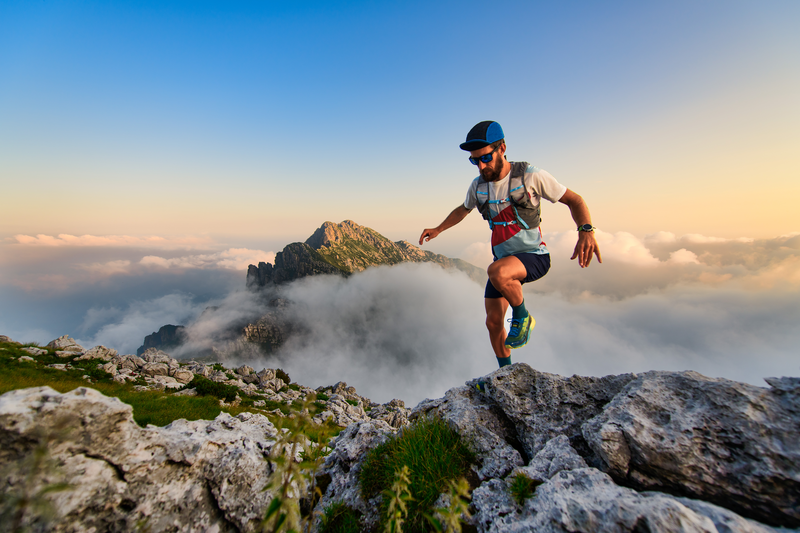
94% of researchers rate our articles as excellent or good
Learn more about the work of our research integrity team to safeguard the quality of each article we publish.
Find out more
REVIEW article
Front. Immunol.
Sec. Parasite Immunology
Volume 16 - 2025 | doi: 10.3389/fimmu.2025.1516176
This article is part of the Research Topic Interactions among Immune Cells in Leishmaniasis: Exploring Markers, Enzymes and Cytokines View all 3 articles
The final, formatted version of the article will be published soon.
You have multiple emails registered with Frontiers:
Please enter your email address:
If you already have an account, please login
You don't have a Frontiers account ? You can register here
Most cases of visceral leishmaniasis (VL) and human immunodeficiency virus (HIV) co-infection (VL/HIV) in the Americas occur in Brazil, and the prevalence of VL/HIV has been increasing since 2019, reaching 19% in 2023. This association presents a challenge for the control and elimination of VL, since both VL and HIV infection share immunopathogenic characteristics that can reciprocally affect co-infected patients. Thus, VL may contribute to the immunosuppression and other immunological disturbances associated with the rapid progression to acquired immunodeficiency syndrome (AIDS), whereas HIV infection accelerates the development of active VL and reduces the probability of a successful response to anti-Leishmania therapy, resulting in an increase in the relapse and lethality rates of VL. In this synergistic impairment, one of the most critical hallmarks of VL/HIV co-infection is the enhancement of immunosuppression and intense chronic immune activation, caused not only by each infection per se, but also by the cytokine storm and translocation of microbial products. Thus, co-infected patients present with an impaired effector immune response that may result in inefficient parasitic control. In addition, the chronic activation environment in VL/HIV patients may favor progression to early immunosenescence and exhaustion, worsening the patients’ clinical condition and increasing the frequency of disease relapse. Herein, we review the immunological parameters associated with the immunopathogenesis of VL/HIV co-infection that could serve as good biomarkers of clinical prognosis in terms of relapse and severity of VL.
Keywords: VL/HIV co-infection, Visceral leishmaniasis, immune response, Cellular activation, immunosenescence, relapses
Received: 23 Oct 2024; Accepted: 19 Feb 2025.
Copyright: © 2025 Silva-Freitas, Castro, Da-Cruz and Santos-Oliveira. This is an open-access article distributed under the terms of the Creative Commons Attribution License (CC BY). The use, distribution or reproduction in other forums is permitted, provided the original author(s) or licensor are credited and that the original publication in this journal is cited, in accordance with accepted academic practice. No use, distribution or reproduction is permitted which does not comply with these terms.
* Correspondence:
Joanna Santos-Oliveira, Instituto Federal de Educação, Ciência e Tecnologia - IFRJ, Rio de Janeiro, Brazil
Disclaimer: All claims expressed in this article are solely those of the authors and do not necessarily represent those of their affiliated organizations, or those of the publisher, the editors and the reviewers. Any product that may be evaluated in this article or claim that may be made by its manufacturer is not guaranteed or endorsed by the publisher.
Research integrity at Frontiers
Learn more about the work of our research integrity team to safeguard the quality of each article we publish.