- Department of Rheumatology, Research Center of Clinical Medicine, Research Center of Clinical Immunology, Affiliated Hospital of Nantong University, Medical School of Nantong University, Nantong University, Nantong, China
Systemic lupus erythematosus (SLE) is a chronic, inflammatory, and progressive autoimmune disease. The unclear pathogenesis, high heterogeneity, and prolonged course of the disease present significant challenges for effective clinical management of lupus patients. Dysregulation of the immune system and disruption of immune tolerance, particularly through the abnormal activation of B lymphocytes and the production of excessive autoantibodies, lead to widespread inflammation and tissue damage, resulting in multi-organ impairment. Currently, there is no systematic review that examines the specificity of B cell characteristics and pathogenic mechanisms across various organs. This paper reviews current research on B cells in lupus patients and summarizes the distinct characteristics of B cells in different organs. By integrating clinical manifestations of organ damage in patients with a focus on the organ-specific features of B cells, we provide a new perspective on enhancing the efficacy of lupus-targeted B cell therapy strategies.
1 Introduction
Systemic lupus erythematosus (SLE) is a systemic autoimmune disease that affects multiple organ systems. It is characterized by the abnormal activation of lymphocytes, disruption of immune tolerance, and production of autoantibodies (1–6). The high heterogeneity of the disease, its diverse features, and the alternating stages of onset and remission create significant challenges for effective management of lupus patients (4). Patients with lupus experience a range of issues, including sleep disorders, sexual dysfunction, fertility concerns, periodontal disease, and secondary osteoporosis (7–12). Additionally, anxiety, depression, and other emotional disorders in lupus patients relate to the abnormalities in lymphocyte function and inflammation (13–15). These adverse factors severely impact the prognosis and quality of life for lupus patients, sometimes leading to suicidal ideation and behavior (16). Therefore, gaining a comprehensive understanding of the pathogenesis of lupus is crucial.
An imbalance in immune systems, along with genetic factors, infections, and other influences, can trigger the onset of lupus. Various inflammatory mediators, dysregulated adaptive immune responses, and impaired immune tolerance contribute to abnormal cytokine secretion, as well as disrupted intracellular and intercellular signaling. These disruptions lead to impaired recruitment and activation of B lymphocytes, which ultimately participate in the onset and progression of lupus (17, 18). Autoreactive B cells produce excessive amounts of autoantibodies and pro-inflammatory cytokines, initiating an inflammatory cascade and immune response that ultimately induce organ damage in patients (19). Notably, these autoantibodies can emerge several years before the clinical symptoms of lupus appear (20, 21).
Given the crucial role of B cells in lupus, scientists and medical experts have actively explored treatment strategies targeting these cells. Clinically, non-specific treatments such as glucocorticoids and immunosuppressive drugs are widely employed. In recent years, lupus patients have also received treatment with targeted biological agents related to B cells, including belimumab, anifrolumab, and telitacicept, as well as CAR-T therapy strategies (22–25). However, many patients do not benefit from these therapies. SLE is a systemic, diffuse autoimmune disease with variable multi-organ and multi-system involvement, and B cells may exhibit distinct phenotypes and functions in different tissues and organs (Table 1). Therefore, understanding B cell heterogeneity across organs and further elucidating the pathogenesis of lupus may unlock new therapeutic targets and improve treatment outcomes, which is essential for improving organ damage and prognosis in lupus patients. This review will focus on the development and differentiation pathways of B cells in lupus patients and their specific characteristics in different organs, providing evidence for potential therapies (Figure 1).
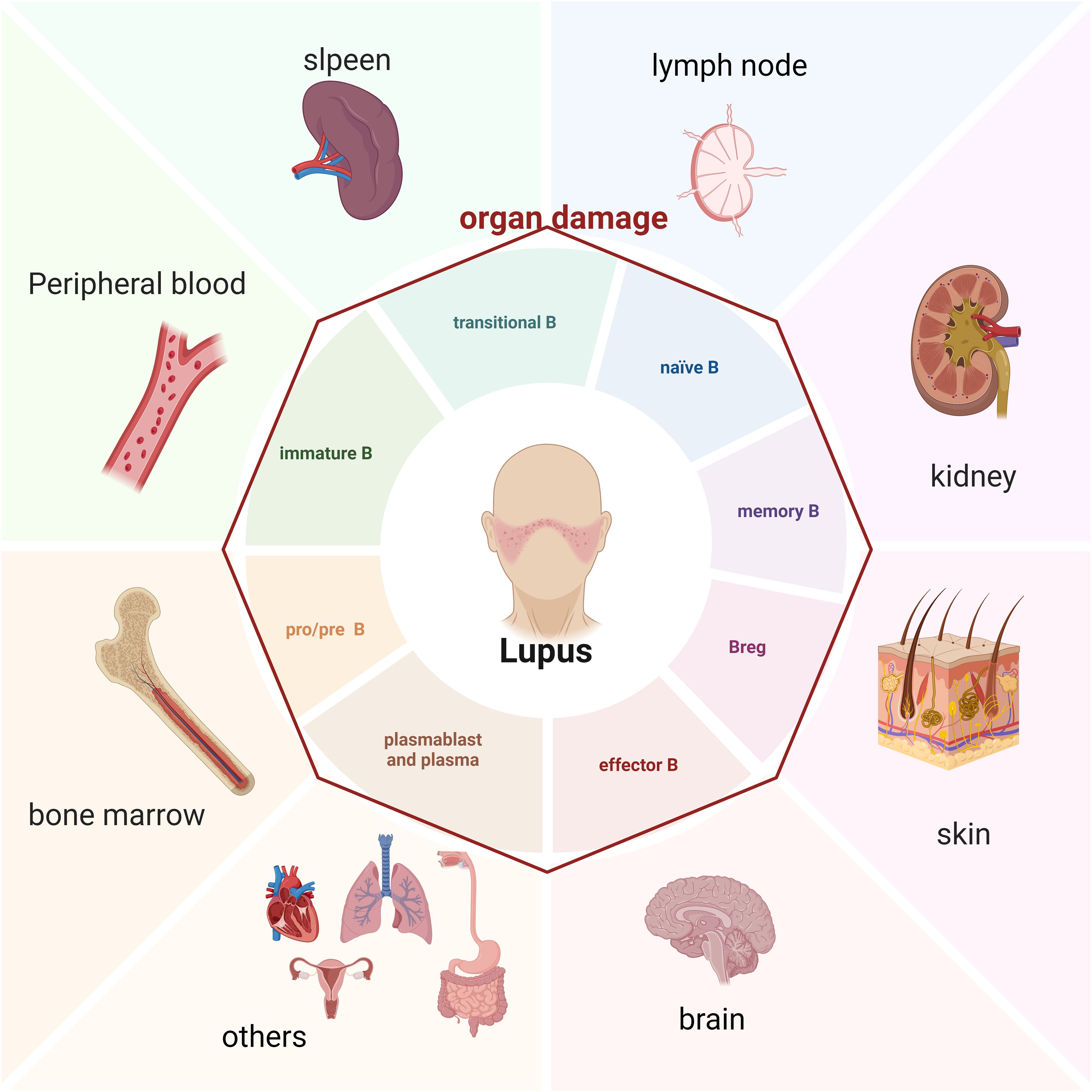
Figure 1. B cell and damaged organ in SLE patients (Created in BioRender. Dong, C. (2025) https://BioRender.com/s25g537).
2 Imbalance of B-cell immune tolerance of lupus patients
The adaptive immune system can recognize multiple pathogens and induce specific immune responses, thereby providing effective immune defense. During this process, adaptive immune cells, particularly B cells, may exhibit potential auto-reactivity. To manage this risk, the body has evolved mechanisms of immune tolerance to monitor auto-reactive cells, preventing them from becoming pathogenic effector cells and thereby avoiding autoimmune diseases such as lupus.
The control mechanisms for B cell development can be categorized into central immune tolerance and peripheral immune tolerance, depending on the stage and location of development. Central immune tolerance eliminates 50% to 75% of auto-reactive immature B cells generated in the bone marrow through processes such as clonal deletion, receptor modification, or receptor editing [the rearrangement of new light chains and their substitution on the original B-cell receptor (BCR)]. Tolerance is also induced on B cells by down modulation of BCRs (26). Despite this, a significant number of auto-reactive B cells (20% to 40%) escape central tolerance and migrate to the periphery, where they exist as transitional and naïve B cells (27). In the peripheral compartment, immune tolerance predominantly regulates the affinity maturation of auto-reactive B cells and eliminates those that strongly respond to self-antigens through clonal inactivation and clearance. Key mechanisms of peripheral immune tolerance include BCR signaling transduction, VDJ gene rearrangement, competitive inhibition of BAFF, and the induction of auto-reactive B cell migration to the periphery (28–30). Dysregulated BCR signaling initiates autoimmunity by modulating the naïve BCR repertoire during the development of immature and transitional B cells, and by promoting the activation of autoreactive B cell clones (31). VDJ gene usage is significantly biased of SLE patients, particularly in CD27+IgD+ unswitched memory B cells and plasmablast (32). The levels of BAFF are commonly overexpressed in SLE, which support the survival of autoreactive B cells and prevent their deletion. Overexpression of BAFF in mice may result in the development of an SLE-like disease, such as polyclonal B cell proliferation, production of multiple autoantibody, and increased circulation of immune complexes and renal immunoglobulin deposition (33).
Auto-reactive B cells may evade the monitoring mechanisms of central and early peripheral immune tolerance and subsequently enter the germinal center. Within the germinal center, IgG+ memory B cells can recover the quantity or type of cells previously eliminated during central immune tolerance through high-frequency somatic mutations and other processes. Non-auto-reactive B cells can also acquire self-reactivity, highlighting the crucial role of germinal center immune checkpoints in regulating auto-reactive plasma cells (34, 35). Numerous studies using animal models demonstrate that germinal center immune checkpoints function through various pathways, including follicular rejection, apoptosis mediated by FAS, inhibition via FcyRIIB, receptor modification, follicular dendritic cells, regulatory T cells, and follicular regulatory T cells (36–40). Furthermore, organized aggregates of immune cells formed in non-lymphoid tissues, such as tertiary lymphoid organs and other ectopic structures, may contribute to autoimmune diseases by failing to respond to tissue-specific auto-reactive B cells or even promoting their expansion (41, 42). The extra-follicular pathway represents another special pathogenic mechanism, which has been implicated in the pathogenesis of lupus in both mice and humans, particularly in the progression of LN (41, 43, 44). Thus, an imbalance in immune tolerance, including functional abnormalities in central, early peripheral, and late peripheral immune checkpoints, may lead to the expansion of auto-reactive B cells, resulting in the development of autoimmune diseases such as lupus.
The mechanisms underlying the disruption of immune tolerance remain unclear. Currently, researchers commonly use single-cell transcriptome sequencing, single-cell BCR sequencing, and flow cytometry with the 9G4 antibody to characterize lupus-specific autoantibodies encoded by the immunoglobulin heavy chain gene VH4-34. These methods help measure auto-reactivity and reflect the landscape of human B-cell immune tolerance. Healthy individuals rarely produce 9G4+ class-switched B cells; however, lupus patients with high disease activity exhibit significantly elevated levels of 9G4+ IgG in serum, along with an enrichment of VH4-34 in antibody-secreting cells (45, 46). Furthermore, VH4-34 accounts for a substantial proportion of naïve B cells (47), with levels reaching up to 2% to 10%. The 9G4 antibody can generate self-reactivity against dsDNA, apoptotic cells, and other self-antigens associated with lupus (48, 49). Studies indicate that somatic hypermutations can produce amino acid changes in the hydrophobic residues of VH4-34 or enhance glycosylation to mask these hydrophobic residues, which may help reduce auto-reactivity. Although these mutations can diminish some auto-reactivity, VH4-34+ B cell and plasma cell clones continue to undergo high-frequency amplification due to their strong auto-reactivity to various self-antigens. Thus, merely clearing VH4-34+ B cells is insufficient to suppress lupus-specific self-reactivity (46, 48, 50). In healthy individuals, the germinal center effectively inhibits 9G4+ B cells, whereas lupus patients exhibit high expression levels of these cells, producing large quantities of autoantibodies. The failure to clear VH4-34 alone underscores the impaired immune tolerance in lupus patients, making effective control of the disease particularly challenging (51).
3 B cells in different organs and tissues of lupus
3.1 Abnormal development of B cells in bone marrow may be the initiating factor for lupus
The development and differentiation of B cells begin in the bone marrow. Histopathological changes observed in the bone marrow, along with abnormal quantitative and qualitative features of hematopoietic stem cells, indicate that the bone marrow is one of the primary damaged organs in lupus patients (52, 53). In active lupus patients, CD34+ hematopoietic stem/progenitor cells are fewer than in healthy donors. These cells show high expression of apoptosis-related markers and tend to differentiate into bone marrow stromal cells (54, 55). Furthermore, the diversity of the BCR-H pool and the length of the BCR-H complementarity-determining region 3 (CDR3) in peripheral blood mononuclear cells from lupus patients are significantly reduced. Additionally, pre-selection BCR-H CDR3 sequences in lupus patients exhibit abnormal shortening, suggesting that the early development of bone marrow B cells and the BCR repertoire are compromised in these individuals (56).
Recent studies in mice have enhanced our understanding of how several pro-inflammatory cytokines, including interferon (IFN)-I, tumor necrosis factor (TNF)-α, and interleukin (IL)-6, inhibit the development and generation of lupus B cells (57–60). For instance, knocking out EZH2 in B cells downregulates the key transcription factor XBP1, which impairs the differentiation of plasmablasts in MRL/lpr mice. This impairment reduces autoantibody production and improves the progression of glomerulonephritis (61). Additionally, the drug Ophiopogonin D can enhance disease progression in MRL/lpr mice by decreasing the number of CD19+ B cells in both bone marrow and peripheral circulation (62). However, due to the invasiveness of bone marrow puncture in humans, research on bone marrow B cells in lupus patients remains limited.
In a case report involving a lupus patient with progressive multifocal leukoencephalopathy, researchers observed a significant reduction in bone marrow B cells, along with mutations in the GATA2 and CDH7 genes (63). These genes are closely associated with the proliferation, differentiation, and functional maintenance of B cells. Palanichamy et al. reported that inhibition of early B cell development correlates strongly with elevated interferon levels in the bone marrow (58). Using a combination of single-cell RNA sequencing and BCR sequencing, Dong et al. revealed developmental disorders in early B cells (pro-B and pre-B) in lupus patients. These disorders are linked to increased disease activity, heightened interferon signaling, systemic and local inflammatory activation, oxidative phosphorylation, and other overactivated metabolic pathways. Notably, the abnormal features of bone marrow B cells can be reversed once the patient achieves clinical remission (64–66). Additionally, researchers noted elevated levels of atypical memory B cells and long-lived plasma cells in the bone marrow of lupus patients (64). Overall, existing research on the development, differentiation, pathogenesis, and targeted therapy of bone marrow B cells in lupus patients is relatively limited. Therefore, further in-depth investigations are essential.
3.2 Abnormal circulating B cells in lupus patients are associated with disease activity
Lupus patients exhibit abnormal manifestations of B cells, characterized by an imbalance in B cell subtypes. Notably, there is an increased number of memory B cells relative to immature B cells, alongside excessive BCR responses, heightened calcium influx due to receptor cross-linking, and increased tyrosine phosphorylation of downstream signaling molecules (67). Abnormal BCR signaling can increase the risk of lupus development: This enhanced signal transduction mediated by BCR can lower the activation threshold for peripheral B cells, leading to a disordered lupus B cell phenotype (68). The BCR signaling pathways (VAV2, PLC-γ2) is significantly upregulated, affecting calcium influx and cytoskeletal remodeling (69). Abnormal calcium influx mediated by BCR may interfer RAG expression and immunoglobulin receptor editing, which contributes to the persistence of autoreactive B cells and the progression of lupus (70). Additionally, B cells from lupus patients show elevated rates of somatic hypermutations and class switching recombination, which contribute to the pathogenicity of plasma cells (46, 71, 72). To identify various B cell phenotypes, researchers use flow cytometry based on surface markers. In clinical practice, flow cytometry is a key tool used in B cell research, which allows absolute and relative B cell counts, and enables the assessment of B cell phenotype and function at the single-cell level, while allowing the analysis of large cell populations (73). Studies have reported that certain B cell subsets, including regulatory B cells (Breg, defined as CD19+CD24hiCD27+)↓, plasma cells↑, CXCR5- immature B cells↑, double-negative B cells (DNB, IgD-CD27-)↑, age-associated B cells (ABC, defined as CD11chiT-bet+)↑, unswitched memory B cells (UMBC, CD27+IgD+)↓, CD19+Siglec-10+ B cells↑, and CD226+ B cells↑ in lupus patients (74–76). Notably, lupus patients have a reduced number of Breg cells, which demonstrate functional impairments. The proportion of Bregs is negatively correlated with SLE disease activity index (SLEDAI) score, but positively correlated with the level of serum C3 and C4 in patient with SLE (77). These cells exhibit decreased responses to CD40 stimulation and lower IL-10 secretion, creating barriers to inhibiting immune responses, maintaining immune tolerance, and restoring immune system homeostasis (18, 78). ABCs can persist in tissues and rapidly differentiate into antibody-secreting cells upon re-exposure to the antigens or innate stimuli. ABCs exhibit upregulation of B cell signaling, lipid and glucose metabolism, and endocytosis in lupus (79). The proportion of ABCs, CD19+Siglec-10+ B cells, and CD226+ B cells are positively correlated with SLEDAI score and anti-dsDNA titers, but negatively correlated with serum complement levels (75, 80). The number of CD27+IgD+ B cells is significantly reduced in SLE patients, with impaired production of natural antibody-like IgM and IL-10, and defective clearance of apoptotic cells. These cells correlate positively with white blood cell (WBC), blood platelet (PLT), and serum C3 levels, but negatively with serum creatinine levels, SLEDAI score, and anti-dsDNA titers (81).
The abnormal number and function of B cells in lupus are regulated by key factors such as BAFF, serum soluble B cell maturation antigen (BCMA), Toll-like receptors (TLRs), IL-6, and IL-21 (20, 82–86). Abnormal upregulation of recombinant activated genes in the peripheral blood B cells of lupus patients can lead to BCR mutations, resulting in the production of self-reactive B cells (87). A significant correlation exists between BCMA levels and various indicators of disease activity, including antinuclear antibody (ANA) titers, anti-dsDNA titers, immunoglobulin levels, and hematological parameters in lupus patients (88). BCMA may serve as a biomarker and a potential therapeutic target for SLE. BAFF promotes the survival, proliferation, antigen presentation, and differentiation of self-reactive B cells by binding to its receptors, including transmembrane activators and calcium modulators (TACI), BCMA, and other ligands (89). Belimumab, a therapeutic anti-BAFF monoclonal antibody, may demonstrate clinical efficacy in SLE patients through various mechanisms (31): belimumab binds to soluble BAFF and inhibits it from binding to its receptors (BAFF receptor, TACI, and BCMA), reducing activation of early B cells and differentiation into memory B cells and antibody-producing plasma cells (90, 91); belimumab significantly reduces expression of BCMA on the surface of immature B cells, non-class switched and class-switched memory B cells (92). TLRs play a crucial role in B cell activation; BCR-driven immune complex uptake stimulates TLR-7 and TLR-9, which further activates the type I interferon response in B cells and enhances the production of autoantibodies, contributing to the pathogenicity of lupus (93). TLR-7 is expressed in various B cell subtypes and causes chronic stimulation of endogenous RNA through an autophagy-dependent mechanism, leading to further upregulation of TLR7 and B cell activation (94, 95). In lupus patients, immature B cells are regulated by sensors of viral RNA such as TLR-7 and can differentiate into extra-follicular double-negative memory B cells (CXCR5-CD11c+). These cells exhibit high expression of interferon-related genes and undergo significant amplification, ultimately differentiating into plasma cells and contributing to the pathogenesis of lupus (96). TLR-9 signaling is essential for autoantibody production in mice and enhances the differentiation of B cells and plasma cells that produce autoantibodies in humans (97). TLRs are considered potential promising therapeutic targets in SLE. Using TLR antagonists or anti-TLR monoclonal antibodies to selectively prevent extracellular or endosomal TLR ligation has become an attractive treatment strategy for SLE, including TLR7 blockers (IRS661, DS-7011a), TLR7/8/9 antagonists (chloroquine), TLR7/8 blockers (Afimetoran, Enpatoran, E6742) and TLR2/4 inhibitors (vitamin D3) (98, 99). Additionally, abnormal interactions between T cells and B cells, such as shortened interaction times in the germinal center, can lead to insufficient signaling for self-reactive B cells, thereby increasing their survival rates (100).
3.3 Splenic and lymph node B cell abnormalities unlock new therapeutic targets for lupus
As secondary lymphoid organs, spleen and lymph nodes play a crucial role in regulating immune tolerance, particularly in the pathogenesis of lupus. Splenomegaly and lymphadenopathy are important indicators of pathological damage in SLE. It is reported that approximately 60% of SLE patients present with symptoms of localized or generalized lymphadenopathy at some stage of disease progression (101). Nakatani K et al. (102) found that the number of splenic B cells decreased in an age-dependent manner in MRL/lpr mice, with a reduced expression of their inhibitory receptors FcγRIIb1 and CD22. A functional defect of these receptors exacerbated autoimmune symptoms in mice models. The proportion of MZB1+ MZ B cells and MZB1+ plasma cells was increased both in lupus patients and lupus-prone (NZB x NZW)F (1) [BWF (1)] mice. MZB1, a B cell-specific and endoplasmic reticulum (ER)-localized protein, is a key participant in antibody secretion. MZB1 may enhance lupus disease progression by modulating Ca2+ homeostasis and IgM production of B cells (101). In imiquimod-induced lupus mouse model, imiquimod activated TLR7 receptor, activating downstream MyD88 and TRAF6, and releasing mitochondrial dsDNA and dsRNA through MyD88 and TRAF6 to further activate of MDA5 and cGAS pathways in splenic B cells (103). These intracellular signaling pathways activated B cells and increased autoantibody production.
In recent years, a series of diagnostic and therapeutic strategies of targeting spleen and lymph node B cells have been developed in lupus. TIGIT-Fc fusion protein suppressed autoantibody production through the regulation of SPI-B-PAX5-XBP1 axis-mediated B-cell differentiation. In MRL/lpr mice, TIGIT-Fc fusion protein decreased the proportion of B220+ B cells, plasmablasts, and plasma cells, while increased the proportion of naïve B cells. In cGVHD mice, the proportion and number of B cells were reduced, including plasmablasts, germinal center (GC) B cells, and memory B cells with the treatment of TIGIT-Fc fusion protein (104). AIM2 was highly expressed in B cells of lupus patients and mice models. AIM2 deficiency in B cells alleviated lupus symptoms and reduced the frequency of CD19+ cells in lymph nodes and spleen, as well as splenic GC B cells and plasmablasts/plasma cells of lymph node via Blimp1-Bcl-6 axis in pristane-induced lupus mice (105). Currently, researches on spleen and lymph node B cells of lupus mainly focus on animal models, and large clinical trials are still lacking, requiring more investigation in the further.
3.4 Local infiltration of B cells exacerbates renal tissue damage in lupus patients
The kidney is a vital organ affected by lupus, with approximately 50% of lupus patients developing LN, which can result in kidney failure or even death. The primary pathological feature of LN is the production of autoantibodies against nuclear and cellular antigens, leading to the deposition of immune complexes in the glomerulus. Glomerular situ immune complex is formed by secondary binding to nucleosomes from renal cells, leading to widespread acute renal damage and loss of nephron units. which ultimately results in chronic, irreversible kidney dysfunction (106). Additionally, the deposition of immune complexes activate the complement system, and complement factors can directly induce immune complex–related renal inflammation and immunopathology (107). Various subtypes of B cells have been characterized as playing significant roles in the onset and progression of LN. Notably, the proportion of IFNβ+ naïve B cells show a strong positive correlation with the incidence of LN and the deposition of immune complexes in the glomerular basement membrane (108). Additionally, TLR4+CXCR4+ B cells have been implicated in driving the development of LN (109).
Reports indicate that more than half of LN patients exhibit abnormal infiltration of B cells in renal tissue (110). Abnormalities in ion channels are associated with the involvement of B cells in the pathogenesis of LN. B cells infiltrating the renal tissue of LN patients activate tissue adaptation programs in response to sodium stress, which upregulates Na+-K+-ATPase by renal epithelial cells, and moves three Na+ molecules extracellularly and two K+ molecules intracellularly against their concentration gradients. The process promotes the survival of pathologic B cells infiltrating the renal tissue under hyperosmolar conditions and aggravates the production of pathologic proteinuria (111). Inhibiting Na+ and K+ ATPase can reduce the number of infiltrating B cells and improve proteinuria levels in mouse models (111, 112). Using single-cell sequencing, researchers investigated the cell profile of renal biopsy tissue from lupus patients, enhancing our understanding of immune cell infiltration in LN and offering new insights into its pathogenesis, clinical diagnosis, and treatment. Tang et al. reported that the proportion of B cells in kidney tissue from LN patients reached 9.91%, compared to 6.79% in healthy controls. Additionally, the absolute quantity of B cells was significantly higher, with 84% in LN patients versus 16% in controls (113). Furthermore, Der et al. (114, 115) identified highly expressed interferon (IFN)-related genes in the renal parenchymal cells of LN patients through single-cell sequencing, however, they detected almost no B cells or lymphocytes in their study (116).
Additionally, we observed an increase in atypical memory B cells and long-lived plasma cells (LLPC), both of which play significant pathogenic roles in LN. Long-lived plasma cells infiltrate the renal tissue of LN patients, where they produce autoantibodies that form immune complexes, activating neutrophils and dendritic cells and ultimately leading to renal inflammation (117). Moreover, CD24-CD20hi atypical memory B cells are significantly elevated in lupus patients and tend to aggregate in renal tissue, correlating with the severity of the disease (79). Single-cell sequencing of renal tissue from LN patients revealed that B cells also express high levels of age-associated markers. This characteristic differs from that of B cells found in the peripheral blood of these patients (44). The specific infiltration of B cells in renal tissue may drive an in situ immune response, contributing to tissue damage in lupus patients. Research indicates that the activated mTORC1 pathway is involved in the differentiation of atypical memory B cells. This differentiation enhances BCR activation and leads to metabolic dysregulation, which ultimately increases autoantibody secretion and promotes disease progression (79). Furthermore, ZEB2, a transcription factor, facilitates the formation of age-associated B cells (atypical memory B cells) (85). The infiltration of pathogenic B cells significantly contributes to local tissue damage in the kidneys of lupus patients. Depleting renal ABCs has been shown to alleviate lupus disease in the MRL/lpr lupus model (118).
3.5 B cell infiltration is involved in skin lesions of lupus patients
Approximately 70% of patients with SLE experience skin involvement, with about 20% presenting skin lesions as their initial manifestation. Skin lesions, such as butterfly-shaped erythema on the face, serve as specific indicators of lupus disease activity. In addition to these characteristic lesions, lupus patients may exhibit non-specific skin injuries, including thrombophlebitis, hair loss, Raynaud’s phenomenon, cutaneous ulceration, necrosis, and subungual splinter hemorrhages. Lupus can be classified into cutaneous lupus erythematosus and systemic lupus erythematosus based on the presence of affected organs beyond the skin (119). Lupus-related skin lesions are categorized into three types: acute cutaneous lupus erythematosus, subacute cutaneous lupus erythematosus, and chronic cutaneous lupus erythematosus (120). Notably, approximately 50% of cases of subacute cutaneous lupus erythematosus may progress to systemic lupus erythematosus, resulting in damage to multiple organs as the disease advances (120). Researchers primarily attribute these lesions to environmental factors, such as ultraviolet radiation, as well as imbalances in immune homeostasis. For instance, cytotoxic T cells may target keratinocyte apoptosis, while infiltrating neutrophils and plasma cell-like dendritic cells produce large amounts of interferon, contributing to the development of lupus-related cutaneous lesions (121).
In recent years, researchers have increasingly recognized the role of B cells in lupus-related cutaneous lesions. Reports indicate that lupus patients experience imbalanced B cell homeostasis; however, those with cutaneous lupus erythematosus display decreased levels of double negative B cells and increased levels of unswitched B cells compared to patients with systemic lupus erythematosus (122). Compared to healthy donors, lupus patients show significantly elevated expression of BAFF in both skin and serum. Specifically, the proportion of BAFF+ cells in the skin of lupus patients averages around 6.945 ± 1.386%, while in healthy donors, it is only 1.650 ± 0.884%. BAFF primarily localizes to the dermis, with minimal expression found in the epidermis (123). The infiltration of immune cells in the dermis and the activation of BAFF contribute to the pathological progression of lupus lesions.
In addition to BAFF, receptors such as BAFF-R, BCMA, and TACI are highly expressed in the skin of patients with subacute cutaneous lupus erythematosus and discoid lupus erythematosus, linking these receptors to pro-inflammatory mediators (124). Regarding the infiltration characteristics of different B cell subgroups, the average infiltration degree of CD20+ B cells is 17%. For BAFF-sensitive B cells, CD20+BAFF-R+ cells constitute 13%, while CD20+BAFF-R+MHC-II+ (antigen-presenting, BAFF-sensitive B cells) make up 12%. CD20+BAFF-R+MHC-II+CD80/86+ B cells (antigen-presenting, T cell-activated, BAFF-sensitive B cells) represent 1%. In terms of morphological characteristics, B cells can appear clustered or loosely arranged, and they may exhibit features of diffuse infiltration as individual cells (125).
Regarding the mechanisms of B cell involvement in lupus lesions, studies have shown that autoreactive B cells can be recruited into the tissue in response to inflammatory signals such as interferon, IL-21, and TLR7/9. This recruitment leads to the infiltration of CD20+ B cells and the formation of tertiary lymphoid structures in the skin, resulting in the secretion of large amounts of immunoglobulin and autoantibodies. These factors may further exacerbate local tissue inflammation and contribute to the progression of lupus (126–130). In a study involving 150 patients with lupus erythematosus, researchers observed that, compared to patients with subacute and acute cutaneous lupus erythematosus, those with discoid lupus erythematosus exhibited increased local infiltration and transcriptional activity of B cells in the tissue. Additionally, the infiltration of B cells, particularly memory B cells, in the skin lesions of patients with cutaneous lupus erythematosus was higher than that seen in patients with systemic lupus erythematosus. However, there was no significant difference in B cell infiltration in the skin of systemic lupus erythematosus patients compared to healthy donors. Interestingly, positive circulating autoantibodies, such as anti-nuclear antibodies and double-stranded DNA antibodies, in patients with cutaneous lupus did not correlate with B cell infiltration in skin lesions. These findings suggest that B cells exert pathogenic effects not only by secreting antibodies in skin lesions but also by producing antibodies that may not enter the circulation, indicating a strong organ heterogeneity in their function (131).
3.6 B cells and structural or functional brain damage in lupus patients
Neuropsychiatric lupus (NPSLE) is a complication involving the central nervous system in lupus patients, making it challenging to diagnose and treat. This condition primarily presents as headaches, anxiety, depression, and chronic cognitive dysfunction. Currently, the diagnosis of NPSLE relies mainly on scale assessments and brain magnetic resonance imaging (MRI). Scale assessments indicate that lupus patients experience a high incidence of anxiety and depression, alongside impaired cognitive function (132, 133). Imaging evaluations reveal compensatory reorganization of neural circuits in these patients, which may indicate adaptive or extensible neuroplasticity (134). Compared to healthy individuals, lupus patients exhibit abnormal blood flow perfusion in multiple brain regions, with significantly reduced average flow velocities observed in the hypothalamus, putamen, right posterior thalamus, and right anterior insula. Additionally, lupus patients show a marked increase in high-signal areas in the white matter related to perfusion indicators, such as cerebral blood flow, cerebral blood volume, and blood-brain barrier leakage parameters compared to normal white matter (135). The extensive leakage of the blood-brain barrier and the structural brain damage identified in lupus patients through imaging are significantly correlated with declines in cognitive function (136).
Due to the significant challenges in acquiring brain tissue from patients, current research on the mechanisms of central nervous system damage in lupus primarily focuses on analyzing cerebrospinal fluid and blood samples. Only a limited number of studies have reported pathological changes in the brain tissue of these patients (137, 138). Vascular lesions represent a key pathological feature of brain damage in lupus patients. These lesions include extensive micro-infarctions, lymphocytic perivascular infiltrates, atrophy, microbleeds, white matter hyperintensities, ischemic demyelination, and small subcortical infarcts (138, 139). An autopsy case report of a patient with NPSLE indicated that aggregates of white blood cells can lead to small vessel occlusions (140).
Studies using model animals indicate that B cells and autoantibodies can traverse the blood-brain barrier, promote an inflammatory microenvironment, and activate microglia. However, evidence supporting the presence of B cells in the central nervous system of lupus patients remains limited. In the cerebrospinal fluid and blood samples of lupus patients, high levels of cytokines such as BAFF and APRIL, along with the production of intrathecal IgG, are considered important indicators of B cell activation involved in brain tissue damage (141, 142). Injecting serum IgG from lupus patients into the brains of mice can induce microglial inflammation via the Fc signaling pathway. This finding suggests that elevated serum immunoglobulin levels may contribute to brain damage in lupus patients (143). In patients experiencing disease flares, particularly those with NPSLE, the expression of the chemokine receptor CXCR4 on B cells increases (144). Furthermore, the expression of brain-derived neurotrophic factor precursor (proBDNF) in peripheral antibody-secreting cells (ASCs, defined as CD19+CD27hiCD38hi) is significantly upregulated, and the presence of proBDNF+ ASCs closely correlates with disease activity in lupus patients (145). Dong et al. also reported a significant increase in ASCs in the peripheral blood of depressed lupus patients (13). Additionally, various autoantibodies, such as anti-NMDAR antibodies, anti-ribosomal P antibodies, and anti-phospholipid antibodies, are highly expressed in the cerebrospinal fluid of NPSLE patients (146). However, some studies have shown increased blood-brain barrier permeability, brain cell apoptosis, and high levels of cytokines in B cell-depleted mice. This suggests that B cells and autoantibodies may not be the primary factors leading to NPSLE (147). Overall, the scarcity of brain tissue samples from patients significantly limits our understanding of brain damage in lupus. Currently, there is no definitive conclusion regarding the role of B cells in the structural or functional damage to the brain in lupus patients.
3.7 B cells and cardiac, respiratory, ovarian gastrointestinal diseases, and arthritis in lupus patients
Cardiac involvement represents a significant complication in patients with lupus. These patients often experience vascular inflammation, where endothelial damage can promote atherosclerosis through the deposition of circulating immune complexes or the interaction of antibodies with endothelial cells. Endothelial dysfunction, along with the secretion of pro-inflammatory cytokines, can activate B cells, leading to the production of autoantibodies and the formation of immune complexes. This process further accelerates the intracellular accumulation of lipids in atherosclerotic plaques. Reports indicate that the carotid intima-media thickness in lupus patients is increased, indicating a susceptibility to subclinical atherosclerosis. This increase correlates with a decrease in the number of regulatory B cells (CD19+CD24hiCD38hi) (148). Immunohistochemical analyses of myocardial biopsy tissues from 14 lupus patients revealed deposits of immunoglobulin G and fibrinogen in the myocardial cell membrane and interstitium. These findings were accompanied by T cell infiltration, but no evidence of B cell infiltration was observed (149).
The lungs are also frequently affected in lupus patients, particularly in cases of interstitial lung disease. Among respiratory complications, diffuse alveolar hemorrhage is the most severe, with onset occurring within hours to days and a mortality rate as high as 50% (150). Treatment with CD19-targeting CAR-T or CD20-targeting rituximab has been shown to reduce levels of various autoantibodies and immunoglobulins, resulting in improvements in lung function (151–153). However, direct evidence demonstrating the involvement of B cells in the pathological mechanisms underlying lung injury in lupus patients is currently lacking.
Lupus is more prevalent in young women. Estrogen binding to B lymphocytes may trigger autoimmune reactions, resulting in the production of high-affinity autoantibodies and pro-inflammatory cytokines. This process can lead to estrogen-induced autoimmune diseases, such as autoimmune ovarian inflammation (154). Due to the combined effects of the disease and treatments like cyclophosphamide, lupus patients often experience reproductive organ dysfunction. This dysfunction can lead to decreased ovarian reserve, infertility, premature ovarian failure, and an increased risk of adverse pregnancy outcomes (155). Currently, there are no direct studies linking B cell abnormalities to ovarian dysfunction in lupus patients.
Regarding gastrointestinal diseases in lupus patients, research using animal models suggests that Helicobacter pylori infection can exacerbate inflammation, activate splenic B cells, and increase antibody secretion, ultimately promoting disease progression (156). An imbalance in gut microbiota is also suspected to contribute to the onset of lupus (157). Notably, the anaerobic bacteria from the Ruminococcus genus are found to be expanded in patients with active LN. Transplanting patient-derived strains into C57BL/6 mice leads to increased intestinal permeability, resulting in intestinal leakage and elevated levels of anti-dsDNA antibodies (158). Overall, research on the role of B cells in the gastrointestinal system of lupus patients remains limited.
Additionally, arthritis is one of the important organ disorders in SLE. However, current research on lupus B cells in articular tissue is still lacking, and further studies are needed to explore potential commonalities and distinctions between lupus and other joint diseases such as rheumatoid arthritis and osteoarthritis.
4 Clinical evaluation of B cell-targeted therapies
Current B cell-targeted therapies mainly include directly killing B cells, modulating B cell function, targeting molecular pathways for B cell growth and survival, and accelerating the degradation of autoantibodies. Traditional immunosuppressants are widely used in clinical practice, such as corticosteroids, hydroxychloroquine (HCQ), cyclophosphamide, mycophenolate mofetil, and azathioprine. There are also some drugs could inhibit B cell activation, proliferation, differentiation, and receptor signaling, such as Tripterygium wilfordii (159), SB431542 (160), rapamycin (161), KZR-616 (162), and Povetacicept (163). Additionally, biologic agents targeting B cells have emerged in recent years, as well as chimeric antigen receptor T cell (CAR-T) targeting the CD19 antigen, providing new hope for lupus patients (164).
Rituximab, a chimeric anti-CD20 monoclonal antibody, is used to deplete mature B cells and reduce the production of autoantibodies. About 98.6% patients exhibited depletion of peripheral blood CD19+B cells and reduction in serum levels of complement and anti-dsDNA antibody with rituximab treatment in LUNAR trial including 144 patients with LN of type III and type IV (165).
Belimumab, a fully recombinant humanized IgG2λ monoclonal antibody, is the first inhibitor targeting B-lymphocyte stimulator (BAFF/BLyS) (166). Belimumab reduced the number of immature B cells and CD11c+CD21-B cell at 3 months (167). After 76 weeks of treatment, significant reductions in B cell subpopulations were observed: naïve B cells, activated B cells, plasmacytoid cells, and plasma cells (168). Clinically, belimumab was effective in active lupus patients, who had a reduced risk of LN disease activity (169).
Telitacept, a human recombinant fusion targeting B lymphocyte stimulator, is a dual-target inhibitor of BAFF and APRIL (170). Telitacept could prevent the development and maturation of B cells by blocking BAFF, and reduce the number of CD19+ and IgD+ B cells in peripheral blood by blocking APRIL, decreasing the release of autoantibodies and immunoglobulin, and increasing complement levels (171, 172).
CD19-targeted CAR-T cell therapy may induce B cell depletion or co-target plasmablasts. Recently, researchers have been exploring CAR-T therapy targeting B cells in autoimmune diseases such as lupus (173). Some studies with small samples have produced great results for SLE patients and reported the CD19 CAR-T therapies in lupus appeared feasible, safe, efficacious and hopeful (174, 175).
The development of new drugs and therapies has brought significant progress, however, some patients still show unresponsive to treatment. Therefore, it is important to go further into exploring the characteristics of B cells in different organs or tissues.
5 Conclusion
The imbalance of B cell immune tolerance and the production of autoantibodies are central to the pathogenesis of lupus. However, the mechanisms by which immune tolerance is disrupted in lupus patients, the regulation of B cells during their development, differentiation, and maturation, and the targeted therapy of tissue-specific pathogenic B cells remain insufficiently understood. Disruption of peripheral immune tolerance, particularly involving immune checkpoints in the germinal center, is closely linked to the maturation of antibody affinity in autoreactive B cells. Additionally, B cells in the peripheral blood, kidney, skin, and bone marrow of lupus patients exhibit tissue-specific characteristics. For instance, pathogenic B cells in kidney tissue show high levels of infiltration and expression, primarily in the form of age-associated B cells. In contrast, skin tissue contains fewer disease-specific double-negative B cells, while bone marrow B cells demonstrate early developmental disorders, revealing significant abnormalities in their BCR repertoire during early development. Given these tissue-specific features, current strategies for targeted B cell therapy have yet to address these specific issues effectively (Figure 2). Through this review, we aim to offer new perspectives for future research related to B cells in lupus. This includes the development of targeted therapies and an important angle on the high heterogeneity of the disease.
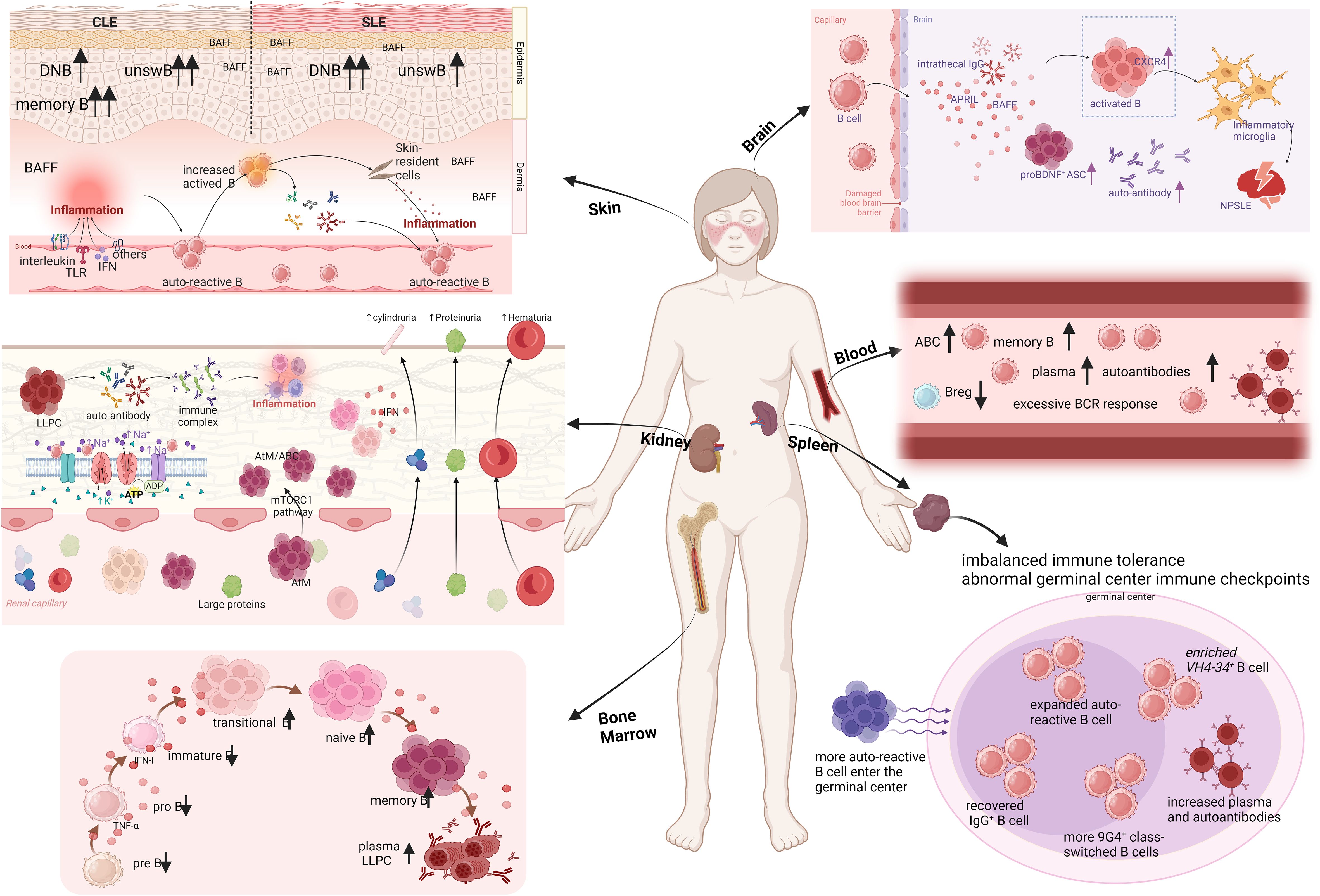
Figure 2. Organ-based characterization of B cells in lupus patients. CLE, cutaneous lupus erythematosus; SLE, systemic lupus erythematosus; DNB, double negative B cells; unswB, unswitched B cells; TLR, Toll-like receptors; IFN, interferon; ASC, antibody-secreting cells; NPSLE, neuropsychiatric lupus; LLPC, long-lived plasma cells; AtM, atypical memory B cells; ABC, age associated B cell (Created in BioRender. Dong, C. (2025) https://BioRender.com/o27s426).
Author contributions
YW: Data curation, Writing – original draft. RZ: Writing – original draft. QL: Writing – original draft. SN: Funding acquisition, Investigation, Validation, Writing – original draft. MY: Investigation, Validation, Writing – original draft. LQ: Funding acquisition, Investigation, Validation, Writing – original draft. JJ: Investigation, Validation, Writing – original draft. ZG: Funding acquisition, Writing – review & editing. CD: Conceptualization, Data curation, Investigation, Methodology, Writing – review & editing.
Funding
The author(s) declare that financial support was received for the research, authorship, and/or publication of this article. This study was supported by National Key Research and Development Program of China (2023YFC2306501), National Natural Science Foundation of China (No. 82071838, 82302048, 82402102), Jiangsu Provincial Medical Key Discipline Cultivation Unit (No. JSDW202205), Jiangsu Provincial Research Hospital (No. YJXYY202204), and Jiangsu Provincial Preventive Medicine Research Project (No. Ym2023112).
Conflict of interest
The authors declare that the research was conducted in the absence of any commercial or financial relationships that could be construed as a potential conflict of interest.
Generative AI statement
The author(s) declare that no Generative AI was used in the creation of this manuscript.
Publisher’s note
All claims expressed in this article are solely those of the authors and do not necessarily represent those of their affiliated organizations, or those of the publisher, the editors and the reviewers. Any product that may be evaluated in this article, or claim that may be made by its manufacturer, is not guaranteed or endorsed by the publisher.
References
1. Yuuki H, Itamiya T, Nagafuchi Y, Ota M, Fujio K. B cell receptor repertoire abnormalities in autoimmune disease. Front Immunol. (2024) 15:1326823. doi: 10.3389/fimmu.2024.1326823
2. Ceccarelli F, Perricone C, Natalucci F, Picciariello L, Olivieri G, Cafaro G, et al. Organ damage in Systemic Lupus Erythematosus patients: A multifactorial phenomenon. Autoimmun Rev. (2023) 22:103374. doi: 10.1016/j.autrev.2023.103374
3. Tsokos GC. Systemic lupus erythematosus. N Engl J Med. (2011) 365:2110–21. doi: 10.1056/NEJMra1100359
4. Nakano M, Iwasaki Y, Fujio K. Transcriptomic studies of systemic lupus erythematosus. Inflammation Regen. (2021) 41:11. doi: 10.1186/s41232-021-00161-y
5. Perez RK, Gordon MG, Subramaniam M, Kim MC, Hartoularos GC, Targ S, et al. Single-cell RNA-seq reveals cell type-specific molecular and genetic associations to lupus. Science. (2022) 376:eabf1970. doi: 10.1126/science.abf1970
6. Banchereau R, Hong S, Cantarel B, Baldwin N, Baisch J, Edens M, et al. Personalized immunomonitoring uncovers molecular networks that stratify lupus patients. Cell. (2016) 165(3):551–65. doi: 10.1016/j.cell.2016.05.057
7. Wang Y, Zhao R, Gu C, Gu Z, Li L, Li Z, et al. The impact of systemic lupus erythematosus on health-related quality of life assessed using the SF-36: a systematic review and meta-analysis. Psychol Health Med. (2019) 24:978–91. doi: 10.1080/13548506.2019.1587479
8. Wang X, Li J, Liang Q, Ni X, Zhao R, Fu T, et al. Reproductive concerns and contributing factors in women of childbearing age with systemic lupus erythematosus. Clin Rheumatol. (2022) 41:2383–91. doi: 10.1007/s10067-022-06156-5
9. Shen B, Feng G, Tang W, Huang X, Yan H, He Y, et al. The quality of life in Chinese patients with systemic lupus erythematosus is associated with disease activity and psychiatric disorders: a path analysis. Clin Exp Rheumatol. (2014) 32(1):101–7. doi: 10.1016/j.rbre.2014.02.013
10. Zhao Q, Deng N, Chen S, Cui Y, Du X, Gu Z. Systemic lupus erythematosus is associated with negatively variable impacts on domains of sleep disturbances: a systematic review and meta-analysis. Psychol Health Med. (2018) 23:685–97. doi: 10.1080/13548506.2018.1442011
11. Zhang Q, Zhang X, Feng G, Fu T, Yin R, Zhang L, et al. Periodontal disease in Chinese patients with systemic lupus erythematosus. Rheumatol Int. (2017) 37:1373–9. doi: 10.1007/s00296-017-3759-5
12. Gu C, Zhao R, Zhang X, Gu Z, Zhou W, Wang Y, et al. A meta-analysis of secondary osteoporosis in systemic lupus erythematosus: prevalence and risk factors. Arch Osteoporos. (2019) 15:1. doi: 10.1007/s11657-019-0667-1
13. Dong C, Yang N, Zhao R, Yang Y, Gu X, Fu T, et al. SVM-based model combining patients’ Reported outcomes and lymphocyte phenotypes of depression in systemic lupus erythematosus. Biomolecules. (2023) 13(5):723. doi: 10.3390/biom13050723
14. Gu XX, Jin Y, Fu T, Zhang XM, Li T, Yang Y, et al. Relevant characteristics analysis using natural language processing and machine learning based on phenotypes and T-cell subsets in systemic lupus erythematosus patients with anxiety. Front Psychiatry. (2021) 12:793505. doi: 10.3389/fpsyt.2021.793505
15. Kong X, Zhang Z, Fu T, Ji J, Yang J, Gu Z. TNF-alpha regulates microglial activation via the NF-kappaB signaling pathway in systemic lupus erythematosus with depression. Int J Biol Macromol. (2019) 125:892–900. doi: 10.1016/j.ijbiomac.2018.12.146
16. Li Z, Yang Y, Dong C, Li L, Cui Y, Zhao Q, et al. The prevalence of suicidal ideation and suicide attempt in patients with rheumatic diseases: a systematic review and meta-analysis. Psychol Health Med. (2018) 23:1025–36. doi: 10.1080/13548506.2018.1476724
17. Sutanto H, Yuliasih Y. Disentangling the pathogenesis of systemic lupus erythematosus: close ties between immunological, genetic and environmental factors. Medicina (Kaunas). (2023) 59(6):1033. doi: 10.3390/medicina59061033
18. Pan L, Lu MP, Wang JH, Xu M, Yang SR. Immunological pathogenesis and treatment of systemic lupus erythematosus. World J Pediatr. (2020) 16:19–30. doi: 10.1007/s12519-019-00229-3
19. Sang A, Danhorn T, Peterson JN, Rankin AL, O’Connor BP, Leach SM, et al. Innate and adaptive signals enhance differentiation and expansion of dual-antibody autoreactive B cells in lupus. Nat Commun. (2018) 9(1):3973. doi: 10.1038/s41467-018-06293-z
20. Ameer MA, Chaudhry H, Mushtaq J, Khan OS, Babar M, Hashim T, et al. An overview of systemic lupus erythematosus (SLE) pathogenesis, classification, and management. Cureus. (2022) 14(10):e30330. doi: 10.7759/cureus.30330
21. Iwata S, Hajime Sumikawa M, Tanaka Y. B cell activation via immunometabolism in systemic lupus erythematosus. Front Immunol. (2023) 14:1155421. doi: 10.3389/fimmu.2023.1155421
22. Brunner HI, Abud-Mendoza C, Viola DO, Calvo Penades I, Levy D, Anton J, et al. Safety and efficacy of intravenous belimumab in children with systemic lupus erythematosus: results from a randomised, placebo-controlled trial. Ann Rheum Dis. (2020) 79:1340–8. doi: 10.1136/annrheumdis-2020-217101
23. Fanouriakis A, Kostopoulou M, Andersen J, Aringer M, Arnaud L, Bae SC, et al. EULAR recommendations for the management of systemic lupus erythematosus: 2023 update. Ann Rheum Dis. (2024) 83:15–29. doi: 10.1136/ard-2023-224762
24. Dhillon S. Telitacicept: first approval. Drugs. (2021) 81(14):1671–5. doi: 10.1007/s40265-021-01591-1
25. Schett G, Mackensen A, Mougiakakos D. CAR T-cell therapy in autoimmune diseases. Lancet. (2023) 402(10416):2034–44. doi: 10.1016/S0140-6736(23)01126-1
26. Johnson JL, Scholz JL, Marshak-Rothstein A, Cancro MP. Molecular pattern recognition in peripheral B cell tolerance: lessons from age-associated B cells. Curr Opin Immunol. (2019) 61:33–8. doi: 10.1016/j.coi.2019.07.008
27. Wardemann H, Yurasov S, Schaefer A, Young JW, Meffre E, Nussenzweig MC. Predominant autoantibody production by early human B cell precursors. Science. (2003) 301(5638):1374–7. doi: 10.1126/science.1086907
28. Kraus M, Alimzhanov MB, Rajewsky N, Rajewsky K. Survival of resting mature B lymphocytes depends on BCR signaling via the Igalpha/beta heterodimer. Cell. (2004) 117(6):787–800. doi: 10.1016/j.cell.2004.05.014
29. Schiemann B, Gommerman JL, Vora K, Cachero TG, Shulga-Morskaya S, Dobles M, et al. An essential role for BAFF in the normal development of B cells through a BCMA-independent pathway. Science. (2001) 293(5537):2111–4. doi: 10.1126/science.1061964
30. Wang YH, Diamond B. B cell receptor revision diminishes the autoreactive B cell response after antigen activation in mice. J Clin Invest. (2008) 118:2896–907. doi: 10.1172/JCI35618
31. Rawlings DJ, Metzler G, Wray-Dutra M, Jackson SW. Altered B cell signalling in autoimmunity. Nat Rev Immunol. (2017) 17:421–36. doi: 10.1038/nri.2017.24
32. Ota M, Nakano M, Nagafuchi Y, Kobayashi S, Hatano H, Yoshida R, et al. Multimodal repertoire analysis unveils B cell biology in immune-mediated diseases. Ann Rheum Dis. (2023) 82:1455–63. doi: 10.1136/ard-2023-224421
33. Möckel T, Basta F, Weinmann-Menke J, Schwarting A. B cell activating factor (BAFF): Structure, functions, autoimmunity and clinical implications in Systemic Lupus Erythematosus (SLE). Autoimmun Rev. (2021) 20:102736. doi: 10.1016/j.autrev.2020.102736
34. Schroeder K, Herrmann M, Winkler TH. The role of somatic hypermutation in the generation of pathogenic antibodies in SLE. Autoimmunity. (2013) 46(2):121–7. doi: 10.3109/08916934.2012.748751
35. Tiller T, Tsuiji M, Yurasov S, Velinzon K, Nussenzweig MC, Wardemann H. Autoreactivity in human IgG+ memory B cells. Immunity. (2007) 26(2):205–13. doi: 10.1016/j.immuni.2007.01.009
36. Espeli M, Bashford-Rogers R, Sowerby JM, Alouche N, Wong L, Denton AE, et al. FcgammaRIIb differentially regulates pre-immune and germinal center B cell tolerance in mouse and human. Nat Commun. (2019) 10(1):1970. doi: 10.1038/s41467-019-09434-0
37. Shlomchik MJ. Sites and stages of autoreactive B cell activation and regulation. Immunity. (2008) 28(1):18–28. doi: 10.1016/j.immuni.2007.12.004
38. Yau IW, Cato MH, Jellusova J, Hurtado de Mendoza T, Brink R, Rickert RC. Censoring of self-reactive B cells by follicular dendritic cell-displayed self-antigen. J Immunol. (2013) 191:1082–90. doi: 10.4049/jimmunol.1201569
39. McCarron MJ, Marie JC. TGF-beta prevents T follicular helper cell accumulation and B cell autoreactivity. J Clin Invest. (2014) 124:4375–86. doi: 10.1172/JCI76179
40. Sage PT, Paterson AM, Lovitch SB, Sharpe AH. The coinhibitory receptor CTLA-4 controls B cell responses by modulating T follicular helper, T follicular regulatory, and T regulatory cells. Immunity. (2014) 41:1026–39. doi: 10.1016/j.immuni.2014.12.005
41. Jenks SA, Cashman KS, Woodruff MC, Lee FE, Sanz I. Extrafollicular responses in humans and SLE. Immunol Rev. (2019) 288:136–48. doi: 10.1111/imr.2019.288.issue-1
42. Bombardieri M, Lewis M, Pitzalis C. Ectopic lymphoid neogenesis in rheumatic autoimmune diseases. Nat Rev Rheumatol. (2017) 13:141–54. doi: 10.1038/nrrheum.2016.217
43. Liarski VM, Kaverina N, Chang A, Brandt D, Yanez D, Talasnik L, et al. Cell distance mapping identifies functional T follicular helper cells in inflamed human renal tissue. Sci Transl Med. (2014) 6:230ra46. doi: 10.1126/scitranslmed.3008146
44. Arazi A, Rao DA, Berthier CC, Davidson A, Liu Y, Hoover PJ, et al. The immune cell landscape in kidneys of patients with lupus nephritis. Nat Immunol. (2019) 20:902–14. doi: 10.1038/s41590-019-0398-x
45. Bhat NM, Lee LM, van Vollenhoven RF, Teng NN, Bieber MM. VH4-34 encoded antibody in systemic lupus erythematosus: effect of isotype. J Rheumatol. (2002) 29(10):2114–21. doi: 10.1016/S1297-319X(02)00445-1
46. Tipton CM, Fucile CF, Darce J, Chida A, Ichikawa T, Gregoretti I, et al. Diversity, cellular origin and autoreactivity of antibody-secreting cell population expansions in acute systemic lupus erythematosus. Nat Immunol. (2015) 16:755–65. doi: 10.1038/ni.3175
47. Isenberg D, Spellerberg M, Williams W, Griffiths M, Stevenson F. Identification of the 9G4 idiotope in systemic lupus erythematosus. Br J Rheumatol. (1993) 32:876–82. doi: 10.1093/rheumatology/32.10.876
48. Richardson C, Chida AS, Adlowitz D, Silver L, Fox E, Jenks SA, et al. Molecular basis of 9G4 B cell autoreactivity in human systemic lupus erythematosus. J Immunol. (2013) 191:4926–39. doi: 10.4049/jimmunol.1202263
49. Jenks SA, Palmer EM, Marin EY, Hartson L, Chida AS, Richardson C, et al. 9G4+ autoantibodies are an important source of apoptotic cell reactivity associated with high levels of disease activity in systemic lupus erythematosus. Arthritis Rheumatol. (2013) 65:3165–75. doi: 10.1002/art.v65.12
50. Sabouri Z, Schofield P, Horikawa K, Spierings E, Kipling D, Randall KL, et al. Redemption of autoantibodies on anergic B cells by variable-region glycosylation and mutation away from self-reactivity. Proc Natl Acad Sci U S A. (2014) 111:E2567–75. doi: 10.1073/pnas.1406974111
51. Cappione A 3rd, Anolik JH, Pugh-Bernard A, Barnard J, Dutcher P, Silverman G, et al. Germinal center exclusion of autoreactive B cells is defective in human systemic lupus erythematosus. J Clin Invest. (2005) 115:3205–16. doi: 10.1172/JCI24179
52. Voulgarelis M, Giannouli S, Tasidou A, Anagnostou D, Ziakas PD, Tzioufas AG. Bone marrow histological findings in systemic lupus erythematosus with hematologic abnormalities: a clinicopathological study. Am J Hematol. (2006) 81:590–7. doi: 10.1002/(ISSN)1096-8652
53. Meffre E, Wardemann H. B-cell tolerance checkpoints in health and autoimmunity. Curr Opin Immunol. (2008) 20:632–8. doi: 10.1016/j.coi.2008.09.001
54. Grigoriou M, Banos A, Filia A, Pavlidis P, Giannouli S, Karali V, et al. Transcriptome reprogramming and myeloid skewing in haematopoietic stem and progenitor cells in systemic lupus erythematosus. Ann Rheum Dis. (2020) 79:242–53. doi: 10.1136/annrheumdis-2019-215782
55. Papadaki HA, Boumpas DT, Gibson FM, Jayne DR, Axford JS, Gordon-Smith EC, et al. Increased apoptosis of bone marrow CD34(+) cells and impaired function of bone marrow stromal cells in patients with systemic lupus erythematosus. Br J Haematol. (2001) 115:167–74. doi: 10.1046/j.1365-2141.2001.03076.x
56. Hou X, Wei W, Zhang J, Liu Z, Wang G, Yang X, et al. Characterisation of T and B cell receptor repertoire in patients with systemic lupus erythematosus. Clin Exp Rheumatol. (2023) 41(11):2216–23. doi: 10.55563/clinexprheumatol/1rjr4s
57. Wang J, Lin Q, Langston H, Cooper MD. Resident bone marrow macrophages produce type 1 interferons that can selectively inhibit interleukin-7-driven growth of B lineage cells. Immunity. (1995) 3:475–84. doi: 10.1016/1074-7613(95)90176-0
58. Palanichamy A, Bauer JW, Yalavarthi S, Meednu N, Barnard J, Owen T, et al. Neutrophil-mediated IFN activation in the bone marrow alters B cell development in human and murine systemic lupus erythematosus. J Immunol. (2014) 192:906–18. doi: 10.4049/jimmunol.1302112
59. Ueda Y, Yang K, Foster SJ, Kondo M, Kelsoe G. Inflammation controls B lymphopoiesis by regulating chemokine CXCL12 expression. J Exp Med. (2004) 199:47–58. doi: 10.1084/jem.20031104
60. Maeda K, Malykhin A, Teague-Weber BN, Sun XH, Farris AD, Coggeshall KM. Interleukin-6 aborts lymphopoiesis and elevates production of myeloid cells in systemic lupus erythematosus-prone B6.Sle1.Yaa animals. Blood. (2009) 113:4534–40. doi: 10.1182/blood-2008-12-192559
61. Zheng X, Dozmorov MG, Strohlein CE, Bastacky S, Sawalha AH. Ezh2 knockout in B cells impairs plasmablast differentiation and ameliorates lupus-like disease in MRL/lpr mice. Arthritis Rheumatol. (2023) 75:1395–406. doi: 10.1002/art.42492
62. Nie Y, Li C, Sun N. Ophiopogonin D attenuates the progression of murine systemic lupus erythematosus by reducing B cell numbers. J Biochem Mol Toxicol. (2023) 37:e23361. doi: 10.1002/jbt.23361
63. Emmanouilidou E, Kosmara D, Papadaki E, Mastorodemos V, Constantoulakis P, Repa A, et al. Progressive multifocal leukoencephalopathy in systemic lupus erythematosus: A consequence of patient-intrinsic or -extrinsic factors? J Clin Med. (2023) 12(21):6945. doi: 10.3390/jcm12216945
64. Dong C, Guo Y, Chen Z, Li T, Ji J, Sun C, et al. Single-cell profiling of bone marrow B cells uncovers early B cell developmental disorders associated with systemic lupus erythematosus. Arthritis Rheumatol. (2024) 76(4):599–613. doi: 10.1002/art.42750
65. Mahla RS. Bone Marrow B Cell Developmental Aberrations and Systemic Lupus Erythematosus: comment on the article by Dong et al. Arthritis Rheumatol. (2024) 76(5):813–4. doi: 10.1002/art.42786
66. Dong C, Chen Z, Zhang X, Gu Z. Reply. Arthritis Rheumatol. (2024) 76(5):814–5. doi: 10.1002/art.42787
67. Karrar S, Cunninghame Graham DS. Abnormal B cell development in systemic lupus erythematosus: what the genetics tell us. Arthritis Rheumatol. (2018) 70:496–507. doi: 10.1002/art.40396
68. Nashi E, Wang Y, Diamond B. The role of B cells in lupus pathogenesis. Int J Biochem Cell Biol. (2010) 42:543–50. doi: 10.1016/j.biocel.2009.10.011
69. Kang N, Liu X, You X, Sun W, Haneef K, Sun X, et al. Aberrant B-cell activation in systemic lupus erythematosus. Kidney Dis (Basel). (2022) 8:437–45. doi: 10.1159/000527213
70. Hemon P, Renaudineau Y, Debant M, Le Goux N, Mukherjee S, Brooks W, et al. Calcium signaling: from normal B cell development to tolerance breakdown and autoimmunity. Clin Rev Allergy Immunol. (2017) 53:141–65. doi: 10.1007/s12016-017-8607-6
71. Boes M, Schmidt T, Linkemann K, Beaudette BC, Marshak-Rothstein A, Chen J. Accelerated development of IgG autoantibodies and autoimmune disease in the absence of secreted IgM. Proc Natl Acad Sci U S A. (2000) 97:1184–9. doi: 10.1073/pnas.97.3.1184
72. William J, Euler C, Christensen S, Shlomchik MJ. Evolution of autoantibody responses via somatic hypermutation outside of germinal centers. Science. (2002) 297(5589):2066–70. doi: 10.1126/science.1073924
73. Scott DA, Coombs GH, Sanderson BE. Effects of methotrexate and other antifolates on the growth and dihydrofolate reductase activity of Leishmania promastigotes. Biochem Pharmacol. (1987) 36:2043–5. doi: 10.1016/0006-2952(87)90508-9
74. Kajihara A, Morita T, Kato Y, Konaka H, Murakami T, Yamaguchi Y, et al. The proliferative activity levels of each immune cell population evaluated by mass cytometry are linked to the clinical phenotypes of systemic lupus erythematosus. Int Immunol. (2023) 35:27–41. doi: 10.1093/intimm/dxac042
75. Nakano M, Ayano M, Kushimoto K, Kawano S, Higashioka K, Inokuchi S, et al. Increased proportion of CD226(+) B cells is associated with the disease activity and prognosis of systemic lupus erythematosus. Front Immunol. (2021) 12:713225. doi: 10.3389/fimmu.2021.713225
76. Peng Y, Guo F, Liao S, Liao H, Xiao H, Yang L, et al. Altered frequency of peripheral B-cell subsets and their correlation with disease activity in patients with systemic lupus erythematosus: A comprehensive analysis. J Cell Mol Med. (2020) 24:12044–53. doi: 10.1111/jcmm.v24.20
77. Xiong H, Tang Z, Xu Y, Shi Z, Guo Z, Liu X, et al. CD19(+)CD24(high)CD27(+) B cell and interleukin 35 as potential biomarkers of disease activity in systemic lupus erythematosus patients. Adv Rheumatol. (2022) 62:48. doi: 10.1186/s42358-022-00279-8
78. Catalan D, Mansilla MA, Ferrier A, Soto L, Oleinika K, Aguillon JC, et al. Immunosuppressive mechanisms of regulatory B cells. Front Immunol. (2021) 12:611795. doi: 10.3389/fimmu.2021.611795
79. Wu C, Fu Q, Guo Q, Chen S, Goswami S, Sun S, et al. Lupus-associated atypical memory B cells are mTORC1-hyperactivated and functionally dysregulated. Ann Rheum Dis. (2019) 78:1090–100. doi: 10.1136/annrheumdis-2019-215039
80. Ju B, Wang J, Mo L, Huang J, Hao Z, Lv X, et al. Elevated CD19(+)Siglec-10(+) B cell levels are correlated with systemic lupus erythematosus disease activity. Int Immunopharmacol. (2022) 102:108403. doi: 10.1016/j.intimp.2021.108403
81. Zhang W, Wang YF, Hu FL, Lu FA, Wu T, Feng YL, et al. Dysfunction of CD27(+)IgD(+) B cells correlates with aggravated systemic lupus erythematosus. Clin Rheumatol. (2022) 41:1551–9. doi: 10.1007/s10067-022-06051-z
82. Guimaraes PM, Scavuzzi BM, Stadtlober NP, Franchi Santos L, Lozovoy MAB, Iriyoda TMV, et al. Cytokines in systemic lupus erythematosus: far beyond Th1/Th2 dualism lupus: cytokine profiles. Immunol Cell Biol. (2017) 95:824–31. doi: 10.1038/icb.2017.53
83. Yap DYH, Chan TM. B cell abnormalities in systemic lupus erythematosus and lupus nephritis-role in pathogenesis and effect of immunosuppressive treatments. Int J Mol Sci. (2019) 20(24):6231. doi: 10.3390/ijms20246231
84. Pang J, Li Y, Tao R, Li J, Wang F, Xu H. Correlation between B-cell activating factor of the tumor necrosis factor family level in serum and immune inflammation in patients with neuropsychiatric systemic lupus erythematosus and its clinical value. Immunol Invest. (2024) 53(4):559–73. doi: 10.1080/08820139.2024.2309567
85. Dai D, Gu S, Han X, Ding H, Jiang Y, Zhang X, et al. The transcription factor ZEB2 drives the formation of age-associated B cells. Science. (2024) 383(6681):413–21. doi: 10.1126/science.adf8531
86. Huang T, Pi C, Xu X, Feng Y, Zhang J, Gu H, et al. Effect of BAFF blockade on the B cell receptor repertoire and transcriptome in a mouse model of systemic lupus erythematosus. Front Immunol. (2023) 14:1307392. doi: 10.3389/fimmu.2023.1307392
87. Girschick HJ, Grammer AC, Nanki T, Vazquez E, Lipsky PE. Expression of recombination activating genes 1 and 2 in peripheral B cells of patients with systemic lupus erythematosus. Arthritis Rheumatol. (2002) 46:1255–63. doi: 10.1002/art.10264
88. Lin Z, Yu N, Cheng C, Jin B, Zhang Q, Zhuang H, et al. Serum levels and significance of soluble B-cell maturation antigen in childhood-onset systemic lupus erythematosus with renal involvement. Lupus. (2023) 32:680–7. doi: 10.1177/09612033231164633
89. Alvarez Gomez JA, Salazar-Camarena DC, Roman-Fernandez IV, Ortiz-Lazareno PC, Cruz A, Munoz-Valle JF, et al. BAFF system expression in double negative 2, activated naive and activated memory B cells in systemic lupus erythematosus. Front Immunol. (2023) 14:1235937. doi: 10.3389/fimmu.2023.1235937
90. Hirano A, Fujioka K, Kida T, Omura S, Sofue H, Sakashita A, et al. Association between early immunophenotypic changes and therapeutic response of belimumab in patients with systemic lupus erythematosus. Lupus. (2023) 32:63–73. doi: 10.1177/09612033221137249
91. Szelinski F, Stefanski AL, Schrezenmeier E, Rincon-Arevalo H, Wiedemann A, Reiter K, et al. Plasmablast-like phenotype among antigen-experienced CXCR5-CD19(low) B cells in systemic lupus erythematosus. Arthritis Rheumatol. (2022) 74:1556–68. doi: 10.1002/art.42157
92. Martin J, Cheng Q, Laurent SA, Thaler FS, Beenken AE, Meinl E, et al. B-cell maturation antigen (BCMA) as a biomarker and potential treatment target in systemic lupus erythematosus. Int J Mol Sci. (2024) 25(19):10845. doi: 10.3390/ijms251910845
93. Devarapu SK, Anders HJ. Toll-like receptors in lupus nephritis. J BioMed Sci. (2018) 25:35. doi: 10.1186/s12929-018-0436-2
94. Celhar T, Hopkins R, Thornhill SI, De Magalhaes R, Hwang SH, Lee HY, et al. RNA sensing by conventional dendritic cells is central to the development of lupus nephritis. Proc Natl Acad Sci U S A. (2015) 112:E6195–204. doi: 10.1073/pnas.1507052112
95. Weindel CG, Richey LJ, Bolland S, Mehta AJ, Kearney JF, Huber BT. B cell autophagy mediates TLR7-dependent autoimmunity and inflammation. Autophagy. (2015) 11:1010–24. doi: 10.1080/15548627.2015.1052206
96. Jenks SA, Cashman KS, Zumaquero E, Marigorta UM, Patel AV, Wang X, et al. Distinct effector B cells induced by unregulated toll-like receptor 7 contribute to pathogenic responses in systemic lupus erythematosus. Immunity. (2018) 49:725–39 e6. doi: 10.1016/j.immuni.2018.08.015
97. Jackson SW, Scharping NE, Kolhatkar NS, Khim S, Schwartz MA, Li QZ, et al. Opposing impact of B cell-intrinsic TLR7 and TLR9 signals on autoantibody repertoire and systemic inflammation. J Immunol. (2014) 192:4525–32. doi: 10.4049/jimmunol.1400098
98. Ma K, Li J, Fang Y, Lu L. Roles of B cell-intrinsic TLR signals in systemic lupus erythematosus. Int J Mol Sci. (2015) 16:13084–105. doi: 10.3390/ijms160613084
99. Kalliolias GD, Basdra EK, Papavassiliou AG. Targeting TLR signaling cascades in systemic lupus erythematosus and rheumatoid arthritis: an update. Biomedicines. (2024) 12(1):138. doi: 10.3390/biomedicines12010138
100. Sinai P, Dozmorov IM, Song R, Schwartzberg PL, Wakeland EK, Wulfing C. T/B-cell interactions are more transient in response to weak stimuli in SLE-prone mice. Eur J Immunol. (2014) 44:3522–31. doi: 10.1002/eji.201444602
101. Miyagawa-Hayashino A, Yoshifuji H, Kitagori K, Ito S, Oku T, Hirayama Y, et al. Increase of MZB1 in B cells in systemic lupus erythematosus: proteomic analysis of biopsied lymph nodes. Arthritis Res Ther. (2018) 20:13. doi: 10.1186/s13075-018-1511-5
102. Nakatani K, Qu WM, Zhang MC, Fujii H, Furukawa H, Miyazaki T, et al. A genetic locus controlling aging-sensitive regression of B lymphopoiesis in an autoimmune-prone MRL/lpr strain of mice. Scand J Immunol. (2007) 66:654–61. doi: 10.1111/j.1365-3083.2007.02020.x
103. Su YJ, Li FA, Sheu JJ, Li SC, Weng SW, Shen FC, et al. A study on MDA5 signaling in splenic B cells from an imiquimod-induced lupus mouse model with proteomics. Cells. (2022) 11(21):3350. doi: 10.3390/cells11213350
104. Zhao J, Li L, Feng X, Gao C, Gao L, Zhan Y, et al. TIGIT-Fc fusion protein alleviates murine lupus nephritis through the regulation of SPI-B-PAX5-XBP1 axis-mediated B-cell differentiation. J Autoimmun. (2023) 139:103087. doi: 10.1016/j.jaut.2023.103087
105. Yang M, Long D, Hu L, Zhao Z, Li Q, Guo Y, et al. AIM2 deficiency in B cells ameliorates systemic lupus erythematosus by regulating Blimp-1-Bcl-6 axis-mediated B-cell differentiation. Signal Transduct Target Ther. (2021) 6:341. doi: 10.1038/s41392-021-00725-x
106. Parodis I, Tamirou F, Houssiau FA. Prediction of prognosis and renal outcome in lupus nephritis. Lupus Sci Med. (2020) 7:e000389. doi: 10.1136/lupus-2020-000389
107. Lech M, Anders HJ. The pathogenesis of lupus nephritis. J Am Soc Nephrol. (2013) 24:1357–66. doi: 10.1681/ASN.2013010026
108. Alduraibi F, Fatima H, Hamilton JA, Chatham WW, Hsu HC, Mountz JD. Lupus nephritis correlates with B cell interferon-beta, anti-Smith, and anti-DNA: a retrospective study. Arthritis Res Ther. (2022) 24:87. doi: 10.1186/s13075-022-02766-1
109. Ma K, Li J, Wang X, Lin X, Du W, Yang X, et al. TLR4(+)CXCR4(+) plasma cells drive nephritis development in systemic lupus erythematosus. Ann Rheum Dis. (2018) 77:1498–506. doi: 10.1136/annrheumdis-2018-213615
110. Peterson KS, Huang JF, Zhu J, D’Agati V, Liu X, Miller N, et al. Characterization of heterogeneity in the molecular pathogenesis of lupus nephritis from transcriptional profiles of laser-captured glomeruli. J Clin Invest. (2004) 113:1722–33. doi: 10.1172/JCI200419139
111. Chernova I, Song W, Steach H, Hafez O, Al Souz J, Chen PM, et al. The ion transporter Na(+)-K(+)-ATPase enables pathological B cell survival in the kidney microenvironment of lupus nephritis. Sci Adv. (2023) 9:eadf8156. doi: 10.1126/sciadv.adf8156
112. Allison SJ. Mechanism of B cell survival in lupus nephritis. Nat Rev Nephrol. (2023) 19:213. doi: 10.1038/s41581-023-00696-y
113. Tang Y, Zhang Y, Li X, Xu R, Ji Y, Liu J, et al. Immune landscape and the key role of APOE+ monocytes of lupus nephritis under the single-cell and spatial transcriptional vista. Clin Transl Med. (2023) 13:e1237. doi: 10.1002/ctm2.v13.4
114. Der E, Ranabothu S, Suryawanshi H, Akat KM, Clancy R, Morozov P, et al. Single cell RNA sequencing to dissect the molecular heterogeneity in lupus nephritis. JCI Insight. (2017) 2(9):e93009. doi: 10.1172/jci.insight.93009
115. Der E, Suryawanshi H, Morozov P, Kustagi M, Goilav B, Ranabothu S, et al. Tubular cell and keratinocyte single-cell transcriptomics applied to lupus nephritis reveal type I IFN and fibrosis relevant pathways. Nat Immunol. (2019) 20:915–27. doi: 10.1038/s41590-019-0386-1
116. Zhu J, Lu J, Weng H. Single-cell RNA sequencing for the study of kidney disease. Mol Med. (2023) 29:85. doi: 10.1186/s10020-023-00693-8
117. Espeli M, Bokers S, Giannico G, Dickinson HA, Bardsley V, Fogo AB, et al. Local renal autoantibody production in lupus nephritis. J Am Soc Nephrol. (2011) 22:296–305. doi: 10.1681/ASN.2010050515
118. Nickerson KM, Smita S, Hoehn KB, Marinov AD, Thomas KB, Kos JT, et al. Age-associated B cells are heterogeneous and dynamic drivers of autoimmunity in mice. J Exp Med. (2023) 220(5):e20221346. doi: 10.1084/jem.20221346
119. Maz MP, Michelle Kahlenberg J. Cutaneous and systemic connections in lupus. Curr Opin Rheumatol. (2020) 32:583–9. doi: 10.1097/BOR.0000000000000739
120. Li Q, Wu H, Liao W, Zhao M, Chan V, Li L, et al. A comprehensive review of immune-mediated dermatopathology in systemic lupus erythematosus. J Autoimmun. (2018) 93:1–15. doi: 10.1016/j.jaut.2018.07.007
121. Patel J, Borucki R, Werth VP. An update on the pathogenesis of cutaneous lupus erythematosus and its role in clinical practice. Curr Rheumatol Rep. (2020) 22:69. doi: 10.1007/s11926-020-00946-z
122. Jenks SA, Wei C, Bugrovsky R, Hill A, Wang X, Rossi FM, et al. B cell subset composition segments clinically and serologically distinct groups in chronic cutaneous lupus erythematosus. Ann Rheum Dis. (2021) 80:1190–200. doi: 10.1136/annrheumdis-2021-220349
123. Chen Y, Yang M, Long D, Li Q, Zhao M, Wu H, et al. Abnormal expression of BAFF and its receptors in peripheral blood and skin lesions from systemic lupus erythematosus patients. Autoimmunity. (2020) 53(4):192–200. doi: 10.1080/08916934.2020.1736049
124. Wenzel J, Landmann A, Vorwerk G, Kuhn A. High expression of B lymphocyte stimulator in lesional keratinocytes of patients with cutaneous lupus erythematosus. Exp Dermatol. (2018) 27:95–7. doi: 10.1111/exd.2018.27.issue-1
125. de Vos L, Guel T, Niebel D, Bald S, Ter Steege A, Bieber T, et al. Characterization of B cells in lupus erythematosus skin biopsies in the context of different immune cell infiltration patterns. Front Med (Lausanne). (2022) 9:1037408. doi: 10.3389/fmed.2022.1037408
126. Chasset F, Ribi C, Trendelenburg M, Huynh-Do U, Roux-Lombard P, Courvoisier DS, et al. Identification of highly active systemic lupus erythematosus by combined type I interferon and neutrophil gene scores vs classical serologic markers. Rheumatol (Oxford). (2020) 59:3468–78. doi: 10.1093/rheumatology/keaa167
127. Niebel D, de Vos L, Fetter T, Bragelmann C, Wenzel J. Cutaneous lupus erythematosus: an update on pathogenesis and future therapeutic directions. Am J Clin Dermatol. (2023) 24:521–40. doi: 10.1007/s40257-023-00774-8
128. Chen Y, Tian B. IFN-gamma promotes the development of systemic lupus erythematosus through the IFNGR1/2-PSTAT1-TBX21 signaling axis. Am J Transl Res. (2022) 14:6874–88.
129. Xie Y, Jinnin M, Zhang X, Wakasugi S, Makino T, Inoue Y, et al. Immunohistochemical characterization of the cellular infiltrate in discoid lupus erythematosus. Biosci Trends. (2011) 5:83–8. doi: 10.5582/bst.2011.v5.2.83
130. Zhou S, Li Q, Zhou S, Zhao M, Lu L, Wu H, et al. A novel humanized cutaneous lupus erythematosus mouse model mediated by IL-21-induced age-associated B cells. J Autoimmun. (2021) 123:102686. doi: 10.1016/j.jaut.2021.102686
131. Abernathy-Close L, Lazar S, Stannard J, Tsoi LC, Eddy S, Rizvi SM, et al. B cell signatures distinguish cutaneous lupus erythematosus subtypes and the presence of systemic disease activity. Front Immunol. (2021) 12:775353. doi: 10.3389/fimmu.2021.775353
132. Shen B, Tan W, Feng G, He Y, Liu J, Chen W, et al. The correlations of disease activity, socioeconomic status, quality of life, and depression/anxiety in Chinese patients with systemic lupus erythematosus. Clin Dev Immunol. (2013) 2013:270878. doi: 10.1155/2013/270878
133. Raghunath S, Glikmann-Johnston Y, Vincent FB, Morand EF, Stout JC, Hoi A. Patterns and prevalence of cognitive dysfunction in systemic lupus erythematosus. J Int Neuropsychol Soc. (2023) 29:421–30. doi: 10.1017/S1355617722000418
134. Valdes Hernandez MDC, Smith K, Bastin ME, Nicole Amft E, Ralston SH, Wardlaw JM, et al. Brain network reorganisation and spatial lesion distribution in systemic lupus erythematosus. Lupus. (2021) 30(2):285–98. doi: 10.1177/0961203320979045
135. Salomonsson T, Rumetshofer T, Jonsen A, Bengtsson AA, Zervides KA, Nilsson P, et al. Abnormal cerebral hemodynamics and blood-brain barrier permeability detected with perfusion MRI in systemic lupus erythematosus patients. NeuroImage Clin. (2023) 38:103390. doi: 10.1016/j.nicl.2023.103390
136. Kamintsky L, Beyea SD, Fisk JD, Hashmi JA, Omisade A, Calkin C, et al. Blood-brain barrier leakage in systemic lupus erythematosus is associated with gray matter loss and cognitive impairment. Ann Rheum Dis. (2020) 79:1580–7. doi: 10.1136/annrheumdis-2020-218004
137. Ho RC, Thiaghu C, Ong H, Lu Y, Ho CS, Tam WW, et al. A meta-analysis of serum and cerebrospinal fluid autoantibodies in neuropsychiatric systemic lupus erythematosus. Autoimmun Rev. (2016) 15:124–38. doi: 10.1016/j.autrev.2015.10.003
138. Sarbu N, Alobeidi F, Toledano P, Espinosa G, Giles I, Rahman A, et al. Brain abnormalities in newly diagnosed neuropsychiatric lupus: systematic MRI approach and correlation with clinical and laboratory data in a large multicenter cohort. Autoimmun Rev. (2015) 14:153–9. doi: 10.1016/j.autrev.2014.11.001
139. Hanly JG, Walsh NM, Sangalang V. Brain pathology in systemic lupus erythematosus. J Rheumatol. (1992) 19:732–41.
140. Ellison D, Gatter K, Heryet A, Esiri M. Intramural platelet deposition in cerebral vasculopathy of systemic lupus erythematosus. J Clin Pathol. (1993) 46:37–40. doi: 10.1136/jcp.46.1.37
141. George-Chandy A, Trysberg E, Eriksson K. Raised intrathecal levels of APRIL and BAFF in patients with systemic lupus erythematosus: relationship to neuropsychiatric symptoms. Arthritis Res Ther. (2008) 10:R97. doi: 10.1186/ar2484
142. Mok MY, Chan EY, Wong WS, Lau CS. Intrathecal immunoglobulin production in patients with systemic lupus erythematosus with neuropsychiatric manifestations. Ann Rheum Dis. (2007) 66:846–7. doi: 10.1136/ard.2006.061069
143. Yang C, Hou X, Feng Q, Li Y, Wang X, Qin L, et al. Lupus serum IgG induces microglia activation through Fc fragment dependent way and modulated by B-cell activating factor. J Transl Med. (2019) 17:426. doi: 10.1186/s12967-019-02175-0
144. Wang A, Guilpain P, Chong BF, Chouzenoux S, Guillevin L, Du Y, et al. Dysregulated expression of CXCR4/CXCL12 in subsets of patients with systemic lupus erythematosus. Arthritis Rheumatol. (2010) 62:3436–46. doi: 10.1002/art.v62:11
145. Shen WY, Luo C, Hurtado PR, Liu XJ, Luo RY, Li H, et al. Up-regulation of proBDNF/p75(NTR) signaling in antibody-secreting cells drives systemic lupus erythematosus. Sci Adv. (2022) 8:eabj2797. doi: 10.1126/sciadv.abj2797
146. Wen J, Stock AD, Chalmers SA, Putterman C. The role of B cells and autoantibodies in neuropsychiatric lupus. Autoimmun Rev. (2016) 15:890–5. doi: 10.1016/j.autrev.2016.07.009
147. Wen J, Doerner J, Chalmers S, Stock A, Wang H, Gullinello M, et al. B cell and/or autoantibody deficiency do not prevent neuropsychiatric disease in murine systemic lupus erythematosus. J Neuroinflamm. (2016) 13(1):73. doi: 10.1186/s12974-016-0537-3
148. Abdelaziz MM, Fathi N, Hetta HF, Abdel-Galeel A, Zidan M, Shawky EM, et al. Regulatory B cells evaluation in systemic lupus erythematosus patients with subclinical atherosclerosis and secondary antiphospholipid syndrome. Mediterr J Rheumatol. (2023) 34:486–94. doi: 10.31138/mjr.03823.rbc
149. Sakaguchi Y, Nakamura Y, Sutani T, Tsuchihashi M, Yamano S, Hashimoto T, et al. Immunohistochemical study of the endomyocardial biopsy of systemic lupus erythematosus. J Cardiol. (1995) 25:181–8.
150. Zhuang H, Han S, Lee PY, Khaybullin R, Shumyak S, Lu L, et al. Pathogenesis of diffuse alveolar hemorrhage in murine lupus. Arthritis Rheumatol. (2017) 69:1280–93. doi: 10.1002/art.40077
151. Pecher AC, Hensen L, Klein R, Schairer R, Lutz K, Atar D, et al. CD19-targeting CAR T cells for myositis and interstitial lung disease associated with antisynthetase syndrome. JAMA. (2023) 329(24):2154–62. doi: 10.1001/jama.2023.8753
152. Pottier V, Pierrot M, Subra JF, Mercat A, Kouatchet A, Parrot A, et al. Successful rituximab therapy in a lupus patient with diffuse alveolar haemorrhage. Lupus. (2011) 20:656–9. doi: 10.1177/0961203310386276
153. Na JO, Chang SH, Seo KH, Choi JS, Lee HS, Lyu JW, et al. Successful early rituximab treatment in a case of systemic lupus erythematosus with potentially fatal diffuse alveolar hemorrhage. Respiration. (2015) 89(1):62–5. doi: 10.1159/000369038
154. Oktem O, Guzel Y, Aksoy S, Aydin E, Urman B. Ovarian function and reproductive outcomes of female patients with systemic lupus erythematosus and the strategies to preserve their fertility. Obstet Gynecol Surv. (2015) 70:196–210. doi: 10.1097/OGX.0000000000000160
155. Han YF, Yan Y, Wang HY, Chu MY, Sun K, Feng ZW, et al. Effect of systemic lupus erythematosus on the ovarian reserve: A systematic review and meta-analysis. Joint Bone Spine. (2024) 91:105728. doi: 10.1016/j.jbspin.2024.105728
156. Surawut S, Panpetch W, Makjaroen J, Tangtanatakul P, Thim-Uam A, Wongphoom J, et al. Helicobacter pylori infection increased anti-dsDNA and enhanced lupus severity in symptomatic fcgammaRIIb-deficient lupus mice. Front Microbiol. (2018) 9:1488. doi: 10.3389/fmicb.2018.01488
157. Apperloo-Renkema HZ, Bootsma H, Mulder BI, Kallenberg CG, van der Waaij D. Host-microflora interaction in systemic lupus erythematosus (SLE): colonization resistance of the indigenous bacteria of the intestinal tract. Epidemiol Infect. (1994) 112:367–73. doi: 10.1017/S0950268800057770
158. Silverman GJ, Deng J, Azzouz DF. Sex-dependent Lupus Blautia (Ruminococcus) gnavus strain induction of zonulin-mediated intestinal permeability and autoimmunity. Front Immunol. (2022) 13:897971. doi: 10.3389/fimmu.2022.897971
159. Cai B, Lu H, Ye Q, Xiao Q, Wu X, Xu H. Identification of potent target and its mechanism of action of Tripterygium wilfordii Hook F in the treatment of lupus nephritis. Int J Rheum Dis. (2023) 26:1529–39. doi: 10.1111/1756-185X.14780
160. Xia Y, Jiang C, Yang M, Liu T, Zou X, Li C, et al. SB431542 alleviates lupus nephritis by regulating B cells and inhibiting the TLR9/TGFβ1/PDGFB signaling. J Autoimmun. (2022) 132:102894. doi: 10.1016/j.jaut.2022.102894
161. Zhang J, Wang X, Wang R, Chen G, Wang J, Feng J, et al. Rapamycin treatment alleviates chronic GVHD-induced lupus nephritis in mice by recovering IL-2 production and regulatory T cells while inhibiting effector T cells activation. Biomedicines. (2023) 11(3):949. doi: 10.3390/biomedicines11030949
162. Muchamuel T, Fan RA, Anderl JL, Bomba DJ, Johnson HWB, Lowe E, et al. Zetomipzomib (KZR-616) attenuates lupus in mice via modulation of innate and adaptive immune responses. Front Immunol. (2023) 14:1043680. doi: 10.3389/fimmu.2023.1043680
163. Evans LS, Lewis KE, DeMonte D, Bhandari JG, Garrett LB, Kuijper JL, et al. Povetacicept, an enhanced dual APRIL/BAFF antagonist that modulates B lymphocytes and pathogenic autoantibodies for the treatment of lupus and other B cell-related autoimmune diseases. Arthritis Rheumatol. (2023) 75:1187–202. doi: 10.1002/art.42462
164. Krustev E, Clarke AE, Barber MRW. B cell depletion and inhibition in systemic lupus erythematosus. Expert Rev Clin Immunol. (2023) 19:55–70. doi: 10.1080/1744666X.2023.2145281
165. Rovin BH, Furie R, Latinis K, Looney RJ, Fervenza FC, Sanchez-Guerrero J, et al. Efficacy and safety of rituximab in patients with active proliferative lupus nephritis: the Lupus Nephritis Assessment with Rituximab study. Arthritis Rheumatol. (2012) 64:1215–26. doi: 10.1002/art.34359
166. Plüß M, Piantoni S, Tampe B, Kim AHJ, Korsten P. Belimumab for systemic lupus erythematosus - Focus on lupus nephritis. Hum Vaccin Immunother. (2022) 18(5):2072143. doi: 10.1080/21645515.2022.2072143
167. Ramsköld D, Parodis I, Lakshmikanth T, Sippl N, Khademi M, Chen Y, et al. B cell alterations during BAFF inhibition with belimumab in SLE. EBioMedicine. (2019) 40:517–27. doi: 10.1016/j.ebiom.2018.12.035
168. Stohl W, Hiepe F, Latinis KM, Thomas M, Scheinberg MA, Clarke A, et al. Belimumab reduces autoantibodies, normalizes low complement levels, and reduces select B cell populations in patients with systemic lupus erythematosus. Arthritis Rheumatol. (2012) 64:2328–37. doi: 10.1002/art.34400
169. Dooley MA, Houssiau F, Aranow C, D’Cruz DP, Askanase A, Roth DA, et al. Effect of belimumab treatment on renal outcomes: results from the phase 3 belimumab clinical trials in patients with SLE. Lupus. (2013) 22:63–72. doi: 10.1177/0961203312465781
170. Shi F, Xue R, Zhou X, Shen P, Wang S, Yang Y. Telitacicept as a BLyS/APRIL dual inhibitor for autoimmune disease. Immunopharmacol Immunotoxicol. (2021) 43:666–73. doi: 10.1080/08923973.2021.1973493
171. Ji L, Geng Y, Zhang X, Deng X, Song Z, Tan M, et al. B cell pathway dual inhibition for systemic lupus erythematosus: a prospective single-arm cohort study of telitacicept. MedComm (2020). (2024) 5:e515. doi: 10.1002/mco2.v5.4
172. Zeng L, Yang K, Wu Y, Yu G, Yan Y, Hao M, et al. Telitacicept: A novel horizon in targeting autoimmunity and rheumatic diseases. J Autoimmun. (2024) 148:103291. doi: 10.1016/j.jaut.2024.103291
173. Lyu X, Gupta L, Tholouli E, Chinoy H. Chimeric antigen receptor T cell therapy: a new emerging landscape in autoimmune rheumatic diseases. Rheumatol (Oxford). (2024) 63:1206–16. doi: 10.1093/rheumatology/kead616
174. Müller F, Taubmann J, Bucci L, Wilhelm A, Bergmann C, Völkl S, et al. CD19 CAR T-cell therapy in autoimmune disease - A case series with follow-up. N Engl J Med. (2024) 390(8):687–700. doi: 10.1056/NEJMoa2308917
Keywords: systemic lupus erythematosus, B cell, organ damage, organ specific features, therapy strategies
Citation: Wang Y, Zhao R, Liang Q, Ni S, Yang M, Qiu L, Ji J, Gu Z and Dong C (2025) Organ-based characterization of B cells in patients with systemic lupus erythematosus. Front. Immunol. 16:1509033. doi: 10.3389/fimmu.2025.1509033
Received: 10 October 2024; Accepted: 06 January 2025;
Published: 23 January 2025.
Edited by:
Joerg Wenzel, University Hospital Bonn, GermanyReviewed by:
Saeed Mohammadi, Golestan University of Medical Sciences, IranShigeru Iwata, Wakayama Medical University, Japan
Erin Taylor, University of Mississippi Medical Center, United States
Copyright © 2025 Wang, Zhao, Liang, Ni, Yang, Qiu, Ji, Gu and Dong. This is an open-access article distributed under the terms of the Creative Commons Attribution License (CC BY). The use, distribution or reproduction in other forums is permitted, provided the original author(s) and the copyright owner(s) are credited and that the original publication in this journal is cited, in accordance with accepted academic practice. No use, distribution or reproduction is permitted which does not comply with these terms.
*Correspondence: Zhifeng Gu, Z3V6ZkBudHUuZWR1LmNu; Chen Dong, ZG9uZ2NoZW5zY0AxMjYuY29t
†These authors have contributed equally to this work