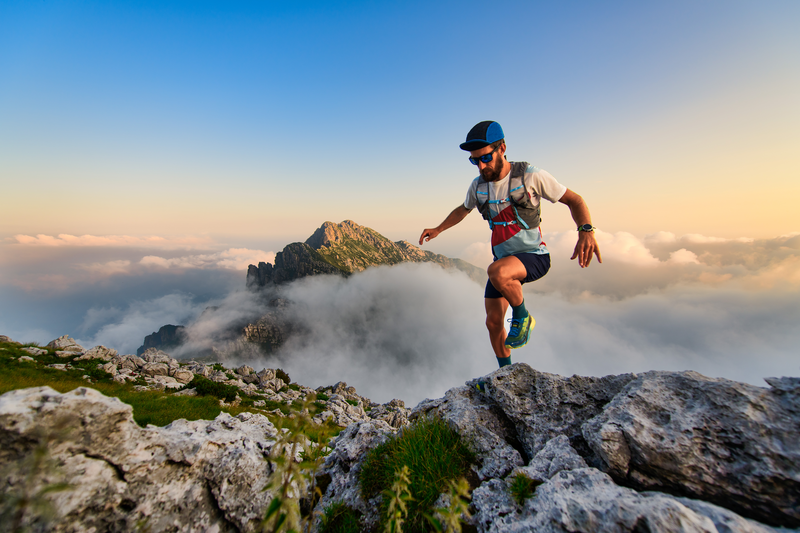
95% of researchers rate our articles as excellent or good
Learn more about the work of our research integrity team to safeguard the quality of each article we publish.
Find out more
REVIEW article
Front. Immunol. , 06 February 2025
Sec. Microbial Immunology
Volume 16 - 2025 | https://doi.org/10.3389/fimmu.2025.1438683
This article is part of the Research Topic Gut Feelings: Investigating the Link Between Microbiota and Kidney Disease Progression View all 9 articles
Immunoglobulin A nephropathy (IgAN) is the most prevalent glomerulonephritis in the world, and it is one of the leading causes of end-stage kidney disease. It is now believed that the pathogenesis of IgAN is the mesangial deposition of immune complex containing galactose-deficient IgA1, resulting in glomerular injury. Current treatments for IgAN include supportive care and immunosuppressive therapy. A growing number of studies found that the gut microbiota in IgAN was dysregulated. Gut microbiota may be involved in the development and progression of IgAN through three main aspects: destruction of intestinal barrier, changes in metabolites and abnormal mucosal immunity. Interestingly, therapies by modulating the gut microbiota, such as fecal microbiota transplantation, antibiotic treatment, probiotic treatment, Chinese herbal medicine Zhen Wu Tang treatment, gluten-free diet, and hydroxychloroquine treatment, can improve IgAN. In this review, the alteration of gut microbiota in IgAN, potential pathogenic roles of gut microbiota on IgAN and potential approaches to treat IgAN by modulating the gut microbiota are summarized.
Immunoglobulin A nephropathy (IgAN) is the most common form of glomerulonephritis in the world, characterized by the deposition of IgA-dominated immune complexes in the glomerular mesangium (1). The prevalence of IgAN varies in geography, with the Asia-Pacific region having the highest prevalence and Africa the lowest (1). It is reported that the overall population incidence of IgAN is at least 2.5 per 100000 (2). IgAN accounts for the largest proportion of causes of chronic kidney disease (CKD) in China (3). The clinical manifestations of IgAN are varied, with the most common manifestations being asymptomatic hematuria and progressive kidney disease (4). It is now believed that four major hits are involved in the pathogenesis of IgAN. Firstly, circulating galactose-deficient IgA1(Gd-IgA1) increases hereditarily; secondly, the autoimmune antibodies IgG and IgA directed against Gd-IgA1 are synthesized to form immune complexes; finally, the immune complexes containing Gd-IgA1 are deposited in the glomerular mesangium, which activates the mesangial cells and the complement system, leading to glomerular injury (5). 30% to 40% of patients with IgAN will develop end-stage renal disease (ESRD) within 20 to 30 years after the diagnosis (6). The current treatment of IgAN mainly includes supportive care and immunosuppressive therapy (7). Further, supportive care mainly includes control of blood pressure and proteinuria and correction of diet and lifestyle, while immunosuppressive therapy mainly includes the use of corticosteroids (8). All IgAN patients are recommended to receive supportive care, while immunosuppressive therapy is recommended for patients whose proteinuria is not effectively controlled after receiving maximal supportive care (8). However, immunosuppressive therapy with corticosteroids is still controversial due to its efficacy and side effects (9).
Gut microbiota, a highly complex community of microbes in the gastrointestinal tract, contains over 1,000 different species of bacteria (10, 11). It coexists and interacts with the host, and plays an important role in substance metabolism, maintaining the integrity of intestinal mucosa, defending against pathogens and immune regulation (12). In recent years, crosstalk between the gut and kidney, also known as the gut-kidney axis, has been found in some kidney diseases (13). For example, in CKD, uremia has an influence on the composition and metabolism of the gut microbiota, while the metabolism of gut microbiota produces important uremic toxins (14). It was also found that the gut-kidney axis plays an important role in IgAN (15). Gut microbiota might be involved in the development and progression of IgAN (16). Interestingly, some treatments that modulate the gut microbiota have been shown to improve IgAN (17). In this review, we summarize the changes of gut microbiota in IgAN, the potential pathogenic role of gut microbiota in IgAN, and the potential therapies of IgAN by modulating gut microbiota.
In recent years, some studies have found that the gut microbiota of IgAN animal model was changed compared with that of normal control animals. Li et al. (18) found that IgAN rats had different diversity and composition of gut microbiota from normal rats. Moreover, they showed that the blood creatinine and urine protein were closely related to the changes of gut microbiota in IgAN rats (18). And some biochemical indices such as blood creatinine, blood urea nitrogen, urine volume, 24-h protein urine, etc., were positively or negatively correlated with the abundance of several genera of gut microbiota (18). These suggested that there is a potential association between gut microbiota dysbiosis and renal insufficiency. Likewise, IgAN mice also had the gut microbiota with different diversity and composition compared with normal control mice. These mice showed gut microbiota dysbiosis with a significant reduction in Bifidobacterium levels, which can also be found in IgAN patients (19). Studies comparing gut microbiota between IgAN animal models and normal animal controls are summarized in Table 1.
In conclusion, IgAN animal models exhibit gut microbiota dysbiosis. The difference of gut microbiota is expected to be a biomarker for the non-invasive diagnosis of IgAN.
Similarly, increasing studies also have revealed that IgAN patients have different gut microbiota from normal people (20–23). Furthermore, IgAN patients have a different diversity of gut microbiota from normal people, and the diversity of gut microbiota in IgAN patients is lower than normal people (19, 24, 25). It was also found that the composition of gut microbiota in IgAN patients was significantly different from that in normal controls (19, 25–31). Moreover, the dysbiosis of gut microbiota in IgAN patients also differs between men and women (32). Most studies compared the gut microbiota of IgAN patients with normal controls and found the consistent result that the relative abundance of Escherichia-Shigella increased in the gut microbiota of IgAN patients (22–24, 26, 33–36). Zhong et al. (26) reported that patients with a higher urine RBC count and more severe proteinuria showed a higher abundance of Escherichia-Shigella. Furthermore, it was reported that Escherichia-Shigella was positively correlated with the urinary albumin-to-creatinine ratio and urine red blood cells account, but was negatively correlated with the estimated glomerular filtration rate (eGFR) (23, 33, 35). Interestingly, Zhao et al. (24) found that the abundance of Escherichia-Shigella in patients who received immunosuppressive therapy and achieved clinical remission returned to be similar to that in normal controls. Helicobacter pylori (H. pylori) infection is an important factor in gastric mucosal injury, and about 1% of patients with H. pylori infection will develop into gastric cancer (37). Liu et al. (38) found that the infection rate of type I H. pylori in IgAN patients was significantly higher than that in normal controls. Blood pressure, proteinuria and blood urea nitrogen levels in IgAN patients with H. pylori infection were significantly higher than those in uninfected patients, especially in patients with the infection of type I H. pylori (38). Moreover, IgAN patients with type I H. pylori infection had higher plasma Gd-IgA1 levels compared with uninfected patients (38). Bifidobacterium is considered to be the most important probiotic bacteria in humans (39). Tan et al. (19) found that the dysbiosis of gut microbiota in IgAN patients was characterized by a significant reduction in Bifidobacterium, which was negatively correlated to the level of proteinuria and hematuria. These indicated that the abundance of gut microbiota was related to the severity of IgAN. Additionally, some studies have also established random forest models based on gut microbiota to identify IgAN patients (22, 24, 25, 33). This suggested that gut microbiota has the potential to be a biomarker for the diagnosis of IgAN. Studies comparing the gut microbiota of IgAN patients with those of normal people are summarized in Table 2. The major intestinal dysbiosis in IgAN is depicted in Figure 1.
Figure 1. Gut microbiota dysbiosis and aberrant mucosal immune response in IgAN. Dysbiosis of gut microbiota in IgAN is manifested by a decrease in beneficial bacteria such as Bifidobacterium and an increase in harmful bacteria such as Escherichia-Shigella and Helicobacter pylori. Gut microbiota dysbiosis leads to intestinal microbial infection. Intestinal microbial infections boost the class switch of naive B lymphocytes to IgA antibody-secreting cells by T cell-independent and T cell-dependent pathways in GALT. In the T cell-dependent pathway, dendritic cells express TLR that recognizes microbial antigens and thus activating T cells, which promotes the differentiation of B cells into IgA+ B cells. In the T cell-independent pathway, microbial antigens can promote the release of BAFF and APRIL, and active TGF-β1, BAFF, and APRIL promote the preferential switching of TLR-activated B cells from IgM to IgA. IgA+ B cells differentiate into IgA+ plasma cells. Due to abnormal homing, lymphoplasmic cells producing mucosal IgA1 gradually migrate from mucosal lymphoid tissue to bone marrow, resulting in excessive production of mucosal IgA1 in the systemic circulation. These overproduced IgA are galactose-deficient IgA1, and the immune complex containing galactose-deficient IgA1 is deposited in the mesangium leading to glomerular damage. IECs, intestinal epithelial cells; DC, dendritic cell; TLR, Toll-like receptor; BAFF, B cell activating factor of the tumor necrosis factor family; APRIL, a proliferation-inducing ligand; TGF-β1, transforming growth factor β1; GALT, gut-associated lymphoid tissue; Gd-IgA1, galactose-deficient IgA1. Created with BioRender.com.
The aforementioned studies suggested that gut microbiota dysbiosis is present in patients with IgAN, but they are observational studies and therefore cannot establish a causal relationship between gut microbiota dysbiosis and IgAN. Recently, two Mendelian randomization studies both revealed the causal relationship between gut microbiota and IgAN (40, 41). However, there is a difference between two studies results. Ren et al. found (40) that Enterorhabdus is a protective factor for IgAN and Butyricicoccus is a risk factor for IgAN, while Wang et al. found (41) that Actinobacteria is associated with a high risk of IgAN, which may be due to differences in the way the two studies selected instrumental variables.
To sum up, IgAN patients showed gut microbiota dysbiosis, characterized by an increase in pathogenic bacteria and a decrease in beneficial bacteria. The dysbiosis of gut microbiota may be involved in the pathogenesis of IgAN. And the gut microbiota is expected to be a biomarker for the diagnosis of IgAN.
Normal gut microbiota plays an important role in maintaining the integrity of the intestinal mucosa. The destruction of the intestinal mucosal barrier is involved in the pathogenesis of IgAN (42). Increased intestinal permeability was found in both IgAN animal models and patients. Peng et al. (43) reported that IgAN rats had decreased intestinal epithelial tight junction protein expression and increased intestinal permeability. Serum levels of intestinal permeability markers were elevated in pseudosterile IgAN mice, which indicated that dysbiosis of gut microbiota might contribute to the disruption of intestinal mucosal barrier (25). Intestinal permeability was increased in mice induced by Lactobacillus casei cell wall extract and increased IgA in serum and IgA deposition in glomeruli were associated with intestinal permeability (44). Similarly, intestinal permeability was also found to be increased in IgAN patients. Tang et al. (27) also found that the indexes of intestinal mucosal barrier injury were higher in IgAN patients compared with normal subjects and positively related to the levels of Gd-IgA1 both in serum and urine. Furthermore, some genera of gut microbiota were correlated with intestinal mucosal barrier injury indexes (27). These suggest that intestinal mucosal destruction of IgAN may be related to dysbiosis of gut microbiota. In the case of damage to the intestinal mucosal barrier, toxic metabolites enter the blood circulation and produce an inflammatory response. P-cresol sulfate and indole sulfate can stimulate inflammation by promoting the release of pro-inflammatory cytokines, which is related to IgAN (45, 46).
Gut microbiota may also be involved in the development and progression of IgAN through altered metabolites. It was found that microbial metabolic pathways altered in IgAN, and the alteration of microbial metabolic pathways might be triggered by the alteration of gut microbiota (18). Wu et al. (20) reported that IgAN patients had different serum and fecal metabolites from normal people, and these metabolites had a correlation with gut microbiota. Hard-to-digest carbohydrates in food are fermented by anaerobic colonic bacteria to produce short-chain fatty acids (SCFAs), which mainly include acetate, propionate and butyrate (47). Chai et al. (31) found that levels of SCFAs including acetic acid, butyric acid, caproic acid, isobutyric acid, and propionic acid were significantly lower in IgAN patients compared with normal controls. And caproic acid was negatively correlated with 24-hour urinary protein levels (31). Interestingly, supplementation of IgAN mice with SCFAs reduced IgA renal deposition, improved mesangial hyperplasia and decreased urinary protein levels, which was achieved by the inhibition of NLRP3/ASC/Caspase 1 signaling pathway (19). In addition, Wu et al. (20) reported that the changes of gut microbiota in IgAN affected the metabolism and absorption of polyunsaturated fatty acids associated with gut microbiota. Long-chain n-3 polyunsaturated fatty acid in fish oil can inhibit the IgAN induced by trichothecene mycotoxin deoxynivalenol in mice (48). Dysbiosis of gut microbiota causes dysregulation of linoleic acid and arachidonic acid metabolism, reduction of prostaglandin derivatives, and imbalance between anti-inflammatory and pro-inflammatory fatty acids, which are associated with impaired intestinal mucosal barrier (49).
In addition to lipid metabolism disorders, there are also amino acid metabolism disorders in intestinal metabolism of IgAN patients. In amino acid metabolism disorder, aromatic tryptophan metabolism disorder is the most remarkable (49). The serum level of l-tryptophan is higher in IgAN patients, and it was positively correlated with blood urea nitrogen, serum creatinine and 24 total urinary protein, but negatively correlated with the eGFR (22). Interestingly, l-tryptophan is positively correlated with Eubacterium-coprostanoligenes (22). However, the gut-derived metabolite of tryptophan 3-indolepropionic acid significantly decreases in the gut and blood, which is believed to be related to decreased levels of intestinal Bacteroidetes (49). Furthermore, 3-indolepropionic acid is the primary ligand of the aryl hydrocarbon receptors (AhR) signaling and an important part of the intestinal mucosal immune barrier, promoting the renewal of the intestinal mucosal epithelium and maintaining the integrity of the intestinal mucosa (50). 3-indolepropionic acid was negatively correlated with Gd-IgA1 serum and positively correlated with eGFR (49). In addition, the serum B cell activating factor of the tumor-necrosis factor family (BAFF) levels were significantly higher in IgAN patients, and the five bacterial metabolites of 4-(1,1,3,3-tetramethylbutyl) phenol, p-tert-butyl-phenol, methyl neopentyl phthalic acid, hexadecyl ester benzoic acid and furanone A were positively correlated with serum BAFF levels (51).
In brief, the changes of metabolites caused by gut microbiota dysbiosis might be involved in the pathogenesis and progression of IgAN.
Excessive production of aberrantly glycosylated IgA1 is considered to be the causative factor of IgAN (52). It was found that the glomeruli of IgAN patients had abundant deposition of these aberrantly glycosylated IgA1, also called Gd-IgA1 (53). However, the source of Gd-IgA1 is not fully understood. Some studies showed that gut-associated lymphoid tissue (GALT) is involved in the production of Gd-IgA1 (54, 55). Under normal circumstances, gut microbiota, intestinal adaptive immune cells, and secretory immunoglobulin A coordinate with each other to maintain tolerance to symbiotic bacteria and defense against invading pathogens, thereby maintaining intestinal homeostasis (56). Alterations of the gut microbiota in IgAN may disrupt this homeostasis and lead to intestinal microbial infections. Toll-like receptors (TLRs), regarded as recognizers of pathogen-associated molecular patterns, play an important role in sensing microbial infection and participate in the pathogenesis of IgAN (54). Long-term and excessive activation of TLRs eventually leads to the overproduction of IgA1/Gd-IgA1 (57). Intestinal microbial infections boost the class switch of naive B lymphocytes to IgA antibody-secreting cells by T cell-independent and T cell-dependent pathways (58). BAFF and a proliferation-inducing ligand (APRIL), which are thought to be secreted by gut macrophage-dendritic cells and stromal cells, are involved in the T cell-independent pathway thereby promoting class switching of B cells from IgM to IgA (58). Due to abnormal homing, the lymphoplasmic cells producing mucosal IgA1 gradually migrate from mucosal lymphoid tissue to bone marrow, which leads to excessive production of mucosal-type IgA1 in the systemic circulation (59). Increased IgA1 complexes resulted in the shedding of IgA Fc receptor called CD89 from myeloid cell surfaces and integrated the soluble part of CD89 into complexes, which then deposits in kidney mesangium (60, 61).
The speculation that gut microbiota is involved in the aberrant intestinal mucosal immune response in IgAN is supported by the following studies. BAFF overexpressing transgenic mice exhibited IgAN manifestations including high circulating levels of abnormally glycosylated polymerized IgA and renal deposition of IgA exclusively in the presence of gut microbiota, and pathological manifestations of IgAN were not seen in germ-free mice (62, 63). In a specific pathogen-free environment, a1K1 mice had less mesangial IgA deposition than in conventional environments (64). Transplantation of gut microbiota from patients with progressive IgAN to humanized IgAN mice resulted in increased serum BAFF and Gd-IgA1 and decreased surface CD89 expression on blood CD11b+ cells which was related to mesangial deposition of soluble CD89 and IgA1 (65). Multiple intraperitoneal injections of Lactobacillus casei cell wall extract resulted in an increase in circulating IgA and IgA-IgG complexes and deposition of IgA in the glomerular mesangium in mice (44). Tang et al. (25) found that the levels of BAFF and APRIL were increased in the intestinal tissue of IgAN mouse model and revealed that dysbiosis of gut microbiota might contribute to local immune responses in IgAN. In addition, the abundance of Bifidobacterium in IgAN patients is lower than that in healthy controls (19, 26, 27). Bifidobacterium was found to have a negative correlation with serum Gd-IgA1 (26). Bifidobacterium was negatively correlated with urine Gd-IgA1 which had greater value in the diagnosis of IgAN (25, 27). The gut microbiota of IgAN patients was rich in Flavonifractor plautii, which can secrete α-galactosidase and α-N-acetyl-galactosaminidase that might be associated with the production of Gd-IgA1 (21). IgAN patients had an increased abundance of mucin-degrading bacteria including Akkermansia muciniphilan which can deglycosylate IgA1 and make it be recognized by autoreactive IgG in the serum of patients with IgAN (66). Mice expressing human IgA1 and human FC-α receptors developed an aggravated IgAN phenotype under intestinal colonization by Akkermansia muciniphilan, which indicated that gut microbiota dysbiosis contributes to the generation of auto-antigens in IgAN (66). Furthermore, mice colonized with gut microbiota from IgAN patients showed the IgAN phenotype with the activation of TLR4/MyD88/NF-κB pathway and B-cell stimulators (67). In peripheral blood mononuclear cells, lipopolysaccharide activated TLR4/MyD88/NF-κB pathway, B-cell stimulators, and proinflammatory cytokines and resulted in the overproduction of Gd-IgA1, which suggested gut dysbiosis can stimulate the overproduction of Gd-IgA1 through TLR4 signaling pathway (67). Similarly, Tang et al. (68) also revealed that gut dysbiosis mediated renal injury in IgAN by activating TLR4 signaling pathway. Interestingly, in recent years, Nefecon, a novel budesonide capsule designed to be released in the distal ileum, targeting to the mucosal immune system, has been found to delay the decline of eGFR and lastingly reduce proteinuria in IgAN patients in a phase III clinical trial (69).
In a word, gut microbiota dysbiosis leads to intestinal microbial infection, which in turn leads to aberrant immune responses causing the increase of Gd-IgA1 in the circulation, and the deposition of immune complexes containing Gd-IgA1 in the glomerular mesangium results in glomerular injury. The aberrant immune responses caused by the alterations in the gut microbiota are summarized in Figure 1.
Based on the possible involvement of gut microbiota in the pathogenesis of IgAN, more and more studies have attempted to treat IgAN by modulating gut microbiota. Fecal microbiota transplantation can modulate the composition of gut microbiota in IgAN mice. After receiving gut microbiota from healthy people, humanized IgAN mice exhibited decreased proteinuria, increased blood CD11b+ cell surface CD89 expression, and decreased expression of KC chemokine in the kidney, which is found to stimulate the proliferation of renal epithelial cells (65). Zhen Wu Tang, a classic Chinese herbal prescription, has been widely used to treat various kidney diseases in clinics in China for more than one thousand years for effectively relieving edema, dysuria, and oliguria symptoms (70, 71). A recent study found that treatment of Zhen Wu Tang made the gut microbiota of IgAN rats tend to approximate that of normal rats, modulated the metabolicphenotype perturbation, reversed morphological changes including glomerulus swelling, proliferation of mesangial cells and extension of mesangial matrix, decreased inflammation of intestinal mucosa and reduced kidney damage in IgAN rats (18). Probiotics have been used in the treatment of diarrhea, inflammatory bowel disease, irritable bowel syndrome and other diseases with certain efficacy and safety (72). Interestingly, probiotic supplementation has also been found to improve IgAN. Soylu et al. found (73) that Saccharomyces boulardii could prevent IgAN induced by oral poliovirus vaccine in mice, which may be achieved by downregulating systemic IgA response to the stimulation of enteral antigenic. Treatment of probiotics containing Bifidobacterium relieved gut microbiota dysbiosis in IgAN mice through increasing beneficial bacteria and decreasing potentially pathogenic bacteria and alleviated the IgAN manifestations in mouse models by blunting the NLRP3 activating signal (19). In addition, the treatment of antibiotics depleted the gut microbiota of IgAN mice and impaired GALT architecture, significantly preventing human immunoglobulin A1 deposition in mesangium, glomerular inflammation and proteinuria (74). Rifaximin is thought to promote the growth of probiotics, prevent intestinal inflammation, and regulate intestinal barrier function (75, 76). IgAN mice treated with antibiotic rifaximin showed decreased urinary protein, serum hIgA1-sCD89 and mIgG-hIgA1 complex levels, gut expression levels of BAFF, human IgA1 glomerular deposition and CD11b+ cell infiltration (77). Hydroxychloroquine treatment diminished abnormal glycosylation levels in IgAN through activating the C1GALT1/Cosmc pathway, ameliorated renal tissue pathological damage, and mitigated intestinal permeability and the dysbiosis of gut microbiota in IgAN rats (78). Gluten is a key protein found in wheat, barley and rye, and the gluten-free diet can affect the composition of human gut microbiota (79). Papista et al. (80) found that a gluten-free diet prevented the development of IgAN in α1KI-CD89Tg mice.
The treatment of modulating the gut microbiota has not only played a role in IgAN animal models but also achieved certain effects in IgAN patients. Zhao et al. (81) performed the first fecal microbiota transplantation to IgAN patients and found that feces from healthy people modulate the gut microbiota of two patients with refractory IgAN, resulting in decreased 24-hour urinary protein, elevated serum albumin, and stable renal function. After fecal microbiota transplantation, urinary protein had a significant decrease and even turned negative in one IgAN patient who refused to take immunosuppressive drugs (82).
Although the methods mentioned above for modulating the gut microbiota all seem to improve IgAN, long-term use of broad-spectrum antibiotics comes with inevitable side effects. Fecal microbiota transplantation appears to be the most powerful method for modulating the gut microbiota, and it has become an effective treatment for recurrent Clostridium difficile infections (83). However, the long-term effects of fecal microbiota transplantation are still unknown. Furthermore, treatment of IgAN by fecal microbiota transplantation is also performed only in individual populations currently.
In summary, gut microbiota is a potential target for the treatment of IgAN, and the treatments by modulating gut microbiota are expected to become a new therapy for IgAN. Current therapeutic approaches to try to treat IgAN by modulating the gut microbiota are shown in Figure 2.
Figure 2. Modulation of gut microbiota treats IgAN animal models and patients. Created with BioRender.com.
Gut microbiota dysbiosis is present in IgAN, which is characterized by a decrease in beneficial bacteria and an increase in potentially pathogenic bacteria. Gut microbiota may be involved in the development and progression of IgAN through the destruction of intestinal barrier, altered metabolites and aberrant mucosal immune responses. Gut microbiota may be a potential biomarker for the diagnosis of IgAN and a potential target for the treatment of IgAN. Therapies through modulating gut microbiota have effects on the improvement of IgAN. However, attempts to treat IgAN by modulating gut microbiota have mostly only been implemented in animal models now. Large-scale clinical trials are needed to verify the efficacy and safety of methods for modulating gut microbiota in the treatment of IgAN.
KY: Data curation, Investigation, Writing – original draft. LZ: Investigation, Validation, Writing – review & editing. WC: Investigation, Validation, Writing – review & editing. YX: Validation, Writing – review & editing. CL: Validation, Writing – review & editing. TZ: Conceptualization, Validation, Visualization, Writing – review & editing.
The author(s) declare that financial support was received for the research, authorship, and/or publication of this article. This review was supported by Guangdong Province Science and Technology Special Fund.
Figures are created with BioRender.com.
The authors declare that the research was conducted in the absence of any commercial or financial relationships that could be construed as a potential conflict of interest.
All claims expressed in this article are solely those of the authors and do not necessarily represent those of their affiliated organizations, or those of the publisher, the editors and the reviewers. Any product that may be evaluated in this article, or claim that may be made by its manufacturer, is not guaranteed or endorsed by the publisher.
IgAN, Immunoglobulin A nephropathy; CKD, chronic kidney disease; Gd-IgA1, galactose-deficient IgA1; ESRD, end-stage renal disease; SCFAs, short-chain fatty acids; eGFR, estimated glomerular filtration rate; AhR, aryl hydrocarbon receptors; GALT, gut-associated lymphoid tissue; TLRs, Toll-like receptors; BAFF, B cell activating factor of the tumor-necrosis factor family; APRIL, a proliferation-inducing ligand.
1. Stamellou E, Seikrit C, Tang SCW, Boor P, Tesař V, Floege J, et al. IgA nephropathy. Nat Rev Dis primers. (2023) 9:67. doi: 10.1038/s41572-023-00476-9
2. McGrogan A, Franssen CF, de Vries CS. The incidence of primary glomerulonephritis worldwide: a systematic review of the literature. Nephrology dialysis transplantation: Off Publ Eur Dialysis Transplant Assoc - Eur Renal Assoc. (2011) 26:414–30. doi: 10.1093/ndt/gfq665
3. Xie Y, Chen X. Epidemiology, major outcomes, risk factors, prevention and management of chronic kidney disease in China. Am J nephrology. (2008) 28:1–7. doi: 10.1159/000108755
4. Rodrigues JC, Haas M, Reich HN. IgA nephropathy. Clin J Am Soc Nephrology: CJASN. (2017) 12:677–86. doi: 10.2215/CJN.07420716
5. Suzuki H, Kiryluk K, Novak J, Moldoveanu Z, Herr AB, Renfrow MB, et al. The pathophysiology of IgA nephropathy. J Am Soc Nephrology: JASN. (2011) 22:1795–803. doi: 10.1681/ASN.2011050464
6. Lai KN, Tang SC, Schena FP, Novak J, Tomino Y, Fogo AB, et al. IgA nephropathy. Nat Rev Dis primers. (2016) 2:16001. doi: 10.1038/nrdp.2016.1
7. Floege J, Rauen T, Tang SCW. Current treatment of IgA nephropathy. Semin immunopathology. (2021) 43:717–28. doi: 10.1007/s00281-021-00888-3
8. Rovin BH, Adler SG, Barratt J, Bridoux F, Burdge KA, Chan TM, et al. Executive summary of the KDIGO 2021 guideline for the management of glomerular diseases. Kidney Int. (2021) 100:753–79. doi: 10.1016/j.kint.2021.05.015
9. Coppo R. Corticosteroids in igA nephropathy: lessons from recent studies. J Am Soc Nephrology: JASN. (2017) 28:25–33. doi: 10.1681/ASN.2016060647
10. Gareau MG, Sherman PM, Walker WA. Probiotics and the gut microbiota in intestinal health and disease. Nat Rev Gastroenterol hepatology. (2010) 7:503–14. doi: 10.1038/nrgastro.2010.117
11. Hooper LV, Macpherson AJ. Immune adaptations that maintain homeostasis with the intestinal microbiota. Nat Rev Immunol. (2010) 10:159–69. doi: 10.1038/nri2710
12. Jandhyala SM, Talukdar R, Subramanyam C, Vuyyuru H, Sasikala M, Nageshwar Reddy D. Role of the normal gut microbiota. World J gastroenterology. (2015) 21:8787–803. doi: 10.3748/wjg.v21.i29.8787
13. Chen YY, Chen DQ, Chen L, Liu JR, Vaziri ND, Guo Y, et al. Microbiome-metabolome reveals the contribution of gut-kidney axis on kidney disease. J Trans Med. (2019) 17:5. doi: 10.1186/s12967-018-1756-4
14. Evenepoel P, Poesen R, Meijers B. The gut-kidney axis. Pediatr Nephrol (Berlin Germany). (2017) 32:2005–14. doi: 10.1007/s00467-016-3527-x
15. Luvizotto MJ, Menezes-Silva L, Woronik V, Monteiro RC, Câmara NOS. Gut-kidney axis in IgA nephropathy: Role on mesangial cell metabolism and inflammation. Front Cell Dev Biol. (2022) 10:993716. doi: 10.3389/fcell.2022.993716
16. Haniuda K, Gommerman JL, Reich HN. The microbiome and IgA nephropathy. Semin immunopathology. (2021) 43:649–56. doi: 10.1007/s00281-021-00893-6
17. Wang Y, Tian L, Sun L, Zhou W, Zhi W, Qing J, et al. Gut microbes in immunoglobulin A nephropathy and their potential therapeutic applications. Front Med. (2022) 9:823267. doi: 10.3389/fmed.2022.823267
18. Li J, Cao Y, Lu R, Li H, Pang Y, Fu H, et al. Integrated fecal microbiome and serum metabolomics analysis reveals abnormal changes in rats with immunoglobulin A nephropathy and the intervention effect of zhen wu tang. Front Pharmacol. (2020) 11:606689. doi: 10.3389/fphar.2020.606689
19. Tan J, Dong L, Jiang Z, Tan L, Luo X, Pei G, et al. Probiotics ameliorate IgA nephropathy by improving gut dysbiosis and blunting NLRP3 signaling. J Trans Med. (2022) 20:382. doi: 10.1186/s12967-022-03585-3
20. Wu H, Tang D, Zheng F, Li S, Zhang X, Yin L, et al. Identification of a novel interplay between intestinal bacteria and metabolites in Chinese patients with IgA nephropathy via integrated microbiome and metabolome approaches. Ann Trans Med. (2021) 9:32. doi: 10.21037/atm-20-2506
21. Liang X, Zhang S, Zhang D, Hu L, Zhang L, Peng Y, et al. Metagenomics-based systematic analysis reveals that gut microbiota Gd-IgA1-associated enzymes may play a key role in IgA nephropathy. Front Mol biosciences. (2022) 9:970723. doi: 10.3389/fmolb.2022.970723
22. Dong Y, Chen J, Zhang Y, Wang Z, Shang J, Zhao Z. Development and validation of diagnostic models for immunoglobulin A nephropathy based on gut microbes. Front Cell infection Microbiol. (2022) 12:1059692. doi: 10.3389/fcimb.2022.1059692
23. Bao WH, Tang W. Changes of gut microflora in newly diagnosed IgA nephropathy patients and its correlation with clinical risk factors. Beijing da xue xue bao Yi xue ban = J Peking Univ Health Sci. (2023) 55:124–32. doi: 10.19723/j.issn.1671-167X.2023.01.019
24. Zhao J, Bai M, Ning X, Qin Y, Wang Y, Yu Z, et al. Expansion of escherichia-shigella in gut is associated with the onset and response to immunosuppressive therapy of igA nephropathy. J Am Soc Nephrology: JASN. (2022) 33:2276–92. doi: 10.1681/ASN.2022020189
25. Tang Y, Xiao Y, He H, Zhu Y, Sun W, Hu P, et al. Aberrant gut microbiome contributes to barrier dysfunction, inflammation, and local immune responses in igA nephropathy. Kidney Blood Pressure Res. (2023) 48:261–76. doi: 10.1159/000528973
26. Zhong Z, Tan J, Tan L, Tang Y, Qiu Z, Pei G, et al. Modifications of gut microbiota are associated with the severity of IgA nephropathy in the Chinese population. Int Immunopharmacol. (2020) 89:107085. doi: 10.1016/j.intimp.2020.107085
27. Tang Y, Zhu Y, He H, Peng Y, Hu P, Wu J, et al. Gut dysbiosis and intestinal barrier dysfunction promotes igA nephropathy by increasing the production of gd-igA1. Front Med. (2022) 9:944027. doi: 10.3389/fmed.2022.944027
28. Sugurmar ANK, Mohd R, Shah SA, Neoh HM, Cader RA. Gut microbiota in immunoglobulin A nephropathy: a Malaysian perspective. BMC nephrology. (2021) 22:145. doi: 10.1186/s12882-021-02315-z
29. De Angelis M, Montemurno E, Piccolo M, Vannini L, Lauriero G, Maranzano V, et al. Microbiota and metabolome associated with immunoglobulin A nephropathy (IgAN). PloS One. (2014) 9:e99006. doi: 10.1371/journal.pone.0099006
30. He JW, Zhou XJ, Li YF, Wang YN, Liu LJ, Shi SF, et al. Associations of genetic variants contributing to gut microbiota composition in immunoglobin A nephropathy. mSystems. (2021) 6:e00819-20. doi: 10.1128/mSystems.00819-20
31. Chai L, Luo Q, Cai K, Wang K, Xu B. Reduced fecal short-chain fatty acids levels and the relationship with gut microbiota in IgA nephropathy. BMC nephrology. (2021) 22:209. doi: 10.1186/s12882-021-02414-x
32. Zhang P, Fang J, Li G, Zhang L, Lai X, Xu L, et al. Sex differences in fecal microbiota correlation with physiological and biochemical indices associated with end-stage renal disease caused by immunoglobulin a nephropathy or diabetes. Front Microbiol. (2021) 12:752393. doi: 10.3389/fmicb.2021.752393
33. Hu X, Du J, Xie Y, Huang Q, Xiao Y, Chen J, et al. Fecal microbiota characteristics of Chinese patients with primary IgA nephropathy: a cross-sectional study. BMC nephrology. (2020) 21:97. doi: 10.1186/s12882-020-01741-9
34. Dong R, Bai M, Zhao J, Wang D, Ning X, Sun S. A comparative study of the gut microbiota associated with immunoglobulin a nephropathy and membranous nephropathy. Front Cell infection Microbiol. (2020) 10:557368. doi: 10.3389/fcimb.2020.557368
35. Shah NB, Nigwekar SU, Kalim S, Lelouvier B, Servant F, Dalal M, et al. The gut and blood microbiome in igA nephropathy and healthy controls. Kidney360. (2021) 2:1261–74. doi: 10.34067/KID.0000132021
36. Hu X, Fan R, Song W, Qing J, Yan X, Li Y, et al. Landscape of intestinal microbiota in patients with IgA nephropathy, IgA vasculitis and Kawasaki disease. Front Cell infection Microbiol. (2022) 12:1061629. doi: 10.3389/fcimb.2022.1061629
37. de Vries AC, van Grieken NC, Looman CW, Casparie MK, de Vries E, Meijer GA, et al. Gastric cancer risk in patients with premalignant gastric lesions: a nationwide cohort study in the Netherlands. Gastroenterology. (2008) 134:945–52. doi: 10.1053/j.gastro.2008.01.071
38. Liu XZ, Zhang YM, Jia NY, Zhang H. Helicobacter pylori infection is associated with elevated galactose-deficient IgA1 in IgA nephropathy. Renal failure. (2020) 42:539–46. doi: 10.1080/0886022X.2020.1772295
39. Amalaradjou MA, Bhunia AK. Modern approaches in probiotics research to control foodborne pathogens. Adv Food Nutr Res. (2012) 67:185–239. doi: 10.1016/B978-0-12-394598-3.00005-8
40. Ren F, Jin Q, Liu T, Ren X, Zhan Y. Causal effects between gut microbiota and IgA nephropathy: a bidirectional Mendelian randomization study. Front Cell infection Microbiol. (2023) 13:1171517. doi: 10.3389/fcimb.2023.1171517
41. Wang F, Li N, Ni S, Min Y, Wei K, Sun H, et al. The effects of specific gut microbiota and metabolites on igA nephropathy-based on mendelian randomization and clinical validation. Nutrients. (2023) 15:2407. doi: 10.3390/nu15102407
42. Kiryluk K, Li Y, Scolari F, Sanna-Cherchi S, Choi M, Verbitsky M, et al. Discovery of new risk loci for IgA nephropathy implicates genes involved in immunity against intestinal pathogens. Nat Genet. (2014) 46:1187–96. doi: 10.1038/ng.3118
43. Peng SN, Zeng HH, Fu AX, Chen XW, Zhu QX. Effects of rhein on intestinal epithelial tight junction in IgA nephropathy. World J gastroenterology. (2013) 19:4137–45. doi: 10.3748/wjg.v19.i26.4137
44. Wan F, Wang H, Wang M, Lv J, Zhao M, Zhang H. Sustained release of Lactobacillus casei cell wall extract can induce a continuous and stable IgA deposition model. J pathology. (2022) 257:262–73. doi: 10.1002/path.v257.3
45. Tang WH, Wang Z, Kennedy DJ, Wu Y, Buffa JA, Agatisa-Boyle B, et al. Gut microbiota-dependent trimethylamine N-oxide (TMAO) pathway contributes to both development of renal insufficiency and mortality risk in chronic kidney disease. Circ Res. (2015) 116:448–55. doi: 10.1161/CIRCRESAHA.116.305360
46. Yacoub R, Wyatt CM. Manipulating the gut microbiome to decrease uremic toxins. Kidney Int. (2017) 91:521–3. doi: 10.1016/j.kint.2017.01.003
47. Cummings JH, Macfarlane GT. The control and consequences of bacterial fermentation in the human colon. J Appl bacteriology. (1991) 70:443–59. doi: 10.1111/j.1365-2672.1991.tb02739.x
48. Shi Y, Pestka JJ. Mechanisms for suppression of interleukin-6 expression in peritoneal macrophages from docosahexaenoic acid-fed mice. J Nutr Biochem. (2009) 20:358–68. doi: 10.1016/j.jnutbio.2008.04.006
49. Wu H, Tang D, Yun M, Liu H, Huang S, Yun C, et al. Metabolic dysfunctions of intestinal fatty acids and tryptophan reveal immuno-inflammatory response activation in igA nephropathy. Front Med. (2022) 9:811526. doi: 10.3389/fmed.2022.811526
50. Sun M, Ma N, He T, Johnston LJ, Ma X. Tryptophan (Trp) modulates gut homeostasis via aryl hydrocarbon receptor (AhR). Crit Rev Food Sci Nutr. (2020) 60:1760–8. doi: 10.1080/10408398.2019.1598334
51. Sallustio F, Curci C, Chaoul N, Fontò G, Lauriero G, Picerno A, et al. High levels of gut-homing immunoglobulin A+ B lymphocytes support the pathogenic role of intestinal mucosal hyperresponsiveness in immunoglobulin A nephropathy patients. Nephrology dialysis transplantation: Off Publ Eur Dialysis Transplant Assoc - Eur Renal Assoc. (2021) 36:452–64. doi: 10.1093/ndt/gfaa264
52. Wyatt RJ, Julian BA. IgA nephropathy. New Engl J Med. (2013) 368:2402–14. doi: 10.1056/NEJMra1206793
53. Yasutake J, Suzuki Y, Suzuki H, Hiura N, Yanagawa H, Makita Y, et al. Novel lectin-independent approach to detect galactose-deficient IgA1 in IgA nephropathy. Nephrology dialysis transplantation: Off Publ Eur Dialysis Transplant Assoc - Eur Renal Assoc. (2015) 30:1315–21. doi: 10.1093/ndt/gfv221
54. Gesualdo L, Di Leo V, Coppo R. The mucosal immune system and IgA nephropathy. Semin immunopathology. (2021) 43:657–68. doi: 10.1007/s00281-021-00871-y
55. Barratt J, Rovin BH, Cattran D, Floege J, Lafayette R, Tesar V, et al. Why target the gut to treat igA nephropathy? Kidney Int Rep. (2020) 5:1620–4. doi: 10.1016/j.ekir.2020.08.009
56. Wang L, Zhu L, Qin S. Gut microbiota modulation on intestinal mucosal adaptive immunity. J Immunol Res. (2019) 2019:4735040. doi: 10.1155/2019/4735040
57. Rollino C, Vischini G, Coppo R. IgA nephropathy and infections. J nephrology. (2016) 29:463–8. doi: 10.1007/s40620-016-0265-x
58. Fagarasan S, Kawamoto S, Kanagawa O, Suzuki K. Adaptive immune regulation in the gut: T cell-dependent and T cell-independent IgA synthesis. Annu Rev Immunol. (2010) 28:243–73. doi: 10.1146/annurev-immunol-030409-101314
59. Al Hussain T, Hussein MH, Al Mana H, Akhtar M. Pathophysiology of igA nephropathy. Adv anatomic pathology. (2017) 24:56–62. doi: 10.1097/PAP.0000000000000134
60. Launay P, Grossetête B, Arcos-Fajardo M, Gaudin E, Torres SP, Beaudoin L, et al. Fcalpha receptor (CD89) mediates the development of immunoglobulin A (IgA) nephropathy (Berger’s disease). Evidence pathogenic soluble receptor-Iga complexes patients CD89 Transgenic mice. J Exp Med. (2000) 191:1999–2009. doi: 10.1084/jem.191.11.1999
61. Berthelot L, Papista C, Maciel TT, Biarnes-Pelicot M, Tissandie E, Wang PH, et al. Transglutaminase is essential for IgA nephropathy development acting through IgA receptors. J Exp Med. (2012) 209:793–806. doi: 10.1084/jem.20112005
62. McCarthy DD, Chiu S, Gao Y, Summers-deLuca LE, Gommerman JL. BAFF induces a hyper-IgA syndrome in the intestinal lamina propria concomitant with IgA deposition in the kidney independent of LIGHT. Cell Immunol. (2006) 241:85–94. doi: 10.1016/j.cellimm.2006.08.002
63. McCarthy DD, Kujawa J, Wilson C, Papandile A, Poreci U, Porfilio EA, et al. Mice overexpressing BAFF develop a commensal flora-dependent, IgA-associated nephropathy. J Clin Invest. (2011) 121:3991–4002. doi: 10.1172/JCI45563
64. Oruc Z, Oblet C, Boumediene A, Druilhe A, Pascal V, Le Rumeur E, et al. IgA structure variations associate with immune stimulations and igA mesangial deposition. J Am Soc Nephrology: JASN. (2016) 27:2748–61. doi: 10.1681/ASN.2015080911
65. Lauriero G, Abbad L, Vacca M, Celano G, Chemouny JM, Calasso M, et al. Fecal microbiota transplantation modulates renal phenotype in the humanized mouse model of igA nephropathy. Front Immunol. (2021) 12:694787. doi: 10.3389/fimmu.2021.694787
66. Gleeson PJ, Benech N, Chemouny J, Metallinou E, Berthelot L, da Silva J, et al. The gut microbiota posttranslationally modifies IgA1 in autoimmune glomerulonephritis. Sci Trans Med. (2024) 16:eadl6149. doi: 10.1126/scitranslmed.adl6149
67. Zhu Y, He H, Sun W, Wu J, Xiao Y, Peng Y, et al. IgA nephropathy: gut microbiome regulates the production of hypoglycosilated IgA1via the TLR4 signaling pathway. Nephrology dialysis transplantation: Off Publ Eur Dialysis Transplant Assoc - Eur Renal Assoc. (2024) 39:1624–41. doi: 10.1093/ndt/gfae052
68. Tang Y, Sun W, He H, Hu P, Jin M, Liu P, et al. Activation of intestinal mucosal TLR4/NF-κB pathway is associated with renal damage in mice with pseudo-sterile IgA nephropathy. Xi bao yu fen zi mian yi xue za zhi = Chin J Cell Mol Immunol. (2023) 39:865–71.
69. Lafayette R, Kristensen J, Stone A, Floege J, Tesař V, Trimarchi H, et al. Efficacy and safety of a targeted-release formulation of budesonide in patients with primary IgA nephropathy (NefIgArd): 2-year results from a randomised phase 3 trial. Lancet (London England). (2023) 402:859–70. doi: 10.1016/S0140-6736(23)01554-4
70. Liu Q, Hu S, He Y, Zhang J, Zeng X, Gong F, et al. The protective effects of Zhen-Wu-Tang against cisplatin-induced acute kidney injury in rats. PloS One. (2017) 12:e0179137. doi: 10.1371/journal.pone.0179137
71. La L, Wang L, Qin F, Jiang J, He S, Wang C, et al. Zhen-wu-tang ameliorates adenine-induced chronic renal failure in rats: regulation of the canonical Wnt4/beta-catenin signaling in the kidneys. J ethnopharmacology. (2018) 219:81–90. doi: 10.1016/j.jep.2017.12.013
72. Suez J, Zmora N, Segal E, Elinav E. The pros, cons, and many unknowns of probiotics. Nat Med. (2019) 25:716–29. doi: 10.1038/s41591-019-0439-x
73. Soylu A, Berktaş S, Sarioğlu S, Erbil G, Yilmaz O, Demir BK, et al. Saccharomyces boulardii prevents oral-poliovirus vaccine-induced IgA nephropathy in mice. Pediatr Nephrol (Berlin Germany). (2008) 23:1287–91. doi: 10.1007/s00467-008-0813-2
74. Chemouny JM, Gleeson PJ, Abbad L, Lauriero G, Boedec E, Le Roux K, et al. Modulation of the microbiota by oral antibiotics treats immunoglobulin A nephropathy in humanized mice. Nephrology dialysis transplantation: Off Publ Eur Dialysis Transplant Assoc - Eur Renal Assoc. (2019) 34:1135–44. doi: 10.1093/ndt/gfy323
75. Lopetuso LR, Napoli M, Rizzatti G, Gasbarrini A. The intriguing role of Rifaximin in gut barrier chronic inflammation and in the treatment of Crohn’s disease. Expert Opin investigational Drugs. (2018) 27:543–51. doi: 10.1080/13543784.2018.1483333
76. Yang L, Liu B, Zheng J, Huang J, Zhao Q, Liu J, et al. Rifaximin alters intestinal microbiota and prevents progression of ankylosing spondylitis in mice. Front Cell infection Microbiol. (2019) 9:44. doi: 10.3389/fcimb.2019.00044
77. Di Leo V, Gleeson PJ, Sallustio F, Bounaix C, Da Silva J, Loreto G, et al. Rifaximin as a potential treatment for igA nephropathy in a humanized mice model. J personalized Med. (2021) 11:309. doi: 10.3390/jpm11040309
78. Wang C, Cai X, Lin S, Lin Y. Hydroxychloroquine ameliorates immune functionality and intestinal flora disorders of IgA nephropathy by inhibition of C1GALT1/Cosmc pathway. Immunopharmacol immunotoxicology. (2024) 46:218–28. doi: 10.1080/08923973.2023.2300306
79. De Palma G, Nadal I, Collado MC, Sanz Y. Effects of a gluten-free diet on gut microbiota and immune function in healthy adult human subjects. Br J Nutr. (2009) 102:1154–60. doi: 10.1017/S0007114509371767
80. Papista C, Lechner S, Ben Mkaddem S, LeStang MB, Abbad L, Bex-Coudrat J, et al. Gluten exacerbates IgA nephropathy in humanized mice through gliadin-CD89 interaction. Kidney Int. (2015) 88:276–85. doi: 10.1038/ki.2015.94
81. Zhao J, Bai M, Yang X, Wang Y, Li R, Sun S. Alleviation of refractory IgA nephropathy by intensive fecal microbiota transplantation: the first case reports. Renal failure. (2021) 43:928–33. doi: 10.1080/0886022X.2021.1936038
82. Zhi W, Song W, Abdi Saed Y, Wang Y, Li Y. Fecal capsule as a therapeutic strategy in igA nephropathy: A brief report. Front Med. (2022) 9:914250. doi: 10.3389/fmed.2022.914250
Keywords: immunoglobulin A nephropathy, gut microbiota, dysbiosis, immune responses, modulate
Citation: Yao K, Zheng L, Chen W, Xie Y, Liao C and Zhou T (2025) Characteristics, pathogenic and therapeutic role of gut microbiota in immunoglobulin A nephropathy. Front. Immunol. 16:1438683. doi: 10.3389/fimmu.2025.1438683
Received: 26 May 2024; Accepted: 21 January 2025;
Published: 06 February 2025.
Edited by:
Marijn M. Speeckaert, Ghent University Hospital, BelgiumReviewed by:
Laureline Berthelot, INSERM U1064 Centre de Recherche en Transplantation et Immunologie, FranceCopyright © 2025 Yao, Zheng, Chen, Xie, Liao and Zhou. This is an open-access article distributed under the terms of the Creative Commons Attribution License (CC BY). The use, distribution or reproduction in other forums is permitted, provided the original author(s) and the copyright owner(s) are credited and that the original publication in this journal is cited, in accordance with accepted academic practice. No use, distribution or reproduction is permitted which does not comply with these terms.
*Correspondence: Tianbiao Zhou, emhvdXRiQGFsaXl1bi5jb20=
Disclaimer: All claims expressed in this article are solely those of the authors and do not necessarily represent those of their affiliated organizations, or those of the publisher, the editors and the reviewers. Any product that may be evaluated in this article or claim that may be made by its manufacturer is not guaranteed or endorsed by the publisher.
Research integrity at Frontiers
Learn more about the work of our research integrity team to safeguard the quality of each article we publish.