are crucial for normal immune system development and its maintenance (18). In addition to producing antibodies, B lymphocytes also can release immune regulatory cytokines, antigen-presenting cell functions, and regulate T cells and the innate response (17, 18). Studies have shown that after the death of dopaminergic neurons, their antigens are presented to the immune system, with activation of B lymphocytes. Then, B lymphocytes or specific autoantibodies might enter the central nervous system through the dysfunctional blood brain barrier, produce cytokines that activate microglia, and release autoantibodies. This may lead to further inflammation and subsequent cell death (4).
Inflammation and aberrant immune responses also play a crucial role in the development of PD (19, 20). Regulatory B cells (Bregs), a subpopulation of lymphocytes, have been associated with the inhibition of excessive inflammation and play a crucial role in maintaining immune homeostasis. Dysfunction of Bregs has been observed in PD. Immune dysfunction has become a research hotspot in the pathogenesis and progression of PD. In recent years, domestic and foreign researchers have further explored immunophenotype of peripheral blood B lymphocytes in Parkinson’s disease (12–16). These results indicate that the alterations of B lymphocytes or B lymphocyte subsets in peripheral blood are associated with the progression of PD (14, 21, 22). These peripheral abnormalities may drive the occurrence and progression of PD by enhancing immune cell infiltration and enhancing neuroinflammation in the central nervous system (23). Although many studies have shown an association between B lymphocytes (or B lymphocyte subsets) and PD, these results are inconsistent in the alterations of B lymphocytes or B lymphocyte subsets in peripheral of PD patients. To comprehensively understand the changes in B lymphocytes or B lymphocyte subsets in PD patients, and to provide crucial evidence for studying the mechanisms underlying this illness, it is necessary to conduct a systematic review on this subject. Moreover, the precise nature of the changes in B lymphocytes or B lymphocyte subsets in peripheral of PD patients remain unclear. Consequently, a systematic review of studies of various B lymphocyte subsets in PD is also required for a greater understanding of the nature of immune dysfunction in this illness.
Studies published before 3rd February 2024 were searched in PubMed, Cochrane Library, and MEDLINE databases. Standard keyword searches were conducted using “Parkinson’s disease”, “B lymphocytes”, “CD19+ B cells”, “CD20+ B cells”, “transitional B cells”, “regulatory B cells (Bregs)”, “naïve B cells”, “memory B cells”or “plasma cells”. Two authors (H-X, M and Z-Y, W) screened titles and abstracts independently and relevant studies were retrieved. Electronic search has been supplemented by hand-searching meta-analysis and review articles. Any differences were resolved through further discussion. The detailed search strategy for each database is presented in the online Supplementary Materials. This review and protocol was prospectively registered on PROSPERO (registration No. CRD42024508329) and conducted following the PRISMA’s Preferred Reporting Items guidelines for Systematic Reviews and Meta-analyses Protocols (24). The PRISMA checklist is presented in Supplementary Table 1.
The research question of our systematic review clearly defined in terms of populations, interventions, comparators, outcomes, and study designs (PICOS): (1) Study type (S): case-control or cohort in design; (2) Participants (P): patients were clinically diagnosed with PD (patients who were on immunomodulatory treatments or had recent vaccinatinos or infections have been excluded); (3)Interventions (I): used flow cytometry, single cell RNA-seq, or related techniques to identify and describe peripheral blood B lymphocytes phenotype; (4) Comparison (C): healthy individuals; (5) Outcome (O): at least one of the following endpoints (the percentage of B lymphocyte subsets or absolute counts of B lymphocyte in peripheral blood) was reported, including CD19+ B cells, CD20+ B cells, transitional B cells, regulatory B cells, naïve B cells, memory B cells, and plasma cells.
Exclusion criteria: (1) Not written in English; (2) Duplicate publication; (3) Case report; (4) Studies that did not report B lymphocyte count or phenotype were excluded.
The following data were collected from each included study: (1) Baseline characteristics: including first author’s name, year of publication, country, sample size, sex ratio, H&Y score, sampling source, case definition, control definition, immune cells assessed, and Newcastle-Ottawa Scale (NOS) Quality scores; (2) Mean and Standard deviation (SD) of the counts or percentage of B lymphocyte and B lymphocyte subsets in peripheral blood in PD patients and controls; (3) Individual immune cell types and cell classifications used in the current investigation (Supplementary Table 2). Two researchers (M, Y and Y-B, Z) were responsible for extracting the necessary data for this meta-analysis and systematic review. In cases where the required data were not reported, we would contact the author to obtain this data. Any disagreements or differences were resolved through further discussion with a third independent researcher (H-X, M), if necessary.
The methodological quality of each included study was assessed independently by two authors (M, Y and Y-B, Z), and any disagreements were resolved through further discussion led by another author (H-X, M). We employed an adapted version of the NOS for case-control studies (25) to assess the methodological quality. The non-response rate, one NOS scoring item, was considered irrelevant to the current investigation, so the maximum possible NOS score here is was 8. A score of 5-8 indicates high-quality research.
As shown in Figure 1, we identified 3263 records from PubMed, Cochrane Library, MEDLINE databases. After excluding the duplicates studies (1586 records) in EndNote, the irrelevant studies (1612 records) were removed through reading titles and abstracts and the remaining 68 articles needed us to read the full text to identify available data. 48 articles were excluded. Ultimately, a total of 20 studies encompassing 2658 unique study participants, including 1407 PD patients and 1251 healthy controls, were included in the systematic review. The basic characteristics of included studies were presented in Table 1.
We used the NOS to assess the methodological quality of studies included in our systematic review. All 20 studies had an NOS score of ≥5, indicating a relatively high quality of these studies. The detailed quality assessment is presented in Supplementary Table 3.
The surface expression of CD19 and CD20 is induced early in B cell development in the bone marrow (26, 27). They are all expressed on subsets of B cells in the blood (28). Therefore, CD19 and CD20 are used to identify B cells (Supplementary Table 2). Researchers are increasingly intrigued by the potential role of B cells in the adaptive immune system’s contribution to the pathogenesis of PD. To understand the phenotypic and functional characteristics of circulating B cells in PD patients, sixteen studies (13–15, 21, 23, 29–39) reported the levels of CD19+ B cells in peripheral blood according to flow cytometry markers assessed. The results of eight studies (13–15, 31–33, 37, 38) showed a significant decrease in CD19+ B cells in PD patients compared with controls. Conversely, the results of other studies (21, 23, 29, 30, 34–36, 39) showed that there was no significant difference in CD19+ B cells between PD patients and controls. Moreover, two studies (22, 40) reported the alteration of CD20+ B cells in peripheral blood according to flow cytometry markers assessed, and the results showed that a significant decrease in CD20+ B cells in PD patients compared with controls. Furthermore, another study by Horvath et al. (41) reported the estimated proportions of B cells based on the Houseman method (42), and the results indicated that PD patients had fewer B cells. However, available information couldn’t be extracted. Therefore, we performed a qualitative analysis in our study. Result is presented in Table 2.
Table 2. Summary of clinical information and the results of B lymphocytes and B lymphocyte subsets in peripheral blood of PD patients.
B lymphocyte subsets were identified according to flow cytometry markers assessed (Supplementary Table 2).
To elucidate the potential mechanisms underlying the roles of circulating B lymphocyte subsets in PD patients, two studies (13, 14) reported the levels of transitional B cells in peripheral of PD patients. The results of Rui Li et al. (13) indicated a decreased disproportionally in transitional B cells in PD patients compared with controls. However, the results of Kirsten M Scott et al. (14) indicated no significant difference in the levels of transitional B cells between PD patients and controls.
To elucidate the potential mechanisms underlying the roles of circulating B lymphocyte subsets in PD patients, four studies (14, 29, 32, 37) reported the alteration of regulatory B cells in peripheral blood of PD patients and controls. In the study by Kirsten M Scott et al., the results showed a reduction in CD1d + (regulatory) B cells in PD patients compared to controls. Similarly, Álvarez-Luquín et al. (29) also observed similar results that the levels of functional Bregs (CD19+ CD38 hi CD24 hi IL-10+) were significantly lower in PD patients than in controls. Meanwhile, they also reported other human regulatory B cells in PD patients and controls, including CD19+ IL-10+, Plasmatic cells IL-10+ (CD19- CD138+ IL-10+), and regulatory B cells (CD19+ CD5+ CD1d+ FOXP3+ IL-10+), but the results indicated no significant difference between PD patients and controls. On the contrary, significantly higher proportions of regulatory B cells (CD19+ CD5+ IL-10+ and CD19+ CD5+ FoxP3+) were detected in PD patients than in controls reported by Garfias S et al (32). Similarly, We also observed that the percentage of regulatory B cells was elevated in early PD patients compared with controls reported by Zhuo Zhang et al.37 However, in their results, no significant difference was observed in the absolute counts of regulatory B cells between PD patients and controls.
Five studies (13, 14, 16, 23, 37) reported the alteration of Naïve B cells in peripheral blood of PD patients and controls. Specifically, the results of three studies (16, 23, 37) indicated a significant decrease in naïve B cells in PD patients compared with controls. However, the results of two studies indicated no significant difference in naïve B cells between PD patients and controls (13, 14).
Memory B cells can be categorized into three groups: Un-class-switched Memory (USM) B cells, Class-switched Memory (CSM) B cells, and Double Negative Memory (DNM) B cells. Five studies (13, 14, 16, 23, 37) reported the levels of memory B cells in peripheral of PD patients. The results of two studies (16, 23) indicated a significant increase of USM B cells in PD patients compared with controls, while no statistically significant differences were observed in the other three studies (13, 14, 37). The alteration of CSM B cells in the peripheral blood of PD patients and controls was reported in five studies (13, 14, 16, 23, 37), but no statistically significant differences were observed. Four studies examined the alteration of DNM B cells in peripheral blood of PD patients and controls. The results of two studies (23, 37) indicated a significant increase in DNM B cells in PD patients. The results of Rui Li et al. (13) indicateda significant decrease in DNM B cells. However, the results of Kirsten M Scott et al (14) indicated no statistically significant differences in DNM B cells between PD patients and controls.
Six studies (13, 14, 16, 30, 37, 41) reported the alteration of plasma cells in peripheral blood of PD patients and controls. Notably, two studies found an increase in plasma cells among individuals with PD (37, 41). Conversely, Álvarez-Luquín DD et al. reported a decrease in plasma cells in PD patients (30). In the remaining three studies, no statistically significant differences were observed between PD patients and controls (13, 14, 16).
Subtle changes in the central nervous system microenvironment caused by peripheral changes in blood circulation or other organs (functional impairment, ecological imbalance, or inflammation) may have a significant impact on the function of the central nervous system (43). Research has shown that IgG antibodies deposit on dopaminergic neurons in PD patients, and the Lewy bodies were also coated by IgG (5). Recent evidence suggests that B lymphocytes in peripheral blood may interact with the central nervous system in complex manners via the meningeal lymphatic system (17, 44, 45) and via the channels in the skull bone marrow that allow the egress of calvarial immune cells, including B lymphocytes (46). These findings suggest that B lymphocytes may participate in the inflammation of PD in complex manners, including centrally and peripherally (47). However, the alterations of B lymphocytes and B lymphocyte subsets in peripheral blood of PD patients remain unclear. Our systematic review showed that compared with the control group, there were changes in the levels of B lymphocytes and B lymphocyte subsets in the peripheral of PD patients, including total B lymphocytes, transitional B cells, regulatory B cells, naïve B cells, memory B cells, and plasma cells, which indicated that Parkinson’s disease may be associated with the immune dysfunction of B lymphocytes or B lymphocyte subsets in peripheral blood. To the best of our knowledge, this is the first systematic review to consider the immune phenotypes of peripheral blood cells in Parkinson’s disease patients. Our findings emphasize the potential role of adaptive immune dysfunction of B lymphocytes in the pathogenesis of Parkinson’s disease.
Firstly, we found an inconsistent result in the alteration of CD19+ B cells in the peripheral blood of PD patients among studies. Interestingly, we noticed a significant decrease in counts of CD19+ B cells in peripheral blood in PD patients compared to controls. However, the results of the studies showed no difference in the percentage of CD19+ B cells in peripheral blood between PD patients and controls. A possible explanation for this phenomenon could be that relative percentages are a holistic representation of the immune landscape at the time of sampling. Unlike the total cell count, changes in relative percentage of one cell type may be influenced by changes in another cell types (48). Therefore, according to the standard practice of immunology, we will focus on the results of the absolute counts of B cells in peripheral blood as primary. In our systematic review, the studies reviewed demonstrated that the alterations of CD19+ B cells in the peripheral blood are influenced by sex (49), race, age, disease severity, PD duration, and the impact of medication use. Clinical variability may be the reason for the differences between studies in the literature (Supplementary Table 4). So, it will be necessary to consider these factors further in future research.
A significant decrease in the level of B cells was observed in PD. Several studies have described possible reasons for the decrease in B lymphocytes in peripheral blood of PD patients, such as clinical severity (H&Y), disease progresses, disease duration, and α-synuclein pathology (Supplementary Table 4).
To investigate the impact of decreased B lymphocytes on the course of parkinson’s disease, Kirsten M Scott et al. (14) conducted a study comparing motor and histological outcomes in two groups of mice: mature B lymphocytes deficient μMT mice and mice treated with a CD20 monoclonal antibody that can deplete mature B cells. The results indicate that depleting B lymphocytes either genetically or using a monoclonal antibody targeting the B cell antigen CD20 can lead to worse motor outcomes and more extensive dopamine loss than controls. Their results indicated that B lymphocytes play an early protective role in dopaminergic cell loss. We have seen similar results in PD patients. The percentage of CD19+ B cells in PD patients with the scores on the Unified Parkinson’s Disease Rating Scale (UPDRS) >24 was significantly lower than that in patients with UPDRS score < 24 reported by Luan Cen et al. (21), which indicated that the level of CD19+ B cells was negatively correlated with PD severity. In another study, we also observed that the decrease in the number of CD19+ B cells varied with the clinical stage of PD (15). Overall, these findings demonstrate an association between changes in B lymphocytes and the progression of PD, indicating that alterations in B lymphocytes in peripheral blood could potentially serve as a predictor of PD progression.
Neuroinflammation plays a fundamental role in the pathophysiology of PD. Neurohistological and neuroimaging studies support the presence of ongoing and end-stage neuroinflammatory processes in PD (50). Research has shown that the immune system is continuously activated in individuals with chronic inflammation, characterized by a deficiency in the number and function of these inhibitory cells in circulation (51). Bregs, an immunosuppressive cells, support immunological tolerance (52). By producing transforming growth factor β (TGF-β), interleukin-35 (IL-35), and interleukin-10 (IL-10), Bregs suppress immunopathology by inhibiting the expansion of pathogenic T cells and other proinflammatory lymphocytes (52). However, unlike regulatory T cells (Tregs), no unique biomarker or release factor was used to define Breg (53). Instead, the term Breg refers to B cells with regulatory functions. Transitional B cells and IL-10-producing B cells which are antiinflammatory B lymphocyte subsets are well recognized in the field of autoimmunity as important checkpoint regulators of autoreactive T cells (13, 54). In the studies we included, the results regarding peripheral Bregs in individuals with PD were inconsistent. In the results of Garfias S et al. (32), significantly higher proportions of Bregs were detected in PD patients than in controls. However, their results showed a decrease in the percentage of Bregs between baseline and follow-up, which indicated that the alterations of Bregs may be related to the duration of PD. Bregs dysfunction has been found in many autoimmune diseases (55–59), such as multiple sclerosis, pemphigus, systemic sclerosis, and myasthenia gravis, but further work is needed to determine the pathogenic significance and the mechanisms that underlie this.
Bregs have been associated with the inhibition of excessive inflammation (60) and play a crucial role in maintaining immune homeostasis (61). The use of transgenic mice lacking B cells (62), and more specifically IL-10-producing B cells (63), has shown that defective Breg cell development and function lead to chronic inflammation. In the study of Kirsten M Scott et al. (14), the evidence that B lymphocyte deficiency or depletion leads to worse pathological and behavioral outcomes was shown in a toxin-based mouse model of PD. Furthermore, Kirsten M Scott et al. reported that PD Patients who had a greater proportion of Bregs had better motor scores. This further demonstrates the important role of B lymphocytes and Bregs in the pathogenesis of PD. At the same time, this also indicates that B lymphocytes or Bregs can serve as targets for the treatment of PD. However, the role of B lymphocytes and Bregs remain unclear in PD patients. Their protective effect can be partially explained by alleviating various immune mediated inflammatory conditions.
In previous studies (64, 65), we noticed that the naïve B cell compartment was significantly reduced after the depletion of the B cell activating factor of the tumor necrosis factor family(BAFF). The BAFF plays critical roles in supporting the survival of mature naïve B cells (66, 67) and has a physiological role in B lymphocyte immune regulation (68). However, inappropriate generation of BAFF may be a key event that disrupts immune tolerance associated with systemic autoimmune diseases. Some evidence suggested that an increase in BAFF levels may not only directly interfere with B cell immunity by triggering their maturation and survival, but also indirectly interfere with B cell immunity by dysregulating innate immune responses (69) and T cell activation and balance (70). In turn, that can lead to the breakdown of immune tolerance, the production of autoantibodies, and sustained local intracerebral inflammation and tissue damage (68). In the study of Zhuo Zhang et al. (37), their results showed that the serum BAFF levels increased in PD patients. Tabalumab, fully anti-BAFF humanized monoclonal antibodies, has been tested in patients with relapsing-remitting multiple sclerosis in a phase II randomized, double-blind, placebo-controlled study (ClinicalTrials.gov study identifier: NCT00882999). The BAFF may also be potential targets for PD intervention. However, there are limited studies on the correlation between BAFF, peripheral blood B lymphocyte subsets, and the severity of PD have been retrieved. Therefore, the role of BAFF in PD remains unclear and warrants further research in the future.
Memory B cells can mediate rapid and strong antibody responses during secondary immune responses (28). Notably, a study provided evidence that the USM B cells exhibited faster and stronger re-stimulation potential and participated in early inflammatory response (71). In the study of Pingping Wang et al., single cell RNA and B cell receptor (BCR) sequencing for B cells from PD patients and controls were performed (16). Their results showed that the level of USM B cells (especially the USM2 subset) increased significantly in PD patients compared to controls, and the USM2 subset had strong activation characteristics and high proliferation potential. Pingping Wang et al. (16) speculate that the USM2 subset might be an important participant in the humoral immune response of PD and might ultimately promote the production of infiltrating antibodies in the brain of PD patients. On the other hand, the DNM B cells are believed to be associated with aging and senescence (72). Studies showed that in the peripheral blood of healthy individuals, the DNM B cells were detected at low levels (73) and were expanded in elderly patients (74) and Alzheimer’s disease (AD) patients (75). The DNM B cells are also associated with inflammatory response (23) and are thought to produce elevated levels of pro-inflammatory cytokines (72). In our article, the studies reviewed demonstrated inconsistent results in the alteration of DNM B cells in the peripheral blood of PD patients across studies. The accumulation of the DNM B cells in chronic infections, autoimmune diseases and with advanced age has been observed (76–78). However, the role of the DNM B cells is still unknown in PD patients.
B lymphocytes eventually differentiate into plasma cells and produce antibodies. Autoantibodies against α-synuclein, dopamine and melanin were found in the serum and cerebrospinal fluid of PD patients (79, 80), and the levels of α-synuclein autoantibodies in plasma and cerebrospinal fluid were associated with disease activity in patients with mild or moderate PD (81). Studies showed that antibody-based therapies against α-synuclein have achieved enviable results in clinical trials, and may be expected to provide a new treatment method for PD (82, 83). Memory B cells can differentiate into plasma cells in a secondary response, either through direct differentiation after antigen attack or by seeding of germinal centers that in turn generate new plasma cells (84). In the current research, we noticed an inconsistent result in the alteration of plasma cells in the peripheral blood of PD patients compared to controls. Due to the fact that plasma cells are not themselves circulating, the upstream progenitor cell pool must contribute (84). This may be the reason for the inconsistent results of plasma cell changes.
The alterations of B lymphocyte subsets are also associated with increased cytokine production in PD patients (13, 23). Although the evidence of cytokine abnormalities in peripheral blood has been well established in PD (85), the alterations of B lymphocyte subsets in this illness are relatively less understood. Identifying the immunophenotype of B lymphocyte subsets in PD may help elucidate the cellular sources of these known cytokine alterations. Future research on the cytokines as biomarkers and therapeutic targets for PD may be warranted (85).
Immunological activation and neuroinflammation are pivotal in the pathogenesis of PD (86). Although these studies have reported the alterations of B lymphocytes and B lymphocyte subsets in peripheral blood of PD, most of them are cross-sectional from a study design standpoint. As a result, they can only capture a snapshot of the immune status of PD patients and cannot relate their findings to the course and outcomes of the patients (13), nor can they infer causality. Therefore, future studies that longitudinally assess the immune profiles of patients may provide more insights into the involvement of B lymphocytes and B lymphocyte subsets in the disease. Furthermore, the studies reviewed demonstrated that the alterations of B cells in the peripheral blood are subject to a variety of influences, including gender, race, age, disease severity, the duration of Parkinson’s disease, and medication use. Consequently, it is imperative that future research designs rigorously control for these confounding variables to mitigate their effects on the analysis of peripheral blood B cells.
B lymphocytes are crucial for normal immune system development and its maintenance (17). In addition to producing antibodies, B lymphocytes also can release immune regulatory cytokines, antigen-presenting cell functions, and regulate T cells and the innate response (17, 18). B cells are activated and the antigen presentation capacity of B cells is enhanced in PD patients (16). It can uptake, process, and present antigens to T cells, activating the immune response of T cells. T cell immune response can trigger type 1 pro-inflammatory activities and suppress type 2 anti-inflammatory activities, eventually resulting in deregulated neuroinflammation and subsequent dopaminergic neurodegeneration (87). After the death of dopaminergic neurons, their antigens are presented to the immune system, with activation of B lymphocytes. Then, B lymphocytes or specific autoantibodies might enter the central nervous system through the dysfunctional blood brain barrier, produce cytokines that activate microglia, or release autoantibodies. This may lead to further inflammation and subsequent cell death (4). The possible mechanism of B cells in PD is shown in Figure 2.
Figure 2. Potential roles for B cells in PD. The possible reasons for the decrease in B cells, the potential involvement of B cells in PD, and the diverse developmental stages of B cell subsets. B cell levels are reduced in PD patients for several possible reasons, including alpha-synuclein pathology, PD effect, age, clinical severity(H&Y), disease progresses, disease duration, the migration of B lymphocytes to other compartments, gender, medication use, and migration to secondary lymphoid organs. But B cells are activated and there is a significant increase in the pro-inflammatory cytokines TNF-α, IL-6 and GM-CSF, and a significant decrease in the anti-inflammatory cytokine IL-10 in PD, which eventually leads to deregulated neuroinflammation and subsequent dopaminergic neurodegeneration. IgG antibodies deposit on dopaminergic neurons in PD patients, and the Lewy bodies are also coated by IgG. Although anti-alpha-synuclein antibodies are present in the blood of PD patients, but it is still unclear whether these antibodies are the same as those that bind to neurons in the substantia nigra and Lewy bodies in these individuals. B cells are activated and the antigen presentation capacity of B cells is enhanced in PD patients. B lymphocytes can uptake, process, and present antigens to T cells, activating the immune response of T cells. T cell immune response can trigger type 1 pro-inflammatory activities and suppress type 2 anti-inflammatory activities, eventually resulting in deregulated neuroinflammation and subsequent dopaminergic neurodegeneration. T, T cell; USM B cells, un-class-switched memory B cells; CSM B cells, Class-switched Memory B cells; DNM B cells, Double Negative Memory B cells.
At present, no treatment method can slow down or arrest the progression of PD (88). The disease modification therapy that reduces the rate of neurodegeneration or prevents the progression of PD is still the biggest treatment requirement in PD (89), but informed by new insights into the immune dysfunction mechanism of PD in our study, immunotherapies targeting dysregulated B lymphocytes and B lymphocyte subsets (Supplementary Table 4) may be a promising strategy for disease-modifying potential.
In the current research, there are several potential limitations: (1)The evolution of high-dimensional phenotype technology has identified many new B lymphocyte subsets (90–93). Given the marked diversity in B lymphocyte subsets phenotype, we selected the main subsets of B lymphocytes in peripheral blood for a systematic review; (2)It is crucial to adopt a unified and consistent method for identifying and describing B lymphocyte subsets to ensure the reproducibility of scientific research and and that similar conclusions can be drawn from them (28). However, to gain a more comprehensive understanding of the alteration in B lymphocytes and B lymphocyte subsets in peripheral blood of PD patients, in addition to flow cytometry, studies using other techniques [such as single-cell RNA-seq and the epigenetic clock (based on the DNA methylation levels)] to identify and describe the alteration in B lymphocytes and B lymphocyte subsets in peripheral blood of PD patients were also included in our study; (3)As is well known, it is difficult to infer causality from case-control studies. As a result of our research, it is currently unclear whether the observed alteration in B lymphocytes and B lymphocyte subsets in acquired immunity are a causal or secondary to central nervous system disorder associated with the pathogenesis of PD.
Our study comprehensively and systematically reviewed the alterations of B lymphocytes and B lymphocyte subsets and provided evidence of a decrease in B lymphocyte levels and immune dysfunction in B lymphocyte subsets in peripheral of PD patients. PD is associated with the alterations of B lymphocytes and (or) B lymphocyte subsets in peripheral blood. This study provides a novel perspective into the pathogenesis of PD, and future investigations into the B lymphocytes and (or) B lymphocyte subsets as biomarkers and therapeutic targets for PD may be warranted.
The original contributions presented in the study are included in the article/Supplementary Material. Further inquiries can be directed to the corresponding author.
JY: Funding acquisition, Project administration, Writing – review & editing. HM: Conceptualization, Data curation, Investigation, Methodology, Software, Visualization, Writing – original draft, Writing – review & editing. ZW: Data curation, Software, Visualization, Writing – original draft. MY: Data curation, Writing – original draft. YZ: Data curation, Writing – original draft.
The author(s) declare that financial support was received for the research and/or publication of this article. This study was funded by the Science and Technology Research of Henan Province (242103810041) and the Key Technologies Program of Henan Province (222102310220. The funder played no role in study design, data collection, analysis and interpretation of data, or the writing of this manuscript.
The authors declare that the research was conducted in the absence of any commercial or financial relationships that could be construed as a potential conflict of interest.
The author(s) declare that no Generative AI was used in the creation of this manuscript.
All claims expressed in this article are solely those of the authors and do not necessarily represent those of their affiliated organizations, or those of the publisher, the editors and the reviewers. Any product that may be evaluated in this article, or claim that may be made by its manufacturer, is not guaranteed or endorsed by the publisher.
The Supplementary Material for this article can be found online at: https://www.frontiersin.org/articles/10.3389/fimmu.2025.1526095/full#supplementary-material
PD, Parkinson’s disease; Bregs, Regulatory B cells; NOS, quality scores Newcastle-Ottawa Scale; SD, Standard deviation; USM B cells, Un-class-switched Memory B cells; CSM B cells, Class-switched Memory B cells; DNM B cells, Double Negative Memory B cells; UPDRS, Unified Parkinson’s Disease Rating Scale; TGF-β, Transforming growth factor β; IL-35, Interleukin-35; IL-10, Interleukin-10; Tregs, Regulatory T cells; BAFF, B cell activating factor of the tumor necrosis factor family; BCR, B cell receptor; AD, Alzheimer’s disease.
1. Feigin VL, Nichols E, Alam T, Bannick MS, Beghi E, Blake N, et al. Global, regional, and national burden of neurological disorders, 1990-2016: a systematic analysis for the Global Burden of Disease Study 2016. Lancet Neurol. (2019) 18:459–80. doi: 10.1016/S1474-4422(18)30499-X
2. Steinmetz JD, Seeher KM, Schiess N, Nichols E, Cao B, Servili C, et al. Global, regional, and national burden of disorders affecting the nervous system, 1990-2021: a systematic analysis for the Global Burden of Disease Study 2021. Lancet Neurol. (2024) 23:344–81. doi: 10.1016/S1474-4422(24)00038-3
3. Armstrong MJ, Okun MS. Diagnosis and treatment of Parkinson disease. JAMA. (2020) 323(6):548–60. doi: 10.1001/jama.2019.22360
4. Monahan AJ, Warren M, Carvey PM. Neuroinflammation and peripheral immune infiltration in Parkinson’s disease: an autoimmune hypothesis. Cell Transplant. (2008) 17:363–72. doi: 10.3727/096368908784423328
5. Orr CF, Rowe DB, Mizuno Y, Mori H, Halliday GM. A possible role for humoral immunity in the pathogenesis of Parkinson’s disease. Brain. (2005) 128:2665–74. doi: 10.1093/brain/awh625
6. Brochard V, Combadière B, Prigent A, Laouar Y, Perrin A, Beray-Berthat V, et al. Infiltration of CD4+ lymphocytes into the brain contributes to neurodegeneration in a mouse model of Parkinson disease. J Clin Invest. (2009) 119:182–92. doi: 10.1172/JCI36470
7. Campolo M, Filippone A, Biondo C, Mancuso G, Casili G, Lanza M, et al. TLR7/8 in the pathogenesis of Parkinson’s disease. Int J Mol Sci. (2020) 21(24):9384. doi: 10.3390/ijms21249384
8. Sanchez-Guajardo V, Annibali A, Jensen PH, Romero-Ramos M. [amp]]alpha;-Synuclein vaccination prevents the accumulation of Parkinson disease-like pathologic inclusions in striatum in association with regulatory T cell recruitment in a rat model. J neuropathology Exp Neurol. (2013) 72:624–45. doi: 10.1097/NEN.0b013e31829768d2
9. Theodore S, Cao S, McLean PJ, Standaert DG. Targeted overexpression of human alpha-synuclein triggers microglial activation and an adaptive immune response in a mouse model of Parkinson disease. J neuropathology Exp Neurol. (2008) 67:1149–58. doi: 10.1097/NEN.0b013e31818e5e99
10. Balzano T, Del Rey NL, Esteban-García N, Reinares-Sebastián A, Pineda-Pardo JA, Trigo-Damas I, et al. Neurovascular and immune factors of vulnerability of substantia nigra dopaminergic neurons in non-human primates. NPJ Parkinson’s Dis. (2024) 10:118. doi: 10.1038/s41531-024-00735-w
11. Barkholt P, Sanchez-Guajardo V, Kirik D, Romero-Ramos M. Long-term polarization of microglia upon α-synuclein overexpression in nonhuman primates. Neuroscience. (2012) 208:85–96. doi: 10.1016/j.neuroscience.2012.02.004
12. Fyfe I. Peripheral B cells altered in Parkinson disease. Nat Rev Neurol. (2023) 19:711. doi: 10.1038/s41582-023-00902-2
13. Li R, Tropea TF, Baratta LR, Zuroff L, Diaz-Ortiz ME, Zhang B, et al. Abnormal B-cell and Tfh-cell profiles in patients with Parkinson disease: A cross-sectional study. Neurology(R) neuroimmunology Neuroinflamm. (2022) 9(2):e1125. doi: 10.1212/NXI.0000000000001125
14. Scott KM, Chong YT, Park S, Wijeyekoon RS, Hayat S, Mathews RJ, et al. B lymphocyte responses in Parkinson’s disease and their possible significance in disease progression. Brain Commun. (2023) 5:fcad060. doi: 10.1093/braincomms/fcad060
15. Stevens CH, Rowe D, Morel-Kopp MC, Orr C, Russell T, Ranola M, et al. Reduced T helper and B lymphocytes in Parkinson’s disease. J neuroimmunology. (2012) 252:95–9. doi: 10.1016/j.jneuroim.2012.07.015
16. Wang P, Luo M, Zhou W, Jin X, Xu Z, Yan S, et al. Global characterization of peripheral B cells in Parkinson’s disease by single-cell RNA and BCR sequencing. Front Immunol. (2022) 13:814239. doi: 10.3389/fimmu.2022.814239
17. Scott KM. B lymphocytes in Parkinson’s disease. J Parkinson’s Dis. (2022) 12:S75–s81. doi: 10.3233/JPD-223418
18. LeBien TW, Tedder TF. B lymphocytes: how they develop and function. Blood. (2008) 112:1570–80. doi: 10.1182/blood-2008-02-078071
19. More SV, Kumar H, Kim IS, Song SY, Choi DK. Cellular and molecular mediators of neuroinflammation in the pathogenesis of Parkinson’s disease. Mediators Inflammation. (2013) 2013:952375. doi: 10.1155/2013/952375
20. Noelker C, Morel L, Osterloh A, Alvarez-Fischer D, Lescot T, Breloer M, et al. Heat shock protein 60: an endogenous inducer of dopaminergic cell death in Parkinson disease. J Neuroinflamm. (2014) 11:86. doi: 10.1186/1742-2094-11-86
21. Cen L, Yang C, Huang S, Zhou M, Tang X, Li K, et al. Peripheral lymphocyte subsets as a marker of Parkinson’s disease in a Chinese population. Neurosci Bull. (2017) 33:493–500. doi: 10.1007/s12264-017-0163-9
22. Gruden MA, Sewell RD, Yanamandra K, Davidova TV, Kucheryanu VG, Bocharov EV, et al. Immunoprotection against toxic biomarkers is retained during Parkinson’s disease progression. J neuroimmunology. (2011) 233:221–7. doi: 10.1016/j.jneuroim.2010.12.001
23. Yan Z, Yang W, Wei H, Dean MN, Standaert DG, Cutter GR, et al. Dysregulation of the adaptive immune system in patients with early-stage Parkinson disease. Neurology(R) neuroimmunology Neuroinflamm. (2021) 8(5):e1036. doi: 10.1212/NXI.0000000000001036
24. Moher D, Liberati A, Tetzlaff J, Altman DG. Preferred reporting items for systematic reviews and meta-analyses: the PRISMA Statement. Open medicine: peer-reviewed independent open-access J. (2009) 3:e123–30.
25. Stang A. Critical evaluation of the Newcastle-Ottawa scale for the assessment of the quality of nonrandomized studies in meta-analyses. Eur J Epidemiol. (2010) 25:603–5. doi: 10.1007/s10654-010-9491-z
26. Bendall SC, Davis KL, Amir el AD, Tadmor MD, Simonds EF, Chen TJ, et al. Single-cell trajectory detection uncovers progression and regulatory coordination in human B cell development. Cell. (2014) 157(3):714–25. doi: 10.1016/j.cell.2014.04.005
27. Scheuermann RH, Racila E. CD19 antigen in leukemia and lymphoma diagnosis and immunotherapy. Leukemia lymphoma. (1995) 18:385–97. doi: 10.3109/10428199509059636
28. Velounias RL, Tull TJ. Human B-cell subset identification and changes in inflammatory diseases. Clin Exp Immunol. (2022) 210:201–16. doi: 10.1093/cei/uxac104
29. Álvarez-Luquín DD, Arce-Sillas A, Leyva-Hernández J, Sevilla-Reyes E, Boll MC, Montes-Moratilla E, et al. Regulatory impairment in untreated Parkinson’s disease is not restricted to Tregs: other regulatory populations are also involved. J Neuroinflamm. (2019) 16:212.
30. Álvarez-Luquín DD, Guevara-Salinas A, Arce-Sillas A, Espinosa-Cárdenas R, Leyva-Hernández J, Montes-Moratilla EU, et al. Increased Tc17 cell levels and imbalance of naïve/effector immune response in Parkinson’s disease patients in a two-year follow-up: a case control study. J Trans Med. (2021) 19:378.
31. Bas J, Calopa M, Mestre M, Molleví DG, Cutillas B, Ambrosio S, et al. Lymphocyte populations in Parkinson’s disease and in rat models of parkinsonism. J neuroimmunology. (2001) 113:146–52. doi: 10.1016/S0165-5728(00)00422-7
32. Garfias S, Tamaya Domínguez B, Toledo Rojas A, Arroyo M, Rodríguez U, Boll C, et al. Peripheral blood lymphocyte phenotypes in Alzheimer and Parkinson’s diseases. Neurologia. (2022) 37:110–21. doi: 10.1016/j.nrl.2018.10.004
33. Hurny A, Michałowska-Wender G, Wender M. Impact of L-DOPA treatment of patients with Parkinson’s disease on mononuclear subsets and phagocytosis in the peripheral blood. Folia neuropathologica. (2013) 51:127–31. doi: 10.5114/fn.2013.35955
34. Perner C, Perner F, Gaur N, Zimmermann S, Witte OW, Heidel FH, et al. Plasma VCAM1 levels correlate with disease severity in Parkinson’s disease. J Neuroinflamm. (2019) 16:94. doi: 10.1186/s12974-019-1482-8
35. Rocha NP, Assis F, Scalzo PL, Vieira É LM, Barbosa IG, de Souza MS, et al. Reduced activated T lymphocytes (CD4+CD25+) and plasma levels of cytokines in Parkinson’s disease. Mol Neurobiol. (2018) 55:1488–97. doi: 10.1007/s12035-017-0404-y
36. Sun C, Zhao Z, Yu W, Mo M, Song C, Si Y, et al. Abnormal subpopulations of peripheral blood lymphocytes are involved in Parkinson’s disease. Ann Trans Med. (2019) 7:637. doi: 10.21037/atm.2019.10.105
37. Zhang Z, Xie X, Cai Y, Liu P, Liu S, Chen R, et al. Abnormal immune function of B lymphocyte in peripheral blood of Parkinson’s disease. Parkinsonism related Disord. (2023) 116:105890. doi: 10.1016/j.parkreldis.2023.105890
38. Zhao X, Jin T, Zheng C, Ma D, Zhang Y. Imbalance of circulating Tfh/Tfr cells in patients with Parkinson’s disease. Front Neurol. (2020) 11:572205. doi: 10.3389/fneur.2020.572205
39. Zhao X, Li L, Ma X, Li Y, Gao B, Luo W. The role of immune and inflammatory-related indicators in cognitive dysfunction and disease severity in patients with parkinson’s disease. J Neural Transm (Vienna Austria: 1996). (2024) 131:13–24. doi: 10.1007/s00702-023-02704-8
40. Niwa F, Kuriyama N, Nakagawa M, Imanishi J. Effects of peripheral lymphocyte subpopulations and the clinical correlation with Parkinson’s disease. Geriatrics gerontology Int. (2012) 12:102–7. doi: 10.1111/j.1447-0594.2011.00740.x
41. Horvath S, Ritz BR. Increased epigenetic age and granulocyte counts in the blood of Parkinson’s disease patients. Aging. (2015) 7:1130–42. doi: 10.18632/aging.100859
42. Houseman EA, Accomando WP, Koestler DC, Christensen BC, Marsit CJ, Nelson HH, et al. DNA methylation arrays as surrogate measures of cell mixture distribution. BMC Bioinf. (2012) 13:86. doi: 10.1186/1471-2105-13-86
43. Prinz M, Priller J. The role of peripheral immune cells in the CNS in steady state and disease. Nat Neurosci. (2017) 20:136–44. doi: 10.1038/nn.4475
44. Louveau A, Herz J, Alme MN, Salvador AF, Dong MQ, Viar KE, et al. CNS lymphatic drainage and neuroinflammation are regulated by meningeal lymphatic vasculature. Nat Neurosci. (2018) 21:1380–91. doi: 10.1038/s41593-018-0227-9
45. Zou W, Pu T, Feng W, Lu M, Zheng Y, Du R, et al. Blocking meningeal lymphatic drainage aggravates Parkinson’s disease-like pathology in mice overexpressing mutated α-synuclein. Trans neurodegeneration. (2019) 8:7. doi: 10.1186/s40035-019-0147-y
46. Herisson F, Frodermann V, Courties G, Rohde D, Sun Y, Vandoorne K, et al. Direct vascular channels connect skull bone marrow and the brain surface enabling myeloid cell migration. Nat Neurosci. (2018) 21:1209–17. doi: 10.1038/s41593-018-0213-2
47. Scott KM, Bloem BR, Brundin P, Tan EK, Harms A, Lindestam Arlehamn C, et al. B Lymphocytes in Parkinson’s Disease. J Parkinsons Dis. (2022) 12(S1):S75–S81.
48. Foley ÉM, Parkinson JT, Mitchell RE, Turner L, Khandaker GM. Peripheral blood cellular immunophenotype in depression: a systematic review and meta-analysis. Mol Psychiatry. (2023) 28:1004–19. doi: 10.1038/s41380-022-01919-7
49. Kedmi M, Bar-Shira A, Gurevich T, Giladi N, Orr-Urtreger A. Decreased expression of B cell related genes in leukocytes of women with Parkinson’s disease. Mol neurodegeneration. (2011) 6:66. doi: 10.1186/1750-1326-6-66
50. Tansey MG, Wallings RL, Houser MC, Herrick MK, Keating CE, Joers V. Inflammation and immune dysfunction in Parkinson disease. Nat Rev Immunol. (2022) 22:657–73. doi: 10.1038/s41577-022-00684-6
51. Nathan C, Ding A. Nonresolving inflammation. Cell. (2010) 140:871–82. doi: 10.1016/j.cell.2010.02.029
52. Rosser EC, Mauri C. Regulatory B cells: origin, phenotype, and function. Immunity. (2015) 42:607–12. doi: 10.1016/j.immuni.2015.04.005
53. Wang L, Xu D, Chu Y. Editorial: insights into regulatory B cells. Front Immunol. (2022) 13. doi: 10.3389/fimmu.2022.903711
54. Mauri C, Menon M. Human regulatory B cells in health and disease: therapeutic potential. J Clin Invest. (2017) 127:772–9. doi: 10.1172/JCI85113
55. Cencioni MT, Ali R, Nicholas R, Muraro PA. Defective CD19+CD24(hi)CD38(hi) transitional B-cell function in patients with relapsing-remitting MS. Multiple sclerosis (Houndmills Basingstoke England). (2021) 27:1187–97. doi: 10.1177/1352458520951536
56. Lin Y, Chang T, Lin J, Sun C, Wei C, Zhao J, et al. Regulatory B cells are decreased and functionally impaired in myasthenia gravis patients. Front Neurol. (2022) 13:808322. doi: 10.3389/fneur.2022.808322
57. Matsushita T, Hamaguchi Y, Hasegawa M, Takehara K, Fujimoto M. Decreased levels of regulatory B cells in patients with systemic sclerosis: association with autoantibody production and disease activity. Rheumatol (Oxford England). (2016) 55:263–7. doi: 10.1093/rheumatology/kev331
58. Sheng JR, Rezania K, Soliven B. Impaired regulatory B cells in myasthenia gravis. J neuroimmunology. (2016) 297:38–45. doi: 10.1016/j.jneuroim.2016.05.004
59. Zhu HQ, Xu RC, Chen YY, Yuan HJ, Cao H, Zhao XQ, et al. Impaired function of CD19(+) CD24(hi) CD38(hi) regulatory B cells in patients with pemphigus. Br J Dermatol. (2015) 172:101–10. doi: 10.1111/bjd.2015.172.issue-1
60. Mauri C, Bosma A. Immune regulatory function of B cells. Annu Rev Immunol. (2012) 30:221–41. doi: 10.1146/annurev-immunol-020711-074934
61. Xing C, Ma N, Xiao H, Wang X, Zheng M, Han G, et al. Critical role for thymic CD19+CD5+CD1dhiIL-10+ regulatory B cells in immune homeostasis. J leukocyte Biol. (2015) 97:547–56. doi: 10.1189/jlb.3A0414-213RR
62. Wolf SD, Dittel BN, Hardardottir F, Janeway CA Jr. Experimental autoimmune encephalomyelitis induction in genetically B cell-deficient mice. J Exp Med. (1996) 184:2271–8. doi: 10.1084/jem.184.6.2271
63. Fillatreau S, Sweenie CH, McGeachy MJ, Gray D, Anderton SM. B cells regulate autoimmunity by provision of IL-10. Nat Immunol. (2002) 3:944–50. doi: 10.1038/ni833
64. Benson MJ, Dillon SR, Castigli E, Geha RS, Xu S, Lam KP, et al. Cutting edge: the dependence of plasma cells and independence of memory B cells on BAFF and APRIL. J Immunol (Baltimore Md.: 1950). (2008) 180:3655–9.
65. Scholz JL, Crowley JE, Tomayko MM, Steinel N, O’Neill PJ, Quinn WJ 3rd, et al. BLyS inhibition eliminates primary B cells but leaves natural and acquired humoral immunity intact. Proc Natl Acad Sci U S A. (2008) 105:15517–22. doi: 10.1073/pnas.0807841105
66. Brooks JF, Zikherman J. The BAFFling persistence of memory B cells. J Exp Med. (2021) 218. doi: 10.1084/jem.20202057
67. Schweighoffer E, Tybulewicz VL. BAFF signaling in health and disease. Curr Opin Immunol. (2021) 71:124–31. doi: 10.1016/j.coi.2021.06.014
68. Magliozzi R, Marastoni D, Calabrese M. The BAFF/APRIL system as therapeutic target in multiple sclerosis. Expert Opin Ther Targets. (2020) 24:1135–45. doi: 10.1080/14728222.2020.1821647
69. Steri M, Orrù V, Idda ML, Pitzalis M, Pala M, Zara I, et al. Overexpression of the cytokine BAFF and autoimmunity risk. New Engl J Med. (2017) 376:1615–26. doi: 10.1056/NEJMoa1610528
70. Huntington ND, Tomioka R, Clavarino C, Chow AM, Liñares D, Maña P, et al. A BAFF antagonist suppresses experimental autoimmune encephalomyelitis by targeting cell-mediated and humoral immune responses. Int Immunol. (2006) 18:1473–85. doi: 10.1093/intimm/dxl080
71. Seifert M, Przekopowitz M, Taudien S, Lollies A, Ronge V, Drees B, et al. Functional capacities of human IgM memory B cells in early inflammatory responses and secondary germinal center reactions. Proc Natl Acad Sci U S A. (2015) 112:E546–55. doi: 10.1073/pnas.1416276112
72. Frasca D. Senescent B cells in aging and age-related diseases: Their role in the regulation of antibody responses. Exp gerontology. (2018) 107:55–8. doi: 10.1016/j.exger.2017.07.002
73. Wei C, Anolik J, Cappione A, Zheng B, Pugh-Bernard A, Brooks J, et al. A new population of cells lacking expression of CD27 represents a notable component of the B cell memory compartment in systemic lupus erythematosus. J Immunol (Baltimore Md.: 1950). (2007) 178:6624–33. doi: 10.4049/jimmunol.178.10.6624
74. Colonna-Romano G, Bulati M, Aquino A, Pellicanò M, Vitello S, Lio D, et al. A double-negative (IgD-CD27-) B cell population is increased in the peripheral blood of elderly people. Mech Ageing Dev. (2009) 130:681–90. doi: 10.1016/j.mad.2009.08.003
75. Bulati M, Buffa S, Martorana A, Gervasi F, Camarda C, Azzarello DM, et al. Double negative (IgG+IgD-CD27-) B cells are increased in a cohort of moderate-severe Alzheimer’s disease patients and show a pro-inflammatory trafficking receptor phenotype. J Alzheimer’s disease: JAD. (2015) 44:1241–51. doi: 10.3233/JAD-142412
76. Cancro MP. Age-associated B cells. Annu Rev Immunol. (2020) 38:315–40. doi: 10.1146/annurev-immunol-092419-031130
77. Liechti T, Kadelka C, Braun DL, Kuster H, Böni J, Robbiani M, et al. Widespread B cell perturbations in HIV-1 infection afflict naive and marginal zone B cells. J Exp Med. (2019) 216:2071–90. doi: 10.1084/jem.20181124
78. Moir S, Ho J, Malaspina A, Wang W, DiPoto AC, O’Shea MA, et al. Evidence for HIV-associated B cell exhaustion in a dysfunctional memory B cell compartment in HIV-infected viremic individuals. J Exp Med. (2008) 205:1797–805. doi: 10.1084/jem.20072683
79. Double KL, Rowe DB, Carew-Jones FM, Hayes M, Chan DK, Blackie J, et al. Anti-melanin antibodies are increased in sera in Parkinson’s disease. Exp Neurol. (2009) 217:297–301. doi: 10.1016/j.expneurol.2009.03.002
80. Yanamandra K, Gruden MA, Casaite V, Meskys R, Forsgren L, Morozova-Roche LA. [amp]]alpha;-synuclein reactive antibodies as diagnostic biomarkers in blood sera of Parkinson’s disease patients. PLoS One. (2011) 6:e18513.
81. Horvath I, Iashchishyn IA, Forsgren L, Morozova-Roche LA. Immunochemical detection of α-synuclein autoantibodies in Parkinson’s disease: correlation between plasma and cerebrospinal fluid levels. ACS Chem Neurosci. (2017) 8:1170–6. doi: 10.1021/acschemneuro.7b00063
82. Castonguay AM, Gravel C, Lévesque M. Treating Parkinson’s disease with antibodies: previous studies and future directions. J Parkinson’s Dis. (2021) 11:71–92. doi: 10.3233/JPD-202221
83. Volc D, Poewe W, Kutzelnigg A, Lührs P, Thun-Hohenstein C, Schneeberger A, et al. Safety and immunogenicity of the α-synuclein active immunotherapeutic PD01A in patients with Parkinson’s disease: a randomised, single-blinded, phase 1 trial. Lancet Neurol. (2020) 19:591–600. doi: 10.1016/S1474-4422(20)30136-8
84. Cancro MP, Tomayko MM. Memory B cells and plasma cells: The differentiative continuum of humoral immunity. Immunol Rev. (2021) 303:72–82. doi: 10.1111/imr.v303.1
85. Qin XY, Zhang SP, Cao C, Loh YP, Cheng Y. Aberrations in peripheral inflammatory cytokine levels in Parkinson disease: A systematic review and meta-analysis. JAMA Neurol. (2016) 73:1316–24. doi: 10.1001/jamaneurol.2016.2742
86. Morris HR, Spillantini MG, Sue CM, Williams-Gray CH. The pathogenesis of Parkinson’s disease. Lancet. (2024) 403:293–304. doi: 10.1016/S0140-6736(23)01478-2
87. Chen Z, Chen S, Liu J. The role of T cells in the pathogenesis of Parkinson’s disease. Prog Neurobiol. (2018) 169:1–23. doi: 10.1016/j.pneurobio.2018.08.002
88. Bloem BR, Okun MS, Klein C. Parkinson’s disease. Lancet (London England). (2021) 397:2284–303. doi: 10.1016/S0140-6736(21)00218-X
89. Kalia LV, Lang AE. Parkinson’s disease. Lancet (London England). (2015) 386:896–912. doi: 10.1016/S0140-6736(14)61393-3
90. Glass DR, Tsai AG, Oliveria JP, Hartmann FJ, Kimmey SC, Calderon AA, et al. An integrated multi-omic single-cell atlas of human B cell identity. Immunity. (2020) 53:217–32. doi: 10.1016/j.immuni.2020.06.013
91. Papalexi E, Satija R. Single-cell RNA sequencing to explore immune cell heterogeneity. Nat Rev Immunol. (2018) 18:35–45. doi: 10.1038/nri.2017.76
92. Siu JHY, Pitcher MJ, Tull TJ, Velounias RL, Guesdon W, Montorsi L, et al. Two subsets of human marginal zone B cells resolved by global analysis of lymphoid tissues and blood. Sci Immunol. (2022) 7:eabm9060. doi: 10.1126/sciimmunol.abm9060
Keywords: B lymphocyte, B lymphocyte subsets, Parkinson’s disease, Pathogenesis, Immunity, Inflammation
Citation: Ma H, Wang Z, Yu M, Zhai Y and Yan J (2025) Aberrations in peripheral B lymphocytes and B lymphocyte subsets levels in Parkinson disease: a systematic review. Front. Immunol. 16:1526095. doi: 10.3389/fimmu.2025.1526095
Received: 11 November 2024; Accepted: 10 March 2025;
Published: 31 March 2025.
Edited by:
Rosaria Talarico, ERN ReCONNET Coordination Team, ItalyReviewed by:
Jose-Noel Ibrahim, Lebanese American University, LebanonCopyright © 2025 Ma, Wang, Yu, Zhai and Yan. This is an open-access article distributed under the terms of the Creative Commons Attribution License (CC BY). The use, distribution or reproduction in other forums is permitted, provided the original author(s) and the copyright owner(s) are credited and that the original publication in this journal is cited, in accordance with accepted academic practice. No use, distribution or reproduction is permitted which does not comply with these terms.
*Correspondence: Junqiang Yan, eWFuanFAaGF1c3QuZWR1LmNu
Disclaimer: All claims expressed in this article are solely those of the authors and do not necessarily represent those of their affiliated organizations, or those of the publisher, the editors and the reviewers. Any product that may be evaluated in this article or claim that may be made by its manufacturer is not guaranteed or endorsed by the publisher.
Research integrity at Frontiers
Learn more about the work of our research integrity team to safeguard the quality of each article we publish.