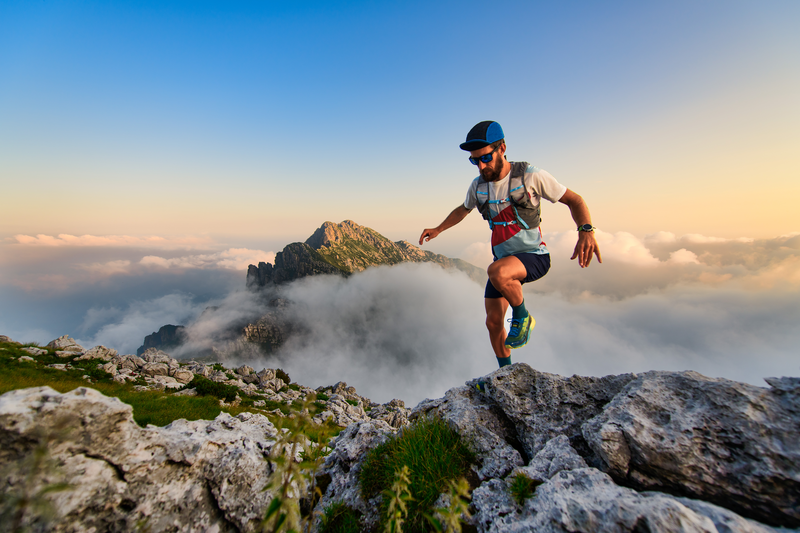
95% of researchers rate our articles as excellent or good
Learn more about the work of our research integrity team to safeguard the quality of each article we publish.
Find out more
REVIEW article
Front. Immunol. , 14 March 2025
Sec. Cancer Immunity and Immunotherapy
Volume 16 - 2025 | https://doi.org/10.3389/fimmu.2025.1506526
Hepatitis B virus (HBV) infection is a well-documented independent risk factor for developing hepatocellular carcinoma (HCC). Consequently, extensive research has focused on elucidating the mechanisms by which HBV induces hepatocarcinogenesis. The majority of studies are dedicated to understanding how HBV DNA integration into the host genome, viral RNA expression, and the resulting protein transcripts affect cellular processes and promote the malignant transformation of hepatocytes. However, considering that most acute HBV infections are curable, immune suppression potentially contributes to the critical challenges in the treatment of chronic infections. Regulatory T cells (Tregs) are crucial in immune tolerance. Understanding the interplay of Tregs within the liver microenvironment following HBV infection could offer novel therapeutic approaches for treating HBV infections and preventing HBV-related HCC. Two viewpoints to targeting Tregs in the liver microenvironment include means of reducing their inhibitory function and decreasing Treg frequency. As these strategies may disrupt the immune balance and lead to autoimmune responses, careful and comprehensive profiling of the patient’s immunological status and genetic factors is required to successfully employ this promising therapeutic approach.
Hepatitis B virus (HBV) is a pararetrovirus discovered by American geneticist Baruch Blumberg in 1965. HBV is an enveloped, double-stranded DNA virus belonging to the genus Orthohepadnavirus and a member of the Hepadnaviridae family. HBV viral particles comprise a double-stranded DNA genome in the form of relaxed circular DNA (rcDNA), containing 3,020–3,320 nucleotides within a capsid made up of hepatitis B core antigen (HBcAg) subunits. This nucleocapsid is encased within a lipid bilayer membrane derived from the host, covered by hepatitis B surface antigen (HBsAg). There are 10 HBV genotypes (A to J), with Genotypes A–D and F being implicated in the development of hepatocellular carcinoma (HCC) (1). The HBV genome contains four overlapping open reading frames (ORFs), namely C, S, P, and X. The C ORF encodes two proteins: the hepatitis B core protein (HBc) and the hepatitis B e antigen (HBe). The S ORF includes the preS1, preS2, and hepatitis B surface antigen (HBs) domains and encodes three envelope proteins, namely the large, middle, and small HBs antigens (HBsAg). The P and X ORFs contain the viral polymerase and HBx genes, which encode the polymerase and viral transcriptional activator HBx proteins, respectively.
HBV infection is the most prevalent chronic viral infection worldwide. The World Health Organization data indicates that 254 million people were living with chronic HBV infection in 2022, with 1.1 million new infections and approximately 1 million deaths, mostly owing to cirrhosis or HCC. HBV was the second leading cause of death from infectious diseases in 2022, second only to COVID-19. In many countries, numerous individuals remain undiagnosed, and even when hepatitis is confirmed, the population that receives treatment is low (2). By the end of 2022, approximately 21% of individuals diagnosed with hepatitis B had received treatment (3). Infection is unevenly distributed worldwide, with sub-Saharan Africa and Southeast Asia being high-prevalence regions. HBV is most commonly transmitted from mother to child at birth (vertical transmission) and from an infected child to a susceptible individual during early childhood (horizontal transmission). The latter carries a 90% risk of developing chronic hepatitis B (CHB) infection (4). The cure for CHB is lacking; however, effective treatments with close monitoring reduce the associated morbidity and mortality (5).
Hepatitis B infection continues to be a significant global burden, impacting millions of people worldwide. Many individuals with HBV remain undiagnosed, leading to delayed treatment and increased viral transmission. Although an effective vaccine is available, vaccination coverage is thought to be low in certain populations. Current treatments for HBV can manage the infection but do not offer a cure, highlighting the urgent need for the development of curative therapies. It is well known that chronic inflammation plays a crucial role in the onset, promotion, and progression of cancer. Chronic HBV infection are characterized by tissue inflammation with subsequent hepatocellular death, leading to continuous immune activation. Even though immune activation contributes to the restoration of tissue function, a heightened or prolonged immune response may lead to the replacement of hepatic parenchyma by fibrotic tissue and vascular architectural distortion, leading to liver cirrhosis and probably HCC (6, 7). This procedure, first postulated by Virchow back in 1863, is now called the “hepatic inflammation–fibrosis–cancer axis” (8), underscoring the importance of early diagnosis and effective management.
Primary liver cancer is a common malignant tumor worldwide, characterized by insidious onset, long latency period, rapid progression, and poor prognosis, with a 5-year survival rate of only 10–18%, of which HCC accounts for approximately 75–85% (9). Liver cancer ranks sixth in incidence among all malignant tumors worldwide and is the third leading cause of cancer-related mortality (10). CHB infection is a major risk factor for HCC and constitutes approximately 50–80% of HCC cases (11). As HBV infection accounts for most of the global liver cancer deaths; therefore, targeting HBV represents a primary strategy for combatting HCC (12).
HBV infection is broadly categorized into two types, namely acute and chronic hepatitis. HBV infection causes various clinical symptoms, from asymptomatic or mild to severely life-threatening (13). Acute HBV infection is self-limiting and is associated with only a few acute inflammation or hepatocellular necrosis cases and a case fatality rate of 0.5–1% (13, 14). CHBV progresses to advanced liver fibrosis, cirrhosis, and HCC (15), and international guidelines recommend antiviral therapies such as interferon-α (IFN-α) and nucleoside analogs for its treatment (16). Although antiviral therapy has demonstrated a pronounced effect in reducing the incidence of HCC in patients with CHB infection (17–19), the prolonged in vivo survival of HBV is caused by the persistent replication of its DNA in hepatocyte nuclei and the resulting low immune system response. In acute HBV infection, the virus is eradicated by the immune system, with the innate and adaptive immune cells playing a crucial role. As HBV infection progresses to a chronic state, it fosters an immune-tolerant microenvironment in the liver by recruiting Foxp3+ T regulatory cells (Tregs) and inactivating CD8+ T cells, creating favorable conditions for the development of HBV-related HCC (20, 21). Tregs are crucial in maintaining immune tolerance. It is hypothesized that reducing Treg-mediated immunosuppression enhances the quantity and functionality of effector immune cells, improving immune surveillance capabilities (22, 23). Therefore, understanding the interaction mechanisms between the liver microenvironment and immune cells following HBV infection, as well as the regulatory role of Tregs on effector immune cells, may offer new insights for diagnosing and treating HBV infection and HBV-related HCC.
HBV is a hepatotropic virus that infects the hepatocytes by binding to the sodium taurocholate cotransporting polypeptide receptor and entering the cell via receptor-mediated endocytosis (24). However, studies have revealed additional hepatocyte receptor proteins, such as transferrin, desialylated glycoprotein, immunoglobulin A receptor, and human hepatic endothelin receptor, as potential binding receptors for HBV (25–29). Upon entering the host cell, HBV releases relaxed circular genomic DNA (rcDNA) into the nucleus. Subsequently, host cell DNA repair enzymes convert HBV rcDNA into the covalently closed circular DNA (cccDNA), which serves as a template for all viral mRNAs (Figure 1). The core protein mRNA, known as pregenomic RNA (pgRNA), serves as the template for the core protein and viral DNA polymerase. Once synthesized, the core protein packages the pgRNA into core particles. Within these particles, the viral DNA polymerase reverse-transcribes the pgRNA into rcDNA (Figure 1) (30). Core particles containing rcDNA may interact with HBsAg in the intracellular membrane to form mature viral particles that are released from the infected hepatocytes. They may also return rcDNA to the nucleus for repair and the amplification of the cccDNA pool. During the reverse transcription of pgRNA, partial double-stranded rcDNA is formed 90% of the time, with the synthesis of double-stranded linear DNA (dslDNA) constituting 10% of the reverse transcription outcome (Figure 1) (31). In contrast to rcDNA, nuclear dslDNA forms replication-deficient cccDNA or integrates into the host cell genome (31–33). Previous studies have shown that integrated HBV DNA may also participate in the synthesis of viral RNA transcripts; however, this integration is not an essential step in the viral life cycle because it does not produce a replication-competent virus (34–36).
HBV dysregulates several pathways in infected hepatocytes, resulting in the development of HCC; however, the exact mechanism is poorly defined. HBV DNA integration reportedly increases genetic instability in infected hepatocytes and is crucial in the progression to HCC (37). A multifunctional HBx protein is significant in HBV pathogenesis and HCC development (38). This HBx protein interferes with multiple signaling pathways, including Wnt/β-catenin and PI3K/Akt/mTOR. The dysregulation of these pathways promotes cell proliferation and survival, resulting in cancerous cell development (38, 39). HBx reportedly interferes with the cell cycle checkpoint, leading to uncontrolled cell division. The HBeAg is among the crucial antigens involved in the HBV infection and the progression to HCC. The presence of HBeAg in the patient’s serum indicates active viral replication with high infectivity, contributing to immune tolerance by inhibiting lymphocyte proliferation and reducing IFN-γ production. HBeAg also impairs T-cell function by increasing programmed cell death-1 (PD-1) and toll-like receptor expression, leading to a weakened immune response and persistent infection. In CHB carriers, HBeAg is associated with liver inflammation and damage, increasing the risk of liver cirrhosis and HCC (40, 41). Given the complex viral biology of HBV and the interplay with host cells, understanding the molecular biology of HBV is crucial in improving the diagnosis, treatment, and prevention of HBV-related diseases, including HCC.
NK cells constitute 25–50% of hepatic lymphocytes, indicating their central role in innate immunity (42, 43). NK cells are associated with early antiviral and anti-tumor responses (44). In virally infected patients, exposed viral nucleotides, host cell debris, and viral proteins activate various cellular receptors, leading to direct antiviral effects by NK cells through perforin, tumor necrosis factor-related apoptosis-inducing ligand (TRAIL), and the induction of IFN-γ production (Figure 2) (45–47). However, HBV may exert immunosuppressive effects by reducing its amplification rate, stimulating TGF-β and IL-10 production and inhibiting the Toll-like receptor 2-induced TNF-α and IL-12 secretion. HBV dissemination within the NK cells is reportedly mediated by HBV-positive exosomes. These exosomes impair NK cell function, affecting IFN-γ production, cytolytic activity, and NK cell proliferation and survival (48). HBV infection induces high IL-10 expression, inhibiting IFN-γ secretion by NK cells and driving the expression of inhibitory receptors PD-1 and CD94 on NK cells. Persistent binding of PD-1/PD-L1 and cytotoxic T-lymphocyte antigen 4 (CTLA4)/CD94 immune checkpoint proteins maintains CD8+ T cell stimulation, leading to T cell insufficiency (49). Thus, the NK cell-mediated depletion of antigen-specific CD8+ T cells impairs adaptive antiviral immunity in patients with CHB infection and contributes to viral persistence (50). In addition, NK cells retain their cytotoxic potential in CHB infection through the upregulation of TRAIL (51). However, they also target and eliminate HBV-specific CD8+ T cells that express high levels of TRAIL death receptors. In CHB infection, NK cells are usually considered more pathogenic than protective, primarily owing to their impaired antiviral cytokine production (52). Therefore, NK cell-based therapeutic strategies should aim to enhance the ability of NK cells to target and destroy virus-infected cells and to reduce the inhibitory effects of NK cells on virus-specific T cells.
Figure 2. Diagram illustrating how different types of immune cell responses affect the outcome of HBV infection after it enters into liver cells. In the microenvironment of acute HBV infection, the effective response of effector immune cells drives the control of the infection. As HBV infection progresses towards chronicity, the influence of cells that promote immune tolerance leads to a shift from the dominance of effector immune cells to immune-tolerant cells, facilitating the spread of the infection. HBV, Hepatitis B virus; NK, Natural killer cells; NKT, Natural killer T cells; Treg, Regulatory T cells; Breg, Regulatory B cells; B, B lymphocytes; CD8+ T, Cytotoxic T lymphocytes; MDSC, Myeloid-derived suppressor cells; Kupffer, Kupffer cells; Th1, Helper T 1 cells; Th17, Helper T 17 cells; M1, M1 macrophages; M2, M2 macrophages.
KCs are in the hepatic sinusoids and represent the largest population of hepatic immune cells (53). In the liver microenvironment following HBV infection, KCs and MNCs play a crucial role in creating an immune-tolerant environment that accelerates disease progression (Figure 2). KCs facilitate CD8+ T cell depletion after HBV infection in mice through interactions with HBcAg (54). A significant mechanism by which CD8+ T cells produce IFN-γ is by directing macrophages to produce cytokines and chemokines that promote fibrosis, such as TNF-α, IL-6, and Monocyte Chemoattractant Protein-1 (55). This process contributes to the progression of CHB to HCC. In the liver microenvironment following HBV infection, KCs and MNCs activate the immune response. For example, circulatory CD14+ monocytes in patients with CHB infection activate CD8+ T cells by upregulating CD137 ligands (55). In mice with CHB infection, the IL-10 released by KCs is crucial for supporting humoral immunity. The dual role of KCs and MNCs on the immune response may be similar to the role of NK cells in CHB infection, both contributing to the protection of hepatocytes from excessive liver inflammation and the maintenance of long-term survival of the organism; however, they affect the antiviral potency. The interplay between KCs and MNCs in the liver microenvironment is critical in shaping the immune response to HBV, usually leading to an immune-tolerant state that benefits virus persistence and disease progression.
MDSCs are a regulatory immune cell population comprising innate immune cells in the liver. A previous study showed that the MDSCs from the liver tissue of HBV transgenic mice suppressed the proliferation of HBsAg-specific allogeneic T cells and lymphocytes (Figure 2) (56). The possible cause was the continuous exposure to high HBsAg levels, which induced MDSC expansion, inhibiting the proliferation of effector T cells by promoting the infiltration of Tregs into the liver microenvironment following HBV infection (Figure 2) (57, 58). MDSCs express and secrete high TGF-β and IL-10 levels, promoting the peripheral transformation of CD4+ T cells to induced Tregs and inducing Treg proliferation (Figure 2) (58–61).
Earlier studies have highlighted the crucial role of antigen-specific CD4+ and CD8+ T cells in preventing viral tropism (62, 63). Effective CD4+ helper T cell and CD8+ effector T cell responses have been observed in patients with hepatitis (64, 65) and experimentally infected chimpanzees (66) to clear HBV infection. In contrast, B cells exert potent antiviral effects primarily through the production of antiviral antibodies and the presentation of antigens to T cells. With the increased infiltration of Tregs and other immunomodulatory cells in the liver microenvironment following HBV infection, adaptive immune cells, which are crucial for the immune response to HBV, undergo gradual exhaustion (67). As the disease progresses, the resulting liver microenvironment following HBV infection impairs the normal proliferative, transcriptional, epigenetic, and metabolic activities of hepatocytes, significantly increasing the risk of developing HCC (49).
As a component of the acquired immune system and a major participant in humoral immunity, B cells are crucial in combating HBV infection. In the early stage of HBV infection, B cells mediate the antiviral response primarily by producing antibodies such as anti-HBs and anti-HBc (Figure 2) (68). Additionally, B cells activate T cell-mediated responses by presenting HBcAg to effector T cells (69). Similarly, effector B cells secrete pro-inflammatory cytokines such as IL-6 and IFN-γ, which influence the differentiation of CD4+ T cells into Th1 cells (Figure 2). As HBV infection progresses to a chronic state, PD-1 expression on specific B cells increases, and these cells exhibit atypical memory phenotypes (CD21- and CD27-), resulting in impaired production of anti-HBs antibodies (70). The absence of B-cell antibody production limits HBV clearance, leading to persistent inflammation and promoting the progression of liver disease (71).
The HBV-induced chronic inflammatory microenvironment drives an increased frequency of B cell differentiation into regulatory B cells (Bregs), enhancing the production of immunosuppressive cytokines such as IL-10 and TGF-β (72–74). Bregs orchestrate the immunosuppressive environment to limit tissue damage under chronic inflammatory conditions; however, this allows the immune surveillance system to evade tumors (72–75). Initial studies in the early 2000s attributed this immunomodulation to IL-10, which became the hallmark of Breg suppression (76, 77); however, this notion has lately expanded as new Breg-derived suppressive mediators have been discovered. Human Breg subsets have been identified in various B cell subgroups, and their immunosuppressive functions can be summarized as follows (75): a) IL-10+ Bregs inhibit Th1, Th17, and CD8+ T cell responses; convert naïve CD4+ T cells into regulatory T cell populations; and modulate pro-inflammatory innate cells through the production of IL-10. b) Likewise, TGF-β+ Bregs operate on naïve CD4+ T cells to generate FoxP3+ Tregs, in addition to induce anergy in CD4+ and CD8+ T cells. c) IL-35+ Bregs can promote “infectious tolerance” by inducing IL-35-producing Tregs and expanding the generation of IL-35+ Bregs. d) GrB+ (Granzyme B) B cells have been shown to inhibit Th1 and Th17 cell responses and to reduce CD4+ T cell proliferation by degrading the TCR ζ-chain. Breg cells have been described in various human cancers and are often associated with cancer progression. A higher frequency of IL-10-producing B cells was observed in late-stage esophageal cancer samples compared to early-stage samples, suggesting that Breg cells play a role in the progression of esophageal cancer (78). IL-10 producing B cells were also detected in the tumors of patients with gastric cancer, breast cancer, head and neck squamous carcinoma, and esophageal squamous carcinoma (79–82). B lymphocytes are crucial in combatting HBV infection through antibody production and immune activation; however, the loss of antibody production function in B cells and the increased proportion of Bregs in patients with hepatitis B contribute to HBV-induced liver cancer (Figure 2).
During acute HBV infection, HBV-specific CD8+ T cells produce pro-inflammatory cytokines such as IFN-γ, IL-2, and TNF-α, as well as cytotoxic molecules, including granzymes and perforins, to control the infection (Figure 2) (68). The chronic phase of HBV infection is characterized by various circulating HBV-specific CD8+ T cells, including HBsAg-, HBcAg-, and HBV polymerase- CD8+ T cells (83). Virus-specific CD8+ T cells are crucial in mediating effective antiviral immune responses during CHB infection. However, in CHB infection, HBV-specific CD8+ T cells are progressively exhausted. This exhaustion is driven by several mechanisms, including sustained high viral loads, increased Tregs within the liver microenvironment following HBV infection, and the presence of immunosuppressive cytokines such as IL-10 and TGF-β (68, 84, 85). Exhausted T cells are prone to apoptosis owing to the upregulation of TRAIL-2 and the pro-apoptotic mediator Bcl-2 interacting mediator of cell death (50, 86). In addition to diminishing immune surveillance, the exhaustion of CD8+ T cells contributes to chronic liver inflammation. During the immune tolerance phase, inflammatory cytokines produced by effector CD8+ T cells, such as IFN-γ, TNF, IL-17A, and IL-22, are significantly elevated in patients compared with those in healthy individuals (87). Thus, exhausted CD8+ T cells remain partially activated in response to the persistent viral antigen load, inducing ongoing and chronic hepatocellular damage. Chronic liver inflammation accelerates hepatocyte apoptosis, necrosis, and regeneration, resulting in recurrent DNA damage, genomic instability, and mutation accumulation that drive HCC tumorigenesis (88–90). Exhausted CD8+ effector T cells impair the adaptive immune system’s surveillance of tumors, allowing tumor cells to evade immune detection (Figure 2). Exhausted HBV-specific CD8+ T cells characteristically express various co-inhibitory receptors, including PD-1, CTLA-4, T-cell immunoglobulin and mucin domain 3, and T cell immune receptor with Ig and Immunoreceptor Tyrosine-based Inhibitory Motif (ITIM) domains (91–94). The expression of these co-inhibitory receptors in HBV-specific CD8+ T cells is associated with high viral loads and reflects the phenotypic and functional characteristics of T cell exhaustion (94). These co-inhibitory receptors, also known as immune checkpoints, have been extensively studied, and some drugs targeting them have shown varying degrees of efficacy in HCC treatment.
Naïve CD4+ T cells differentiate into multiple effector T cell subsets. Each subset produces distinct cytokines that drive different immune responses. Th1 cells produce pro-inflammatory cytokines such as IFN-γ, TNF-α, and IL-2, which are vital in anti-tumor and anti-viral immunity by inducing CD8+ T cell activation and increasing autoimmune activity (Figure 2) (95). HBV-associated HCC tissues exhibit a lower frequency of Th1 cells than do corresponding non-tumor tissues, and a low frequency of Th1 cells is significantly negatively correlated with disease-free survival (96). Th17 cells produce IL-17, IL-21, IL-22, IL-26, and TNF-α. During CHB infection, the balance of circulating helper T cells shifts from Th1 dominance to Th17 dominance (Figure 2). As Th17 cells are related to high viral loads and substantial liver injury, this shift towards Th17 dominance is associated with advanced liver disease and a poor prognosis in CHB-related liver disease (97). An increased Th17/Th1 ratio has been identified as an independent predictor of poor disease-free and overall survival in patients undergoing hepatectomy for HCC (96). CD4+ cytotoxic T cells secrete granzymes and perforins that exert the direct killing of infected hepatocytes, which depends on the direct recognition of target cells via major histocompatibility complex Class II receptors. In HBV-associated HCC, CD4+ cytotoxic T cells are progressively reduced as HCC progresses, and a reduced number and/or functional impairment of CD4+ cytotoxic T cells correlates with poor clinical outcomes (98). In patients with HBV-associated HCC, CD4+ cytotoxic T cell defects have been linked to a high Treg population (98). Several immunosuppressive mechanisms have been reported for Tregs (99, 100), including the (1) secretion of immunosuppressive cytokines (TGF-β, IL-10, and IL-35), (2) direct killing of effector cells or dendritic cells through granzyme and perforin-mediated cytolysis, (3) suppression of target cells via CTLA4 interactions with CD80/CD86 on T effector cells or dendritic cells, and (4) induction of apoptosis in conventional T cells through profound IL-2 depletion. Thus, Tregs establish an immunosuppressive microenvironment conducive to HBV chronicity and HCC formation by compromising the immunosurveillance functions of the innate and adaptive immune systems.
During viral infection, immune cells release cytokines that are essential for resolving the infection and eliminating infected cells. Tregs are crucial in maintaining immune tolerance and regulating excessive immune activation by preventing immune cells from attacking uninfected self-cells (101–103). Increased Treg frequency inhibits CD8+ and CD4+ T cell activities, preventing inflammatory liver injury and benefiting the host, and simultaneously shields the virus from immune attack by these T cells (104, 105). This dual effect contributes to disease progression and the development of HCC. An HBV infection study involving mice revealed that in a self-limiting HBV acute infection model, the frequency of liver Tregs briefly peaked before returning to baseline (106). In contrast, in the CHB infection model, Tregs underwent substantial expansion, constituting approximately 20–30% of liver CD4+ T cells, and this expansion persisted for over 200 days. HBV-induced hepatic fibrosis showed a similar trend, where the number of Tregs is increased in advanced HBV-associated hepatic fibrosis compared with that in early HBV fibrosis (107). A study (108) involving the measurement of the number of circulating and liver-resident CD4+CD25+ Tregs in patients with CHB and individuals with HCC showed that the frequency of circulating CD4+CD25+ Tregs did not differ significantly between them. However, among patients with HBsAg-positive HCC, circulating CD4+CD25+ Tregs were more frequent than those in individuals with HBsAg-negative HCC, with a 5% difference. Additionally, analysis of Tregs in acute, chronic, and chronic severe HBV infections showed that their numbers were higher in chronic severe HBV infections than in acute or chronic HBV infections (109, 110). These studies demonstrate the significance of Tregs in the pathogenesis of CHB infections and tumor immunity. Increased Tregs in patients with CHB inhibit specific anti-tumor immune responses (Figure 2). Furthermore, the HBV transfection of HCC cell lines caused increased frequency of CD4+CD25+ Tregs and pronounced suppression of tumor antigen-specific CD8+ T cell responses. Data from Xus et al. and Franzeses et al. indicated that patients with CHB and higher serum HBV DNA levels had significantly increased circulating CD4+CD25+ Tregs than did healthy controls, and addressing the exhaustion of these Tregs enhanced antigen-specific CD8+ T cell function in those with CHB (109, 111). In the liver microenvironment following HBV Infection, the increased Treg frequency induces immune dysfunction, resulting in viral persistence, chronic tissue damage, cirrhosis, and progression to HCC (Figure 2) (109, 112). These results indicate that the frequency of Tregs in the CHB microenvironment is crucial in the progression of viral infection and is significant in preventing the development of CHB-related HCC. Understanding the specific mechanisms by which Tregs inhibit immune cells could be crucial for restoring immune cell activity in the liver microenvironment following HBV infection.
Tregs include natural Tregs, which emerge from the thymus, and induced from the peripheral inflammatory microenvironment (113). The induced Tregs are the predominant types in tumors (114) and are classified into three subpopulations, namely Tr1, Th3, and iTR35. Tr1 subpopulation: This subset is characterized by CD4, CD18, CD49b, LAG3, and GATA3 expression, while lacking FOXP3 and exhibiting relatively high CD25 levels (115). Tr1 cells are functionally impaired in vitro; they suppress immune cell proliferation by producing IL-10 (116). Th3 subpopulation: they produce TGF-β, IL-4, and IL-10. A study suggests (117) that Th3 cells may also express the surface molecules typically on natural Tregs, such as CD25, FOXP3, and CTLA4. The iTR35 subpopulation is IL-35-producing CD4+ T cells. IL-35, which comprises two subunits, IL-12p35 and Ebi3, reportedly promotes the development of Tregs that mediate suppression in an IL-35-dependent manner (118). Under homeostatic conditions, Tregs exhibit reduced recirculation and tissue infiltration compared with those of conventional T cells. However, under pathological conditions, Tregs show increased recirculation to inflamed, tumor, and infection sites through lymphoid tissues (119). In humans and other mammals, Tregs prevent autoantigenic responses by suppressing innate (e.g., NK cells) and adaptive (e.g., T and B lymphocytes) immune cells (120, 121). Releasing the Treg-induced suppression of immune cells enhances the immune response of these cells against HBV or tumors (111, 122). Therefore, understanding the specific mechanisms of Treg interactions with immune cells could be beneficial for controlling HBV infection and treating HCC.
It is well established that the number and function of anti-infective and anti-tumor immune cells are determinants of disease progression, among which effector T cells are crucial. Tregs primarily function is to maintain immune tolerance and prevent peripheral autoimmunity through the secretion of immunosuppressive cytokines (TGF-β, IL-10, and IL-35), the expression of CTLA4, and competition for IL-2, which inhibits the secretion and proliferation of cytokines (granzymes A and B, perforin, and γ-interferon) from effector T cells to maintain immune tolerance and prevent autoimmunity in the periphery (Figure 3) (123). Studies have also shown that Tregs directly kill effector immune cells through the galectin-1-, granzyme B-, and TRAIL pathways, inhibiting their anti-infection and anti-tumor activities (99, 100). Recent studies revealed that Tregs secrete extracellular vesicles (EVs) as a novel inhibitory mechanism, capable of modulating the immune response in a manner independent of cell contact or targeting. Treg-derived EVs suppress effector T cell-mediated responses by transferring packaged Let-7d miRNA to Th1 cells, inhibiting their proliferation and IFN-γ secretion (124). Aiello et al. further demonstrated that EVs derived from Tregs convert T cells into Tregs by delivering miR-503, miR-330, and miR-9 (125). Exposure to these Treg-derived EVs results in an increased secretion of IL-10 and the expression of T lymphocyte immunoglobulin mucin 3 in naïve T cells, indicating that these EVs regulate T cell behavior. Treg-derived EVs effectively inhibited T cell proliferation in a dose-dependent manner (126). In addition to their effects on effector T cells, Tregs demonstrate their relationship with adaptive immunity by inhibiting the antigen presentation of B cells to self-reactive T cells. For example, Tregs inhibit the secretion of pro-inflammatory cytokines such as IL-6 and IFN-γ by effector B cells to regulate the differentiation of CD4+ T cells to Th1 and inhibit the activation of cytotoxic T cells through the reduction of IL-2, suppressing immune function (Figure 3) (71).
Figure 3. Chronic HBV infection leads to the differentiation of CD4+ CD25+ T cells into CD4+ CD25+ FOXP3+ regulatory T cells (Tregs). Although the function of Tregs is crucial for limiting host damage, persistent HBV infection causes Tregs to promote the conversion of effector immune cells to an immune-tolerant phenotype, creating an immunosuppressive microenvironment prone to tumors. This occurs through: (1) the production of suppressive cytokines; (2) direct killing of effector cells via perforin and granzyme; (3) direct inhibition of antigen-presenting cells through CTLA4-CD80/86 interactions; (4) consumption of IL-2 via CD25; (5) secretion of extracellular vesicles that inhibit effector immune cells; (6) direct killing of effector cells by TRAIL binding to death receptors DR4 (TRAIL-RI) and DR5 (TRAIL-RII) on their surface. HBV, Hepatitis B virus; Treg, Regulatory T cells; Teff, Effector T cells; B, B lymphocytes; NK, Natural killer cells; HCC, Hepatocellular carcinoma; IL, Interleukin; TGF, Transforming growth factor; IFN, Interferon.
In the early stages of HBV infection, NK cells play a primary role in immune defense. However, as the disease progresses to a chronic state and transforms into HCC, NK cells exhibit reduced cell frequency and decreased IFN-γ production, reflecting diminished cytotoxic activity (Figure 3) (127, 128). Enhancing the cytotoxic activity of NK cells improves the overall and relapse-free survival in HCC (129). The above studies suggest that NK cells play a central role in innate immunity. For example, monalizumab, a humanized antibody targeting the inhibitory receptor NKG2A on NK cells, reportedly enhances NK cell activity against tumor cells. This effect is further amplified when combined with immune checkpoint blockade (PD-1/PD-L1), which helps restore CD8+ T cell function. Similarly, the role of Tregs in suppressing NK cell function has been shown in animal models (130). In this model, reducing Treg frequency by administering an anti-CD25 monoclonal antibody before tumor inoculation limited tumor growth and promoted cytotoxic cell production, predominantly NK cells (131). Tregs reduced IL-2 levels in the liver cancer microenvironment through competitive uptake; however, IL-2 enhanced NK cell function and decreased lung metastasis of liver cancer in mice (132, 133). The inhibition of Tregs led to reduced TGF-β and IL-10 expression, enhancing NK cell function and increasing anti-infective and anti-tumor activities (130). Additionally, Tregs produce granzyme B and perforin, which induce NK cell apoptosis and contribute to disease progression (134). Their relationship has also been validated in other common liver diseases beyond HCC. NK cells and Tregs increase in parallel during acute episodes of liver inflammation and autoimmune liver disease (135), suggesting that inhibiting Tregs could enhance NK cell function.
The primary treatments for HBV are IFN-α and nucleoside analogs. However, these therapies have significant limitations, including their inability to completely eradicate HBV, implying that patients usually require lifelong treatment to manage infection. Additionally, the functional cure (HBV DNA suppression and loss of HBsAg levels with or without the detection of anti-HBsAg antibody) is rarely achieved after treatment with nucleoside/nucleotide analogs; however, this occurs in approximately 10% of patients who receive pegylated interferon therapy (136). Failure of the functional cure usually leads to CHB-associated HCC, particularly in patients with cirrhosis. This underscores the need for more effective therapies to prevent cancer progression. This is among the pivotal approaches for improving patient outcomes.
In patients with HBV-associated HCC, chimeric antigen receptor T-cells (CAR-T) and T-cell receptor-engineered T-cells targeting HBV antigens have shown antiviral and anti-HCC activities in vitro (137, 138). A study (139) of CAR-T therapy showed that five out of six patients with CHB-associated HCC lung metastases treated with HBV-specific CAR-T cells for 1 year experienced a reduction in lung metastases. Additionally, these cells did not adversely affect liver function over the same period. Mucosal-associated invariant T (MAIT) cells, which are naturally abundant in the liver, represent a significant subset of innate-like T cells with potent effects within the hepatic environment (140, 141). Healy et al. explored the redirection of T-cell receptors to MAIT cells in the HBV using a preclinical HCC cell model. Their findings support the potential use of MAIT cells in liver-targeted immunotherapy for HBV-associated HCC (142).
NK cell exhaustion leads to decreased cytotoxicity and impaired cytokine production (143). NK cells are significant components of the human innate immune system. The absolute numbers of circulating and intrahepatic NK cells are positively correlated with the control of HBV infection and the survival and prognosis of patients with HBV-associated HCC (144, 145). To enhance the targeting and effectiveness of NK cells against HBV and tumor cells, various genetic engineering strategies have been developed. The techniques used to create CAR-T cells have also been adapted for NK cells, improving the specificity and efficacy of NK cell therapy (146, 147).
Tregs are among the most prevalent suppressor cells in the HBV-associated tumor microenvironment. Tregs play a crucial role in suppressing effector immune cells and influencing disease prognosis in patients with hepatitis B by maintaining the immune-tolerant microenvironment established by HBV infection (109, 111, 148). There are two approaches to targeting Tregs in the liver microenvironment following HBV Infection, namely reducing their inhibitory function and decreasing Treg frequency.
The evident breakthrough in immunotherapy has been the use of immune checkpoint inhibitors (ICIs), antibodies that block key immunomodulatory molecules such as CTLA-4, as well as PD-1 and its ligand PD-L1. Notably, Tregs express many checkpoint molecules, including CTLA-4, PD-1, OX40, and LAG-3, making them direct targets for ICI immunotherapy (149). A recent meta-analysis (150) demonstrated that patients with advanced HCC treated with ICIs had a low risk of HBV infection and that ICI therapy can be safely administered to individuals with concurrent HCC and CHB. This study highlights the feasibility and significant therapeutic potential of ICI immunotherapy against HBV infection.
The stable expression of FOXP3 is associated with the demethylation of a regulatory region known as the Treg-specific demethylated region (FOXP3-TSDR), which helps to maintain the suppressive phenotype and ensures the expression of the Treg-specific gene (151). Using tools such as CRISPR/Cas9 to precisely modify the FOXP3-TSDR in Tregs enhances their suppressive function in autoimmune diseases. This could also be used as the target to reduce their immunosuppressive effects in cancer therapy (152). In addition, FOXP3 mRNA was proven to be targeted by miR-1231, miR-31, and miR-647. Targeting FOXP3 mRNA may effectively reduce the suppressive effects of Tregs, enhancing the immune function (153). Recent data showed that targeting the CD27/CD70 co-signalling axis affects Treg functions. CD27+CD70+ Tregs specifically show low levels of DNA methylation in TSDR, indicating the suppressive function of Tregs; however, CD27−CD70+ Tregs exhibit high DNA methylation levels in the TSDR region, which affects their function. Tregs with high CD27 expression tended to maintain high levels of FOXP3 expression, whereas CD70 expression was negatively correlated with FOXP3 expression levels (154). Thus, understanding and manipulating the CD27/CD70 interaction could provide novel approaches to controlling immune responses, which could be beneficial in treating various immune-related conditions.
In 2019, Zania et al. discovered a unique intracellular structure termed Enclysis, which refers to hepatocyte-specific phagocytosis of active CD4+ T cells (155). This structure regulates the number of Tregs. Enhancing Enclysis reduces Treg frequency, alleviates local immunosuppression in the liver microenvironment following HBV Infection, and restores immune cell activity. This process occurs in normal hepatocytes, hepatocellular carcinoma cells, and isolated liver samples. Therefore, understanding how Enclysis regulates the frequency of Treg cells in the liver is crucial for the treatment of chronic liver inflammation and HBV-associated HCC.
Hepatocytes selectively engulf CD4+ T cells rather than CD8+ T or B cells. This selectivity may be because CD8+ T and B cells migrate through hepatocytes paracellularly, while Tregs enter hepatocytes via vesicles, where they are acidified and degraded. The interaction between CD4+ T cells and hepatocytes can be divided into three primary stages, namely early adhesion, firm binding, and spontaneous migration. The binding of CD4+ T cells to hepatocytes relies on intercellular adhesion molecule-1, which facilitates early adhesion and the subsequent internalization of T cells. β-Catenin, a key molecule in cellular structure for epithelial cells, also plays a crucial role in the formation of Enclysis vesicles. Similarly, the formation of Enclysis vesicles shares similarities with endocytosis, as both processes rely on the actin cytoskeleton to transport vesicles from the extracellular space into the cell. The key difference is that endocytosis is a non-specific process, whereas hepatocytes exhibit a high degree of specificity for CD4+ T cells (155, 156). Therefore, Enclysis is a natural process that potentially alters Treg frequency in the liver. This is crucial for immune regulation in the liver and introduces a novel, specific mechanism for modulating Treg frequency in the liver microenvironment following HBV Infection. Although the molecular mechanisms underlying the regulation of Enclysis in the liver are partially understood, the discovery of this phenomenon may be significant for the future of immunotherapy in liver diseases.
The advent of immunotherapy has improved outcomes in patients with HCC; however, its application in HBV remains limited. Immunotherapy aims to boost the number and function of immune effector cells, such as effector T and NK cells, while inhibiting immunosuppressive cells, such as Tregs, within the liver microenvironment following HBV infection. A primary challenge is translating these promising strategies from research into clinical practice, which involves extensive clinical trials to ensure safety and efficacy. Personalized approaches to tailor the treatments based on a patient’s specific tumor profile and immune status, as well as genetic factors, are essential and pose the key challenge for treatment development. This requires the combination of advanced diagnostic tools and biomarkers to identify the most effective treatment. Similarly, immune evasion is a major challenge in immunotherapy, as HBV infection and cancer cells downregulate antigen presentation, activate immune checkpoints, and create an immunosuppressive tumor microenvironment.
The interplay between immune tolerance and effector immune cells is essential for maintaining the liver's immune microenvironment, with their regulation being crucial for sustaining immune homeostasis. In the context of HBV infection and related diseases, inhibiting immune tolerance cells or enhancing the quantity and function of effector immune cells is a significant focus in immunotherapy research. Tregs, as key mediators of the immune-tolerant microenvironment in HBV-infected livers, are intricately linked to other hepatic immune cells. The proposed approach emphasizes reducing Treg frequency while simultaneously enhancing the functionality of effector immune cells to support a comprehensive immune response. This strategy could bolster both innate and adaptive immunity against HBV infection and suppress HBV-related HCC. However, removing or depleting Tregs in the liver presents significant challenges, including risks of severe inflammation, autoimmunity, and immune overactivation. Precise targeting is essential to avoid systemic effects and compensatory mechanisms that might diminish therapy effectiveness. Balancing safety and efficacy, understanding long-term consequences, and managing potential side effects are crucial for developing effective Treg-targeted therapies in the liver.
HZ: Visualization, Writing – original draft, Writing – review & editing. BX: Visualization, Writing – review & editing. AT: Writing – review & editing. YF: Writing – review & editing. ZS: Writing – review & editing. FW: Funding acquisition, Writing – review & editing.
The author(s) declare that financial support was received for the research and/or publication of this article. This work was supported by the Central Guided Local Science and Technology Development Funds Project (2023ZY0021); The General Program of Inner Mongolia Natural Science Foundation (2024MS08009); The National Natural Science Foundation of China Regional Science Fund Project (82260481); The Science and Technology Program of Inner Mongolia Autonomous Region (2022YFSH0112); The Scientific Research Collaborative Fund of Public Hospitals in Inner Mongolia (2024GLLH0332); The National Natural Science Foundation Cultivation Program of the Affiliated Hospital of Inner Mongolia Medical University (2023NYFYPY004); The Talent Innovation and Entrepreneurship Start-up Program for Overseas Returnees. We are sincerely grateful for their generous financial support.
We want to thank our supervisor and colleagues in the department for their guidance and support during the writing of this thesis. We also would like to express our sincere gratitude to the reviewers for their valuable and constructive feedback, which significantly improved the quality of this manuscript. Their insightful comments and suggestions were greatly appreciated.
The authors declare that the research was conducted in the absence of any commercial or financial relationships that could be construed as a potential conflict of interest.
The author(s) declare that no Generative AI was used in the creation of this manuscript.
All claims expressed in this article are solely those of the authors and do not necessarily represent those of their affiliated organizations, or those of the publisher, the editors and the reviewers. Any product that may be evaluated in this article, or claim that may be made by its manufacturer, is not guaranteed or endorsed by the publisher.
HBV, Hepatitis B virus; HCC, hepatocellular carcinoma; Tregs, regulatory T cells; rcDNA, relaxed circular DNA; HBcAg, hepatitis B core antigen; HBsAg; hepatitis B surface antigen; ORFs, open reading frames; HBc, hepatitis B core protein; HBe, hepatitis B core protein; HBs, hepatitis B surface antigen; CHB, chronic hepatitis B; Tregs, T regulatory cells; cccDNA, covalently closed circular DNA; pgRNA, pregenomic RNA; PD-1, programmed cell death-1; WHV, Woodchuck Hepatitis Virus; NK, natural killer; IFN, interferon; TGF, tumor growth factor; TRAIL, tumor necrosis factor-related apoptosis-inducing ligand; KCs, Kupffer Cells; MNCs, mononuclear cells; MDSCs, myeloid-derived suppressor cells; Bregs, regulatory B cells; TNF, tumor necrosis factor; IL, interleukin; CTLA-4, cytotoxic T-lymphocyte antigen 4; Evs, extracellular vesicles; MAIT, Mucosal-associated invariant T; CAR-T, chimeric antigen receptor T-cells; ICIs, immune checkpoint inhibitors.
1. El-Serag HB. Epidemiology of viral hepatitis and hepatocellular carcinoma. Gastroenterology. (2012) 142:1264–1273.e1. doi: 10.1053/j.gastro.2011.12.061
2. World Health Organization. Global hepatitis report 2024: action for access in low- and middle-income countries (2024). Available online at: https://www.who.int/publications/i/item/9789240091672 (Accessed December 26, 2024).
3. GBD 2019 Hepatitis B Collaborators. Global, regional, and national burden of hepatitis B, 1990-2019: a systematic analysis for the Global Burden of Disease Study 2019. Lancet Gastroenterol Hepatol. (2022) 7:796–829. doi: 10.1016/S2468-1253(22)00124-8
4. Psarris A, Sindos M, Daskalakis G, Chondrogianni ME, Panayiotou S, Antsaklis P, et al. Immunizations during pregnancy: How, when and why. Eur J Obstet Gynecol Reprod Biol. (2019) 240:29–35. doi: 10.1016/j.ejogrb.2019.06.019
5. Conners EE, Panagiotakopoulos L, Hofmeister MG, Spradling PR, Hagan LM, Harris AM, et al. Screening and testing for hepatitis B virus infection: CDC recommendations - United States, 2023. MMWR Recomm Rep. (2023) 72:1–25. doi: 10.15585/mmwr.rr7201a1
6. Robinson MW, Harmon C, O'Farrelly C. Liver immunology and its role in inflammation and homeostasis. Cell Mol Immunol. (2016) 13:267–76. doi: 10.1038/cmi.2016.3
7. Del Campo JA, Gallego P, Grande L. Role of inflammatory response in liver diseases: Therapeutic strategies. World J Hepatol. (2018) 10:1–7. doi: 10.4254/wjh.v10.i1.1
8. Balkwill F, Charles KA, Mantovani A. Smoldering and polarized inflammation in the initiation and promotion of Malignant disease. Cancer Cell. (2005) 7:211–7. doi: 10.1016/j.ccr.2005.02.013
9. Llovet JM, Kelley RK, Villanueva A, Singal AG, Pikarsky E, Roayaie S, et al. Hepatocellular carcinoma. Nat Rev Dis Primers. (2021) 7:6. doi: 10.1038/s41572-020-00240-3
10. Bray F, Laversanne M, Sung H, Ferlay J, Siegel RL, Soerjomataram I, et al. Global cancer statistics 2022: GLOBOCAN estimates of incidence and mortality worldwide for 36 cancers in 185 countries. CA Cancer J Clin. (2024) 74:229–63. doi: 10.3322/caac.21834
11. Jiang Y, Han Q, Zhao H, Zhang J. The mechanisms of HBV-induced hepatocellular carcinoma. J Hepatocell Carcinoma. (2021) 8:435–50. doi: 10.2147/JHC.S307962
12. Plummer M, de Martel C, Vignat J, Ferlay J, Bray F, Franceschi S. Global burden of cancers attributable to infections in 2012: a synthetic analysis. Lancet Glob Health. (2016) 4:e609–16. doi: 10.1016/S2214-109X(16)30143-7
13. Liang TJ. Hepatitis B: the virus and disease. Hepatology. (2009) 49:S13–21. doi: 10.1002/hep.22881
14. Aspinall EJ, Hawkins G, Fraser A, Hutchinson SJ, Goldberg D. Hepatitis B prevention, diagnosis, treatment and care: a review. Occup Med (Lond). (2011) 61:531–40. doi: 10.1093/occmed/kqr136
15. Kayaaslan B, Guner R. Adverse effects of oral antiviral therapy in chronic hepatitis B. World J Hepatol. (2017) 9:227–41. doi: 10.4254/wjh.v9.i5.227
16. Terrault NA, Lok ASF, McMahon BJ, Chang KM, Hwang JP, Jonas MM, et al. Update on prevention, diagnosis, and treatment of chronic hepatitis B: AASLD 2018 hepatitis B guidance. Clin Liver Dis (Hoboken). (2018) 12:33–4. doi: 10.1002/cld.728
17. Papatheodoridis GV, Chan HL, Hansen BE, Janssen HL, Lampertico P. Risk of hepatocellular carcinoma in chronic hepatitis B: assessment and modification with current antiviral therapy. J Hepatol. (2015) 62:956–67. doi: 10.1016/j.jhep.2015.01.002
18. Zhang S, Gao S, Zhao M, Liu Y, Bu Y, Jiang Q, et al. Anti-HBV drugs suppress the growth of HBV-related hepatoma cells via down-regulation of hepatitis B virus X protein. Cancer Lett. (2017) 392:94–104. doi: 10.1016/j.canlet.2017.02.003
19. Lok AS, McMahon BJ, Brown RS Jr, Wong JB, Ahmed AT, Farah W, et al. Antiviral therapy for chronic hepatitis B viral infection in adults: A systematic review and meta-analysis. Hepatology. (2016) 63:284–306. doi: 10.1002/hep.28280
20. Fisicaro P, Barili V, Montanini B, Acerbi G, Ferracin M, Guerrieri F, et al. Targeting mitochondrial dysfunction can restore antiviral activity of exhausted HBV-specific CD8 T cells in chronic hepatitis B. Nat Med. (2017) 23:327–36. doi: 10.1038/nm.4275
21. Yang P, Li QJ, Feng Y, Zhang Y, Markowitz GJ, Ning S, et al. TGF-β-miR-34a-CCL22 signaling-induced Treg cell recruitment promotes venous metastases of HBV-positive hepatocellular carcinoma. Cancer Cell. (2012) 22:291–303. doi: 10.1016/j.ccr.2012.07.023
22. Sangro B, Sarobe P, Hervás-Stubbs S, Melero I. Advances in immunotherapy for hepatocellular carcinoma. Nat Rev Gastroenterol Hepatol. (2021) 18:525–43. doi: 10.1038/s41575-021-00438-0
23. Kumagai S, Itahashi K, Nishikawa H. Regulatory T cell-mediated immunosuppression orchestrated by cancer: towards an immuno-genomic paradigm for precision medicine. Nat Rev Clin Oncol. (2024) 21:337–53. doi: 10.1038/s41571-024-00870-6
24. Li W, Urban S. Entry of hepatitis B and hepatitis D virus into hepatocytes: Basic insights and clinical implications. J Hepatol. (2016) 64:S32–40. doi: 10.1016/j.jhep.2016.02.011
25. Hayes CN, Zhang Y, Makokha GN, Hasan MZ, Omokoko MD, Chayama K. Early events in hepatitis B virus infection: From the cell surface to the nucleus. J Gastroenterol Hepatol. (2016) 31:302–9. doi: 10.1111/jgh.13175
26. Huang HC, Chen CC, Chang WC, Tao MH, Huang C. Entry of hepatitis B virus into immortalized human primary hepatocytes by clathrin-dependent endocytosis. J Virol. (2012) 86:9443–53. doi: 10.1128/JVI.00873-12
27. Herrscher C, Roingeard P, Blanchard E. Hepatitis B virus entry into cells. Cells. (2020) 9:1486. doi: 10.3390/cells9061486
28. Yan H, Zhong G, Xu G, He W, Jing Z, Gao Z, et al. Sodium taurocholate cotransporting polypeptide is a functional receptor for human hepatitis B and D virus. Elife. (2012) 1:e00049. doi: 10.7554/eLife.00049
29. Boora S, Sharma V, Kaushik S, Bhupatiraju AV, Singh S, Kaushik S. Hepatitis B virus-induced hepatocellular carcinoma: a persistent global problem. Braz J Microbiol. (2023) 54:679–89. doi: 10.1007/s42770-023-00970-y
30. Chuang YC, Tsai KN, Ou JJ. Pathogenicity and virulence of Hepatitis B virus. Virulence. (2022) 13:258–96. doi: 10.1080/21505594.2022.2028483
31. Tu T, Budzinska MA, Shackel NA, Urban S. HBV DNA integration: molecular mechanisms and clinical implications. Viruses. (2017) 9:75. doi: 10.3390/v9040075
32. Yang W, Summers J. Integration of hepadnavirus DNA in infected liver: evidence for a linear precursor. J Virol. (1999) 73:9710–7. doi: 10.1128/JVI.73.12.9710-9717.1999
33. Yang W, Summers J. Infection of ducklings with virus particles containing linear double-stranded duck hepatitis B virus DNA: illegitimate replication and reversion. J Virol. (1998) 72:8710–7. doi: 10.1128/JVI.72.11.8710-8717.1998
34. Ou J, Rutter WJ. Hybrid hepatitis B virus-host transcripts in a human hepatoma cell. Proc Natl Acad Sci U S A. (1985) 82:83–7. doi: 10.1073/pnas.82.1.83
35. Magri A, Harris JM, D'Arienzo V, Minisini R, Jühling F, Wing PAC, et al. Inflammatory Gene Expression Associates with Hepatitis B Virus cccDNA- but Not Integrant-Derived Transcripts in HBeAg Negative Disease. Viruses. (2022) 14:1070. doi: 10.3390/v14051070
36. Rizzo GEM, Cabibbo G, Craxì A. Hepatitis B virus-associated hepatocellular carcinoma. Viruses. (2022) 14:986. doi: 10.3390/v14050986
37. Stella L, Santopaolo F, Gasbarrini A, Pompili M, Ponziani FR. Viral hepatitis and hepatocellular carcinoma: From molecular pathways to the role of clinical surveillance and antiviral treatment. World J Gastroenterol. (2022) 28:2251–81. doi: 10.3748/wjg.v28.i21.2251
38. Sivasudhan E, Blake N, Lu Z, Meng J, Rong R. Hepatitis B viral protein HBx and the molecular mechanisms modulating the hallmarks of hepatocellular carcinoma: A comprehensive review. Cells. (2022) 11:741. doi: 10.3390/cells11040741
39. Murakami S. Hepatitis B virus X protein: a multifunctional viral regulator. J Gastroenterol. (2001) 36:651–60. doi: 10.1007/s005350170027
40. Seeger C, Mason WS. Molecular biology of hepatitis B virus infection. Virology. (2015) 479-480:672–86. doi: 10.1016/j.virol.2015.02.031
41. Nassal M. HBV cccDNA: viral persistence reservoir and key obstacle for a cure of chronic hepatitis B. Gut. (2015) 64:1972–84. doi: 10.1136/gutjnl-2015-309809
42. Doherty DG, Norris S, Madrigal-Estebas L, McEntee G, Traynor O, Hegarty JE, et al. The human liver contains multiple populations of NK cells, T cells, and CD3+CD56+ natural T cells with distinct cytotoxic activities and Th1, Th2, and Th0 cytokine secretion patterns. J Immunol. (1999) 163:2314–21. doi: 10.4049/jimmunol.163.4.2314
43. Tian Z, Chen Y, Gao B. Natural killer cells in liver disease. Hepatology. (2013) 57:1654–62. doi: 10.1002/hep.26115
44. He Y, Tian Z. NK cell education via nonclassical MHC and non-MHC ligands. Cell Mol Immunol. (2017) 14:321–30. doi: 10.1038/cmi.2016.26
45. Dessouki O, Kamiya Y, Nagahama H, Tanaka M, Suzu S, Sasaki Y, et al. Chronic hepatitis C viral infection reduces NK cell frequency and suppresses cytokine secretion: Reversion by anti-viral treatment. Biochem Biophys Res Commun. (2010) 393:331–7. doi: 10.1016/j.bbrc.2010.02.008
46. Oliviero B, Varchetta S, Paudice E, Michelone G, Zaramella M, Mavilio D, et al. Natural killer cell functional dichotomy in chronic hepatitis B and chronic hepatitis C virus infections. Gastroenterology. (2009) 137:1151–60, 1160.e1-7. doi: 10.1053/j.gastro.2009.05.047
47. Rehermann B. Natural killer cells in viral hepatitis. Cell Mol Gastroenterol Hepatol. (2015) 1:578–88. doi: 10.1016/j.jcmgh.2015.09.004
48. Yang Y, Han Q, Hou Z, Zhang C, Tian Z, Zhang J. Exosomes mediate hepatitis B virus (HBV) transmission and NK-cell dysfunction. Cell Mol Immunol. (2017) 14:465–75. doi: 10.1038/cmi.2016.24
49. Zheng M, Tian Z. Liver-mediated adaptive immune tolerance. Front Immunol. (2019) 10:2525. doi: 10.3389/fimmu.2019.02525
50. Peppa D, Gill US, Reynolds G, Easom NJ, Pallett LJ, Schurich A, et al. Up-regulation of a death receptor renders antiviral T cells susceptible to NK cell-mediated deletion. J Exp Med. (2013) 210:99–114. doi: 10.1084/jem.20121172
51. Dunn C, Brunetto M, Reynolds G, Christophides T, Kennedy PT, Lampertico P, et al. Cytokines induced during chronic hepatitis B virus infection promote a pathway for NK cell-mediated liver damage. J Exp Med. (2007) 204:667–80. doi: 10.1084/jem.20061287
52. Fisicaro P, Rossi M, Vecchi A, Acerbi G, Barili V, Laccabue D, et al. The good and the bad of natural killer cells in virus control: perspective for anti-HBV therapy. Int J Mol Sci. (2019) 20:5080. doi: 10.3390/ijms20205080
53. Jenne CN, Kubes P. Immune surveillance by the liver. Nat Immunol. (2013) 14:996–1006. doi: 10.1038/ni.2691
54. Li M, Sun R, Xu L, Yin W, Chen Y, Zheng X, et al. Kupffer cells support hepatitis B virus-mediated CD8+ T cell exhaustion via hepatitis B core antigen-TLR2 interactions in mice. J Immunol. (2015) 195:3100–9. doi: 10.4049/jimmunol.1500839
55. Wang J, Zhao W, Cheng L, Guo M, Li D, Li X, et al. CD137-mediated pathogenesis from chronic hepatitis to hepatocellular carcinoma in hepatitis B virus-transgenic mice. J Immunol. (2010) 185:7654–62. doi: 10.4049/jimmunol.1000927
56. Chen S, Akbar SM, Abe M, Hiasa Y, Onji M. Immunosuppressive functions of hepatic myeloid-derived suppressor cells of normal mice and in a murine model of chronic hepatitis B virus. Clin Exp Immunol. (2011) 166:134–42. doi: 10.1111/j.1365-2249.2011.04445.x
57. Hoechst B, Ormandy LA, Ballmaier M, Lehner F, Krüger C, Manns MP, et al. A new population of myeloid-derived suppressor cells in hepatocellular carcinoma patients induces CD4(+)CD25(+)Foxp3(+) T cells. Gastroenterology. (2008) 135:234–43. doi: 10.1053/j.gastro.2008.03.020
58. Pal S, Nandi M, Dey D, Chakraborty BC, Shil A, Ghosh S, et al. Myeloid-derived suppressor cells induce regulatory T cells in chronically HBV infected patients with high levels of hepatitis B surface antigen and persist after antiviral therapy. Aliment Pharmacol Ther. (2019) 49:1346–59. doi: 10.1111/apt.15226
59. Yang G, Liu A, Xie Q, Guo TB, Wan B, Zhou B, et al. Association of CD4+CD25+Foxp3+ regulatory T cells with chronic activity and viral clearance in patients with hepatitis B. Int Immunol. (2007) 19:133–40. doi: 10.1093/intimm/dxl130
60. Fantini MC, Becker C, Monteleone G, Pallone F, Galle PR, Neurath MF. Cutting edge: TGF-beta induces a regulatory phenotype in CD4+CD25- T cells through Foxp3 induction and down-regulation of Smad7. J Immunol. (2004) 172:5149–53. doi: 10.4049/jimmunol.172.9.5149
61. Ichikawa S, Mucida D, Tyznik AJ, Kronenberg M, Cheroutre H. Hepatic stellate cells function as regulatory bystanders. J Immunol. (2011) 186:5549–55. doi: 10.4049/jimmunol.1003917
62. Rehermann B. Pathogenesis of chronic viral hepatitis: differential roles of T cells and NK cells. Nat Med. (2013) 19:859–68. doi: 10.1038/nm.3251
63. Abdel-Hakeem MS, Shoukry NH. Protective immunity against hepatitis C: many shades of gray. Front Immunol. (2014) 5:274. doi: 10.3389/fimmu.2014.00274
64. Lechner F, Wong DK, Dunbar PR, Chapman R, Chung RT, Dohrenwend P, et al. Analysis of successful immune responses in persons infected with hepatitis C virus. J Exp Med. (2000) 191:1499–512. doi: 10.1084/jem.191.9.1499
65. Rehermann B, Ferrari C, Pasquinelli C, Chisari FV. The hepatitis B virus persists for decades after patients' recovery from acute viral hepatitis despite active maintenance of a cytotoxic T-lymphocyte response. Nat Med. (1996) 2:1104–8. doi: 10.1038/nm1096-1104
66. Schmidt J, Blum HE, Thimme R. T-cell responses in hepatitis B and C virus infection: similarities and differences. Emerg Microbes Infect. (2013) 2:e15. doi: 10.1038/emi.2013.14
67. Penna A, Pilli M, Zerbini A, Orlandini A, Mezzadri S, Sacchelli L, et al. Dysfunction and functional restoration of HCV-specific CD8 responses in chronic hepatitis C virus infection. Hepatology. (2007) 45:588–601. doi: 10.1002/hep.21541
68. Bertoletti A, Ferrari C. Adaptive immunity in HBV infection. J Hepatol. (2016) 64:S71–83. doi: 10.1016/j.jhep.2016.01.026
69. Milich DR, Chen M, Schödel F, Peterson DL, Jones JE, Hughes JL. Role of B cells in antigen presentation of the hepatitis B core. Proc Natl Acad Sci U S A. (1997) 94:14648–53. doi: 10.1073/pnas.94.26.14648
70. Burton AR, Pallett LJ, McCoy LE, Suveizdyte K, Amin OE, Swadling L, et al. Circulating and intrahepatic antiviral B cells are defective in hepatitis B. J Clin Invest. (2018) 128:4588–603. doi: 10.1172/JCI121960
71. Cai Y, Yin W. The multiple functions of B cells in chronic HBV infection. Front Immunol. (2020) 11:582292. doi: 10.3389/fimmu.2020.582292
72. Sarvaria A, Madrigal JA, Saudemont A. B cell regulation in cancer and anti-tumor immunity. Cell Mol Immunol. (2017) 14:662–74. doi: 10.1038/cmi.2017.35
73. Xiao X, Lao XM, Chen MM, Liu RX, Wei Y, Ouyang FZ, et al. PD-1hi identifies a novel regulatory B-cell population in human hepatoma that promotes disease progression. Cancer Discovery. (2016) 6:546–59. doi: 10.1158/2159-8290.CD-15-1408
74. Michaud D, Steward CR, Mirlekar B, Pylayeva-Gupta Y. Regulatory B cells in cancer. Immunol Rev. (2021) 299:74–92. doi: 10.1111/imr.12939
75. Catalán D, Mansilla MA, Ferrier A, Soto L, Oleinika K, Aguillón JC, et al. Immunosuppressive mechanisms of regulatory B cells. Front Immunol. (2021) 12:611795. doi: 10.3389/fimmu.2021.611795
76. Mizoguchi A, Mizoguchi E, Takedatsu H, Blumberg RS, Bhan AK. Chronic intestinal inflammatory condition generates IL-10-producing regulatory B cell subset characterized by CD1d upregulation. Immunity. (2002) 16:219–30. doi: 10.1016/s1074-7613(02)00274-1
77. Fillatreau S, Sweenie CH, McGeachy MJ, Gray D, Anderton SM. B cells regulate autoimmunity by provision of IL-10. Nat Immunol. (2002) 3:944–50. doi: 10.1038/ni833
78. Qian L, Bian GR, Zhou Y, Wang Y, Hu J, Liu X, et al. Clinical significance of regulatory B cells in the peripheral blood of patients with oesophageal cancer. Cent Eur J Immunol. (2015) 40:263–5. doi: 10.5114/ceji.2015.52840
79. Ishigami E, Sakakibara M, Sakakibara J, Masuda T, Fujimoto H, Hayama S, et al. Coexistence of regulatory B cells and regulatory T cells in tumor-infiltrating lymphocyte aggregates is a prognostic factor in patients with breast cancer. Breast Cancer. (2019) 26:180–9. doi: 10.1007/s12282-018-0910-4
80. Lechner A, Schlößer HA, Thelen M, Wennhold K, Rothschild SI, Gilles R, et al. Tumor-associated B cells and humoral immune response in head and neck squamous cell carcinoma. Oncoimmunology. (2019) 8:1535293. doi: 10.1080/2162402X.2018.1535293
81. Mao Y, Wang Y, Dong L, Zhang Q, Wang C, Zhang Y, et al. Circulating exosomes from esophageal squamous cell carcinoma mediate the generation of B10 and PD-1high Breg cells. Cancer Sci. (2019) 110:2700–10. doi: 10.1111/cas.14122
82. Murakami Y, Saito H, Shimizu S, Kono Y, Shishido Y, Miyatani K, et al. Increased regulatory B cells are involved in immune evasion in patients with gastric cancer. Sci Rep. (2019) 9:13083. doi: 10.1038/s41598-019-49581-4
83. Schuch A, Salimi Alizei E, Heim K, Wieland D, Kiraithe MM, Kemming J, et al. Phenotypic and functional differences of HBV core-specific versus HBV polymerase-specific CD8+ T cells in chronically HBV-infected patients with low viral load. Gut. (2019) 68:905–15. doi: 10.1136/gutjnl-2018-316641
84. Benechet AP, Iannacone M. Determinants of hepatic effector CD8+ T cell dynamics. J Hepatol. (2017) 66:228–33. doi: 10.1016/j.jhep.2016.07.011
86. Boni C, Lampertico P, Talamona L, Giuberti T, Invernizzi F, Barili V, et al. Natural killer cell phenotype modulation and natural killer/T-cell interplay in nucleos(t)ide analogue-treated hepatitis e antigen-negative patients with chronic hepatitis B. Hepatology. (2015) 62:1697–709. doi: 10.1002/hep.28155
87. Kennedy PTF, Sandalova E, Jo J, Gill U, Ushiro-Lumb I, Tan AT, et al. Preserved T-cell function in children and young adults with immune-tolerant chronic hepatitis B. Gastroenterology. (2012) 143:637–45. doi: 10.1053/j.gastro.2012.06.009
88. Hao X, Chen Y, Bai L, Wei H, Sun R, Tian Z. HBsAg-specific CD8+ T cells as an indispensable trigger to induce murine hepatocellular carcinoma. Cell Mol Immunol. (2021) 18:128–37. doi: 10.1038/s41423-019-0330-1
89. Xie Y. Hepatitis B virus-associated hepatocellular carcinoma. Adv Exp Med Biol. (2017) 1018:11–21. doi: 10.1007/978-981-10-5765-6_2
90. Ringelhan M, McKeating JA, Protzer U. Viral hepatitis and liver cancer. Philos Trans R Soc Lond B Biol Sci. (2017) 372:20160274. doi: 10.1098/rstb.2016.0274
91. SCHURISchurich A, Khanna P, Lopes AR, Han KJ, Peppa D, Micco L, et al. Role of the coinhibitory receptor cytotoxic T lymphocyte antigen-4 on apoptosis-Prone CD8 T cells in persistent hepatitis B virus infection. Hepatology. (2011) 53:1494–503. doi: 10.1002/hep.24249
92. Ye B, Liu X, Li X, Kong H, Tian L, Chen Y. T-cell exhaustion in chronic hepatitis B infection: current knowledge and clinical significance. Cell Death Dis. (2015) 6:e1694. doi: 10.1038/cddis.2015.42
93. Heim K, Binder B, Sagar, Wieland D, Hensel N, Llewellyn-Lacey S, et al. TOX defines the degree of CD8+ T cell dysfunction in distinct phases of chronic HBV infection. Gut. (2020) 70:1550–60. doi: 10.1136/gutjnl-2020-322404
94. Nebbia G, Peppa D, Schurich A, Khanna P, Singh HD, Cheng Y, et al. Upregulation of the Tim-3/galectin-9 pathway of T cell exhaustion in chronic hepatitis B virus infection. PloS One. (2012) 7:e47648. doi: 10.1371/journal.pone.0047648
95. Kennedy R, Celis E. Multiple roles for CD4+ T cells in anti-tumor immune responses. Immunol Rev. (2008) 222:129–44. doi: 10.1111/j.1600-065X.2008.00616.x
96. Yan J, Liu XL, Xiao G, Li NL, Deng YN, Han LZ, et al. Prevalence and clinical relevance of T-helper cells, Th17 and Th1, in hepatitis B virus-related hepatocellular carcinoma. PloS One. (2014) 9:e96080. doi: 10.1371/journal.pone.0096080
97. Ge J, Wang K, Meng QH, Qi ZX, Meng FL, Fan YC. Implication of Th17 and Th1 cells in patients with chronic active hepatitis B. J Clin Immunol. (2010) 30:60–7. doi: 10.1007/s10875-009-9328-2
98. Fu J, Zhang Z, Zhou L, Qi Z, Xing S, Lv J, et al. Impairment of CD4+ cytotoxic T cells predicts poor survival and high recurrence rates in patients with hepatocellular carcinoma. Hepatology. (2013) 58:139–49. doi: 10.1002/hep.26054
99. Trehanpati N, Vyas AK. Immune regulation by T regulatory cells in hepatitis B virus-related inflammation and cancer. Scand J Immunol. (2017) 85:175–81. doi: 10.1111/sji.12524
100. Li C, Jiang P, Wei S, Xu X, Wang J. Regulatory T cells in tumor microenvironment: new mechanisms, potential therapeutic strategies and future prospects. Mol Cancer. (2020) 19:116. doi: 10.1186/s12943-020-01234-1
101. Suvas S, Azkur AK, Kim BS, Kumaraguru U, Rouse BT. CD4+CD25+ regulatory T cells control the severity of viral immunoinflammatory lesions. J Immunol. (2004) 172:4123–32. doi: 10.4049/jimmunol.172.7.4123
102. Suvas S, Kumaraguru U, Pack CD, Lee S, Rouse BT. CD4+CD25+ T cells regulate virus-specific primary and memory CD8+ T cell responses. J Exp Med. (2003) 198:889–901. doi: 10.1084/jem.20030171
103. Choi YS, Lee J, Lee HW, Chang DY, Sung PS, Jung MK, et al. Liver injury in acute hepatitis A is associated with decreased frequency of regulatory T cells caused by Fas-mediated apoptosis. Gut. (2015) 64:1303–13. doi: 10.1136/gutjnl-2013-306213
104. Chang KM. Regulatory T cells and the liver: a new piece of the puzzle. Hepatology. (2005) 41:700–2. doi: 10.1002/hep.20678
105. Cheng LS, Liu Y, Jiang W. Restoring homeostasis of CD4+ T cells in hepatitis-B-virus-related liver fibrosis. World J Gastroenterol. (2015) 21:10721–31. doi: 10.3748/wjg.v21.i38.10721
106. Barnaba V, Paroli M, Piconese S. The ambiguity in immunology. Front Immunol. (2012) 3:18. doi: 10.3389/fimmu.2012.00018
107. Li X, Su Y, Hua X, Xie C, Liu J, Huang Y, et al. Levels of hepatic Th17 cells and regulatory T cells upregulated by hepatic stellate cells in advanced HBV-related liver fibrosis. J Transl Med. (2017) 15:75. doi: 10.1186/s12967-017-1167-y
108. Zhang HH, Mei MH, Fei R, Liu F, Wang JH, Liao WJ, et al. Regulatory T cells in chronic hepatitis B patients affect the immunopathogenesis of hepatocellular carcinoma by suppressing the anti-tumour immune responses. J Viral Hepat. (2010) 17 Suppl 1:34–43. doi: 10.1111/j.1365-2893.2010.01269.x
109. Xu D, Fu J, Jin L, Zhang H, Zhou C, Zou Z, et al. Circulating and liver resident CD4+CD25+ regulatory T cells actively influence the antiviral immune response and disease progression in patients with hepatitis B. J Immunol. (2006) 177:739–47. doi: 10.4049/jimmunol.177.1.739
110. Stoop JN, van der Molen RG, Kuipers EJ, Kusters JG, Janssen HL. Inhibition of viral replication reduces regulatory T cells and enhances the antiviral immune response in chronic hepatitis B. Virology. (2007) 361:141–8. doi: 10.1016/j.virol.2006.11.018
111. Franzese O, Kennedy PT, Gehring AJ, Gotto J, Williams R, Maini MK, et al. Modulation of the CD8+-T-cell response by CD4+ CD25+ regulatory T cells in patients with hepatitis B virus infection. J Virol. (2005) 79:3322–8. doi: 10.1128/JVI.79.6.3322-3328.2005
112. Lin CY, Tsai MC, Huang CT, Hsu CW, Tseng SC, Tsai IF, et al. Liver injury is associated with enhanced regulatory T-cell activity in patients with chronic hepatitis B. J Viral Hepat. (2007) 14:503–11. doi: 10.1111/j.1365-2893.2006.00835.x
113. Satilmis B, Sahin TT, Cicek E, Akbulut S, Yilmaz S. Hepatocellular carcinoma tumor microenvironment and its implications in terms of anti-tumor immunity: future perspectives for new therapeutics. J Gastrointest Cancer. (2021) 52:1198–205. doi: 10.1007/s12029-021-00725-8
114. Whiteside TL. Disarming suppressor cells to improve immunotherapy. Cancer Immunol Immunother. (2012) 61:283–8. doi: 10.1007/s00262-011-1171-7
115. Fabien S, Olivier M, Khaldoun G, Vivian V, Lynda A, Laurissa O, et al. CD49b, a major marker of regulatory T-cells type 1, predicts the response to antiviral therapy of recurrent hepatitis C after liver transplantation. BioMed Res Int. (2014) 2014:290878. doi: 10.1155/2014/290878
116. Roncarolo MG, Gregori S, Battaglia M, Bacchetta R, Fleischhauer K, Levings MK. Interleukin-10-secreting type 1 regulatory T cells in rodents and humans. Immunol Rev. (2006) 212:28–50. doi: 10.1111/j.0105-2896.2006.00420.x
117. Neumann K, Rudolph C, Neumann C, Janke M, Amsen D, Scheffold A. Liver sinusoidal endothelial cells induce immunosuppressive IL-10-producing Th1 cells via the Notch pathway. Eur J Immunol. (2015) 45:2008–16. doi: 10.1002/eji.201445346
118. Collison LW, Chaturvedi V, Henderson AL, Giacomin PR, Guy C, Bankoti J, et al. IL-35-mediated induction of a potent regulatory T cell population. Nat Immunol. (2010) 11:1093–101. doi: 10.1038/ni.1952
119. Huehn J, Hamann A. Homing to suppress: address codes for Treg migration. Trends Immunol. (2005) 26:632–6. doi: 10.1016/j.it.2005.10.001
120. Kobayashi N, Hiraoka N, Yamagami W, Ojima H, Kanai Y, Kosuge T, et al. FOXP3+ regulatory T cells affect the development and progression of hepatocarcinogenesis. Clin Cancer Res. (2007) 13:902–11. doi: 10.1158/1078-0432.CCR-06-2363
121. Zhang X, Lou J, Bai L, Chen Y, Zheng S, Duan Z. Immune regulation of intrahepatic regulatory T cells in fibrotic livers of mice. Med Sci Monit. (2017) 23:1009–16. doi: 10.12659/msm.899725
122. Ormandy LA, Hillemann T, Wedemeyer H, Manns MP, Greten TF, Korangy F. Increased populations of regulatory T cells in peripheral blood of patients with hepatocellular carcinoma. Cancer Res. (2005) 65:2457–64. doi: 10.1158/0008-5472.CAN-04-3232
123. Broholm M, Mathiasen AS, Apol ÁD, Weis N. The adaptive immune response in hepatitis B virus-associated hepatocellular carcinoma is characterized by dysfunctional and exhausted HBV-specific T cells. Viruses. (2024) 16:707. doi: 10.3390/v16050707
124. Okoye IS, Coomes SM, Pelly VS, Czieso S, Papayannopoulos V, Tolmachova T, et al. MicroRNA-containing T-regulatory-cell-derived exosomes suppress pathogenic T helper 1 cells. Immunity. (2014) 41:503. doi: 10.1016/j.immuni.2014.08.008
125. Aiello S, Rocchetta F, Longaretti L, Faravelli S, Todeschini M, Cassis L, et al. Extracellular vesicles derived from T regulatory cells suppress T cell proliferation and prolong allograft survival. Sci Rep. (2017) 7:11518. doi: 10.1038/s41598-017-08617-3
126. Yu X, Huang C, Song B, Xiao Y, Fang M, Feng J, et al. CD4+CD25+ regulatory T cells-derived exosomes prolonged kidney allograft survival in a rat model. Cell Immunol. (2013) 285:62–8. doi: 10.1016/j.cellimm.2013.06.010
127. Cai L, Zhang Z, Zhou L, Wang H, Fu J, Zhang S, et al. Functional impairment in circulating and intrahepatic NK cells and relative mechanism in hepatocellular carcinoma patients. Clin Immunol. (2008) 129:428–37. doi: 10.1016/j.clim.2008.08.012
128. Wu Y, Kuang DM, Pan WD, Wan YL, Lao XM, Wang D, et al. Monocyte/macrophage-elicited natural killer cell dysfunction in hepatocellular carcinoma is mediated by CD48/2B4 interactions. Hepatology. (2013) 57:1107–16. doi: 10.1002/hep.26192
129. Nguyen T, Chen PC, Pham J, Kaur K, Raman SS, Jewett A, et al. Current and future states of natural killer cell-based immunotherapy in hepatocellular carcinoma. Crit Rev Immunol. (2024) 44:71–85. doi: 10.1615/CritRevImmunol.2024052486
130. Ghiringhelli F, Ménard C, Terme M, Flament C, Taieb J, Chaput N, et al. CD4+CD25+ regulatory T cells inhibit natural killer cell functions in a transforming growth factor-beta-dependent manner. J Exp Med. (2005) 202:1075–85. doi: 10.1084/jem.20051511
131. Ghiringhelli F, Puig PE, Roux S, Parcellier A, Schmitt E, Solary E, et al. Tumor cells convert immature myeloid dendritic cells into TGF-beta-secreting cells inducing CD4+CD25+ regulatory T cell proliferation. J Exp Med. (2005) 202:919–29. doi: 10.1084/jem.20050463
132. Chen Z, Tong L, Neo SY, Li S, Gao J, Schlisio S, et al. CD25bright NK cells display superior function and metabolic activity under regulatory T cell-mediated suppression. Oncoimmunology. (2023) 12:2175517. doi: 10.1080/2162402X.2023.2175517
133. Rai V, Abdo J, Alsuwaidan AN, Agrawal S, Sharma P, Agrawal DK. Cellular and molecular targets for the immunotherapy of hepatocellular carcinoma. Mol Cell Biochem. (2018) 437:13–36. doi: 10.1007/s11010-017-3092-z
134. Cao X, Cai SF, Fehniger TA, Song J, Collins LI, Piwnica-Worms DR, et al. Granzyme B and perforin are important for regulatory T cell-mediated suppression of tumor clearance. Immunity. (2007) 27:635–46. doi: 10.1016/j.immuni.2007.08.014
135. Jeffery HC, Braitch MK, Bagnall C, Hodson J, Jeffery LE, Wawman RE, et al. Changes in natural killer cells and exhausted memory regulatory T Cells with corticosteroid therapy in acute autoimmune hepatitis. Hepatol Commun. (2018) 2:421–36. doi: 10.1002/hep4.1163
136. Hermann A, Wennmann DO, Gromnitza S, Edeling M, Van Marck V, Sudol M, et al. WW and C2 domain-containing proteins regulate hepatic cell differentiation and tumorigenesis through the hippo signaling pathway. Hepatology. (2018) 67:1546–59. doi: 10.1002/hep.29647
137. Gehring AJ, Xue SA, Ho ZZ, Teoh D, Ruedl C, Chia A, et al. Engineering virus-specific T cells that target HBV infected hepatocytes and hepatocellular carcinoma cell lines. J Hepatol. (2011) 55:103–10. doi: 10.1016/j.jhep.2010.10.025
138. Bohne F, Chmielewski M, Ebert G, Wiegmann K, Kürschner T, Schulze A, et al. T cells redirected against hepatitis B virus surface proteins eliminate infected hepatocytes. Gastroenterology. (2008) 134:239–47. doi: 10.1053/j.gastro.2007.11.002
139. Tan AT, Yang N, Lee Krishnamoorthy T, Oei V, Chua A, Zhao X, et al. Use of expression profiles of HBV-DNA integrated into genomes of hepatocellular carcinoma cells to select T cells for immunotherapy. Gastroenterology. (2019) 156:1862–1876.e9. doi: 10.1053/j.gastro.2019.01.251
140. Jeffery HC, van Wilgenburg B, Kurioka A, Parekh K, Stirling K, Roberts S, et al. Biliary epithelium and liver B cells exposed to bacteria activate intrahepatic MAIT cells through MR1. J Hepatol. (2016) 64:1118–27. doi: 10.1016/j.jhep.2015.12.017
141. Dusseaux M, Martin E, Serriari N, Péguillet I, Premel V, Louis D, et al. Human MAIT cells are xenobiotic-resistant, tissue-targeted, CD161hi IL-17-secreting T cells. Blood. (2011) 117:1250–9. doi: 10.1182/blood-2010-08-303339
142. Healy K, Pavesi A, Parrot T, Sobkowiak MJ, Reinsbach SE, Davanian H, et al. Human MAIT cells endowed with HBV specificity are cytotoxic and migrate towards HBV-HCC while retaining antimicrobial functions. JHEP Rep. (2021) 3:100318. doi: 10.1016/j.jhepr.2021.100318
143. Sun C, Sun HY, Xiao WH, Zhang C, Tian ZG. Natural killer cell dysfunction in hepatocellular carcinoma and NK cell-based immunotherapy. Acta Pharmacol Sin. (2015) 36:1191–9. doi: 10.1038/aps.2015.41
144. Liu P, Chen L, Zhang H. Natural killer cells in liver disease and hepatocellular carcinoma and the NK cell-based immunotherapy. J Immunol Res. (2018) 2018:1206737. doi: 10.1155/2018/1206737
145. Nomura M, Tsuge M, Uchida T, Hiraga N, Kurihara M, Tsushima K, et al. CTL-associated and NK cell-associated immune responses induce different HBV DNA reduction patterns in chronic hepatitis B patients. J Viral Hepat. (2018) 25:1555–64. doi: 10.1111/jvh.12970
146. Nowakowska P, Romanski A, Miller N, Odendahl M, Bonig H, Zhang C, et al. Clinical grade manufacturing of genetically modified, CAR-expressing NK-92 cells for the treatment of ErbB2-positive Malignancies. Cancer Immunol Immunother. (2018) 67:25–38. doi: 10.1007/s00262-017-2055-2
147. Mizukoshi E, Kaneko S. Immune cell therapy for hepatocellular carcinoma. J Hematol Oncol. (2019) 12:52. doi: 10.1186/s13045-019-0742-5
148. Rushbrook SM, Ward SM, Unitt E, Vowler SL, Lucas M, Klenerman P, et al. Regulatory T cells suppress in vitro proliferation of virus-specific CD8+ T cells during persistent hepatitis C virus infection. J Virol. (2005) 79:7852–9. doi: 10.1128/JVI.79.12.7852-7859.2005
149. Alissafi T, Hatzioannou A, Legaki AI, Varveri A, Verginis P. Balancing cancer immunotherapy and immune-related adverse events: The emerging role of regulatory T cells. J Autoimmun. (2019) 104:102310. doi: 10.1016/j.jaut.2019.102310
150. Xia Z, Zhang J, Chen W, Zhou H, Du D, Zhu K, et al. Hepatitis B reactivation in cancer patients receiving immune checkpoint inhibitors: a systematic review and meta-analysis. Infect Dis Poverty. (2023) 12:87. doi: 10.1186/s40249-023-01128-6
151. Ohkura N, Hamaguchi M, Morikawa H, Sugimura K, Tanaka A, Ito Y, et al. T cell receptor stimulation-induced epigenetic changes and Foxp3 expression are independent and complementary events required for Treg cell development. Immunity. (2012) 37:785–99. doi: 10.1016/j.immuni.2012.09.010
152. Chen X, Zhong S, Zhan Y, Zhang X. CRISPR-Cas9 applications in T cells and adoptive T cell therapies. Cell Mol Biol Lett. (2024) 29:52. doi: 10.1186/s11658-024-00561-1
153. Singer M, Elsayed AM, Husseiny MI. Regulatory T-cells: the face-off of the immune balance. Front Biosci (Landmark Ed). (2024) 29:377. doi: 10.31083/j.fbl2911377
154. Arroyo Hornero R, Georgiadis C, Hua P, Trzupek D, He LZ, Qasim W, et al. CD70 expression determines the therapeutic efficacy of expanded human regulatory T cells. Commun Biol. (2020) 3:375. doi: 10.1038/s42003-020-1097-8
155. Davies SP, Reynolds GM, Wilkinson AL, Li X, Rose R, Leekha M, et al. Hepatocytes delete regulatory T cells by enclysis, a CD4+ T cell engulfment process. Cell Rep. (2019) 29:1610–1620.e4. doi: 10.1016/j.celrep.2019.09.068
Keywords: hepatitis B virus, hepatocellular carcinoma, immune cells, regulatory T cells, HBV infection
Citation: Zheng H, Xu B, Fan Y, Tuekprakhon A, Stamataki Z and Wang F (2025) The role of immune regulation in HBV infection and hepatocellular carcinogenesis. Front. Immunol. 16:1506526. doi: 10.3389/fimmu.2025.1506526
Received: 05 October 2024; Accepted: 19 February 2025;
Published: 14 March 2025.
Edited by:
Jorge Quarleri, National Scientific and Technical Research Council (CONICET), ArgentinaReviewed by:
Theodoros Androutsakos, National and Kapodistrian University of Athens, GreeceCopyright © 2025 Zheng, Xu, Fan, Tuekprakhon, Stamataki and Wang. This is an open-access article distributed under the terms of the Creative Commons Attribution License (CC BY). The use, distribution or reproduction in other forums is permitted, provided the original author(s) and the copyright owner(s) are credited and that the original publication in this journal is cited, in accordance with accepted academic practice. No use, distribution or reproduction is permitted which does not comply with these terms.
*Correspondence: Fei Wang, d2FuZ2ZlaV9hbGxlbkBpbW11LmVkdS5jbg==
†Present address: Hailong Zheng, Department of General Surgery, People's Hospital of Wulateqian Banner, Bayannur, Inner Mongolia Autonomous Region, China
‡These authors share senior authorship
Disclaimer: All claims expressed in this article are solely those of the authors and do not necessarily represent those of their affiliated organizations, or those of the publisher, the editors and the reviewers. Any product that may be evaluated in this article or claim that may be made by its manufacturer is not guaranteed or endorsed by the publisher.
Research integrity at Frontiers
Learn more about the work of our research integrity team to safeguard the quality of each article we publish.