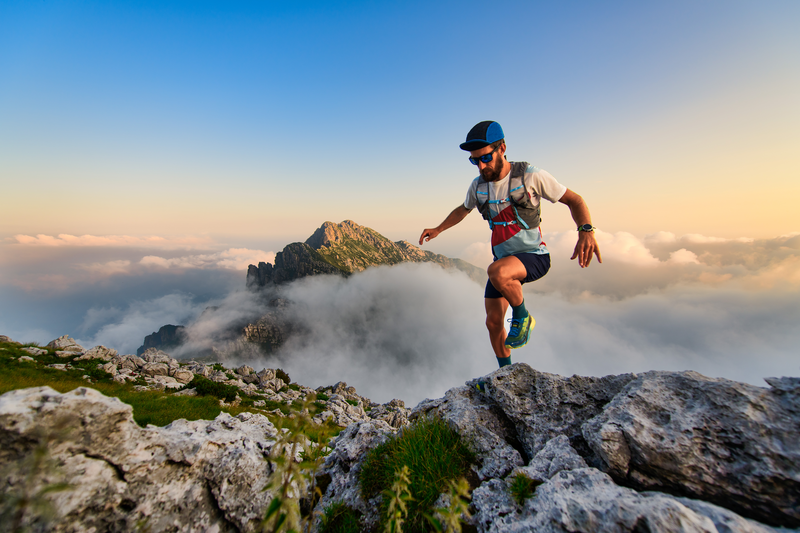
95% of researchers rate our articles as excellent or good
Learn more about the work of our research integrity team to safeguard the quality of each article we publish.
Find out more
ORIGINAL RESEARCH article
Front. Immunol.
Sec. Inflammation
Volume 16 - 2025 | doi: 10.3389/fimmu.2025.1511990
This article is part of the Research Topic Inflammation and Immune Factors in Venous Thromboembolism View all 5 articles
The final, formatted version of the article will be published soon.
You have multiple emails registered with Frontiers:
Please enter your email address:
If you already have an account, please login
You don't have a Frontiers account ? You can register here
Background: Our group recently reported that chymase, a serine protease synthetized and released by mast cells, plays a pivotal role in thrombi stabilization in murine deep vein thrombosis (DVT) models, by interfering with the fibrinolytic properties of endogenous plasmin within the thrombi. However, the impact of mast cell-derived chymase on endogenous plasmin activity in human blood clots has yet to be explored.The antifibrinolytic properties of human recombinant chymase (rCMA-1) were investigated using an in vitro thrombolysis assay based on halo-shaped human blood clots. In addition, a fluorogenic assay was used to detect chymase activity in human thromboembolic biopsies. In both assays, the activity of human chymase was validated using a specific chymase inhibitor, fulacimstat (BAY 1142524). Results: Although rapidly neutralized in plasma, rCMA-1 remains active within the local microenvironment of a blood clot, inducing resistance to endogenous plasmin-mediated fibrinolysis in the presence of recombinant tissue plasminogen activator (tPA). This leads to a concentration-Supprimé: dependent decrease in clot lysis. The plasmin-inactivating properties of rCMA-1 were inhibited by fulacimstat, resulting in an accelerated clot dissolution. The pro-fibrinolytic effects of fulacimstat in human blood clots were reversed by a plasminogen/plasmin inhibitor, BAY 1214237. Finally, fulacimstat-sensitive chymase activity was identified in human thrombus clots, emphasizing the potential role the mast cell-derived serine protease in human blot clot stabilization under pathological conditions.These in vitro experiments with human whole blood suggest that mast cell-derived chymase impairs blood clot resolution by interfering with the fibrinolytic activity of endogenous intraclot plasmin. Our findings provide evidence that recombinant mast cell chymase interferes with endogenous plasmin activity in human whole blood clots in vitro and support the potential of chymase inhibitors, such as fulacimstat, as fibrinolytic agents for thrombotic and thromboembolic disorders.
Keywords: chymase, Mast Cells, Fibrinolysis, Plasmin, fulacimstat, Thrombolysis assay, Human Blood Clots, Human Pulmonary Emboli Biopsies
Received: 15 Oct 2024; Accepted: 20 Mar 2025.
Copyright: © 2025 Vincent, Lapointe, McDonald, Rammos, Rassaf, Kollenberger, Tinel, Heitmeier and D'Orléans-Juste. This is an open-access article distributed under the terms of the Creative Commons Attribution License (CC BY). The use, distribution or reproduction in other forums is permitted, provided the original author(s) or licensor are credited and that the original publication in this journal is cited, in accordance with accepted academic practice. No use, distribution or reproduction is permitted which does not comply with these terms.
* Correspondence:
Pedro D'Orléans-Juste, Université de Sherbrooke, Sherbrooke, Canada
Disclaimer: All claims expressed in this article are solely those of the authors and do not necessarily represent those of their affiliated organizations, or those of the publisher, the editors and the reviewers. Any product that may be evaluated in this article or claim that may be made by its manufacturer is not guaranteed or endorsed by the publisher.
Research integrity at Frontiers
Learn more about the work of our research integrity team to safeguard the quality of each article we publish.