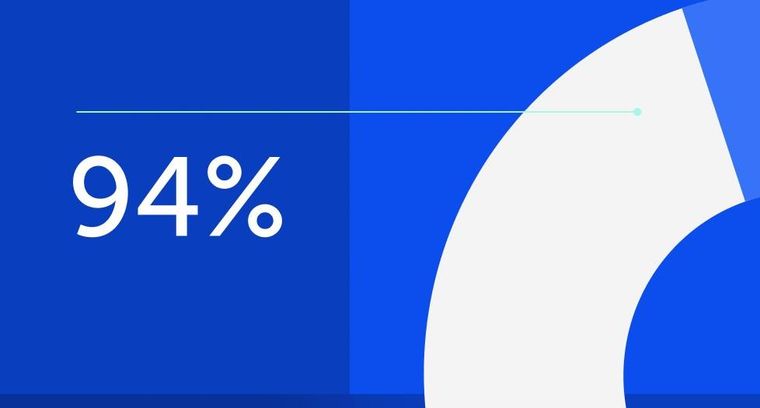
94% of researchers rate our articles as excellent or good
Learn more about the work of our research integrity team to safeguard the quality of each article we publish.
Find out more
REVIEW article
Front. Immunol., 15 April 2025
Sec. Viral Immunology
Volume 16 - 2025 | https://doi.org/10.3389/fimmu.2025.1473475
This article is part of the Research TopicA New Perspective in Immune Polymorphism (The HLA, KIR, and LILR genes)View all 10 articles
Dengue, a viral infection transmitted by Aedes mosquitoes, is an emerging global health threat exacerbated by climate change. Rising temperatures and altered precipitation patterns create favourable conditions for vector proliferation and extended transmission periods, increasing the risk of dengue in endemic regions and facilitating its spread to non-endemic areas. Understanding the interplay between critical genetic factors and dengue susceptibility is crucial for developing effective public health strategies. The Human Leukocyte Antigen (HLA) genes encode proteins essential for an effective immune response against pathogens, and their genetic variations influence susceptibility to severe dengue. In this study, we conducted a comprehensive meta-analysis of HLA alleles associated with dengue infection and dengue severity. We analysed 19 case-control studies on dengue infections in populations worldwide to infer HLA associations with various pathological forms of dengue and to examine differences across different populations. Our findings indicate that HLA-A*02 increases susceptibility to dengue fever (DF), while HLA-A*03 increases the risk of Dengue Haemorrhagic Fever (DHF), with these increased susceptibilities primarily observed in Southeast Asian populations. Additionally, HLA-A*24 is associated with DHF and all symptomatic dengue infections (DEN), contributing to dengue risk in both Southeast Asia and the Caribbean. Conversely, HLA-A*33 and HLA-B*44 show a protective effect against DHF but show significant regional heterogeneity, highlighting divergent, population-specific susceptibility profiles. This study underscores the importance of population-specific genetic risk assessments for dengue infection and emphasizes the need for targeted medical interventions and improved predictive models to mitigate dengue’s impact, especially as climate change accelerates disease spread.
Dengue is a viral infection emerging as a significant threat to global health, caused by the dengue virus (DENV). DENV is primarily transmitted through a human-to-mosquito-to-human cycle by specific mosquito vectors known as Aedes aegypti and Aedes albopictus. In the year 2023, the number of reported dengue cases reached a historical high of 6.5 million (1); as of 30 April 2024, the total number of cases had reached a staggering 7.6 million (2) for the year 2024. It is estimated that 400 million yearly infections occur, and currently, half of the world’s population is at the risk of contracting dengue (1, 3). Previously endemic to the tropics and subtropics of Asia, the Americas, the Western Pacific, and Africa; it has now been reported to be rapidly expanding into previously non-endemic regions, such as temperate and even high-altitude regions due to climate change (4, 5). This growing burden of dengue infections highlights the importance of a more comprehensive understanding of the virus, its role in disease progression, and its interaction with the human immune system.
Dengue is an acute febrile illness caused by four types of single-stranded DENVs from the Flavivirus genus that elicit distinct serological responses with the antibodies in human blood (6). The four DENV serotypes share about 65% of their genome, with subvariants within each serotype (7). The DENV genome comprises 10 genes coding for 3 structural and 7 non-structural proteins. The three structural proteins are capsid (C), membrane (M), and envelope (E), while the seven non-structural proteins are NS1, NS2A, NSB, NS3, NS4A, NS4B and NS5 (8, 9). The C protein forms the nucleocapsid of the virus, and the M protein plays a crucial role in viral maturation (10, 11). The E protein is essential for viral entry into the host cell by receptor binding and subsequent fusion (11). The non-structural proteins NS1, NS2A, NS4A, and NS4B are involved in the RNA replication process, with NS1 and NS5 also serving as antigens to initiate immune responses in the host cell (9, 12–14). The NS3 protein has helicase and protease functions, while NS4A is involved in autophagy (9, 14).
The replication cycle of the DENV begins with viral binding to the host cell receptors through clathrin-dependent receptor-mediated endocytosis (15). Inside the host cells, the virus undergoes endosomal processing facilitated by Rab5 and Rab7 proteins. Subsequently, the viral genome is released into the cytoplasm and translated into the endoplasmic reticulum (ER). The newly synthesised components are assembled into immature viral particles in the ER undergo maturation in the Trans-Golgi Network via furin-mediated cleavage and are eventually released from the cell through exocytosis (16).
Four serotypes of DENV have been reported to date (DENV1-4), and each serotype can cause dengue fever (DF), dengue haemorrhagic fever (DHF) (Grades 1-2), and dengue shock syndrome (DSS) (DHF Grades 3 and 4). DENV1-4 are the primary dengue-causing serotypes circulating in humans, with different regions reporting varying profiles of circulating serotypes (Figure 1). Common symptoms of DHF include high fever, muscle and joint pain, severe headache, vomiting, bleeding from gums, and skin rashes. Without immediate medical treatment, DHF (Grades 1-2) might develop into DSS, the severe form of DHF, characterized by shortness of breath, rapid pulse rate, low blood pressure and severe abdominal pain. 60-80% of individuals with a primary DENV infection are asymptomatic; however, the risk of severe disease increases significantly during a second infection, especially among those with longer intervals since the primary DENV infection (17). DENV-2 and DENV-4 are more commonly identified in secondary dengue infections, while DENV-1 and DENV-3 often cause primary infections (7, 18).
Figure 1. Worldwide pattern of dengue endemicity according to WHO and CDC, and the prevalence of dengue serotypes across different regions.
There is no consensus on the pathogenesis of DENV infection in the scientific community. The three major factors related to the severity of DENV infection include secondary infection, host genetics, and viral virulence (19–23), with the majority view that secondary infection is the main risk factor for DHF. Primary DENV infection involves the stimulation of interferon gamma (IFNγ) (24). During a secondary infection, non-neutralizing antibodies (25, 26) from the primary infection can bind to the serotype of the second infection. Instead of neutralizing the virus, these antibodies facilitate its entry into host cells, leading to increased viral replication and a more severe immune response. The phenomenon is commonly known as Antibody-Dependent Enhancement (ADE) (27, 28). This heightened immune response can cause increased vascular permeability, plasma leakage, and other severe symptoms characteristic of DHF. The detection of DENV by RIG-I (retinoic acid-inducible gene I) and MDA5 (melanoma differentiation-associated protein 5) triggers the phosphorylation of IRF3 (interferon regulatory factors 3) and IRF7 (interferon regulatory factors 7), leading to the production of type I and III interferons (IFNs), the activation of the JAK-STAT pathways, and the upregulation of interferon-stimulated genes (ISGs) (29). During severe infection, immature DENV particles are recognized by TLR2 (Toll-like Receptor 2) and DC-SIGN (Dendritic Cell-Specific Intercellular adhesion molecule-3-Grabbing Non-integrin) on monocytes and immature dendritic cells, resulting in the release of inflammatory mediators like IL-1β (interleukin 1β) and TNF-α (tumour necrosis factor α), which increase endothelial cell permeability or dysfunction, contributing towards severe complications (30, 31). Moreover, excess secretion of anti-DENV antibodies can exacerbate ADE (Figure 2), further increasing autoantibody production and its potential glycosylation, a signature seen in severe DHF and DSS (32, 33).
Figure 2. Adaptive immunity mechanisms which could determine the protective and predisposing outcomes against dengue.
Endothelial dysfunction could lead to complications such as hypotension, ascites, pleural effusions, shock, and organ dysfunction (34). NS1 protein and inflammatory mediators from monocytes, macrophages, dendritic cells, and mast cells contribute to this dysfunction (34, 35). Elevated cytokines and chemokines, such as IL-1β, IL-6, TNF-α, and MMP-9, directly cause endothelial dysfunction, while others like IL-10 may reduce it (36). Lipid mediators, including PAF, leukotrienes, prostaglandins, and sPLA2 enzymes, also play a role in vascular permeability (36). PAF and prostaglandin metabolites are elevated in severe cases and cause endothelial dysfunction (34, 36, 37). Mast cell products such as histamine, tryptase, chymase, VEGF, and serotonin are linked to disease severity and vascular leakage (38–40). Recent studies from Nicaragua and India showed that both primary and secondary DENV infections can range from subclinical to severe implications (41, 42).
DSS also involves severe cases of hepatomegaly and bleeding, especially in gum, nose, and stool. A typical signature of DSS, hepatomegaly and other complications regarding the liver, high levels of aspartate transaminase (AST) levels are inferred to have played an influential role (43). Secondary hemophagocytic lymphohistiocytosis (HLH), characterized by macrophage activation and cytokine storms, is a significant cause of severe liver dysfunction and high mortality in dengue patients (44, 45). Dengue can cause various bleeding manifestations, including petechiae, gum bleeding, and hematemesis (36). Bleeding results from thrombocytopenia, dysfunctional platelets, coagulation pathway abnormalities, and prolonged shock (36, 46). Over 50% of hospitalized dengue patients experience severe thrombocytopenia, which correlates with disease severity (36). Platelets can be directly infected by DENV, leading to activation through multiple pathways, including serotonin release from mast cells and direct activation by the virus (40, 47).
Since DENV is a foreign body infecting host cells, the genes influencing host defence mechanisms against such foreign infecting agents could play a crucial role in influencing effective response against DENV. One such large complex of genes are the HLA genes. HLA genes are known to be the most polymorphic regions in the human genome and are the part of Major Histocompatibility Complex (MHC) region located on the short arm of chromosome 6. Class I and class II HLA genes encode antigen-recognising sites crucial for adaptive immune responses, recognising between self and non-self. HLA class I proteins present foreign peptides to T-cell receptors on CD8+ T cells (cytotoxic T cells) to launch immune responses. HLA class II proteins are expressed on active immune cells like antigen-presenting cells (APCs) and B cells, where they detect antigens and present them to CD4+ T cells (helper T cells) to initiate immune responses. During Antibody-Dependent Enhancement (ADE) where Fcγ receptors (FcγR) facilitate the increased entry of DENV into immune cells, it could lead to the upregulation of HLA class I molecules, suppressing the activity of NK cells (48). Such reduced activity could potentially contribute to disease pathogenesis. HLA class I alleles further influence the magnitude of CD8+ T cell responses against DENV (49–51). Therefore, the host’s HLA allele profile is paramount in determining the immunogenic response to DENV infection. This leads to varied immunopathological outcomes, including susceptibility or protection against DENV infection or more severe outcomes (Figure 2) (52, 53).
Given the crucial role of HLA genes in determining the pathophysiology of DENV infection, numerous case-control studies have investigated how HLA polymorphisms influence susceptibility to and protection against severe outcomes of DENV infection. By late 1970s, the idea that HLA proteins could determine the susceptibility towards viral infections started gaining ground (54). HLA responses against DENV can also show serotype specificity. A study on the Sri Lankan population has demonstrated that DENV-2-specific responses, likely due to the population’s previous history of DENV-2 infection, signifying that epidemiological history could influence serotype-specific immune responses (51). However, HLA allele associations with DENV infection outcomes could be complicated by factors such as HLA alleles typing resolution, the population studied, predominant DENV serotypes, HLA allele composition, and how the different stages/categories of dengue are defined in the study design. Therefore, usage of standardised pathophysiological classifications for dengue, clear definition of populations studied, and large enough case & control sizes are imperative to derive statistically significant inferences.
The earliest case-control study suggesting the influence of HLA alleles in determining susceptibility or protection against DENV infection was performed on Thai children in 1981, identifying several HLA class I antigens as potential crucial regulators in the development of severe forms of dengue (55). Throughout the years, several case-control studies, primarily across Southeast Asia, Central and South America, have further cemented the crucial role of different HLA class I alleles in determining dengue susceptibility (55–73).
Dengue cases have been rising exponentially over the past five decades with the Americas (Caribbean and Latin American), Southeast Asia, and South Asia, bearing the highest burden of dengue cases (Figure 3A). From 2022 to 2023, dengue cases more than doubled in 16 Caribbean and 9 Latin American countries, with St Kitts and Nevis and Argentina reporting increases of 28500% and 17364.4%, respectively. Similarly, cases in Bangladesh in South Asia and Thailand in Southeast Asia showed increases of 414.6% and 241.1% (Figure 3B), respectively. In Europe, the locally transmitted cases increased significantly in 2023, with Spain, Italy, and France reporting 3, 82, and 45 cases, respectively (2).
Figure 3. Worldwide dengue case patterns (A) Total reported cases for the year 2023 (B) Percentage increase in dengue cases from the year 2022-202.
The global increase in dengue cases can be attributed to several factors, including viral evolution (74, 75), human settlement patterns (76), socioeconomic drivers (77), human mobility (78), age (79) and climate change (80). A complex interplay of these factors determines the epidemiological fate of dengue for any given population or geographic setting. Specifically, DENV vectors belonging to Aedes sp have rapidly expanded into new habitats, driven by warmer and more humid conditions due to climate change (81–85). Such climatic conditions could result in a shorter extrinsic incubation period (EIP), the period required for a mosquito to become dengue-transmittable after having an infectious meal, increasing dengue risk (86). Furthermore, rising temperatures have been found to increase the biting incidence of dengue vectors, while extended periods of warmer conditions lengthen the suitable period for dengue transmission, heightening the risk of severe epidemics (87).
It is projected that within the next six decades, close to two-thirds of the world’s population will be vulnerable to dengue infection (82). Without adequate preparations, the potential surge of dengue cases could overwhelm medical infrastructures, particularly considering emerging infectious diseases like COVID-19. This growing threat underscores the importance of understanding genetic factors that influence dengue susceptibility and severity.
In the context of the rising dengue cases due to climate change and other anthropogenic factors, we review and perform a meta-analysis of the known associations of HLA alleles with dengue. As key regulators of adaptive immunity, HLA molecules present viral peptides to T cells, influencing immune activation and viral clearance. Variability in HLA alleles has been linked to differences in dengue susceptibility, severity, and immune response efficiency (88–90). Understanding these associations is crucial to gaining deeper insights into how our adaptive immune system functions and influences DENV infection outcomes.
Global DENV infection cases and endemicity data were compiled and collected from WHO’s 2024 reports (1, 2) and ECDC (91), latest until 24 June 2024. Missing data from DENV infection cases was collected from the official reported figures mentioned governmental health ministry and infectious disease surveillance website for each respective country (92). We had a total of 75 countries reporting endemic dengue cases until the start of 2024.
To look for candidate papers for HLA-Dengue association meta-analysis, we searched two databases, PUBMED and Semantic Scholar. In order to make the best use of the two datasets, custom search strategies were used for each, mentioned below:
● PUBMED.
● We entered the following query in the search option to optimise the retrieval of appropriate studies – (Dengue) AND (HLA) AND ((association) OR (associated)). The submitted query returned 101 potential study candidates.
● Semantic Scholar.
● We utilised the elicit (93) tool to streamline the searching process from Semantic Scholar. For a query of “What are the HLA alleles associated with Dengue infection outcomes in humans?”, it was ensured that the words “Dengue”, “HLA”, “association” or “associated” will should be present within the abstract. This search strategy returned a total of 264 study candidates from an initial 32,604 when searched directly on Semantic Scholar.
With the studies obtained from the above search strategies, we followed the Preferred Reporting Items for Systematic Reviews and Meta-Analyses (PRISMA) guidelines (94) to screen and finalise studies which would be included in the meta-analysis (Figure 4). During the selection of the studies to be included for the meta-analyses, the investigators decided on the final set of candidates without any conflicting viewpoints or opinions.
A total of 19 studies have been included in the meta-analysis (Table 1), DENV infection phenotypes such as DF, DHF and DSS were comprehensively extracted. Symptomatic DENV infection cases defined as DEN and the sum of DF and DHF was considered as DEN in our meta-analysis. Cochrane RevMan Web (99) was used for calculation of effect sizes of the studies, confidence intervals (CIs), heterogeneity across studies (I2 statistics) and generation of forest plots. Fixed-effects model was used to calculate the pooled effect sizes and corresponding 95% CIs. Statistical significance was set at Mantel-Haenszel P < 0.05. Forest plots were plotted based on dengue phenotypes (DF, DHF and DEN) and further stratified by populations.
Table 1. List of previously reported case-control HLA association study with respect to dengue included in the meta-analysis.
Following the PRISMA guideline (94) (Figure 4), a total 19 case-control studies were included in our meta-analysis (Table 1). After selecting the candidate studies, we performed a meta-analysis of the most commonly associated alleles across dengue fever (DF), dengue haemorrhagic fever (DHF) or all symptomatic dengue outcomes taken together (DEN). We also conducted a meta-analysis based on the origin of populations to observe susceptibility tendencies at a population-specific scale for each HLA allele against DEN.
The HLA-A*02 allele shows a significant amount of heterogeneity (P = 1.0E-03) across the five tested studies when DF is compared with healthy controls. Despite such heterogeneity, HLA-A*02 [P = 2.0E-03, OR = 1.37 (1.13–1.67)] is inferred to have a predisposing effect towards DF development (Figure 5).
Figure 5. Meta-analysis of HLA-A*02 for dengue fever (DF), dengue haemorrhagic fever (DHF) and DEN (CI, Confidence interval; M-H, Mantel-Haenszel fixed effect test for Odds Ratio).
When comparing DHF with healthy controls, the data from seven selected studies also show significant heterogeneity [P = 1.0E-02]. The combined odds ratio for HLA-A*02 for DHF against healthy controls is insignificant [P = 0.07, OR = 1.18 (0.99–1.40)], with a tendency towards being more predisposing to DHF (Figure 5).
When comparing DEN with healthy controls based on 13 studies, the combined odds ratio [P = 2.0E-02, OR = 1.16 (1.02–1.32)] suggests a predisposing influence of HLA-A*02 despite the significant heterogeneity in the observation [P = 1.0E-04] (Figure 5).
To infer the regional tendencies of the HLA-A*02 allele influencing the susceptibility to any forms of DEN, we calculated the odds ratio after grouping the studies based on their region of origin (Figure 6). Based on a single study conducted in Jamaica (72), the allele showed a tendency towards being a risk allele with the Caribbean region with the statistics remaining insignificant [P = 0.06, OR = 2.02 (0.97–4.22)]. Meanwhile, in Latin America, no significant trend was observed [P = 0.93, OR = 1.01 (0.81–1.25)].
Figure 6. Meta-analysis of HLA-A*02 across different populations for DEN (CI, Confidence interval; M-H, Mantel-Haenszel fixed effect test for Odds Ratio).
In South Asia, the two studies based on Sri Lankan (71) and Indian (61) cohorts reported an insignificant association with dengue with respect to HLA-A*02. The combined odds ratio remained insignificant [P = 0.19, OR = 0.78 (0.53–1.14)], with a tendency towards being protective (Figure 6). In Southeast Asia, despite significant heterogeneity across studies [P = 2.0E-03] driven primarily by the Vietnamese study done in 2001 (66) and the study on Filipino children in 2015 (56), a clear trend of risk emerged. Based on the six selected studies across Thailand, Vietnam, Malaysia, and Philippines, HLA-A*02 is inferred to be a statistically significant risk allele for DEN [P = 2.0E-03, OR = 1.31 (1.10–1.57)] (Figure 6).
When combined across different regions and accounting for significant heterogeneity (P = 8.0E-04), the risk predisposition characteristic of HLA-A*02 stands [P = 4.0E-02, OR = 1.14 (1.01–1.30)] (Figure 6). It is very likely that this inference is driven primarily by the Southeast Asian studies, given their higher weight in the analysis conducted. Moreover, regardless of significant associations with either being a risk or protective against DEN within each region, the tendency of odds ratio differs significantly between each regional group (P = 2.0E-02). This difference among different region groups suggests a population-specific variability of the susceptibility profile of HLA-A*02.
HLA-A*03 do not show any significant effect on DF susceptibility when compared to healthy controls across four studies [P = 0.72, OR = 0.95 (0.70–1.28)] (Figure 7). When DHF is compared with healthy samples, significant heterogeneity between the four included studies was observed (P = 1.0E-03). Despite this heterogeneity, the combined odds ratio [P = 1.0E-04, OR = 1.83 (1.34–2.50)] suggests a predisposing effect of HLA-A*03 towards DHF, primarily driven by the Thai study conducted in 2005 (64).
Figure 7. Meta-analysis of HLA-A*03 for dengue fever (DF), dengue haemorrhagic fever (DHF) and DEN (CI, Confidence interval; M-H, Mantel-Haenszel fixed effect test for Odds Ratio).
When DEN was compared with healthy controls, no significant associations towards risk or protection were observed [P = 0.81, OR = 1.03 (0.82–1.28)]. Overall, HLA-A*03 shows a statistically insignificant [P = 0.07, OR = 1.15 (0.99–1.34)] tendency to be a risk allele. Within the three tested outcomes, DF, DHF, and DEN, a significant difference between each group was observed [P = 4.0E-03] (Figure 7).
Within the Caribbean [P = 0.07, OR = 2.22 (0.95–5.23)] and Latin American [P = 0.21, OR = 1.12 (0.81–1.56)] populations, statistically insignificant influence of HLA-A*03 on DEN susceptibility was observed (Figure 8). In South Asia [P = 0.80, OR = 0.92 (0.50–1.71)] and Southeast Asia [P = 0.34, OR = 0.84 (0.58–1.21)], no significant influence of HLA-A*03 on DEN was inferred (Figure 8). Overall, when all regions are compared, HLA-A*03, does not show any significant effect on DEN susceptibility [P = 0.81, OR = 1.03 (0.82–1.28)].
Figure 8. Meta-analysis of HLA-A*03 across different populations for DEN (CI, Confidence interval; M-H, Mantel-Haenszel fixed effect test for Odds Ratio).
HLA-A*24 (Figure 9) shows no statistically significant protective or predisposing effect for DF [P = 0.85, OR = 1.04 (0.83–1.30)]. When we compare DHF with healthy controls across eight studies, the significant combined odds ratio [P = 3.0E-04, OR = 1.38 (1.16–1.64)] suggests a predisposing influence of HLA-A*24 towards DHF.
Figure 9. Meta-analysis of HLA-A*24 for dengue fever (DF), dengue haemorrhagic fever (DHF) and DEN (CI = Confidence interval; M-H = Mantel-Haenszel fixed effect test for Odds Ratio).
When we compare DEN with the healthy control across 12 studies (Figure 9), the predisposing influence of HLA-A*24 [P < 1.0E-05, OR = 1.40 (1.23–1.60)] stands despite significant heterogeneity [P = 1.0E-03] across different studies.
In terms of combined influence of HLA-A*24 across the three different subtypes of dengue infection, the statistics [P < 1.0E-05, OR = 1.32 (1.20–1.45)] (Figure 9) suggest an overall predisposing influence towards dengue.
In terms of population specific tendencies (Figure 10), HLA-A*24 shows significant predisposition towards DEN in the Caribbean population [P = 1.0E-03, OR = 14.29 (2.90–70.35)]. It is noted that the wide confidence interval for the study is due to its small sample size and should be interpreted with caution. In the three Latin American studies (59, 68, 69), HLA-A*24 does not show statistically significant associations with being a risk or a protective factor for DEN [P = 0.57, OR = 1.10 (0.78–1.55)]. Having said that, the tendency of the allele could be considered towards being predisposed to DEN.
Figure 10. Meta-analysis of HLA-A*24 across different populations for DEN (CI, Confidence interval; M-H, Mantel-Haenszel fixed effect test for Odds Ratio).
Among South Asians, HLA-A*24 shows a similar trend to that of Latin Americans, with no statistically significant associations towards being a risk or protective factor for DEN [P = 0.33, OR = 1.19 (0.84–1.69)], while having a tendency towards being a risk allele for DEN (Figure 10). However, in Southeast Asia, HLA-A*24 is inferred to be a risk allele for DEN based on the combined odds ratio [P = 3.0E-04, OR = 1.33 (1.14–1.56)] (Figure 10).
When all the regional populations are considered together, HLA-A*24 is inferred to be a predisposing allele for DEN [P < 1.0E-05, OR = 1.30 (1.14–1.49)] (Figure 10). This inference is significantly driven by the outcomes from the studies on Southeast Asian populations, which contributed to a higher number of sample counts.
For HLA-A*33 (Figure 11), when comparing DF with healthy controls based on four studies, no significant influence of HLA-A*33 on DF was inferred [P = 0.71, OR = 0.94 (0.68–1.30)]. Whereas, when we compared DHF with healthy controls, HLA-A*33 shows significant protective influence against DHF [P = 6.0E-04, OR = 0.63 (0.48–0.82)], despite a significant degree of heterogeneity [P = 5.0E-02].
Figure 11. Meta-analysis of HLA-A*33 for dengue fever (DF), dengue haemorrhagic fever (DHF) and DEN (CI, Confidence interval; M-H, Mantel-Haenszel fixed effect test for Odds Ratio).
Despite significant heterogeneity observed across studies [P = 3.0E-04], HLA-A*33 was found to confer a protective effect against DEN [P = 3.0E-02, OR = 0.80 (0.66–0.98)] (Figure 11). When observed across all the three different tested scenarios for dengue (DF, DHF, DEN), HLA-A*33 was inferred to have a protective effect [P = 4.0E-04, OR = 0.77 (0.67–0.89)] (Figure 11). Such an inference could have been driven by HLA-A*33’s stronger protective influence against DHF.
When compared across worldwide populations (Figure 12), HLA-A*33 is observed to be a risk factor for DEN in the Caribbean population [P = 3.0E-03, OR = 4.51 (1.65–12.33)], a trend completely disparate from the general trend observed for HLA-A*33 in the previous set of inference. Furthermore, the small sample size could have played a significant role in the wide confidence interval observed for the Caribbeans. Interestingly, in Latin America, a study on the Brazilian population (68) showed a similar predisposing tendency for HLA-A*33 as the Caribbeans (72), whereas another study on Cubans (69) demonstrated a protective tendency. Overall, HLA-A*33 does not demonstrate any significant protective or predisposing effect with respect to DEN to the Caribbeans [P = 0.76, OR = 0.92 (0.52–1.61)].
Figure 12. Meta-analysis of HLA-A*33 across different populations for DEN (CI, Confidence interval; M-H, Mantel-Haenszel fixed effect test for Odds Ratio).
Similar to Latin America, HLA-A*33 does not show an association with a protective or predisposing effect towards DEN in South Asians [P = 0.53, OR = 1.13 (0.77–1.65)] (Figure 12). In contrast, in Southeast Asia, HLA-A*33 is inferred to confer protection against DEN [P < 1.0E-4, OR = 0.57 (0.43–0.75)] (Figure 12). When examined individually across the four Southeast Asian studies included in the analysis, the protective trend remains consistent throughout (56, 66, 67, 97).
Considering the four regional population groups together, HLA-A*33 is inferred to be a protective allele against DEN [P = 3.0E-02, OR = 0.80 (0.66–0.98)] (Figure 12). This inference is primarily driven by the associations inferred from the analysis of the Southeast Asian populations, having a higher sample size. The statistically significant difference in the combined odds ratio across the different regions further highlights the population-specific difference in the influence of HLA-A*33 on DEN.
HLA-B*44 (Figure 13) showed no significant protective or predisposing association with DF when compared to healthy controls [P = 0.28, OR = 0.88 (0.70–1.11)]. Nonetheless, the observed tendency was towards being protective against DF. When we compare DHF with healthy controls, HLA-B*44 was found to have a protective effect against DHF [P = 1.0E-03, OR = 0.60 (0.44–0.82)]. However, this observation does not stand true when comparing DEN with healthy controls [P = 0.77, OR = 0.97 (0.81–1.17)], as no significant protective or predisposing effect is observed.
Figure 13. Meta-analysis of HLA-B*44 for dengue fever (DF), dengue haemorrhagic fever (DHF) and DEN (CI, Confidence interval; M-H, Mantel-Haenszel fixed effect test for Odds Ratio).
The overall tendency of HLA-B*44 allele suggests a protective effect against dengue [P = 2.0E-02, OR = 0.86 (0.75–0.98)] (Figure 13). The significant difference between each subgroup highlights HLA-B*44’s increased protective influence on DHF likely have influenced the overall role for the allele with respect to dengue.
Comparing regional population tendencies (Figure 14), in the Caribbean, no protective or predisposing influence of HLA-B*44 was observed [P = 0.38, OR = 1.56 (0.58–4.22)], although the observed trend was towards being predisposing. In Latin America, despite showing significant heterogeneity [P = 3.0E-02], HLA-B*44 is inferred to have predisposing influence towards DEN [P = 4.0E-02, OR = 1.37 (1.02–1.85)].
Figure 14. Meta-analysis of HLA-B*44 across different populations for DEN (CI, Confidence interval; M-H, Mantel-Haenszel fixed effect test for Odds Ratio).
In South Asia, HLA-B*44 shows a tendency towards being predisposing; however, no significant association was observed [P = 0.75, OR = 1.07 (0.69–1.67)] (Figure 14). In contrast, from the two Thai-based study in Southeast Asia, we can infer a protective effect of HLA-B*44 against DEN [P = 4.0E-03, OR = 0.65 (0.49–0.87] (Figure 14).
HLA-B*44 shows no significant association with DEN when all four regions are taken together (Figure 14). However, significant heterozygosity [P = 4.0E-03] is observed across each region, highlighting the population-specific influence of HLA-B*44 towards DEN.
Given the observed historical trends, dengue could continue its rapid expansion across the globe, potentially increasing the number of people at risk of infection. Countries in Southeast Asia, South Asia, Caribbean, and Latin America share the primary burden of severe epidemics with longer dengue transmission seasons. For instance, Argentina’s severe dengue epidemic of 2023 (Figures 3A, B), was characterised by a significantly more humid and hotter summer, leading to more favourable climatic conditions for Aedes aegypti to survive (100). Brazil reported 1.5 million cases more in 2023 compared to 2022 (1), driven by fragmentation and degradation of the Amazon for infrastructure development (101, 102) and climate change (103). These factors contribute to the spread of dengue to the most remote regions within Brazil, leaving many previously unexposed populations vulnerable to dengue.
South Asia and Southeast Asia continue to have a significant burden of dengue. Bangladesh recorded its worst dengue epidemic in 2023 with more than 300,000 cases, characterised by 414.4% annual increase (Figures 3A, B). Such a severe epidemic was attributed to climate change-related factors in the deltaic and primarily riverine country, leading to the spread of dengue far beyond the urban centres to the remotest rural areas (4). In fact, South Asia and Southeast Asia are one of the most endemic regions in the world, especially India, Sri Lanka, Myanmar, Thailand, and Indonesia (2). A significant and relatively well understood exception in Southeast Asia is Singapore, which reported a 69% drop in dengue cases from 2022 to 2023 (Figure 3B) due to effective governmental controls despite increased conduciveness for dengue due to climate change (104).
It has been estimated that dengue cases could rise by 13% for each 1°C increase in temperature (105). Studies have also taken into consideration of GDP and economic growth as predictive variables and suggested future economic growth could counterbalance dengue case increase, while population growth could be the driving factor in case of any increase in the number of cases (106). Nevertheless, climate change could raise dengue risk further in future, having serious implications for human health (3, 81, 106, 107). To mitigate this risk, understanding HLA’s role across different populations and dengue outcomes is crucial.
From the meta-analysis, we infer that HLA-A*02 is associated with predisposition towards DF and DEN, while also showing a tendency to be a risk factor for DHF. We demonstrate a significant difference between the tendencies of HLA-A*02 associations towards DEN across different regional populations (Figure 6). The predisposition towards dengue infection is primarily driven by the Southeast Asian populations, which signifies that HLA-A*02 could be considered as a risk allele for Southeast Asia in particular (Figure 6). Our inferences about HLA-A*02 provide a significant improvement compared to previous meta-analyses by delving into different pathological outcomes and population-specific patterns of associations (89, 90). In Southeast Asia, HLA-A*02 is a common HLA allele across numerous populations throughout the region (108), thereby could be considered as a warning signal for preparing effective medical and policy measures to prevent future dengue outbreaks.
We highlight that HLA-A*03 is a risk allele for DHF (Figure 7). However, this observation is primarily driven by a Thai population-based study showing a strong predisposition for DHF compared to other studies in the same group (64). However, such inference may not be enough to conclude the mentioned association at the scale of Southeast Asia (Figure 8). More studies within Southeast Asia and Thailand are needed to confirm this association.
HLA-A*24 is inferred to be a predisposing allele towards DHF and DEN (Figure 9), similar to a previous meta-analysis with smaller statistical power (89). However, we uncover the population-specific association in much higher resolution, indicating that HLA-A*24 could be a risk allele for Southeast Asia, while showing similar tendencies in South America and South Asia (Figure 10). In Southeast Asia, HLA-A*24 is relatively common, with the highest frequency within indigenous populations of Malaysia, Myanmar, and Northeastern Thailand, which could make them susceptible to the disease (108). Although showing similar tendencies, the number of South Asian and Latin American studies remain limited, which may not reveal the true tendencies for association.
HLA-B*44 was inferred to have an overall protective association with significant heterogeneity across different studies (Figure 13). However, it revealed contrast in association tendencies when the studies were stratified based on regional populations. In Latin America, driven primarily by Daniela et al., 2014 (59), HLA-B*44 showed predisposition towards dengue, with South Asia and Caribbean following the same tendency (Figure 14). In contrast, HLA-B*44 is found to be associated with protection against DEN in Southeast Asians, driven by a homogenous tendency within the Southeast Asian studies reporting the allele (Figure 14). Within Latin America, this HLA allele is most common within mestizos in Cuba, Argentina, Ecuador, and Brazil, especially in the Amazonia region (108). Interestingly, HLA-B*44 is most common primarily in the Iberian Peninsula, which could point specifically to the colonial origin of this risk to those regions.
The general tendencies of HLA allele associations could be attributed to the possible results of host-pathogen co-evolution (109). This could be especially true for HLA, where there is much support for the idea of pathogen diversity influencing the high polymorphism of the HLA gene regions. A previous study inferred lower binding efficiency for HLA class I A02 and A24 supertypes for all four dengue serotypes, which could explain their observed predisposing tendency towards DEN (110). Similarly, B44 supertypes demonstrated significantly higher binding efficiency, which could explain its protective association within Southeast Asians, however it fails to explain the contrasting observation within Latin Americans (110). Interestingly, dengue and other flavivirus species showed unique binding characteristics with respect to HLA (110).
The overwhelming majority of HLA class I alleles influencing dengue risk highlights their crucial role in dengue pathogenesis. It has been postulated that HLA class I restricted CD8+ T cell lymphocytes determined the immune response and risk towards more DHF. Two ways could mediate such influence by secretion of anti-viral cytokines and cytolysis of infected cells (66). Moreover, secreted inflammatory cytokines can affect vascular cell permeability (111), which could severely damage the cell integrity when DENV epitopes mimic host proteins leading to the loss of self-tolerance of the T cells (112). Such scenarios could lead to serious vascular damage and leaking, leading to DHF complications. Moreover, HLA class I alleles were also found to be upregulated when infected with DENV in vivo, which led to the suppression of natural killer cell response, suggesting a crucial role in dengue pathogenesis for HLA class I alleles (48).
Despite having valuable inferences from previously published case-control studies, we could be missing out on further potentially vital insights on how HLA allele variations influence dengue. The main factors contributing to this potential missing out of vital insights is the lack of case-control studies from the majority of dengue endemic regions or usage of small sample sizes in association studies, hampering association powers. Until now, vast region of tropical Africa having dengue endemic regions remains unrepresented. Whereas in South Asia, which has witnessed several dengue epidemics in recent years, there have been limited HLA association studies with statistically significant sample size. To our knowledge, studies from a single cohort each from vast countries like India (60, 61) and Sri Lanka (71) are the only studies reporting HLA association with dengue. Given the diverse genetic landscapes across such under-represented regions, we may find further crucial insights into how human HLA diversity influences dengue risk, which could shape regional medical strategies and deeper understanding of disease pathogenesis. Sufficient sample size for significant association power, proper consideration of demographic profiles of populations which play crucial role in determining dengue risk and pathogenesis, like age (79) and gender (113, 114) (especially considering immune modulation by sex hormones) should be considered and investigated in detail to understand the full spectrum of HLA mediated immune reaction towards dengue. We further suggest applying population genetics approach on a finer scale to determine HLA-associated dengue risk characterisation. For instance, in a population with multiple ancestries, will the frequency of dengue-associated HLA alleles vary based on the proportion of any particular ancestral component in the genomic makeup of the samples? This could be more pronounced in the recently admixed populations in the Americas, such as mestizos with Native American, European, and African ancestries. Such inferences may help to shape mitigation strategies based on such risk.
Overall, due to current climate change, the populations at risk in the future are bound to increase by many folds. Without effective preparedness, dengue is bound to have serious negative repercussions on public health, influencing the global and national economy and straining medical infrastructures. Conducting a meta-analysis on HLA allele-associated dengue risk, considering the impacts of climate change and evolutionary influences, can yield numerous long-term benefits. Studying the crucial immune gene HLA’s associated with different forms of dengue potentially aid in understanding host genetic factors and mechanism influencing the pathogenesis of dengue, leading to improved predictive models and more effective personalised medical approaches. This comprehensive knowledge could guide public health policies, allowing for targeted interventions and informed policymaking, leading to improved regional and national preparedness. For instance, since our study highlighted the population-specific association of HLA alleles with dengue, the inferences could be utilised to determine the level of risk within a specific population based on the frequency of such HLA allele, aiding in targeted intervention of health policies.
Climate-informed genetic risk assessments, which integrate future climatic projections under various scenarios of climate change, urbanization, and population growth, along with other demographic, environmental, and societal factors, could play a vital role. By considering these elements alongside the genetic makeup of the studied population, these assessments can help understand population-level predispositions to diseases. Such assessments support effective adaptation strategies and resource allocation, bolstering policy advocacy and community resilience. Understanding the relationship between HLA binding and the evolutionary conservation of viral proteins can provide insights into host-pathogen interactions, informing the design of more effective therapies and vaccines (4, 7). This guidance could be crucial since no specific treatments for dengue currently exist. Overall, these benefits significantly enhance dengue prevention and control efforts in the context of changing climate and inform medical policies.
AG: Conceptualization, Data curation, Formal Analysis, Investigation, Methodology, Resources, Software, Visualization, Writing – original draft, Writing – review & editing. HK: Conceptualization, Funding acquisition, Project administration, Supervision, Writing – review & editing. S-SK: Conceptualization, Data curation, Formal Analysis, Investigation, Methodology, Project administration, Resources, Software, Supervision, Validation, Visualization, Writing – original draft, Writing – review & editing.
The author(s) declare that financial support was received for the research and/or publication of this article. The authors acknowledge the financial support from GenomeAsia 100K (https://www.genomeasia100k.org/). This research is supported by the Ministry of Education, Singapore, under its RG35/24 MOE AcRF Tier 1 Award.
We used Grammarly and its assistive generative AI technology (Grammarly Inc., San Fransisco, USA) to check for grammatical coherence and language consistency. Figure 1 was plotted on Tableau Desktop 2021.1 through openly available OpenStreetMap data. OpenStreetMap® is open data, licensed under the Open Data Commons Open Database License (ODbL) by the OpenStreetMap Foundation (OSMF). Figure 2 was created in Biorender along with a Publication License: Created in BioRender. Ghosh, A. (2025) https://BioRender.com/6i14p3g.
The authors declare that the research was conducted in the absence of any commercial or financial relationships that could be construed as a potential conflict of interest.
All claims expressed in this article are solely those of the authors and do not necessarily represent those of their affiliated organizations, or those of the publisher, the editors and the reviewers. Any product that may be evaluated in this article, or claim that may be made by its manufacturer, is not guaranteed or endorsed by the publisher.
1. WHO. Dengue and severe dengue. (2024). https://www.who.int/news-room/fact-sheets/detail/dengue-and-severe-dengue.
2. WHO. World Health Organisation. Disease Outbreak News; Dengue - Global Situation. (2024). doi: https://www.who.int/emergencies/disease-outbreak-news/item/2024-DON518.
3. Bhatt S, Gething PW, Brady OJ, Messina JP, Farlow AW, Moyes CL, et al. The global distribution and burden of dengue. Nature. (2013) 496:7446. doi: 10.1038/nature12060
4. Araf Y, Akter M, Zhai J, Zheng C, Hossain MG. Emerging health implications of climate change: dengue outbreaks and beyond in Bangladesh. Lancet Microbe. (2024) 5:e213. doi: 10.1016/S2666-5247(23)00366-X
5. Romanello M, Napoli Cd, Green C, Kennard H, Lampard P, Scamman D, et al. The 2023 report of the Lancet Countdown on health and climate change: the imperative for a health-centred response in a world facing irreversible harms. Lancet. (2023) 402:2346–94. doi: 10.1016/S0140-6736(23)01859-7
6. Guzman MG, Halstead SB, Artsob H, Buchy P, Farrar J, Gubler DJ, et al. Dengue: a continuing global threat. Nat Rev Microbiol. (2010) 8:S7–16. doi: 10.1038/nrmicro2460
8. Mukhopadhyay S, Kuhn RJ, Rossmann MG. A structural perspective of the flavivirus life cycle. Nat Rev Microbiol. (2005) 3:13–22. doi: 10.1038/nrmicro1067
9. Kuhn RJ, Zhang W, Rossmann MG, Pletnev SV, Corver J, Lenches E, et al. Structure of dengue virus: Implications for flavivirus organization, maturation, and fusion. Cell. (2002) 108:717–25. doi: 10.1016/S0092-8674(02)00660-8
10. Byk LA, Gamarnik AV. Properties and functions of the dengue virus capsid protein. Annu Rev Virol. (2016) 3:263–81. doi: 10.1146/annurev-virology-110615-042334
11. Dwivedi VD, Tripathi IP, Tripathi RC, Bharadwaj S, Mishra SK. Genomics, proteomics and evolution of dengue virus. Brief Funct Genomics. (2017) 16:217–27. doi: 10.1093/bfgp/elw040
12. Muller DA, Young PR. The flavivirus NS1 protein: molecular and structural biology, immunology, role in pathogenesis and application as a diagnostic biomarker. Antiviral Res. (2013) 98:192–208. doi: 10.1016/j.antiviral.2013.03.008
13. Bhatnagar P, Sreekanth GP, Murali-Krishna K, Chandele A, Sitaraman R. Dengue virus non-structural protein 5 as a versatile, multi-functional effector in host–pathogen interactions. Front Cell Infect Microbiol. (2021) 11:574067. doi: 10.3389/fcimb.2021.574067
14. McLean JE, Wudzinska A, Datan E, Quaglino D, Zakeri Z. Flavivirus NS4A-induced autophagy protects cells against death and enhances virus replication. J Biol Chem. (2011) 286:22147–59. doi: 10.1074/jbc.M110.192500
15. Fragnoud R, Yugueros-Marcos J, Pachot A, Bedin F. Isotope Coded Protein Labeling analysis of plasma specimens from acute severe dengue fever patients. Proteome Sci. (2012) 10:1-8. doi: 10.1186/1477-5956-10-60
16. Acosta EG, Kumar A, Bartenschlager R. Revisiting dengue virus-host cell interaction: new insights into molecular and cellular virology. Adv Virus Res. (2014) 88:1–109. doi: 10.1016/B978-0-12-800098-4.00001-5
17. Montoya M, Gresh L, Mercado JC, Williams KL, Vargas MJ, Gutierrez G, et al. Symptomatic versus inapparent outcome in repeat dengue virus infections is influenced by the time interval between infections and study year. PloS Negl Trop Dis. (2013) 7:e2357. doi: 10.1371/journal.pntd.0002357
18. Guzman MG, Alvarez M, Halstead SB. Secondary infection as a risk factor for dengue hemorrhagic fever/dengue shock syndrome: an historical perspective and role of antibody-dependent enhancement of infection. Arch Virol. (2013) 158:1445–59. doi: 10.1007/s00705-013-1645-3
19. Kouri GP, Guzmán MG, Bravo JR. Why dengue haemorrhagic fever in Cuba? 2. An integral analysis. Trans R Soc Trop Med Hyg. (1987) 81:821–3. doi: 10.1016/0035-9203(87)90042-3
20. Hammon WM. Dengue hemorrhagic fever–do we know its cause? Am J Trop Med Hyg. (1973) 22:82–91. doi: 10.4269/ajtmh.1973.22.82
21. Pang T. Delayed-type hypersensitivity: probable role in the pathogenesis of dengue hemorrhagic fever/dengue shock syndrome. Rev Infect Dis. (1983) 5:346–52. doi: 10.1093/clinids/5.2.346
22. Rosen L. Disease exacerbation caused by sequential dengue infections: myth or reality? Rev Infect Dis. (1989) 11 Suppl 4:S840–2. doi: 10.1093/clinids/11.supplement_4.s840
23. Halstead SB. Antibody, macrophages, dengue virus infection, shock, and hemorrhage: a pathogenetic cascade. Rev Infect Dis. (1989) 11 Suppl 4:830–9. doi: 10.1093/clinids/11.Supplement_4.S830
24. Halstead SB, Mahalingam S, Marovich MA, Ubol S, Mosser DM. Intrinsic antibody-dependent enhancement of microbial infection in macrophages: disease regulation by immune complexes. Lancet Infect Dis. (2010) 10:712–22. doi: 10.1016/S1473-3099(10)70166-3
25. Durham ND, Agrawal A, Waltari E, Croote D, Zanini F, Fouch M, et al. Broadly neutralizing human antibodies against dengue virus identified by single B cell transcriptomics. Elife. (2019) 8:e52384. doi: 10.7554/eLife.52384
26. Flipse J, Wilschut J, Smit JM. Molecular mechanisms involved in antibody-dependent enhancement of dengue virus infection in humans. Traffic. (2013) 14:25–35. doi: 10.1111/tra.2013.14.issue-1
27. Flipse J, Diosa-Toro MA, Hoornweg TE, Van De Pol DPI, Urcuqui-Inchima S, Smit JM. Antibody-dependent enhancement of dengue virus infection in primary human macrophages; balancing higher fusion against antiviral responses. Sci Rep. (2016) 6:29201 1-13. doi: 10.1038/srep29201
28. Huang X, Yue Y, Li D, Zhao Y, Qiu L, Chen J, et al. Antibody-dependent enhancement of dengue virus infection inhibits RLR-mediated Type-I IFN-independent signalling through upregulation of cellular autophagy. Sci Rep. (2016) 6:22303 1-13. doi: 10.1038/srep22303
29. Lee MF, Voon GZ, Lim HX, Chua ML, Poh CL. Innate and adaptive immune evasion by dengue virus. Front Cell Infect Microbiol. (2022) 12. doi: 10.3389/fcimb.2022.1004608
30. Richter MKS, Da Silva Voorham JM, Torres Pedraza S, Hoornweg TE, Van De Pol DPI, Rodenhuis-Zybert IA, et al. Immature dengue virus is infectious in human immature dendritic cells via interaction with the receptor molecule DC-SIGN. PloS One. (2014) 9:e98785. doi: 10.1371/journal.pone.0098785
31. Briseño JAA, Pereira LR, van der Laan M, Pauzuolis M, ter Ellen BM, Upasani V, et al. TLR2 axis on peripheral blood mononuclear cells regulates inflammatory responses to non-infectious immature dengue virus particles. PloS Pathog. (2022) 18:e1010499. doi: 10.1371/JOURNAL.PPAT.1010499
32. Wang TT, Sewatanon J, Memoli MJ, Wrammert J, Bournazos S, Bhaumik SK, et al. IgG antibodies to dengue enhanced for FcγRIIIA binding determine disease severity. Science. (2017) 355:395–8. doi: 10.1126/science.aai8128
33. Bournazos S, Vo HTM, Duong V, Auerswald H, Ly S, Sakuntabhai A, et al. Antibody fucosylation predicts disease severity in secondary dengue infection. Sci (1979). (2021) 372:1102–5. doi: 10.1126/science.abc7303
34. Malavige GN, Ogg GS. Pathogenesis of vascular leak in dengue virus infection. Immunology. (2017) 151:261–9. doi: 10.1111/imm.2017.151.issue-3
35. St John AL, Rathore AP, Raghavan B, Ng ML, Abraham SN. Contributions of mast cells and vasoactive products, leukotrienes and chymase, to dengue virus-induced vascular leakage. Elife. (2013) 2:e00481. doi: 10.7554/eLife.00481
36. Malavige GN, Ogg GS. Molecular mechanisms in the pathogenesis of dengue infections. Trends Mol Med. (2024) 30:484–98. doi: 10.1016/j.molmed.2024.03.006
37. Jeewandara C, Gomes L, Wickramasinghe N, Gutowska-Owsiak D, Waithe D, Paranavitane SA, et al. Platelet activating factor contributes to vascular leak in acute dengue infection. PloS Negl Trop Dis. (2015) 9:e0003459. doi: 10.1371/journal.pntd.0003459
38. van de Weg CAM, Pannuti CS, van den Ham HJ, de Araújo ESA, Boas LSV, Felix AC, et al. Serum angiopoietin-2 and soluble VEGF receptor 2 are surrogate markers for plasma leakage in patients with acute dengue virus infection. J Clin Virol. (2014) 60:328–35. doi: 10.1016/j.jcv.2014.05.001
39. Rathore APS, Senanayake M, Athapathu AS, Gunasena S, Karunaratna I, Leong WY, et al. Serum chymase levels correlate with severe dengue warning signs and clinical fluid accumulation in hospitalized pediatric patients. Sci Rep. (2020) 10:11856. doi: 10.1038/s41598-020-68844-z
40. Masri MFB, Mantri CK, Rathore APS, John ALS. Peripheral serotonin causes dengue virus–induced thrombocytopenia through 5HT2 receptors. Blood. (2019) 133:2325–37. doi: 10.1182/blood-2018-08-869156
41. Grange L, Simon-Loriere E, Sakuntabhai A, Gresh L, Paul R, Harris E. Epidemiological risk factors associated with high global frequency of inapparent dengue virus infections. Front Immunol. (2014) 5. doi: 10.3389/fimmu.2014.00280
42. Aggarwal C, Ahmed H, Sharma P, Reddy ES, Nayak K, Singla M, et al. Severe disease during both primary and secondary dengue virus infections in pediatric populations. Nat Med. (2024) 30:670–4. doi: 10.1038/s41591-024-02798-x
43. Sangkaew S, Ming D, Boonyasiri A, Honeyford K, Kalayanarooj S, Yacoub S, et al. Risk predictors of progression to severe disease during the febrile phase of dengue: a systematic review and meta-analysis. Lancet Infect Dis. (2021) 21:1014–26. doi: 10.1016/S1473-3099(20)30601-0
44. Kan FK, Tan CC, Von Bahr Greenwood T, Khalid KE, Supramaniam P, Hed Myrberg I, et al. Dengue infection complicated by hemophagocytic lymphohistiocytosis: experiences from 180 patients with severe dengue. Clin Infect Dis. (2020) 70:2247–55. doi: 10.1093/cid/ciz499
45. Usmani GN, Woda BA, Newburger PE. Advances in understanding the pathogenesis of HLH. Br J Haematol. (2013) 161:609–22. doi: 10.1111/bjh.2013.161.issue-5
46. Wills BA, Oragui EE, Stephens AC, Daramola OA, Dung NM, Loan HT, et al. Coagulation abnormalities in dengue hemorrhagic Fever: serial investigations in 167 Vietnamese children with Dengue shock syndrome. Clin Infect Dis. (2002) 35:277–85. doi: 10.1086/cid.2002.35.issue-3
47. Ojha A, Nandi D, Batra H, Singhal R, Annarapu GK, Bhattacharyya S, et al. Platelet activation determines the severity of thrombocytopenia in dengue infection. Sci Rep. (2017) 7:41697. doi: 10.1038/srep41697
48. McKechnie JL, Beltrán D, Pitti A, Saenz L, Araúz AB, Vergara R, et al. HLA upregulation during dengue virus infection suppresses the natural killer cell response. Front Cell Infect Microbiol. (2019) 9:473503. doi: 10.3389/fcimb.2019.00268
49. de Alwis R, Bangs DJ, Angelo MA, Cerpas C, Fernando A, Sidney J, et al. Immunodominant dengue virus-specific CD8+ T cell responses are associated with a memory PD-1+ Phenotype. J Virol. (2016) 90:4771–9. doi: 10.1128/JVI.02892-15
50. Simmons CP, Dong T, Chau NV, Dung NTP, Chau TNB, Thao LTT, et al. Early T-cell responses to dengue virus epitopes in Vietnamese adults with secondary dengue virus infections. J Virol. (2005) 79:5665–75. doi: 10.1128/JVI.79.9.5665-5675.2005
51. Weiskopf D, Angelo MA, de Azeredo EL, Sidney J, Greenbaum JA, Fernando AN, et al. Comprehensive analysis of dengue virus-specific responses supports an HLA-linked protective role for CD8 + T cells. Proc Natl Acad Sci. (2013) 110:22E2046-E2053. doi: 10.1073/pnas.1305227110
52. Fink J, Gu F, Vasudevan SG. Role of T cells, cytokines and antibody in dengue fever and dengue haemorrhagic fever. Rev Med Virol. (2006) 16:263–75. doi: 10.1002/(ISSN)1099-1654
53. Zivna I, Green S, Vaughn DW, Kalayanarooj S, Stephens HAF, Chandanayingyong D, et al. T cell responses to an HLA-B*07-restricted epitope on the dengue NS3 protein correlate with disease severity. J Immunol. (2002) 168:5959–65. doi: 10.4049/jimmunol.168.11.5959
54. Munro A, Bright S. Products of the major histocompatibility complex and their relationship to the immune response. Nature. (1976) 264:145–52. doi: 10.1038/264145a0
55. Chiewsilp P, Scott RM, Bhamarapravati N. Histocompatibility antigens and dengue hemorrhagic fever. Am J Trop Med Hyg. (1981) 30:1100–5. doi: 10.4269/ajtmh.1981.30.1100
56. Mercado ES, Espino FE, Perez MLM, Bilar JM, Bajaro JDP, Huy NT, et al. HLA-A∗33:01 as protective allele for severe dengue in a population of Filipino children. PloS One. (2015) 10:1–11. doi: 10.1371/journal.pone.0115619
57. Xavier Eurico De Alencar L, De Mendonça Braga-Neto U, José Moura Do Nascimento E, Tenório Cordeiro M, Maria Silva A, Alexandre Antunes De Brito C, et al. HLA-B*44 is associated with dengue severity caused by DENV-3 in a Brazilian population. J Trop Med. (2013) 2013:648475. doi: 10.1155/2013/648475
58. Monteiro SP, do Brasil PEAA, Cabello GMK, de Souz RV, Brasil P, Georg I, et al. HLA-A*01 allele: A risk factor for dengue haemorrhagic fever in Brazil’s population. Mem Inst Oswaldo Cruz. (2012) 107:224–30. doi: 10.1590/S0074-02762012000200012
59. Cardozo DM, Moliterno RA, Sell AM, Guelsin GAS, Beltrame LM, Clementino SL, et al. Evidence of HLA-DQB1 contribution to susceptibility of dengue serotype 3 in dengue patients in Southern Brazil. J Trop Med. (2014) 2014:968262. doi: 10.1155/2014/968262
60. Alagarasu K, Mulay AP, Singh R, Gavade VB, Shah PS, Cecilia D. Association of HLA-DRB1 and TNF genotypes with dengue hemorrhagic fever. Hum Immunol. (2013) 74:610–7. doi: 10.1016/j.humimm.2013.01.027
61. Alagarasu K, Mulay AP, Sarikhani M, Rashmika D, Shah PS, Cecilia D. Profile of human leukocyte antigen class I alleles in patients with dengue infection from Western India. Hum Immunol. (2013) 74:1624–8. doi: 10.1016/j.humimm.2013.08.004
62. Lan NTP, Kikuchi M, Huong VTQ, Ha DQ, Thuy TT, Tham VD, et al. Protective and enhancing HLA alleles, HLA-DRB1*0901 and HLA-A*24, for severe forms of dengue virus infection, dengue hemorrhagic fever and dengue shock syndrome. PloS Negl Trop Dis. (2008) 2:1–8. doi: 10.1371/journal.pntd.0000304
63. LaFleur C, Granados J, Vargas-Alarcon G, Ruíz-Morales J, Villarreal-Garza C, Higuera L, et al. HLA-DR antigen frequencies in Mexican patients with dengue virus infection: HLA-DR4 as a possible genetic resistance factor for dengue hemorrhagic fever. Hum Immunol. (2002) 63:1039–44. doi: 10.1016/S0198-8859(02)00682-1
64. Vejbaesya S, Thongpradit R, Kalayanarooj S, Luangtrakool K, Luangtrakool P, Gibbons RV, et al. HLA class I supertype associations with clinical outcome of secondary dengue virus infections in ethnic thais. J Infect Diseases. (2015) 212:939–47. doi: 10.1093/infdis/jiv127
65. Fernández-Mestre M, Navarrete CV, Brown J, Brown C, Correa E, Layrisse Z. HLA alleles and dengue virus infection in Venezuelan patients: A preliminary study. Inmunologia. (2009) 28:96–100. doi: 10.1016/S0213-9626(09)70031-9
66. Loke H, Bethell DB, Phuong CXT, Dung M, Schneider J, White NJ, et al. Strong HLA class I-restricted T cell responses in dengue hemorrhagic fever: A double-edged sword? J Infect Dis. (2001) 184:1369–73. doi: 10.1086/jid.2001.184.issue-11
67. Appanna R, Ponnampalavanar S, Chai See LL, Devi Sekaran S. Susceptible and protective HLA Class 1 alleles against dengue fever and dengue hemorrhagic fever patients in a Malaysian population. PloS One. (2010) 5:1–10. doi: 10.1371/journal.pone.0013029
68. Polizel JR, Bueno D, Visentainer JEL, Sell AM, Borelli SD, Tsuneto LT, et al. Association of human leukocyte antigen DQ1 and dengue fever in a white Southern Brazilian population. Mem Inst Oswaldo Cruz. (2004) 99:559–62. doi: 10.1590/S0074-02762004000600003
69. Sierra B, Alegre R, Pérez AB, García G, Sturn-Ramirez K, Obasanjo O, et al. HLA-A, -B, -C, and -DRB1 allele frequencies in Cuban individuals with antecedents of dengue 2 disease: Advantages of the Cuban population for HLA studies of dengue virus infection. Hum Immunol. (2007) 68:531–40. doi: 10.1016/j.humimm.2007.03.001
70. Falcón-Lezama JA, Ramos C, Zuñiga J, Juárez-Palma L, Rangel-Flores H, García-Trejo AR, et al. HLA class I and II polymorphisms in Mexican Mestizo patients with dengue fever. Acta Trop. (2009) 112:193–7. doi: 10.1016/j.actatropica.2009.07.025
71. Malavige GN, Rostron T, Rohanachandra LT, Jayaratne SD, Fernando N, de Silva AD, et al. HLA class I and class II associations in Dengue viral infections in a Sri Lankan population. PloS One. (2011) 6:1–8. doi: 10.1371/journal.pone.0020581
72. Brown MG, Salas R, Vickers I, Heslop O, Smikle M. Dengue HLA associations in Jamaicans. West Indian Med J. (2011) 60:7–12.
73. Lan NTP, Hirayama K. Host genetic susceptibility to severe dengue infection. Trop Med Health. (2011) 39:73–81. doi: 10.2149/tmh.2011-S08
74. Katzelnick LC, Escoto AC, Huang AT, Garcia-Carreras B, Chowdhury N, Berry IM, et al. Antigenic evolution of dengue viruses over 20 years. Sci (1979). (2021) 374:999–1004. doi: 10.1126/science.abk0058
75. Parameswaran P, Wang C, Trivedi SB, Eswarappa M, Montoya M, Balmaseda A, et al. Intrahost selection pressures drive rapid dengue virus microevolution in acute human infections. Cell Host Microbe. (2017) 22:400–410.e5. doi: 10.1016/j.chom.2017.08.003
76. Kolimenakis A, Heinz S, Wilson ML, Winkler V, Yakob L, Michaelakis A, et al. The role of urbanisation in the spread of Aedes mosquitoes and the diseases they transmit—A systematic review. PloS Negl Trop Dis. (2021) 15:e0009631. doi: 10.1371/journal.pntd.0009631
77. Whiteman A, Loaiza JR, Yee DA, Poh KC, Watkins AS, Lucas KJ, et al. Do socioeconomic factors drive Aedes mosquito vectors and their arboviral diseases? A systematic review of dengue, chikungunya, yellow fever, and Zika Virus. One Health. (2020) 11:100188. doi: 10.1016/j.onehlt.2020.100188
78. Barmak DH, Dorso CO, Otero M. Modelling dengue epidemic spreading with human mobility. Physica A: Stat Mechanics its Applications. (2016) 447:129–40. doi: 10.1016/j.physa.2015.12.015
79. Chagas GCL, Rangel AR, Noronha LM, Veloso FCS, Kassar SB, Oliveira MJC, et al. Risk factors for mortality in patients with dengue: A systematic review and meta-analysis. Trop Med Int Health. (2022) 27:656–68. doi: 10.1111/tmi.13797
80. Trejoid I, Barnardid M, Spencerid JA, Keithleyid J, Martinez KM, Crooker I, et al. Changing temperature profiles and the risk of dengue outbreaks. PloS Climate. (2023) 2:e0000115. doi: 10.1371/journal.pclm.0000115
81. Aliaga-Samanez A, Cobos-Mayo M, Real R, Segura M, Romero D, Fa JE, et al. Worldwide dynamic biogeography of zoonotic and anthroponotic dengue. PloS Negl Trop Dis. (2021) 15:e0009496. doi: 10.1371/journal.pntd.0009496
82. Messina JP, Brady OJ, Golding N, Kraemer MUG, Wint GRW, Ray SE, et al. The current and future global distribution and population at risk of dengue. Nat Microbiol. (2019) 4:1508–15. doi: 10.1038/s41564-019-0476-8
83. Oliveira S, Rocha J, Sousa CA, Capinha C. Wide and increasing suitability for Aedes albopictus in Europe is congruent across distribution models. Sci Rep. (2021) 11:1–9. doi: 10.1038/s41598-021-89096-5
84. Lozano-Fuentes S, Hayden MH, Welsh-Rodriguez C, Ochoa-Martinez C, Tapia-Santos B, Kobylinski KC, et al. The Dengue Virus Mosquito Vector Aedes aEgypti at High Elevation in México. Am J Trop Med Hyg. (2012) 87:902. doi: 10.4269/ajtmh.2012.12-0244
85. Liu Z, Zhang Q, Li L, He J, Guo J, Wang Z, et al. The effect of temperature on dengue virus transmission by Aedes mosquitoes. Front Cell Infect Microbiol. (2023) 13:1242173. doi: 10.3389/fcimb.2023.1242173
86. Chan M, Johansson MA. The incubation periods of dengue viruses. PloS One. (2012) 7:e50972. doi: 10.1371/journal.pone.0050972
87. Yang HM, Macoris MLG, Galvani KC, Andrighetti MTM, Wanderley DMV. Assessing the effects of temperature on dengue transmission. Epidemiol Infect. (2009) 137:1179–87. doi: 10.1017/S0950268809002052
88. Wagenaar JFP, Mairuhu ATA, van Gorp ECM. Genetic influences on dengue virus infections. Dengue Bull. (2004) 28:126–34.
89. Cook AC, Thibaut D, Pettersen T. Major histocompatibility complex class I and dengue hemorrhagic fever: A meta-analysis of human leukocyte antigens A*24 and B*44. Cureus. (2022) 14(11):e31485. doi: 10.7759/cureus.31485
90. Chen Y, Liao Y, Yuan K, Wu A, Liu L. HLA-A, -B, -DRB1 alleles as genetic predictive factors for dengue disease: A systematic review and meta-analysis. Viral Immunol. (2019) 32:121–30. doi: 10.1089/vim.2018.0151
91. European Centre for Disease Prevention and Control. Dengue worldwide overview (2024). Available online at: https://www.ecdc.europa.eu/en/dengue-monthly (Accessed June 24, 2024).
92. Government of India. DENGUE SITUATION IN INDIA: National Center for Vector Borne Diseases Control (NCVBDC) (2023). Available online at: https://ncvbdc.mohfw.gov.in/index4.php?lang=1&level=0&linkid=431&lid=3715 (Accessed June 24, 2024).
93. Kung J. Elicit (product review). J Can Health Libraries Assoc / J l’Association Des bibliothèques la santé du Canada. (2023) 44:15–19. doi: 10.29173/jchla29657
94. PRISMA. PRISMA Flow Diagram - “Prisma 2020 flow diagram for new systematic reviews which included searches of databases and other sources” (2020). Available online at: http://prisma-statement.org/documents/PRISMA_2020_flow_diagram_new_SRs_v2.docx (Accessed May 20, 2024).
95. Paradoa Pérez ML, Trujillo Y, Basanta P. Association of dengue hemorrhagic fever with the HLA system. Haematologia (Budap). (1987) 20:83–7.
96. Weiskopf D, Angelo MA, Grifoni A, O’Rourke PH, Sidney J, Paul S, et al. HLA-DRB1 alleles are associated with different magnitudes of dengue virus-specific CD4+ T-cell responses. J Infect Diseases. (2016) 214:1117–24. doi: 10.1093/infdis/jiw309
97. Stephens HAF, Klaythong R, Sirikong M, Vaughn DW, Green S, Kalayanarooj S, et al. HLA-A and -B allele associations with secondary dengue virus infections correlate with disease severity and the infecting viral serotype in ethnic Thais. Tissue Antigens. (2002) 60:309–18. doi: 10.1034/j.1399-0039.2002.600405.x
98. Fernández-Mestre MT, Vargas V, Montagnani S, Cotúa M, Ogando V, Layrisse Z. HLA Class II and class I polymorphism in Venezuelan patients with myasthenia gravis. Hum Immunol. (2004) 65:54–9. doi: 10.1016/j.humimm.2003.10.003
99. Review Manager (RevMan). Version (8.0.0). The Cochrane Collaboration (2024). Available at: http://revman.cochrane.org/ (Accessed June 11, 2024).
100. Taylor L. Dengue: Argentinians turn to homemade repellent amid surge in cases. BMJ. (2024) 385. doi: 10.1136/bmj.q885
101. Lana RM, Gomes MF da C, Lima TFMd, Honório NA, Codeço CT. The introduction of dengue follows transportation infrastructure changes in the state of Acre, Brazil: A network-based analysis. PloS Negl Trop Dis. (2017) 11:e0006070. doi: 10.1371/journal.pntd.0006070
102. Lowe R, Lee S, Martins Lana R, Torres Codeço C, Castro MC, Pascual M. Emerging arboviruses in the urbanized Amazon rainforest. BMJ. (2020) 371. doi: 10.1136/bmj.m4385
103. Lee SA, Economou T, Catão R de C, Barcellos C, Lowe R. The impact of climate suitability, urbanisation, and connectivity on the expansion of dengue in 21st century Brazil. PloS Negl Trop Dis. (2021) 15:e0009773. doi: 10.1371/journal.pntd.0009773
104. NEA.Dengue Cases Dropped By 69 Per Cent To 9,949 Cases In 2023; Vigilance Needed To prevent Dengue Surge In 2024 . Available online at: https://www.nea.gov.sg/media/news/news/index/dengue-cases-dropped-69-per-cent-9-949-in-2023-vigilance-needed-prevent-dengue-surge-2024 (Accessed June 24, 2024).
105. Damtew YT, Tong M, Varghese BM, Anikeeva O, Hansen A, Dear K, et al. Effects of high temperatures and heatwaves on dengue fever: a systematic review and meta-analysis. EBioMedicine. (2023) 91:104582. doi: 10.1016/j.ebiom.2023.104582
106. Colón-González FJ, Gibb R, Khan K, Watts A, Lowe R, Brady OJ. Projecting the future incidence and burden of dengue in Southeast Asia. Nat Commun. (2023) 14:5439. doi: 10.1038/s41467-023-41017-y
107. Wang Y, Li C, Zhao S, Wei Y, Li K, Jiang X, et al. Projection of dengue fever transmissibility under climate change in South and Southeast Asian countries. PloS Negl Trop Dis. (2024) 18:e0012158. doi: 10.1371/journal.pntd.0012158
108. Gonzalez-Galarza FF, McCabe A, Santos EJMD, Jones J, Takeshita L, Ortega-Rivera ND, et al. Allele frequency net database (AFND) 2020 update: gold-standard data classification, open access genotype data and new query tools. Nucleic Acids Res. (2020) 48:D783–8. doi: 10.1093/nar/gkz1029
109. Nunes K, Maia MHT, dos Santos EJM, dos Santos SEB, Guerreiro JF, Petzl-Erler ML, et al. How natural selection shapes genetic differentiation in the MHC region: A case study with Native Americans. Hum Immunol. (2021) 82:523–31. doi: 10.1016/j.humimm.2021.03.005
110. Hertz T, Nolan D, James I, John M, Gaudieri S, Phillips E, et al. Mapping the landscape of host-pathogen coevolution: HLA class I binding and its relationship with evolutionary conservation in human and viral proteins. J Virol. (2011) 85:1310–21. doi: 10.1128/JVI.01966-10
111. Gubler DJ. Dengue and dengue hemorrhagic fever. Clin Microbiol Rev. (1998) 11:480–96. doi: 10.1128/CMR.11.3.480
112. Klenerman P, Rowland-Jones S, Nature SM. U. Cytotoxic T-cell activity antagonized by naturally occurring HIV-1 Gag variants. Nature. (1994) 1994:403-407. doi: 10.1038/369403a0
113. Sciarra F, Campolo F, Franceschini E, Carlomagno F, Venneri M. Gender-specific impact of sex hormones on the immune system. Int J Mol Sci. (2023) 24:6302. doi: 10.3390/ijms24076302
Keywords: dengue, HLA, dengue fever (DF), dengue haemorrhagic fever, serotype, climate change
Citation: Ghosh AG, Kim HL and Khor S-S (2025) HLA alleles and dengue susceptibility across populations in the era of climate change: a comprehensive review. Front. Immunol. 16:1473475. doi: 10.3389/fimmu.2025.1473475
Received: 01 August 2024; Accepted: 26 March 2025;
Published: 15 April 2025.
Edited by:
Luwen Zhang, University of Nebraska-Lincoln, United StatesReviewed by:
Willy A. Valdivia-Granda, Orion Integrated Biosciences, United StatesCopyright © 2025 Ghosh, Kim and Khor. This is an open-access article distributed under the terms of the Creative Commons Attribution License (CC BY). The use, distribution or reproduction in other forums is permitted, provided the original author(s) and the copyright owner(s) are credited and that the original publication in this journal is cited, in accordance with accepted academic practice. No use, distribution or reproduction is permitted which does not comply with these terms.
*Correspondence: Seik-Soon Khor, c2Vpa3Nvb25AZ21haWwuY29t
Disclaimer: All claims expressed in this article are solely those of the authors and do not necessarily represent those of their affiliated organizations, or those of the publisher, the editors and the reviewers. Any product that may be evaluated in this article or claim that may be made by its manufacturer is not guaranteed or endorsed by the publisher.
Research integrity at Frontiers
Learn more about the work of our research integrity team to safeguard the quality of each article we publish.