- 1School of Traditional Chinese Medicine, Hunan University of Chinese Medicine, Changsha, China
- 2Hunan Key Laboratory of Traditional Chinese Medicine Prescription and Syndromes Translational Medicine, Hunan University of Chinese Medicine, Changsha, China
Background: Metformin, the frontline treatment for diabetes, has considerable potential as an immunomodulator; however, detailed bibliometric analyses on this subject are limited.
Methods: This study extracted 640 relevant articles from the Web of Science (WOS) Core Collection and conducted visual analyses using Microsoft Excel, VOSviewer, and CiteSpace.
Results: The findings showed that research on the immunomodulatory function of metformin has grown steadily since 2017, with China and the United States being the leading contributors. These studies have mostly been published in journals such as the International Journal of Molecular Sciences, Cancers, Frontiers in Immunology, and Scientific Reports. Keyword co-occurrence analysis highlighted metformin’s role as an immunomodulator, particularly in the context of the tumor immune microenvironment, immunosuppressive checkpoints, and metformin derivatives. Recent research has highlighted metformin’s application in aging, autoimmune diseases, COVID-19, and tuberculosis. Additionally, its role in regulating inflammation and gut microbiota is also being investigated.
Conclusion: Overall, the immunomodulatory effects of metformin were investigated in anti-tumor, antiviral, anti-aging, and autoimmune disease research. This highlights the scope of metformin use in these fields, while also significantly enhancing its clinical value as a repurposed drug.
1 Introduction
Metformin, a guanidine derivative of herbal goat bean, has long been the primary oral hypoglycemic agent used to treat type 2 diabetes (T2DM). It is recognized for its high-efficiency hypoglycemic and cardiovascular protective effects, without increasing body weight or the risk of hypoglycemia (1). This drug has garnered significant attention due to its extensive clinical application and potential health benefits. Preclinical and observational studies have demonstrated the promising potential of metformin in treating conditions such as obesity, metabolic syndrome, osteoporosis, rheumatoid arthritis, aging, periodontitis, cancer, liver disease, kidney disease, inflammatory bowel disease, tuberculosis, coronavirus disease 2019 (COVID-19), osteoarthritis, and other autoimmune inflammatory rheumatic diseases (2–4). However, the specific mechanisms and clinical efficacy of these effects requires further investigation.
Over the past decade, research has displayed that in addition to its hypoglycemic effects, metformin can regulate cell energy metabolism, proliferation, growth, inflammation, endoplasmic reticulum stress (ERS), and autophagy, as well as improve intestinal flora (5–8). Mounting evidence shows that metformin is an effective immune system activator (9). It not only regulates the host immune function but also demonstrates an enhanced therapeutic efficacy in treating the aforementioned diseases and overcomes immunotherapy resistance, although the molecular mechanisms of these effects are not completely understood (10, 11). The unexpected immunomodulatory benefits of metformin were initially identified by Pearceet al. (12), who demonstrated that metformin enhances the generation and persistence of memory CD8+ T cells by activating fatty acid oxidation in T cells, revealing a novel mechanism for improving immune function. This finding was later supported by Finisguerra et al. (13). Many experimental and clinical studies have demonstrated that metformin can regulate tumor-infiltrating effector immune cells and inhibit immunosuppressive cells in various tumor models of breast, liver, lung, head and neck, and colorectal cancer (14–18). Metformin also exhibits an anti-tumor effect by regulating programmed death-1(PD-1)/programmed death-ligand 1(PD-L1) immunosuppressive checkpoints, which may involve ERS and adenosine monophosphate-activated protein kinase (AMPK) pathways (19, 20). Other studies have shown that metformin prevents age-related ovarian fibrosis by balancing the number of fibroblasts, myofibroblasts and immune cells (21). Furthermore, metformin exerts its anti-inflammatory and immunosuppressive effects by regulating the macrophage expression of the plasticity factor, zinc finger e-box binding homeobox 1(ZEB1) (22). However, in cases of obesity (23), atherosclerosis (24), and bone-related lesions (25), metformin induces M2 polarization. Rodriguez’s team found that supformin, a metformin dimer, disrupts the plasticity of human immune cells, dendritic cells (DCs) and macrophages. Thus, opening avenues for the development of innovative treatments (26). Given its considerable potential, metformin as an immunomodulator is increasingly featured in scientific publications. However, an integration of data and visual analysis is essential to better understand the developments in this domain.
This bibliometric study focuses on the direct relationship between metformin and its immunomodulatory effects. Bibliometric analysis is widely used to quantitatively evaluate published studies and predict future trends. This type of research is based on existing literature involving different countries, institutions, authors, journals, and keywords. It uses mathematical and statistical tools to quantify and predict the status quo of scientific research to objectively evaluate the knowledge framework and identify research hotspots. Recently, the number of research publications in this field has increased rapidly. Nonetheless, bibliometric analyses of metformin as an immunomodulator remain limited. This study evaluated published research on metformin’s role as an immunomodulator over the past decade and aimed to provide new insights for academic dynamics, drug development, and disease treatment, offering a broad perspective and roadmap for future research.
2 Materials and methods
2.1 Search strategy
This study collected research on metformin and immune regulation published from January 1, 2013, to October 1, 2024, from the WOS core collection database. Data collection was completed on October 8, 2024. The search utilized the following keywords ((TS=(Metformin OR Dimethylbiguanidine OR Dimethylguanylguanidine OR Glucophage)) AND (TS=(Immunity OR Immunization OR Immunological OR Immune))).
2.2 Data collection
To ensure the authenticity and reliability of the study, two researchers collaborated closely in retrieving and thoroughly screening the data. By excluding irrelevant literature individually, a total of 640 papers were analyzed. The detailed data screening process is illustrated in Figure 1. All retrieved literature that met the established criteria were used in the bibliometric analysis. The complete records and reference lists were extracted and saved as “txt” files, which served as the data source for the analysis.
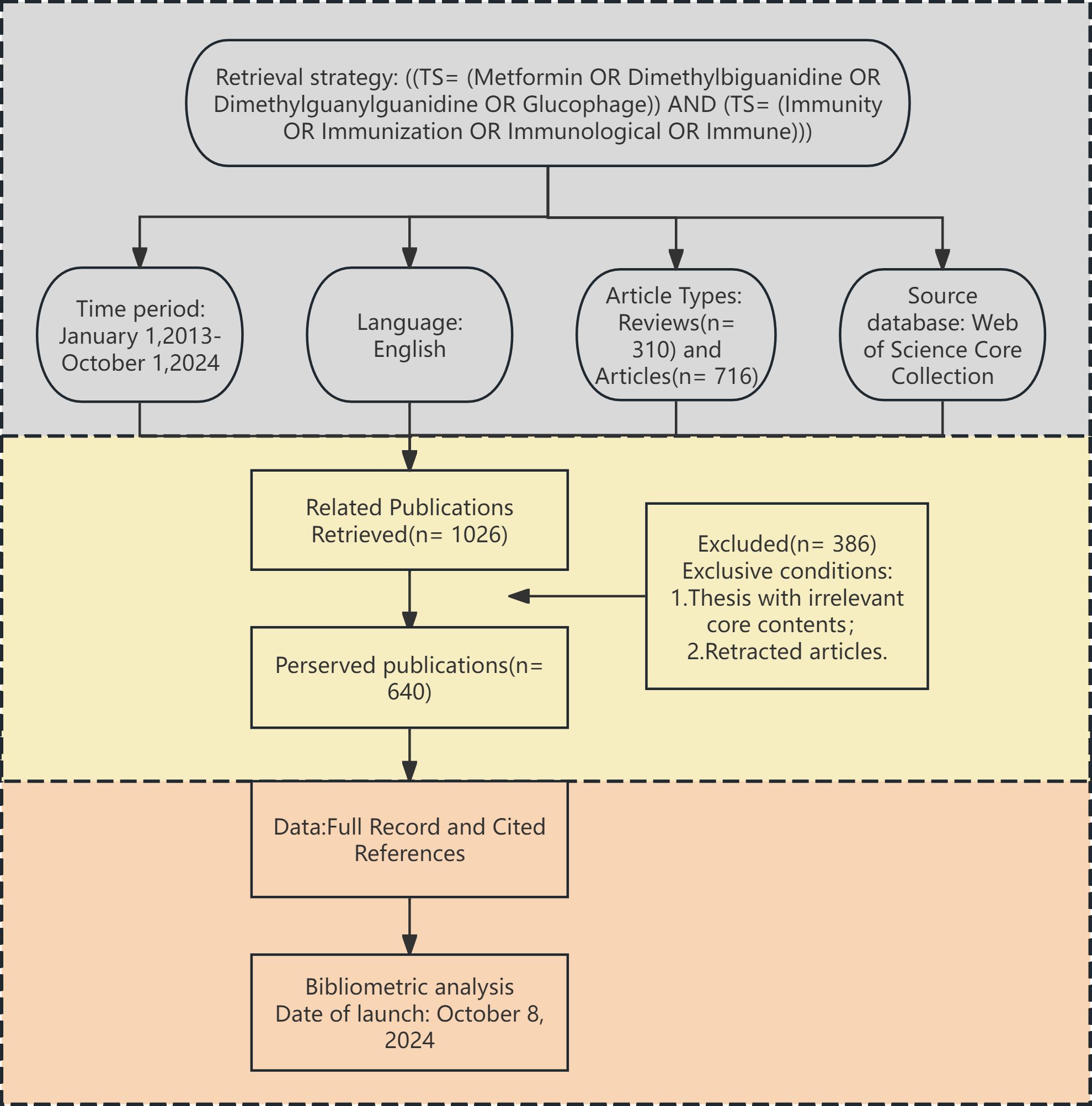
Figure 1. Flow diagram presenting the selection process for studies on metformin as an immunomodulator. This diagram depicts the screening of 640 publications published between January 2013 and October 2024.
2.3 Data analysis and visualization
We used CiteSpace (version 6.4.R1), VOSviewer (version 1.6.20), and Microsoft Excel 365 for analyzing and presenting data. VOSviewer (27) was used to extract detailed information of countries, institutions, journals, authors, citations, and keywords, as well as to construct a network visualization map. Each node in VOSviewer represented an entity, and its size was related to its weight. The thickness of the connection between the nodes was closely related to the strength of cooperation, co-citation and co-occurrence. We used CiteSpace (version 6.4.R1) (28) to calculate the burst of references, timeline of keywords, and burst of keywords to visualize the data. Microsoft Excel 365 was utilized to visualize the annual count of literature, countries, institutions, authors, journals, and the most cited local references.
3 Results
3.1 Quantitative analysis of publications output
This study retrieved and reviewed effective literature from 2013 to 2024 in the WOS core database, screening 640 papers from 4,354 authors across 77 countries and 1,246 institutions. Figure 2 demonstrates a steady increase in the number of articles related to metformin and immune regulation over time. This trend indicated that researchers paid little attention to the immunomodulatory mechanism of metformin between 2013 and 2016, with fewer than 20 articles published annually. As the potential therapeutic value of metformin’s immune regulation was better understood, there was a rapid increase on its research over the following eight years. By 2021, the annual number of publications exceeded 100, which was nearly ten times the previous number. This demonstrated that metformin as an immunomodulator has garnered significant interest from researchers.
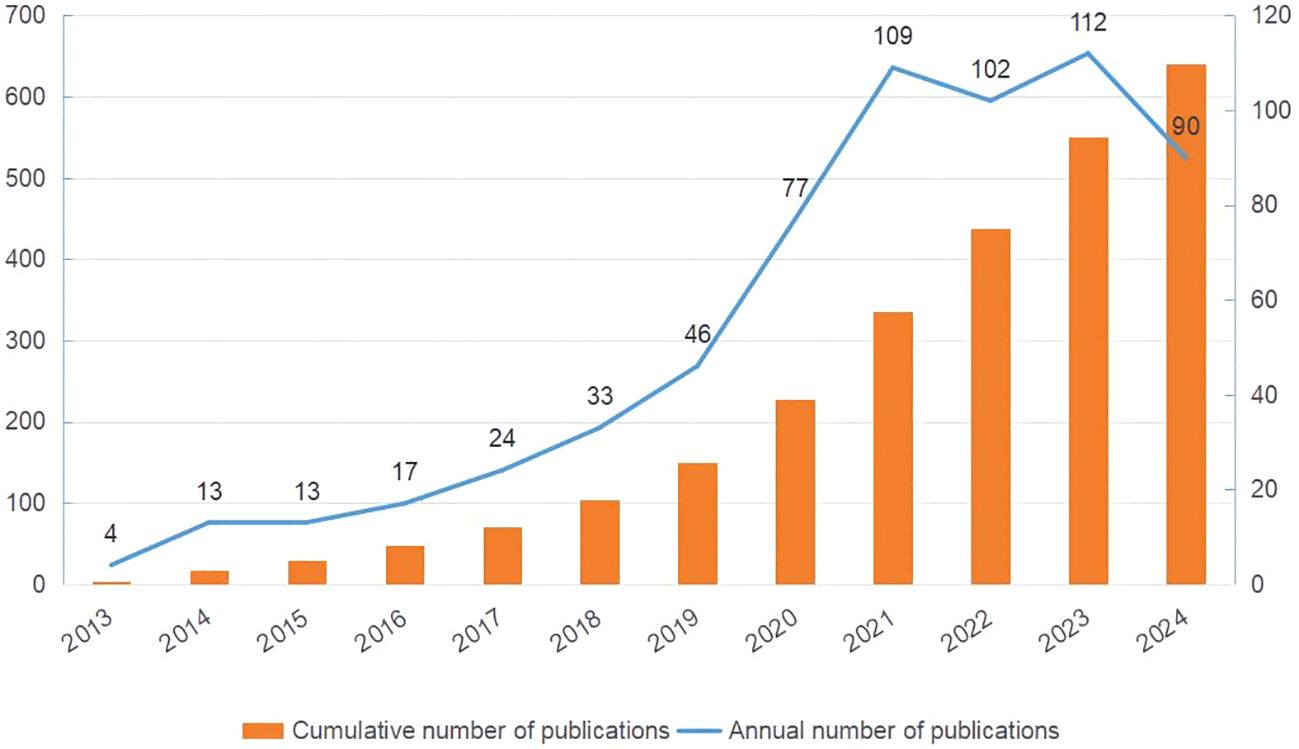
Figure 2. Publication trends of metformin as an immunomodulator. The orange bar chart shows the cumulative number of publications, and the blue line chart represents the annual number of publications. The horizontal axis marks the years, the left vertical axis indicates the cumulative publication count, and the right vertical axis denotes the annual publication count.
3.2 Country and institutional analysis
3.2.1 Inter-country research cooperation network
A total of 77 countries are conducting research on the immunomodulating properties of metformin. Table 1 highlights the top 10 countries based on published articles, with China (n=217) and United States of America (USA) (n=158) accounting for 58.60% of the total number of published articles. This highlights the dominance of these two countries in this field. Notably, although USA has fewer publications than China, the average citation per article (47.95 times) and h-index (47) are higher, indicating greater research quality and influence. Additionally, the size of each node in Figure 3 and the thickness of the connections between adjacent nodes reflect the degree of cooperation between countries. For example, in addition to its deep cooperation with China, USA also has positive cooperative relationships with Germany, Italy, Canada, Japan, and France.
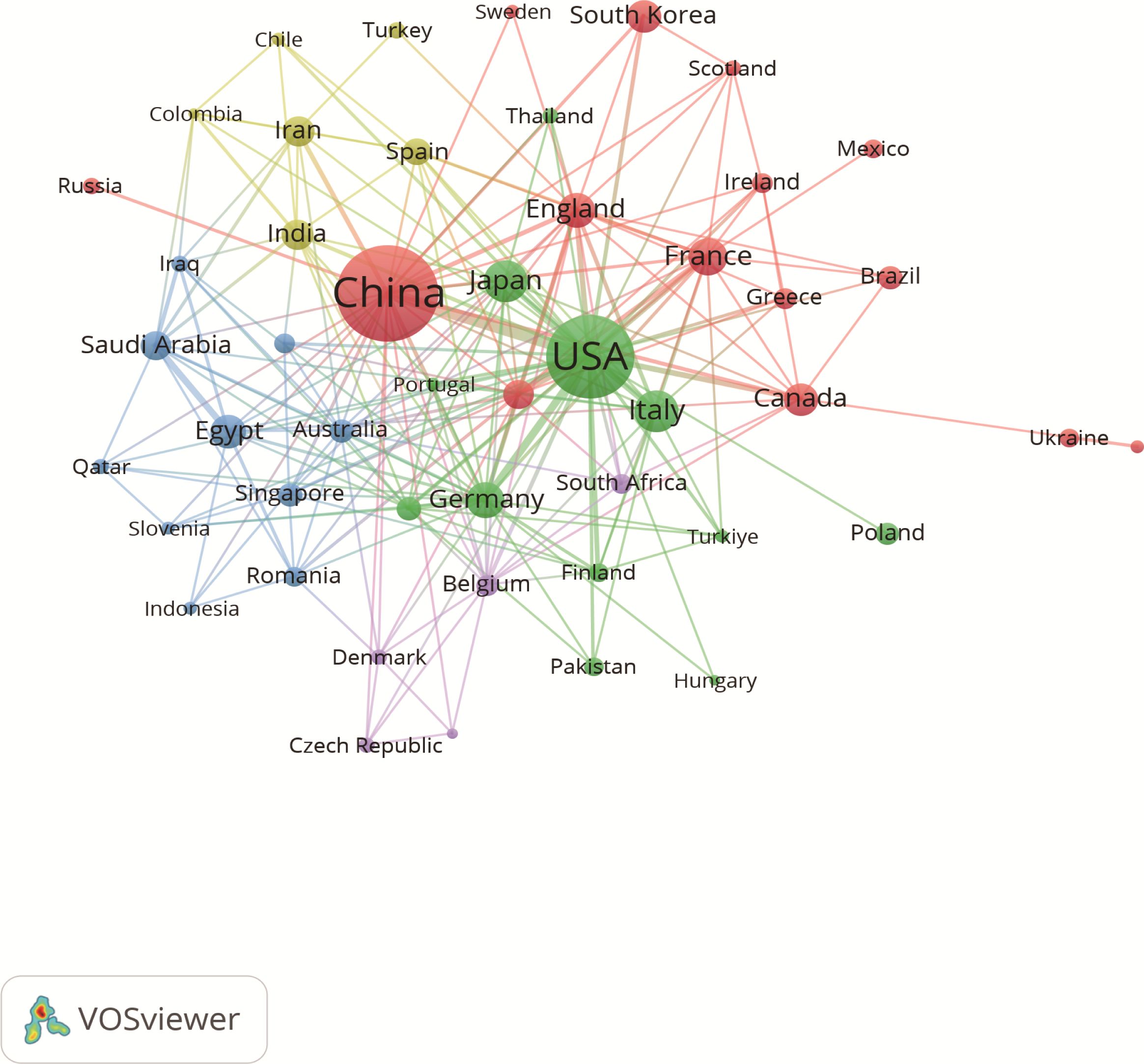
Figure 3. Associations between countries with more than two articles. Each circle represents a node, with larger nodes indicating greater influence of a country. The thickness of the lines denotes the strength of collaboration between two countries.
3.2.2 Inter-agency cooperation network
The studies were performed 1,246 institutions. Table 2 shows the top 14 institutions with more than 7 related publications, including 10 from China, 2 from USA, and 1 from Japan and Austria. Shanghai Jiao Tong Univ (n=17) published the most papers, followed by Okayama Univ (n=15), and Harvard Med School (n=15). Notably, China Med Univ (average citation rate 82.14) and Northwestern Univ (average citation rate 72.14) achieved higher average citation rates. Additionally, the visual map in Figure 4 shows a close cluster effect among different institutions. Specifically, Harvard Med School (Total link strength [TLS]=45), Chinese Acad Sci [TLS=26] exhibit extensive collaborations with other institutions.
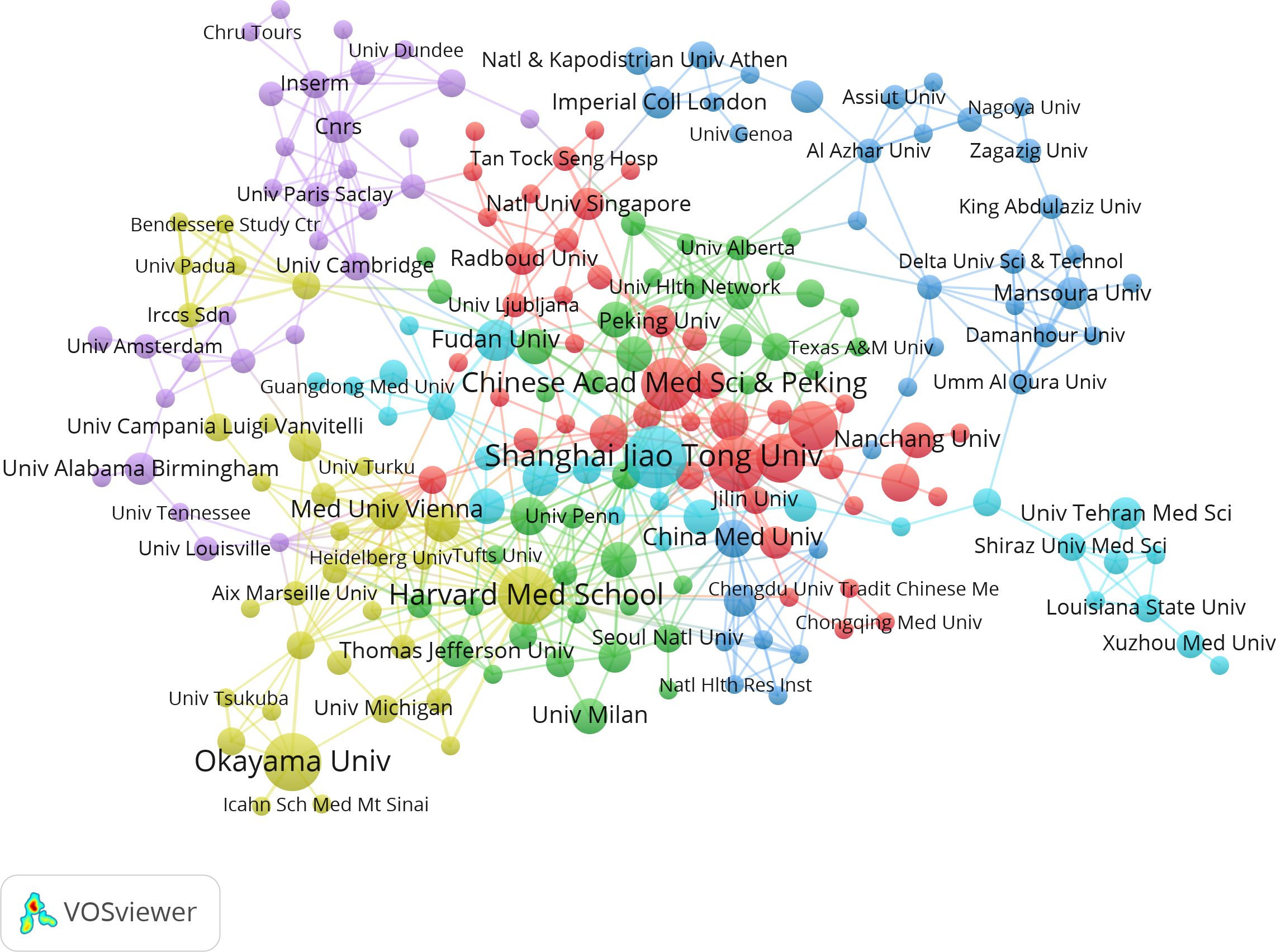
Figure 4. Correlations between institutions with more than two articles. In the network, larger nodes indicate greater importance of an institution and thicker lines show a higher frequency of collaborations between institutions.
3.2.3 Cooperation network among authors
Through screening, a total of 4,353 authors and 28,209 co-cited authors were identified. Table 3 gathers the top 10 most prolific and most frequently co-cited authors. Heiichiro Udono (n=13) emerged as the most prolific author, followed by Mikako Nishida (n=6) and Shingo Eikawa (n=5). Notably, Shingo Eikawa stood out with the highest average citation count of 129.6, highlighting substantial academic influence. Figure 5A illustrates a close collaborative relationships among these cited authors. Furthermore, Figure 5B showcases authors with more than 20 co-citations, with the top three being Shingo Eikawa (113 citations), Marc Foretz (97 citations), and Jeong-Heon Cha (91 citations), indicating the important contributions of these authors in these relevant research fields.
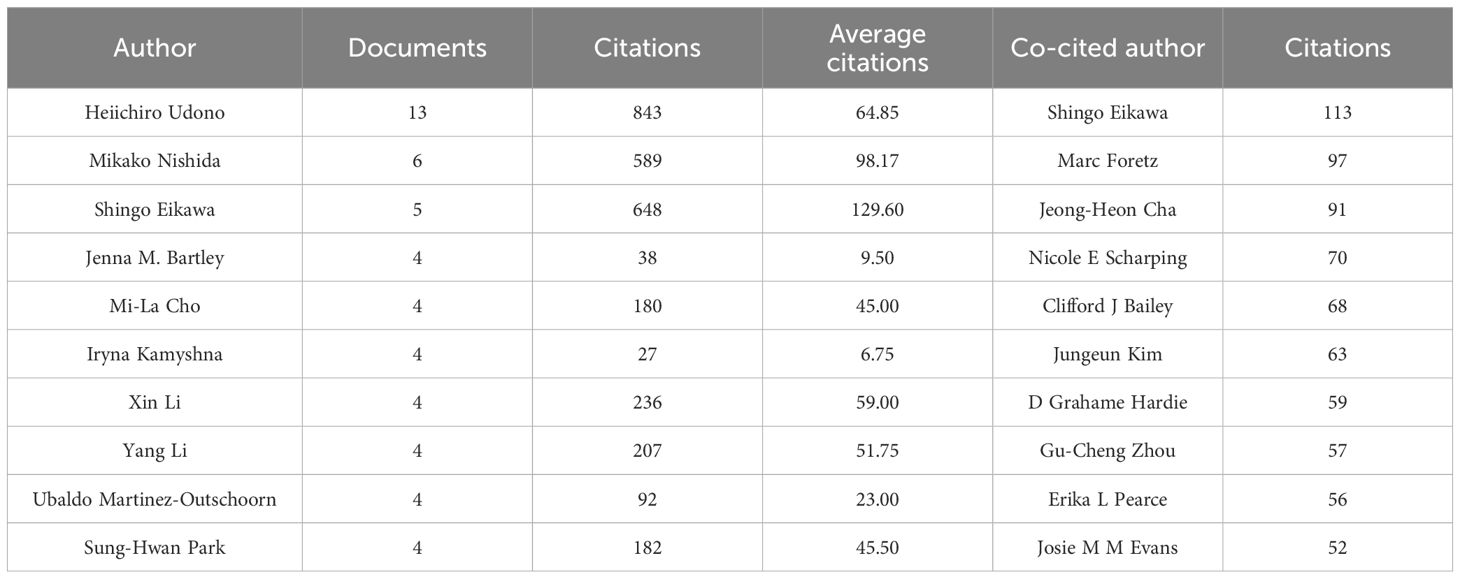
Table 3. Top 10 most prolific authors and the 10 most frequently co-cited authors with the highest citation counts.
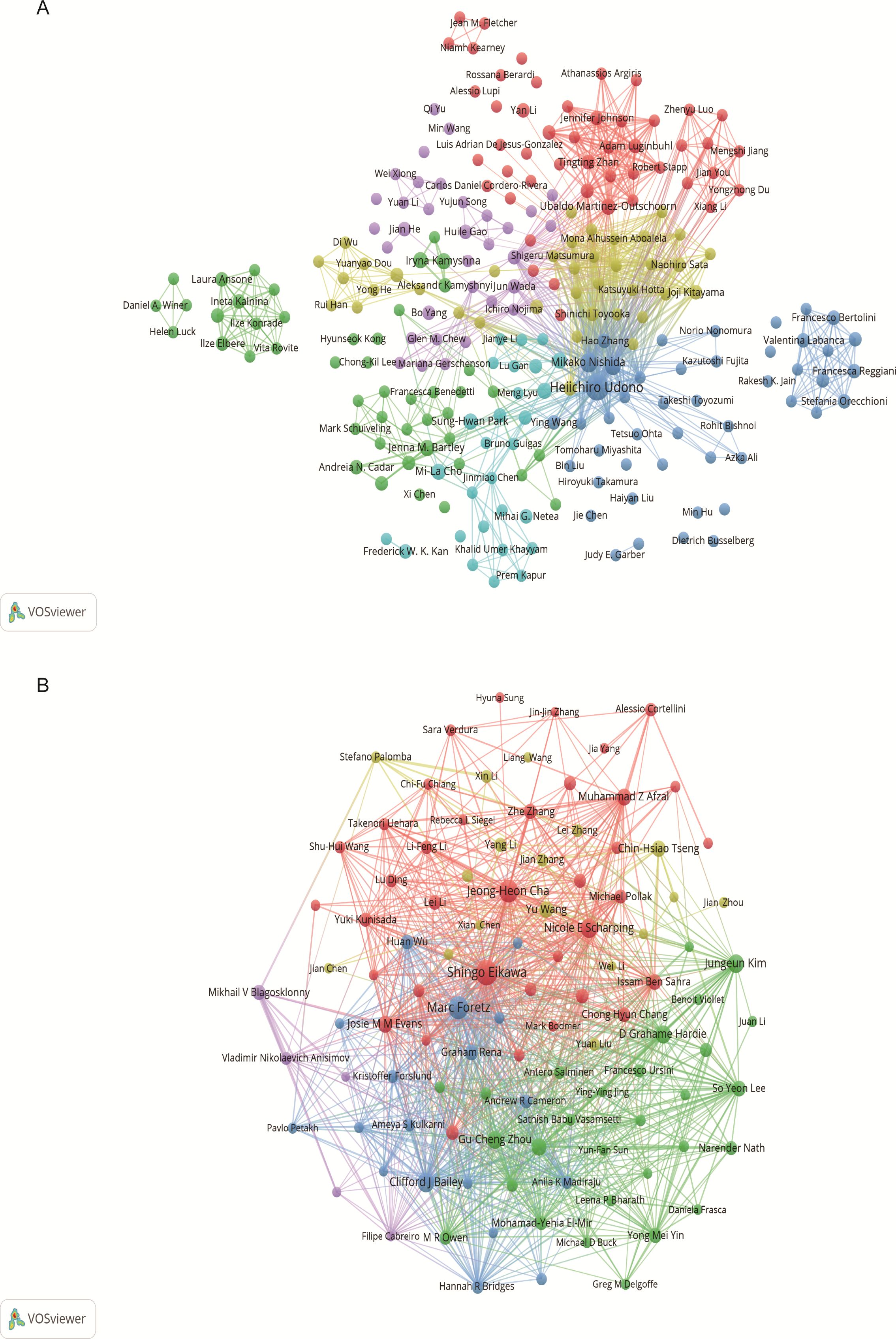
Figure 5. Author visualization in metformin as an immunomodulator. (A) Cited Author Map: This map includes authors cited in at least two studies. Larger nodes indicate higher citation frequencies, and thicker lines denote closer collaborations in the field of metformin and immunology research. (B) Co-cited Author Co-occurrence Map: This map features authors with more than 20 co-citations. Node size and line thickness reflect the frequency of co-citations and the strength of collaborations, respectively. Node colors represent distinct co-cited author collaboration networks.
3.2.4 Cooperation network among journals
The 640 articles included in this study are from 380 journals and 4,438 co-cited journals. The top 10 cited and co-cited journals that published metformin and immunotherapy-related papers are listed in Table 4. Based on the collaboration network of cited journals shown in Figure 6A and the 2024 Journal Citation Report (JCR), International Journal of Molecular Sciences has published the most papers (n=22, IF=4.9), followed by Cancers (n=6, IF=4.5), Frontiers in Immunology (n=16, IF=5.7), Scientific Reports (n=16, IF=3.8), International Immunopharmacology (n=10, IF=4.8), and Journal for Immunotherapy of Cancer (n=10, IF=10.3). The VOSviewer co-occurrence analysis showed that Nature (IF=50.5), Plos One (IF=2.9), and Proceedings of the National Academy of Sciences of the United States of America (PNAS) (IF=9.4) had higher co-citation rates (Figure 6B).
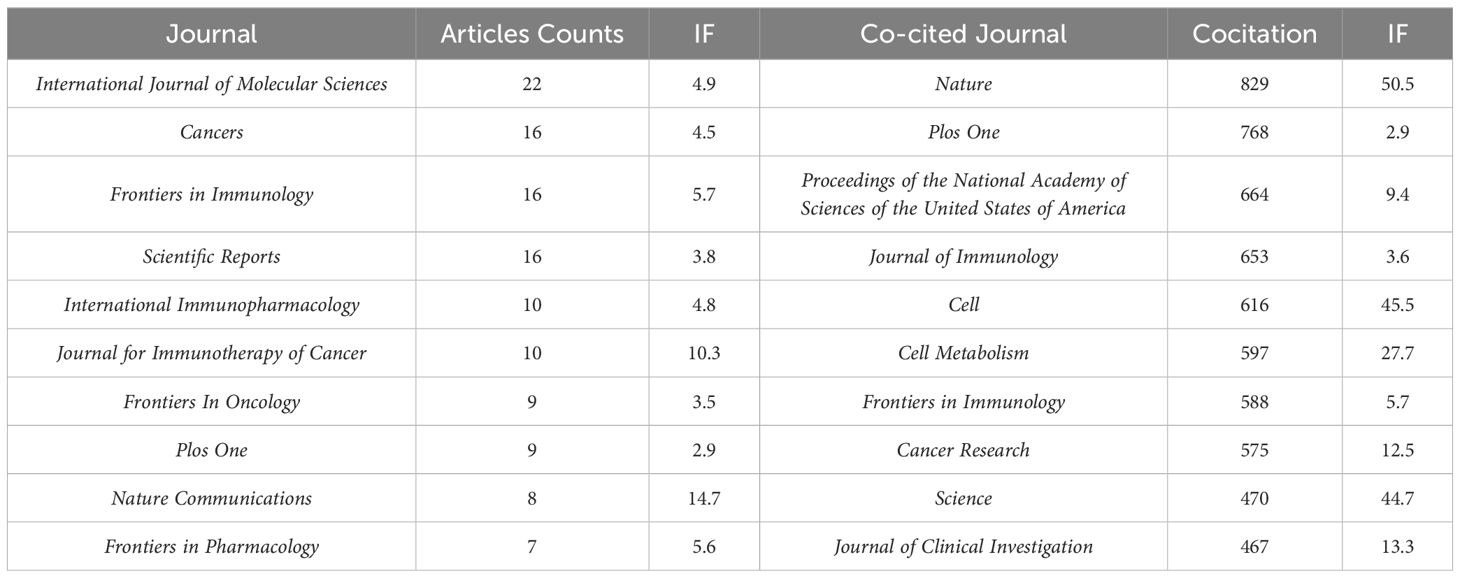
Table 4. Top 10 cited journals and co-cited journals that published literatures on metformin as an immunomodulator.
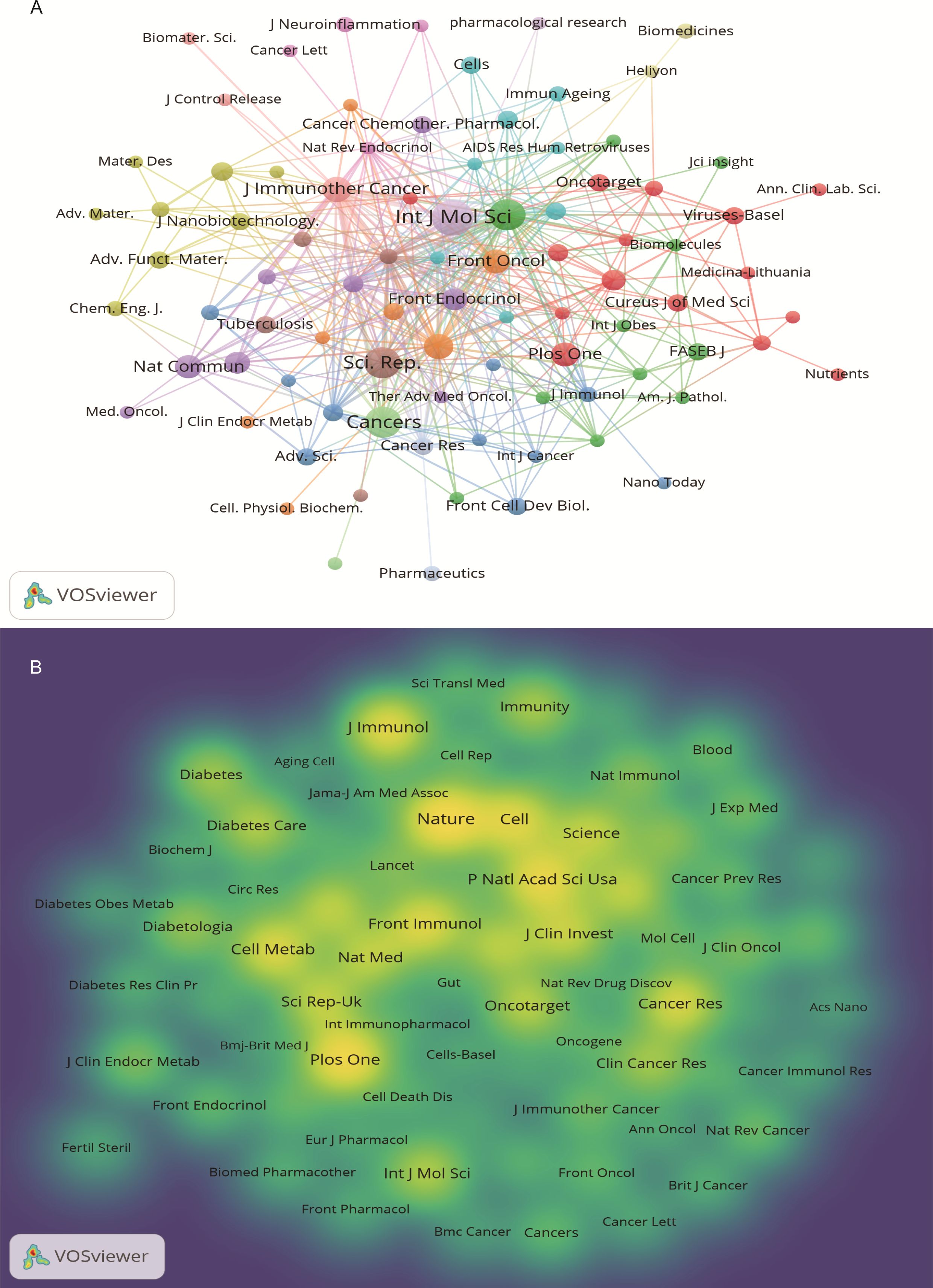
Figure 6. Journal visualization of metformin as an immunomodulator. (A) Cited Journal Co-occurrence Network: Node size represents the influence of journals within the network. Nodes of the same color indicate journal groups with close research connections. (B) Co-cited Journal Density Map: Brightly colored and high-density areas represent journals with high citation frequency, indicating the importance in the field.
3.2.5 Citation and co-citation literature network analysis
An analysis of the top 10 cited and co-cited references using VOSviewer is presented in Tables 5 and 6, with their collaborative networks illustrated in Figures 7A and B. Among the top-cited references, the leading three are Cheng SC. et al. (2014, Science, 1,432 citations); Shin NR et al. (2014, Gut, 1,176 citations); and Jin MZ. et al. (2020, Signal Transduction and Targeted Therapy, 657 citations). These are followed by Cha JH et al. (2018, Nature Immunology, 522 citations) and Eikawa S. et al. (2015, PNAS, 415 citations). For co-cited references, the top three are by Eikawa S. et al. (2015, PNAS, 111 citations); Cha JH et al. (2018, Molecular Cell, 87 citations); and Scharping NE. et al. (2017, Cancer Immunology Research, 63 citations). Figure 8 highlights the top 15 references with the most significant increase in citations since 2013. Notably, the study by Eikawa S. et al., published in 2015, on the anti-tumor immunotherapy effects of metformin, demonstrates the highest citation burst intensity. Moreover, many references continue to show increasing citation rates, indicating that research into the immune mechanisms of metformin remains a prominent and active area of investigation.
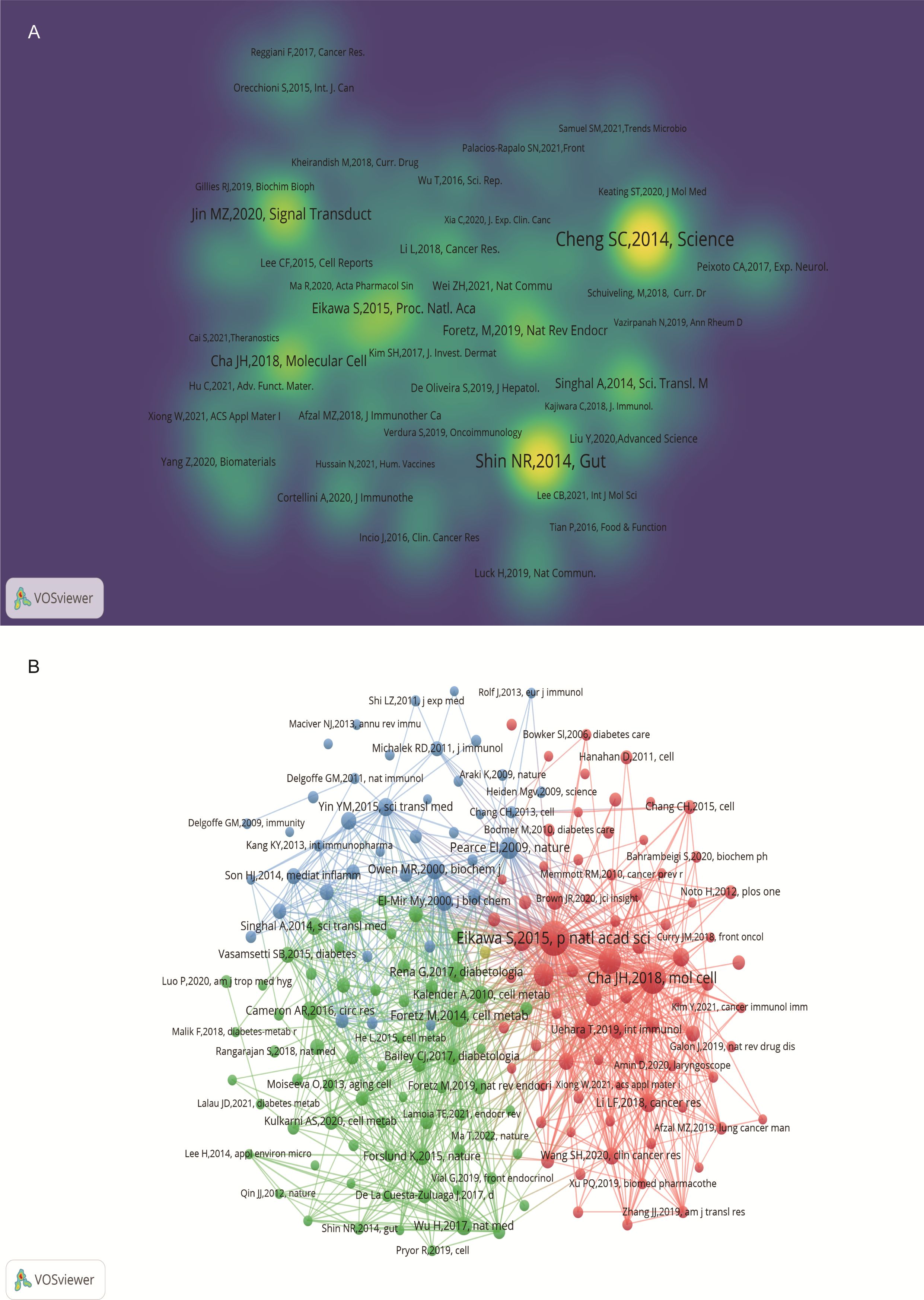
Figure 7. Visualization of cited references on metformin as an immunomodulator. (A) Cited Literature Cluster Analysis: The size of each node is proportional to the citation frequency in literature. Larger nodes indicate higher influence within the network. (B) Co-cited Literature Density Map: Brightly colored and concentrated areas represent literature with higher co-citation frequency, indicating greater importance in the field.
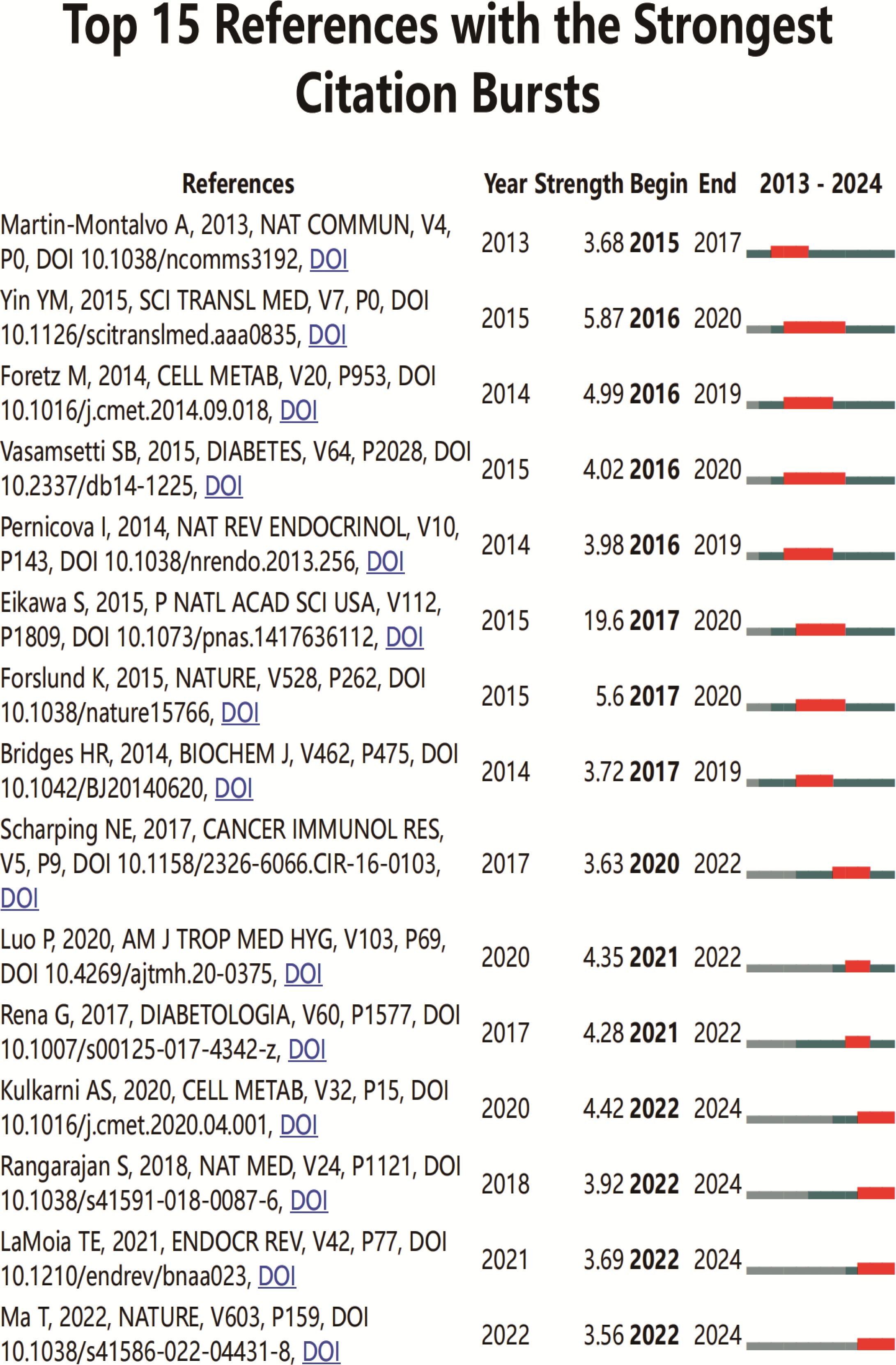
Figure 8. Top 15 co-cited references on metformin as an immunomodulator. Blue line segments represent the timeline from 2013 to 2024, and red markers indicate the specific period and duration of reference bursts.
3.3 Keywords and hotspots
Keyword analysis showed the dynamic progress of metformin’s immune regulation. Using the “author keywords” mode in VOSviewer, a total of 1,615 keywords were obtained. After merging synonyms, 1,537 keywords were analyzed. Table 7 lists the top 20 keywords with the highest frequency, and Figure 9A displays the co-occurring keyword analysis on metformin and immune regulation research showing the evolution of research focus from 2013 to 2024. The top three core keywords were “metformin” (293 occurrences), “diabetes” (78 occurrences), and “immunotherapy” (44 occurrences), followed by “inflammation” (37 occurrences) and “tumor microenvironment” (30 occurrences). These topics have consistently attracted significant interest. Using the density view (Figure 9B) and timeline diagram (Figure 10A) of time evolution, it was observed that since the year 2013, the focus on metformin’s immune regulatory properties gradually shifted from metabolic diseases and insulin resistance to tumor immunity, autoimmune diseases, COVID-19, and aging. The mechanisms broadly involve oxidative stress, inflammation, intestinal flora, immunosuppressive checkpoints, the tumor immune microenvironment, and oxidative glycolysis.
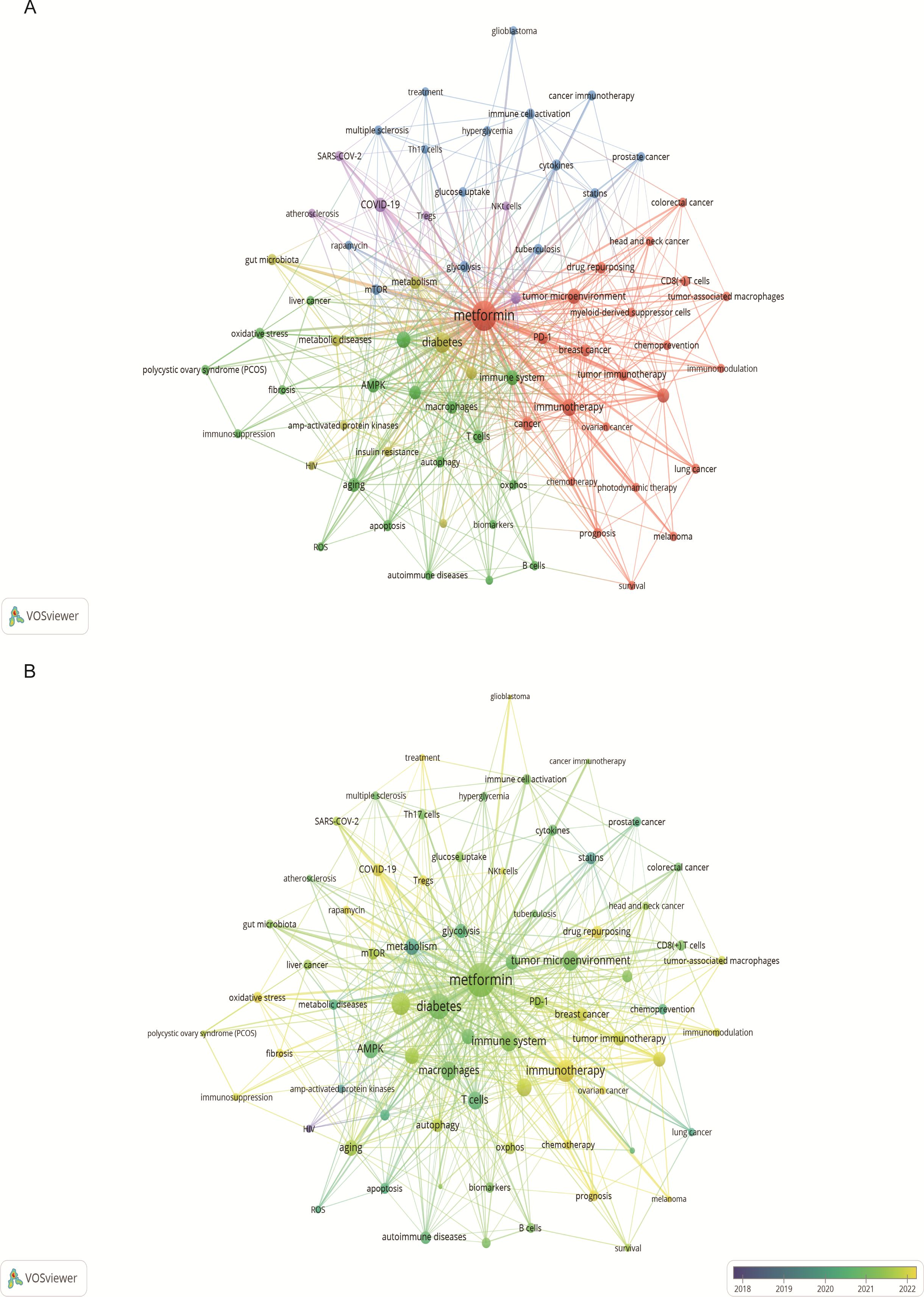
Figure 9. Keyword co-occurrence and annual analysis related to metformin as an immunomodulator. (A) Keyword Co-occurrence Network: Node size corresponds to the frequency of keyword occurrences, and the lines between nodes indicate the strength of the association. Larger nodes denote higher frequencies, and thicker lines represent stronger relationships between keywords. (B) Annual Analysis of Keyword Occurrence: Each node represents a keyword, with its color indicating the average year of occurrence. This visualization shows the research intensity of keywords over specific periods, highlighting the research focus in different time frames.
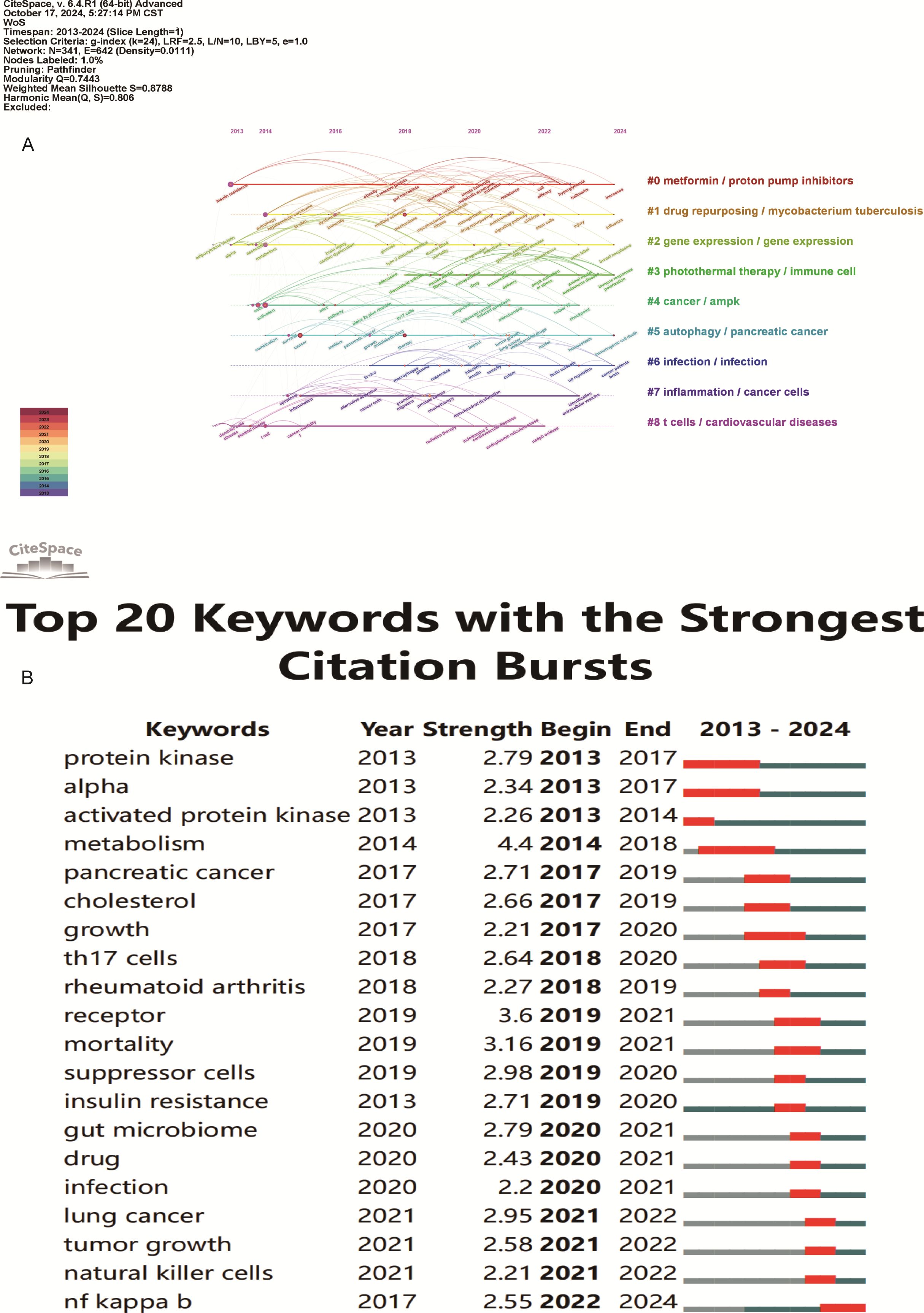
Figure 10. Keyword timeline and burst word analysis of metformin’s role as an Immunomodulator. (A) Keyword Cluster Timeline: Keywords within the same cluster are aligned on the same horizontal line. This visualization shows the number of keywords in each cluster and the time span, indicating the temporal evolution of various research directions. (B) Top 20 Keyword Burst Analysis: Red line segments indicate the start and end of a keyword burst. The “strength” metric represents the intensity of research on a keyword during a specific period, with higher values indicating greater research focus and attention.
From the keyword bursts, the top 20 keywords with the highest burst intensity are shown in Figure 10B. Over the past decade, the focus of metformin immunotherapy has shifted from metabolic and diabetic diseases to the treatment outcomes and mechanisms of tumors. Currently, research is predominantly focused on metformin as an immunomodulator in tumor immunity; however, other mechanisms such as inflammation and intestinal flora are also being explored.
4 Discussion
4.1 General information
This study conducted a visual analysis on data from 640 publications obtained from WOS, using CiteSpace and VOSviewer. The results depict trends in annual publication volume, geographical and institutional cooperation, journal distribution, author co-occurrence, literature cross-citation, and research hotspots. In 2013, this field was in its infancy; however, the academic output increased significantly from 2017 to 2023, with a publication peak observed in 2020. It is expected that the number of articles will continue to grow in 2024 and beyond suggesting of increased interest and focus in this field.
In modern research, the immune response is a crucial factor for understanding the therapeutic mechanism of metformin. Data from various countries and academic institutions showed that most research with high publication volume and h-index has been published from the USA, which has a well-established cooperation network with other countries. Strengthening overall communication would benefit academic development in this field. Analysis of institutional cooperation showed that Chinese institutions dominate research related to metformin immunomodulatory therapy. Domestic institutions frequently cooperate and exhibit regional collection characteristics. The top 3 institutions according to literatures are Shanghai Jiao Tong Univ (17 papers), Okayama Univ (15 papers), and Harvard Med School (15 papers). Regarding journal distribution, the International Journal of Molecular Sciences leads in publication frequency with 22 papers, highlighting its exemplary role in this area of research. The hierarchical structure of journals showed that Cancers, Frontiers in Immunology, and Scientific Reports were the key publishers of this research. Additionally, Nature (co-citation = 829) was the most frequently co-cited journal, with the highest impact factor of 50.5. Heiichiro Udono from Okayama University in Japan was a prolific author in this domain (n=13). He collaborated with Shingo Eikawa on studies investigating the enhanced effect of cancer immunotherapy through metabolic intervention with metformin, and has published several highly cited articles (29, 30). These research results provided new perspectives and potential therapeutic strategies for the application of metformin in cancer immunotherapy, deepening the understanding of its immunoregulatory mechanisms (31–33). The top 10 references mainly focus on tumor immunity, tumor hypoxia, the tumor microenvironment, immune checkpoint inhibition, anti-inflammatory effects, tuberculosis, polycystic ovary syndrome, gut microbiota, and the endoplasmic reticulum pathway. This highlights the immunomodulatory role of metformin in various diseases, etiologies, and pathological mechanisms. Its wide application offers significant guidance for both basic and clinical research.
4.2 Hotspots and frontiers
4.2.1 Metformin-mediated tumor immunity
The study of high-frequency keywords can clarify the hot spot distribution and trajectory on research elated to metformin and host immunity research over the recent years. Previously, researchers have demonstrated promising anticancer effects of metformin on prostate, breast, lung, liver, colon, ovarian, head and neck, glioblastoma cancers (34). Metformin is a highly effective and promising anti-tumor inhibitor. Targeted tumor immunity is an attractive research topic. As shown in the Figure 9A, keyword clusters such as ″tumor immunotherapy″, ″tumor microenvironment″, ″immune checkpoint inhibitors″, ″immunotherapy″, ″drug repurposing″, ″tumor-associated macrophages″, ″CD8(+) T cell″, ″lung cancer″, ″breast cancer″, ″photodynamic therapy″, ″chemoprevention″, and ″survival″ highlight the prominence of metformin’s immune-mediated effects in tumor research. These indicate that the immune-mediated role of metformin in tumor diseases is a current topic of interest. Its detailed mechanism of action involves many aspects of tumor pathogenesis, such as the tumor immune microenvironment, immune checkpoint inhibition, and the application of metformin derivatives.
Firstly, metformin significantly influences the regulation of the tumor immune microenvironment, covering a variety of immune cells and their interactions, inhibiting tumor metastasis, progression, and angiogenesis. It enhances the anti-tumor immune function of CD8+ T cells by regulating glucose metabolism, activating fatty acid oxidation, and targeting oxidative phosphorylation (35). Metformin can promote the maturation of dendritic cells by depressing mitochondrial complex I, enhance the co-stimulatory ability of CD4+ T cells, and further stimulate the immune response (36). Through the p38 mitogen-activated protein kinase pathway, metformin enhances the anti-tumor activity of natural killer cells and increases their toxicity to cancer cells (37). It also inhibits tumor infiltration of Treg and myeloid-derived suppressor cells (38), stimulates the proliferation of natural killer T and T cells (14), and delays cancer growth. Studies have shown that metformin regulates macrophage function, increases tumor-infiltrating T cells, and significantly heightened the anti-tumor effect of cancer vaccines (39, 40). When combined with antitumor drugs such as pazopanib and cyclophosphamide, metformin improves the immune microenvironment. This could be achieved by inhibiting phosphorylated protein kinase B/nuclear factor kappa-light-chain-enhancer of activated B cell/signal transducer and activator of transcription 3 (STAT3p-Akt/NF-κB/STAT3)/PD-L1 pathway (41, 42). In addition, metformin causes immunogenic death of ovarian cancer cells and inhibits tumor growth by activating AMPK (43). It improves prognosis and prolongs survival in patients with pancreatic and breast cancer by reducing the abundance of M2 macrophages and increasing tumor-infiltrating lymphocytes (TIL) (44, 45). In summary, metformin shows a wide range of anticancer potential by modulating anti-tumor immune cells, reducing immunosuppression, and significantly improving the tumor immune microenvironment.
Secondly, immune checkpoint molecules, such as PD-L1 and cytotoxic T-lymphocyte associated protein 4(CTLA4), have been extensively investigated as a cancer therapy, with this immunotherapy notably enhancing the survival rates of patients with certain types of cancer (46). Metformin, as a small molecule immune checkpoint inhibitor, decreases PD-L1 expression on tumor cell surfaces and boosts the cytotoxic T cells ability to kill (20). By activating AMPK, metformin phosphorylates PD-L1, induces endoplasmic reticulum stress, and enhances anti-tumor immunity (47). Concurrently, metformin restrains the expression of mechanistic target of rapamycin (mTOR) signaling and hypoxia-inducible factor 1-alpha (HIF-1α), decreases oxygen consumption, strengthens the efficacy of PD-1 blockade therapy, and diminishes immune escape (48, 49). In addition, metformin combined with anti-PD-1 therapy promotes the normalization of tumor blood vessels, increases the expression of vascular endothelial cadherin (VE-cadherin) and vascular cell adhesion molecule 1(VCAM-1), and infiltration of CD8+ T cells, and slows tumor growth (32). Studies have shown that metformin significantly prolonged overall survival (OS) and progression-free survival (PFS) in patients with non-small cell lung cancer (NSCLC) treated with immune checkpoint inhibitors (50). This combination therapy not only enhances the effectiveness of immunotherapy but enhances the prognosis of cancer patients.
Further, reuse of metformin has gained momentum. Due to its clear anti-cancer effect, good safety, and tolerance, but low drug concentration, researchers have developed a variety of metformin derivatives to enhance its efficacy in anti-tumor immunotherapy. The platinum-metformin conjugate promotes the degradation of PD-L1 through the AMPK/transcription factor EB (TFEB) pathway and enhances the anti-tumor immune effect in NSCLC (51). Researchers have further enhanced the therapeutic effects on cold tumors and drug-resistant tumors by using nanotechnology delivery systems combined with photodynamic therapy, radiotherapy, and immune checkpoint blockade therapy (52–54). For example, MET-HMME/CAT-HMME@Nlip (55) and Mn-MSN@Met-M NPs (56) improves tumor hypoxia, activates the stimulator of interferon genes (STING) pathway, and improves efficacy of PD-1 inhibitors. Tpp-Met@MnO2@Alb nano-adjuvant enhances the sensitivity of tumors to radiotherapy by regulating PD-L1 and transforming growth factor beta 1(TGF-β1) and provides a long-term immune memory effect (52). Nanogels (D@HPMNG) (57) significantly inhibits the growth and recurrence of melanoma by reprogramming tumor-associated macrophages (58), whereas HMMDN-Met@PM enhances tumor inhibition by promoting the transformation of M2 to M1 (59). Through these mechanisms, metformin derivatives not only improve the tumor microenvironment but also inhibit tumor growth and metastasis, providing a long-term anti-tumor immune effect.
In summary, the interplay between metformin and the immune response is a complex and dynamic research area with exciting implications. These results might have important applications in controlling cancer progression and prognosis, opening avenues for the development of innovative treatments.
4.2.2 Application of metformin immunotherapy in other diseases
In addition to anti-tumor immunity, metformin has a broad application in several diseases because of its ability to regulate the immune system. Keywords clusters such as ″diabetes″, ″metabolism″, ″autoimmune diseases″, ″polycystic ovary syndrome″, ″multiple sclerosis″, ″SARS-COV-2″, ″aging″, ″obesity″, etc., are all recent research topics. Metformin improves type 1 diabetes (T1DM), autoimmune diseases, and metabolic syndrome by activating AMPK pathway, inhibiting pro-inflammatory Th1 and Th17 cells, and promoting Tregs development (60, 61). Metformin has been shown to regulate differentiation of immune complexes and B cells and reduce the inflammatory response of diseases such as systemic lupus erythematosus (62). It also improves Sjogren’s syndrome by restoring Ca2+ signaling and inhibiting ERS (63), and enhances immune recognition and lowers viral replication in human immunodeficiency virus type 1(HIV-1) infection (64) and Helicobacter pylori infection. Metformin exhibits anti-inflammatory and antiviral effects in COVID-19 infections likely improving immune response and prognosis (65). Surprisingly, it can also promote osteogenic differentiation of bone marrow mesenchymal stem cells by regulating the macrophage phenotype (66) and enhancing innate immunity in tuberculosis (67, 68). In addition, metformin exhibits anti-aging and anti-fibrosis effects by reducing the number of senescent T cells and improving T cell function (69) and exhibits immunomodulatory effects in diseases such as polycystic ovary syndrome, pregnancy, and liver disease (70).
4.2.3 Research hotspots on the basic mechanism of metformin immunotherapy
A clustered analysis of existing keywords showed that metformin regulated direct immune cells and also regulated immunity through other pathways involving ″fibrosis″, ″oxidative stress″, ″inflammation″, ″apoptosis″, ″ROS″, ″mTOR″, ″AMPK″, ″mitochondrial dysfunction″, ″gut microbiota″, and ″OXPHOS″, and ″mTOR″ signaling (71, 72). In addition, metformin can inhibit the differentiation of pro-inflammatory macrophages (M1) and reduce the production of reactive oxygen species (ROS) showing a strong anti-inflammatory effect (73, 74). By regulating reactive oxygen/nitrogen levels and immune metabolism, metformin may help prevent age-related diseases and provide a new direction for clinical treatment (75). It improves diabetic complications and T cell function in patients with COVID-19 by reprogramming dendritic cell metabolism, inducing tolerance, and reducing pro-inflammatory factors such as IL-2 and tumor necrosis factor alpha (76). Metformin also reduces inflammation caused by obesity and insulin resistance by regulating Th17/Treg balance, intestinal flora, and NF-κB signaling pathway (77, 78). Furthermore, it improves efficacy of anti-PD-L1 antibodies by affecting the intestinal microbiota, promotes anti-tumor immunity (79), and shows antiviral potential for COVID-19 treatment (80). In short, the relationship between anti-inflammatory, intestinal flora, and metformin immune regulation is an emerging research field. Although some studies have reported on alterations in the gut microbiome after the use of metformin (78), the exact mechanism by which metformin regulates the gut microbiome and the impact of these changes on the efficacy of metformin immunotherapy is not known. Further research is required to understand these effects.
4.3 Advantages and limitations
This study for the first time systematically used VOSviewer and CiteSpace to analyze the bibliometrics of research related to metformin and immune regulation, providing an overview of the cooperation network and research trends. However, there are some limitations to this study. In this study, the data was sourced from only the core collection of WOS, excluding other databases and non-English publications. Further, reference delays and database updates might have also affected the accuracy of trend analysis. Nevertheless, by combining published literature, this study offered valuable insights into the clinical application of metformin as an immunomodulator. Although current in vivo and in vitro studies have identified important mechanisms underlying the inhibitory effect of metformin on cancer immunity and other diseases, systematic and large-center clinical research studies are warranted.
5 Conclusion
This study used bibliometric analysis to review the dynamics and developments in the immunomodulatory effects of metformin. With the rapid growth of research on the role of metformin and its derivatives in cancer immunotherapy, the prospects of metformin use are expanding. Notably, as an immunomodulator, metformin not only improves the tumor immune microenvironment and enhances the efficacy of immune checkpoint inhibitors but also boosts the treatment effects of radiotherapy, cold tumors, and drug-resistant tumors, along with minimizing its side effects. Beyond tumor immunity, the immunomodulatory effects of metformin in aging, tuberculosis, viral infections, and autoimmune diseases, and its potential in gut microbiota and anti-inflammatory treatments, are important for future research. Further exploration of the detailed mechanisms of metformin as an immunomodulator and its biological distribution in tissues is crucial for optimizing its clinical application.
Data availability statement
The raw data supporting the conclusions of this article will be made available by the authors, without undue reservation.
Author contributions
TZ: Conceptualization, Writing – original draft, Writing – review & editing. YY: Methodology, Writing – original draft. LL: Formal analysis, Writing – original draft. XL: Data curation, Writing – original draft. QX: Visualization, Writing – original draft. RY: Conceptualization, Supervision, Writing – review & editing.
Funding
The author(s) declare financial support was received for the research, authorship, and/or publication of this article. This study was supported by key projects supported by the National Natural Science Foundation of China and Joint Fund for Regional Innovation and Development (U21A20411); Hunan Provincial Natural Science Foundation Project (2024JJ5303); Hunan Provincial Traditional Chinese Medicine Research Project (B2024003); Graduate Innovation Project of Hunan University of Chinese Medicine(2023CX06).
Conflict of interest
The authors declare that the research was conducted in the absence of any commercial or financial relationships that could be construed as a potential conflict of interest.
Generative AI statement
The author(s) declare that no Generative AI was used in the creation of this manuscript.
Publisher’s note
All claims expressed in this article are solely those of the authors and do not necessarily represent those of their affiliated organizations, or those of the publisher, the editors and the reviewers. Any product that may be evaluated in this article, or claim that may be made by its manufacturer, is not guaranteed or endorsed by the publisher.
References
1. Ahmad E, Sargeant JA, Zaccardi F, Khunti K, Webb DR, Davies MJ. Where does metformin stand in modern day management of type 2 diabetes? Pharm (Basel). (2020) 13:427. doi: 10.3390/ph13120427
2. Foretz M, Guigas B, Viollet B. Metformin: update on mechanisms of action and repurposing potential. Nat Rev Endocrinol. (2023) 19:460–76. doi: 10.1038/s41574-023-00833-4
3. Salvatore T, Pafundi PC, Morgillo F, Di Liello R, Galiero R, Nevola R, et al. Metformin: An old drug against old age and associated morbidities. Diabetes Res Clin Pract. (2020) 160:108025. doi: 10.1016/j.diabres.2020.108025
4. Dutta S, Shah RB, Singhal S, Dutta SB, Bansal S, Sinha S, et al. Metformin: A review of potential mechanism and therapeutic utility beyond diabetes. Drug Des Devel Ther. (2023) 17:1907–32. doi: 10.2147/DDDT.S409373
5. He L. Metformin and systemic metabolism. Trends Pharmacol Sci. (2020) 41:868–81. doi: 10.1016/j.tips.2020.09.001
6. Bharath LP, Nikolajczyk BS. The intersection of metformin and inflammation. Am J Physiol Cell Physiol. (2021) 320:C873–9. doi: 10.1152/ajpcell.00604.2020
7. Lu G, Wu Z, Shang J, Xie Z, Chen C, Zhang C. The effects of metformin on autophagy. BioMed Pharmacother. (2021) 137:111286. doi: 10.1016/j.biopha.2021.111286
8. Pavlo P, Kamyshna I, Kamyshnyi A. Effects of metformin on the gut microbiota: A systematic review. Mol Metab. (2023) 77:101805. doi: 10.1016/j.molmet.2023.101805
9. Panaampon J, Zhou Y, Saengboonmee C. Metformin as a booster of cancer immunotherapy. Int Immunopharmacol. (2023) 121:110528. doi: 10.1016/j.intimp.2023.110528
10. Chen GG, Woo PYM, Ng SCP, Wong GKC, Chan DTM, Van Hasselt C A, et al. Impact of metformin on immunological markers: Implication in its anti-tumor mechanism. Pharmacol Ther. (2020) 213:107585. doi: 10.1016/j.pharmthera.2020.107585
11. Abdelmoneim M, Aboalela MA, Naoe Y, Matsumura S, Eissa IR, Bustos-Villalobos I, et al. The impact of metformin on tumor-infiltrated immune cells: preclinical and clinical studies. Int J Mol Sci. (2023) 24:13353. doi: 10.3390/ijms241713353
12. Pearce EL, Walsh MC, Cejas PJ, Harms GM, Shen H, Wang L S, et al. Enhancing CD8 T-cell memory by modulating fatty acid metabolism. Nature. (2009) 460:103–7. doi: 10.1038/nature08097
13. Finisguerra V, Dvorakova T, Formenti M, Van Meerbeeck P, Mignion L, Gallez B, et al. Metformin improves cancer immunotherapy by directly rescuing tumor-infiltrating CD8 T lymphocytes from hypoxia-induced immunosuppression. J Immunother Cancer. (2023) 11:e005719. doi: 10.1136/jitc-2022-005719
14. Petrovic A, Jovanovic I, Stojanovic B, Dimitrijevic Stojanovic M, Stojanovic BS, Jurisevic M, et al. Harnessing metformin’s immunomodulatory effects on immune cells to combat breast cancer. Int J Mol Sci. (2024) 25:5869. doi: 10.3390/ijms25115869
15. Huang X, Sun T, Wang J, Hong X, Chen H, Yan T, et al. Metformin reprograms tryptophan metabolism to stimulate CD8+ T-cell function in colorectal cancer. Cancer Res. (2023) 83:2358–71. doi: 10.1158/0008-5472.CAN-22-3042
16. Crist M, Yaniv B, Palackdharry S, Lehn MA, Medvedovic M, Stone T, et al. Metformin increases natural killer cell functions in head and neck squamous cell carcinoma through CXCL1 inhibition. J Immunother Cancer. (2022) 10:e005632. doi: 10.1136/jitc-2022-005632
17. Abd El-Fattah EE, Zakaria AY. Metformin modulate immune fitness in hepatocellular carcinoma: Molecular and cellular approach. Int Immunopharmacol. (2022) 109:108889. doi: 10.1016/j.intimp.2022.108889
18. Levy A, Doyen J. Metformin for non-small cell lung cancer patients: Opportunities and pitfalls. Crit Rev Oncol Hematol. (2018) 125:41–7. doi: 10.1016/j.critrevonc.2018.03.001
19. Chen S, Zhou X, Yang X, Li W, Li S, Hu Z, et al. Dual blockade of lactate/GPR81 and PD-1/PD-L1 pathways enhances the anti-tumor effects of metformin. Biomolecules. (2021) 11:1373. doi: 10.3390/biom11091373
20. Park SH, Lee J, Yun HJ, Kim SH, Lee JH. Metformin suppresses both PD-L1 expression in cancer cells and cancer-induced PD-1 expression in immune cells to promote antitumor immunity. Ann Lab Med. (2024) 44:426–36. doi: 10.3343/alm.2023.0443
21. Landry DA, Yakubovich E, Cook DP, Fasih S, Upham J, Vanderhyden BC. Metformin prevents age-associated ovarian fibrosis by modulating the immune landscape in female mice. Sci Adv. (2022) 8:eabq1475. doi: 10.1126/sciadv.abq1475
22. Cortes M, Brischetto A, Martinez-Campanario MC, Ninfali C, Dominguez V, Fernandez S, et al. Inflammatory macrophages reprogram to immunosuppression by reducing mitochondrial translation. Nat Commun. (2023) 14:7471. doi: 10.1038/s41467-023-42277-4
23. Jing Y, Wu F, Li D, Yang L, Li Q, Li R. Metformin improves obesity-associated inflammation by altering macrophages polarization. Mol Cell Endocrinol. (2018) 461:256–64. doi: 10.1016/j.mce.2017.09.025
24. Vasamsetti SB, Karnewar S, Kanugula AK, Thatipalli AR, Kumar JM, Kotamraju S. Metformin inhibits monocyte-to-macrophage differentiation via AMPK-mediated inhibition of STAT3 activation: potential role in atherosclerosis. Diabetes. (2015) 64:2028–41. doi: 10.2337/db14-1225
25. Yan Z, Tian X, Zhu J, Lu Z, Yu L, Zhang D, et al. Metformin suppresses UHMWPE particle-induced osteolysis in the mouse calvaria by promoting polarization of macrophages to an anti-inflammatory phenotype. Mol Med. (2018) 24:20. doi: 10.1186/s10020-018-0013-x
26. Solier S, Muller S, Caneque T, Versini A, Mansart A, Sindikubwabo F, et al. A druggable copper-signalling pathway that drives inflammation. Nature. (2023) 617:386–94. doi: 10.1038/s41586-023-06017-4
27. Van Eck NJ, Waltman L. Software survey: VOSviewer, a computer program for bibliometric mapping. Scientometrics. (2010) 84:523–38. doi: 10.1007/s11192-009-0146-3
28. Chen C. Searching for intellectual turning points: progressive knowledge domain visualization. Proc Natl Acad Sci U.S.A. (2004) 101 Suppl 1:5303–10. doi: 10.1073/pnas.0307513100
29. Nojima I, Eikawa S, Tomonobu N, Hada Y, Kajitani N, Teshigawara S, et al. Dysfunction of CD8 + PD-1 + T cells in type 2 diabetes caused by the impairment of metabolism-immune axis. Sci Rep. (2020) 10:14928. doi: 10.1038/s41598-020-71946-3
30. Kunisada Y, Eikawa S, Tomonobu N, Domae S, Uehara T, Hori S, et al. Attenuation of CD4(+)CD25(+) regulatory T cells in the tumor microenvironment by metformin, a type 2 diabetes drug. EBioMedicine. (2017) 25:154–64. doi: 10.1016/j.ebiom.2017.10.009
31. Nishida M, Yamashita N, Ogawa T, Koseki K, Warabi E, Ohue T, et al. Mitochondrial reactive oxygen species trigger metformin-dependent antitumor immunity via activation of Nrf2/mTORC1/p62 axis in tumor-infiltrating CD8T lymphocytes. J Immunother Cancer. (2021) 9:e002954. doi: 10.1136/jitc-2021-002954
32. Tokumasu M, Nishida M, Zhao W, Chao R, Imano N, Yamashita N, et al. Metformin synergizes with PD-1 blockade to promote normalization of tumor vessels via CD8T cells and IFNgamma. Proc Natl Acad Sci U.S.A. (2024) 121:e2404778121. doi: 10.1073/pnas.2404778121
33. Uehara T, Eikawa S, Nishida M, Kunisada Y, Yoshida A, Fujiwara T, et al. Metformin induces CD11b+-cell-mediated growth inhibition of an osteosarcoma: implications for metabolic reprogramming of myeloid cells and anti-tumor effects. Int Immunol. (2019) 31:187–98. doi: 10.1093/intimm/dxy079
34. Ma R, Yi B, Riker AI, Xi Y. Metformin and cancer immunity. Acta Pharmacol Sin. (2020) 41:1403–9. doi: 10.1038/s41401-020-00508-0
35. Pujalte-Martin M, Belaid A, Bost S, Kahi M, Peraldi P, Rouleau M, et al. Targeting cancer and immune cell metabolism with the complex I inhibitors metformin and IACS-010759. Mol Oncol. (2024) 18:1719–38. doi: 10.1002/1878-0261.13583
36. Turpin R, Liu R, Munne PM, Peura A, Rannikko JH, Philips G, et al. Respiratory complex I regulates dendritic cell maturation in explant model of human tumor immune microenvironment. J Immunother Cancer. (2024) 12:e008053. doi: 10.1136/jitc-2023-008053
37. Xia W, Qi X, Li M, Wu Y, Sun L, Fan X, et al. Metformin promotes anticancer activity of NK cells in a p38 MAPK dependent manner. Oncoimmunology. (2021) 10:1995999. doi: 10.1080/2162402X.2021.1995999
38. Kang J, Lee D, Lee KJ, Yoon JE, Kwon JH, Seo Y, et al. Tumor-suppressive effect of metformin via the regulation of M2 macrophages and myeloid-derived suppressor cells in the tumor microenvironment of colorectal cancer. Cancers (Basel). (2022) 14:2881. doi: 10.3390/cancers14122881
39. Scafidi A, Lind-Holm Mogensen F, Campus E, Pailas A, Neumann K, Legrave N, et al. Metformin impacts the differentiation of mouse bone marrow cells into macrophages affecting tumour immunity. Heliyon. (2024) 10:e37792. doi: 10.1016/j.heliyon.2024.e37792
40. Guo Q, Wang L, Wuriqimuge, Dong L, Feng M, Bao X, et al. Metformin improved a heterologous prime-boost of dual-targeting cancer vaccines to inhibit tumor growth in a melanoma mouse model. Int Immunopharmacol. (2024) 128:111431. doi: 10.1016/j.intimp.2023.111431
41. Abdallah FM, Ghoneim AI, Abd-Alhaseeb MM, Abdel-Raheem IT, Helmy MW. Unveiling the antitumor synergy between pazopanib and metformin on lung cancer through suppressing p-Akt/NF-kappaB/STAT3/PD-L1 signal pathway. BioMed Pharmacother. (2024) 180:117468. doi: 10.1016/j.biopha.2024.117468
42. Zaki HM, Ali KM, Abd Allah MYY, Abouelnaga AM, Abdraboh ME, Hussein O. Metronomic cyclophosphamide and metformin inhibited tumor growth and repopulated tumor-infiltrating lymphocytes in an experimental carcinoma model. BMC Res Notes. (2024) 17:4. doi: 10.1186/s13104-023-06651-1
43. Chen Y, Wang L, Chen N, Tang G. Metformin induces tumor immunogenic cell death in ovarian cancer by activating AMPK pathway. Transl Oncol. (2024) 47:102052. doi: 10.1016/j.tranon.2024.102052
44. Van Eijck CWF, Vadgama D, Van Eijck CHJ, Wilmink JW, Dutch Pancreatic Cancer G. Metformin boosts antitumor immunity and improves prognosis in upfront resected pancreatic cancer: an observational study. J Natl Cancer Inst. (2024) 116:1374–83. doi: 10.1093/jnci/djae070
45. Shiba S, Harao M, Saito A, Sakuragi M, Kitayama J, Sata N. Metformin alters tumor immune microenvironment, improving the outcomes of breast cancer patients with type 2 diabetes mellitus. J Breast Cancer. (2024) 27:121–9. doi: 10.4048/jbc.2023.0285
46. Leong SP. Immune responses and immunotherapeutic approaches in the treatment against cancer. Clin Exp Metast. (2024) 41:473–93. doi: 10.1007/s10585-024-10300-7
47. Cha JH, Yang WH, Xia W, Wei Y, Chan LC, Lim S O, et al. Metformin promotes antitumor immunity via endoplasmic-reticulum-associated degradation of PD-L1. Mol Cell. (2018) 71:606–620 e607. doi: 10.1016/j.molcel.2018.07.030
48. Cao Y, Wo M, Xu C, Fei X, Jin J, Shan Z. An AMPK agonist suppresses the progress of colorectal cancer by regulating the polarization of TAM to M1 through inhibition of HIF-1alpha and mTOR signal pathway. J Cancer Res Ther. (2023) 19:1560–7. doi: 10.4103/jcrt.jcrt_2670_22
49. Song CW, Kim H, Cho H, Kim MS, Paek SH, Park H J, et al. HIF-1alpha inhibition improves anti-tumor immunity and promotes the efficacy of stereotactic ablative radiotherapy (SABR). Cancers (Basel). (2022) 14:3273. doi: 10.3390/cancers14133273
50. Wang J, Lin J, Guo H, Wu W, Yang J, Mao J, et al. Prognostic impact of metformin in solid cancer patients receiving immune checkpoint inhibitors: novel evidences from a multicenter retrospective study. Front Pharmacol. (2024) 15:1419498. doi: 10.3389/fphar.2024.1419498
51. Liu B, Liang BB, Cao WD, Su XX, Cao Q, Mao ZW. Platinum-metformin conjugates acting as promising PD-L1 inhibitors through the AMP-activated protein kinase mediated lysosomal degradation pathway. Angew Chem Int Ed Engl. (2024) 63:e202410586. doi: 10.1002/anie.202410586
52. Yi L, Jiang X, Zhou Z, Xiong W, Xue F, Liu Y, et al. A hybrid nanoadjuvant simultaneously depresses PD-L1/TGF-beta1 and activates cGAS-STING pathway to overcome radio-immunotherapy resistance. Adv Mater. (2024) 36:e2304328. doi: 10.1002/adma.202304328
53. Yang J, Zhang C, Chen X, Zhou D, Sun Z, Niu R, et al. Ultra-efficient radio-immunotherapy for reprogramming the hypoxic and immunosuppressive tumor microenvironment with durable innate immune memory. Biomaterials. (2023) 302:122303. doi: 10.1016/j.biomaterials.2023.122303
54. Zhao Y, Lv B, Xue G, Sun Y, Cao J. Smart nanosystem-mediated inhibition of mitochondrial respiration for enhanced phototherapy-induced antitumor immunity. Int J Nanomed. (2023) 18:3443–57. doi: 10.2147/IJN.S413204
55. Wang T, Chen S, Sun J, Li K. Functional co-delivery nanoliposomes based on improving hypoxia for increasing photoimmunotherapy efficacy of cold tumors. Int J Pharm. (2024) 663:124581. doi: 10.1016/j.ijpharm.2024.124581
56. Dou Y, Zheng J, Kang J, Wang L, Huang D, Liu Y, et al. Mesoporous manganese nanocarrier target delivery metformin for the co-activation STING pathway to overcome immunotherapy resistance. iScience. (2024) 27:110150. doi: 10.1016/j.isci.2024.110150
57. Tian H, Li W, Wang G, Tian Y, Yan J, Zhou S, et al. Self-degradable nanogels reshape immunosuppressive tumor microenvironment via drug repurposing strategy to reactivate cytotoxic CD8(+) T cells. Adv Sci (Weinh). (2023) 10:e2301661. doi: 10.1002/advs.202301661
58. Tang L, Fu C, Liu H, Yin Y, Cao Y, Feng J, et al. Chemoimmunotherapeutic nanogel for pre- and postsurgical treatment of Malignant melanoma by reprogramming tumor-associated macrophages. Nano Lett. (2024) 24:1717–28. doi: 10.1021/acs.nanolett.3c04563
59. Chong L, Jiang YW, Wang D, Chang P, Xu K, Li J. Targeting and repolarizing M2-like tumor-associated macrophage-mediated MR imaging and tumor immunotherapy by biomimetic nanoparticles. J Nanobiotechnol. (2023) 21:401. doi: 10.1186/s12951-023-02122-8
60. Rajeev D, Maciver NJ. Metformin as a therapeutic agent for obesity-associated immune dysfunction. J Nutr. (2024) 154:2534–42. doi: 10.1016/j.tjnut.2024.07.001
61. Halfon M, Tankeu AT, Ribi C. Mitochondrial dysfunction in systemic lupus erythematosus with a focus on lupus nephritis. Int J Mol Sci. (2024) 25:6162. doi: 10.3390/ijms25116162
62. Sumikawa MH, Iwata S, Zhang M, Miyata H, Ueno M, Todoroki Y, et al. An enhanced mitochondrial function through glutamine metabolism in plasmablast differentiation in systemic lupus erythematosus. Rheumatol (Oxford). (2022) 61:3049–59. doi: 10.1093/rheumatology/keab824
63. Nascimento Da Conceicao V, Sun Y, Chai X, Ambrus JL, Mishra BB, Singh BB. Metformin-induced activation of Ca(2+) signaling prevents immune infiltration/pathology in Sjogren’s syndrome-prone mouse models. J Transl Autoimmun. (2023) 7:100210. doi: 10.1016/j.jtauto.2023.100210
64. Fert A, Richard J, Raymond Marchand L, Planas D, Routy JP, Chomont N, et al. Metformin facilitates viral reservoir reactivation and their recognition by anti-HIV-1 envelope antibodies. iScience. (2024) 27:110670. doi: 10.1016/j.isci.2024.110670
65. Martin DE, Cadar AN, Bartley JM. Old drug, new tricks: the utility of metformin in infection and vaccination responses to influenza and SARS-CoV-2 in older adults. Front Aging. (2023) 4:1272336. doi: 10.3389/fragi.2023.1272336
66. Fan S, Zhang C, Sun X, Su C, Xue Y, Song X, et al. Metformin enhances osteogenic differentiation of BMSC by modulating macrophage M2 polarization. Sci Rep. (2024) 14:20267. doi: 10.1038/s41598-024-71318-1
67. Gonzalez-Muniz OE, Rodriguez-Carlos A, Santos-Mena A, Jacobo-Delgado YM, Gonzalez-Curiel I, Rivas-Santiago C, et al. Metformin modulates corticosteroids hormones in adrenals cells promoting Mycobacterium tuberculosis elimination in human macrophages. Tubercul (Edinb). (2024) 148:102548. doi: 10.1016/j.tube.2024.102548
68. Pavan Kumar N, Padmapriyadarsini C, Nancy A, Tamizhselvan M, Mohan A, Reddy D, et al. Effect of Metformin on systemic chemokine responses during anti-tuberculosis chemotherapy. Tubercul (Edinb). (2024) 148:102523. doi: 10.1016/j.tube.2024.102523
69. Yang J, Liu HC, Zhang JQ, Zou JY, Zhang X, Chen W M, et al. The effect of metformin on senescence of T lymphocytes. Immun Ageing. (2023) 20:73. doi: 10.1186/s12979-023-00394-0
70. Ryssdal M, Vanky E, Stokkeland LMT, Jarmund AH, Steinkjer B, Lovvik T S, et al. Immunomodulatory effects of metformin treatment in pregnant women with PCOS. J Clin Endocrinol Metab. (2023) 108:e743–53. doi: 10.1210/clinem/dgad145
71. Petrasca A, Hambly R, Kearney N, Smith CM, Pender EK, Mac Mahon J, et al. Metformin has anti-inflammatory effects and induces immunometabolic reprogramming via multiple mechanisms in hidradenitis suppurativa. Br J Dermatol. (2023) 189:730–40. doi: 10.1093/bjd/ljad305
72. Sorrenti V, Benedetti F, Buriani A, Fortinguerra S, Caudullo G, Davinelli S, et al. Immunomodulatory and antiaging mechanisms of resveratrol, rapamycin, and metformin: focus on mTOR and AMPK signaling networks. Pharm (Basel). (2022) 15:912. doi: 10.3390/ph15080912
73. Wang Z, Wang M, Lin M, Wei P. The immunomodulatory effects of metformin in LPS-induced macrophages: an in vitro study. Inflammation Res. (2024) 73:175–81. doi: 10.1007/s00011-023-01827-8
74. Nassif RM, Chalhoub E, Chedid P, Hurtado-Nedelec M, Raya E, Dang P M, et al. Metformin inhibits ROS production by human M2 macrophages via the activation of AMPK. Biomedicines. (2022) 10:319. doi: 10.3390/biomedicines10020319
75. Hartwig J, Loebel M, Steiner S, Bauer S, Karadeniz Z, Roeger C, et al. Metformin attenuates ROS via FOXO3 activation in immune cells. Front Immunol. (2021) 12:581799. doi: 10.3389/fimmu.2021.581799
76. Ali A, Shaheen S, Imran MZ, Memon Z, Zahid N, Ahmad F, et al. Modulation of altered immune parameters IL-2 and TNF-alpha in diabetic animal models: A therapeutic insinuation of metformin beyond diabetes. Cureus. (2023) 15:e45216. doi: 10.7759/cureus.45216
77. Son HJ, Lee J, Lee SY, Kim EK, Park MJ, Kim K W, et al. Metformin attenuates experimental autoimmune arthritis through reciprocal regulation of Th17/Treg balance and osteoclastogenesis. Mediators Inflammation. (2014) 2014:973986. doi: 10.1155/2014/973986
78. Briviba M, Silamikele L, Kalnina I, Silamikelis I, Birzniece L, Ansone L, et al. Metformin targets intestinal immune system signaling pathways in a high-fat diet-induced mouse model of obesity and insulin resistance. Front Endocrinol (Lausanne). (2023) 14:1232143. doi: 10.3389/fendo.2023.1232143
79. Zhao X, Liu C, Peng L, Wang H. Metformin facilitates anti-PD-L1 efficacy through the regulation of intestinal microbiota. Genes Immun. (2024) 25:7–13. doi: 10.1038/s41435-023-00234-7
Keywords: metformin, immunomodulator, tumor immune microenvironment, immune checkpoint inhibition, aging, inflammation, bibliometric analysis
Citation: Zhou T, Yu Y, Li L, Liu X, Xiang Q and Yu R (2025) Bibliometric analysis of metformin as an immunomodulator (2013–2024). Front. Immunol. 15:1526481. doi: 10.3389/fimmu.2024.1526481
Received: 11 November 2024; Accepted: 13 December 2024;
Published: 08 January 2025.
Edited by:
Qingyu Luo, Dana–Farber Cancer Institute, United StatesReviewed by:
Xiaowei Wu, Dana–Farber Cancer Institute, United StatesHaili Ma, Dana–Farber Cancer Institute, United States
Copyright © 2025 Zhou, Yu, Li, Liu, Xiang and Yu. This is an open-access article distributed under the terms of the Creative Commons Attribution License (CC BY). The use, distribution or reproduction in other forums is permitted, provided the original author(s) and the copyright owner(s) are credited and that the original publication in this journal is cited, in accordance with accepted academic practice. No use, distribution or reproduction is permitted which does not comply with these terms.
*Correspondence: Rong Yu, eXVyb25nMTk2OTA1QDE2My5jb20=
†These authors have contributed equally to this work