- 1The First Affiliated Hospital, Guangxi University of Traditional Chinese Medicine, Nanning, China
- 2Trauma Center, The Second Affiliated Hospital of Nanjing Medical University, Nanjing, China
- 3Department of Neurosurgery, Jiangsu Integrated Traditional Chinese and Western Medicine Hospital, Nanjing, China
- 4Graduate school, Youjiang Medical College for Nationalities, Baise, China
- 5Department of Neurology, School of Medicine, University of Pittsburgh, Pittsburgh, PA, United States
Traumatic brain injury (TBI) is a prevalent disease that poses a significant threat to global public health. Digestive dysfunction, as a common complication, is of particular importance to understand its pathogenesis, diagnostic criteria, and relevant treatment strategies. TBI can affect digestive function through inflammatory immune responses, the enteric nervous system, and hormonal levels. Furthermore, TBI can also impact neurologic recovery through bidirectional communication along the brain-gut axis. Therefore, this article aims to summarize the underlying mechanisms and further explore individualized feeding strategies, therapeutic approaches, long-term prognosis for TBI patients, as well as recent advancements in related technologies. Further understanding of the pathogenesis of digestive system dysfunction after TBI on the basis of the interaction of gut-brain axis is conducive to more future therapies to treat TBI and improve the long-term prognosis of patients through improving digestive function, and achieve good clinical efficacy.
Introduction
Traumatic brain injury (TBI) refers to an acquired insult to the brain caused by an external mechanical force, which can lead to temporary or permanent impairment (1). In the United States, it is estimated that there are between 1.7 and 2.0 million cases of traumatic brain injury (TBI) occurring each year, resulting in approximately 5.3 million Americans currently experiencing long-term comorbidities linked to TBI (2). One comorbidity that is often overlooked following neurotrauma is dysregulation of the gastrointestinal (GI) organs associated with nutrient homeostasis (3). Patients suffering from gastrointestinal dysfunction frequently encounter alterations in the mucosal lining of their intestinal tract, an elevation in gut permeability, and irregularities in gut motility (4, 5). Following brain injury, intestinal epithelial cell dysfunction or apoptosismay occur due to ischemia and/or hypoxia, oxidative stress, and inflammatory reaction. These elements can ultimately result in inflammation, ulceration, and perforation. Intestinal epithelium curtains off the contents of the lumen, preventing the invasion of pathogenic antigens. Digestive dysfunction after TBI is also associated with higher mortality and frequent hospitalizations. With the increasing number of studies on the long-term prognosis of TBI patients in recent years, more studies have proved that digestive system disorders after TBI are related to cognitive function, depression, Parkinson’s disease and other diseases after injury. However, it is still a difficult problem to clarify the molecular mechanism of digestive system dysfunction after TBI, which has a crucial role in improving the long-term prognosis of TBI patients.
Epidemiology and incidence of digestive disorders post-TBI
TBI is a chronic disease process characterized by persistent secondary injury processes which can be exacerbated by subsequent challenges (6). Digestive Disorders Post-TBI can also lead to related symptoms, such as gastrointestinal bleeding (7), gastroesophageal reflux (8), and decreased intestinal motility (9). These symptoms are mainly caused by damage to the mucosa of the digestive organs and changes in their movement patterns. One study showed that gastritis was found on esophagogastroduodenoscopy in 91% of patients (10). The gut microbiota, a vast collection of microorganisms within the human digestive tract numbering in the billions, performs a multitude of physiological roles. Disruption of the gut microbiota balance plays a pivotal role in the development of numerous localized and systemic disorders (11). Consequently, the permeability of the intestinal mucosa increases. TBI-induced intestinal permeability can cause the translocation of endotoxins and bacteria in the intestinal tract, further inducing or aggravating the systemic inflammatory response and resulting in multiple organ failure and death.
Types and characteristics of digestive disorders
Recurrent injury to the gastroduodenal mucosa is frequently observed following a severe head trauma and manifests shortly after the incident. The major gastrointestinal changes observed after traumatic brain injury can be summarized in four aspects. Firstly, there is a reduction in blood supply to the gastrointestinal mucosa, leading to stress ulcers and bleeding in the digestive system (10). Secondly, there is a dysfunction in gut motility, resulting in symptoms such as abdominal bloating, diarrhea, delayed gastric emptying, and even intestinal paralysis (8, 12). Thirdly, there is disruption of the gut barrier function which allows bacteria and endotoxins to enter the bloodstream and contribute to systemic inflammatory response syndrome (SIRS) and sepsis. Lastly, there are alterations in nutrient absorption by the intestinal lining which can lead to malnutrition.
Assessment and diagnosis of digestive disorders in TBI patients
At present, there is no unified standard for the diagnosis of digestive dysfunction after craniocerebral injury, which is mainly judged by history, clinical manifestations and related auxiliary examination. The diagnostic criteria for TBI are a clear history of trauma and confirmed as TBI by head CT or MRI. gastrointestinal injury (AGI) diagnosis criteria were evaluated according to the AGI grading system issued by the European Society of Critical Care Medicine in 2012 (13). In Zhang, D’s study, AGI score was applied to evaluate gastrointestinal functional impairment in critically ill patients (14). Although AGI score has shortcomings such as lack of objective indicators, it is still the most comprehensive method for evaluating gastrointestinal dysfunction in critically ill patients at this stage. Therefore, it can also be jointly diagnosed by laboratory and imaging examinations in clinical practice. Gastroparesis due to TBI or after nutritional support is diagnosed by bedside X-ray technique. Early identification and diagnosis of stress Cushing’s ulcer and clear source of gastrointestinal bleeding can be achieved through gastroscopy (15). Hsieh, JS ET al used gastroscopy to identify early ulcers and took endothelin-1 (ET-1), inducible nitric oxide synthase (iNOS) and macrophage inflammatory protein-1α (MIP-1α) from gastric mucosa to assess the degree of injury (16). Due to the increased intestinal permeability after TBI, harmful substances in the intestine may lead to further digestive dysfunction and even systemic inflammatory response. Hang, CH et al. Assessed intestinal barrier dysfunction by measuring the ratio of lactulose to mannitol (L/M) to determine serum endotoxin levels. Changes in fecal flora can also be used to indicate abnormal digestive system function. In the study of Nicholson, SE et al., the abundance of beneficial microbial phyla (e.g. Firmicteta) decreased when TBI patients were admitted, and opportunistic phyla (e.g. Proteobacteria) also decreased (p < 0.05) (17).
The routine use of dehydrating agents such as mannitol, anesthetic sedatives, and mild hypothermia in the treatment of the majority of TBI patients inevitably heightens the risk of developing digestive disorders (18). Moreover, studies substantiate that the prolonged application of mild hypothermia not only fails to mitigate the onset of secondary brain injuries following TBI but may also trigger gastrointestinal complications including dysfunction (19). Adike, A. et al. found in their research that gastrointestinal motility disturbances represent prevalent complications among patients in critical condition, significantly contributing to mortality rates. These complications frequently correlate with sepsis, mechanical ventilation, and the administration of vasopressors, opioids, or anticholinergic medications (20). In addition, treatment measures such as mechanical ventilation and the use of vasopressors also increase the risk of gastrointestinal bleeding and intestinal dysfunction (21).
Mechanisms and pathophysiology of digestive dysfunction after TBI
Digestive disorders caused by TBI are mostly affected by the gut-brain Axis (GBA), a complex biphasic information exchange network between the Brain and the Gut (22).
The gut-brain axis signaling pathway regulates digestive system function after TBI through the interaction of vagus nerve, intestinal microbiota, immune cell response, and neuroendocrine pathways (Figure 1).
(1) ENS(Enteric nervous system) can combine the unbalanced state of the sympathetic nerve and vagus nerve after TBI to further cause gastrointestinal damage (23). One of the main components of ENS ‘foreign nervous system is the vagus nerve,90% of which extends from the gut to the brain. There are a large number of dopamine neurons in the human digestive tract. The release of dopamine after TBI leads to sympathetic nerve excitation, and the inhibition of vagus nerve leads to digestive tract injury. Bansal, V et al. also confirmed that stimulation of the vagus nerve prevented TBI-induced increase in intestinal permeability in a mouse model of TBI by increasing the activity of glial cells (24).
(2) Changes in intestinal permeability and inflammatory response: TBI blood-brain barrier is destroyed, peripheral white blood cells are infiltrated (25), and immune responses are initiated in glial cells through cytokines, proteases and reactive oxygen species (26), leading to intestinal barrier disruption (4). Hang, CH et al. observed by histopathology and electron microscopy that the earliest intestinal mucosal damage occurred within 3 hours after TBI and lasted for 7 days (5). Results from Cannon AR et al. showed that, compared with healthy controls, TBI patients showed elevated levels of the systemic inflammatory cytokines IFN-γ and MCP-1, as well as elevated levels of IL-6 and IL-8 (p = 0.0551 and p = 0.0549) and the anti-inflammatory cytokine IL-4 Also decreased causing intestinal dysfunction resulting in increased tumor necrosis factor-α (TNF-α) in the gut and decreased bowel movement (27), This may be related to the fact that TNF-α not only induces damage to the close-knit structure of cells but also affects digestive system function by affecting the smooth muscle movement of the small intestine (28, 29). Research indicates that IL-13 is upregulated in human brain tissue and cerebrospinal fluid (CSF) within the first 24 hours following traumatic brain injury (TBI). A comparable increase in IL-13 and its receptor IL-13 Ra1 was observed in mouse models of TBI within 3 hours post-injury (30, 31).
(3) Changes of intestinal flora and hormone levels: With the increase of intestinal permeability and the damage of intestinal mucosa, intestinal flora also began to change, and the changes, translocations and diversity disorders of intestinal flora (reducing symbiotic bacteria and increasing the presence of pathogenic bacteria) induced a series of inflammation and immune responses by disrupting the bidirectional brain-gut axis balance, thus further aggravating gastrointestinal injury (32). It can even cause systemic inflammatory response syndrome (SIRS) (33). Cannon AR’s study also found that TBI significantly increased the number of copies of the patient’s potential pathogen Bilophila wadsworthia, thereby affecting the expression of inflammatory factors affecting the patient’s digestive function. At the same time, intestinal flora and many of its metabolites can lead to rapid and long-term inflammatory response through the secretion of pro-inflammatory lipopolysaccharides, further aggravating digestive disorders and poor prognosis (34, 35). TBI causes elevated hormone levels and can lead to long-term endocrine dysfunction. A multicenter prospective study by Chen, X et al. noted differences in the incidence of gastrointestinal bleeding and 28-day mortality associated with corticosteroids during the subacute phase of TBI (36). The HPA axis and GBA axis can also affect the secretion of gastrointestinal hormones such as somatostatin, further leading to digestive system dysfunction. Future studies are needed to further explore these mechanisms, especially the interactions at the molecular level, to provide new targets for the treatment of TBI-related digestive disorders.
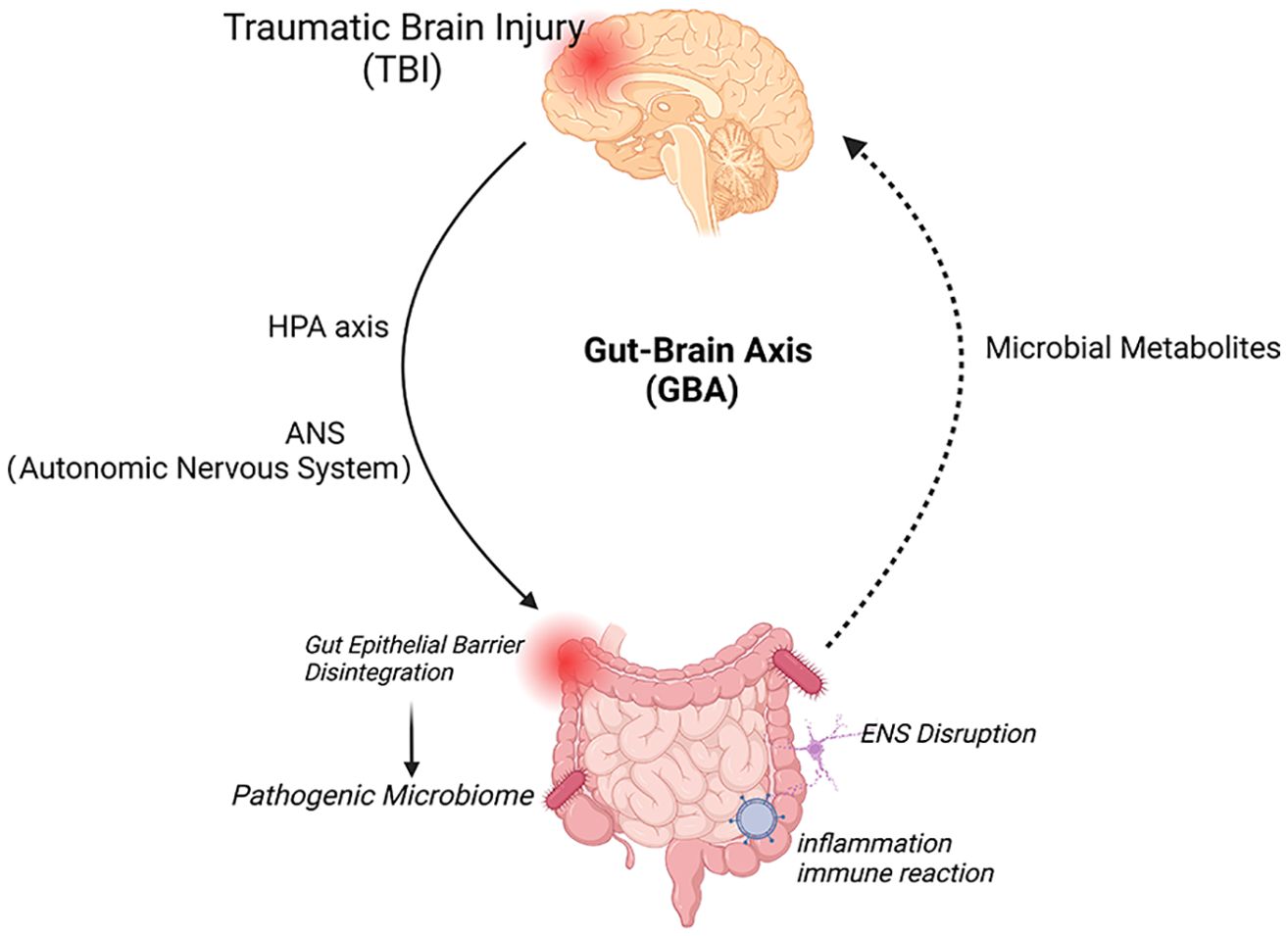
Figure 1. Bidirectional interactions of the brain-gut axis and therapeutic targets. The brain and gut communicate through direct (neurological) and indirect (systemic) two-way pathways. The brain, through the HPA axis and ANS (sympathetic and vagus efferent nerves), causes damage to the intestinal mucosa, including mechanical stimulation, bacterial displacement and other signals from the intestine, induces activation of local and systemic immune responses through ENS neurons, immune and endocrine mechanisms, increases the permeability of the blood-brain barrier and deteriorates the nervous system prognosis. Created with BioRender.com.
Management and treatment approaches for TBI-related digestive disorders
After TBI, due to various complex pathophysiological mechanisms, gastrointestinal motor dysfunction will lead to gastrointestinal symptoms such as vomiting, abdominal distension, diarrhea, gastric retention, and even toxic intestinal paralysis. Improving gastrointestinal motivity is the main direction of drug therapy. At present, the drugs used to improve gastrointestinal motivity mainly include metoclopramide, domperidone and Cisapride (37). Both metoclopramide and domperidone are dopamine receptor blockers, which can affect the central neuroendocrine function at the same time, but cisapride has no effect on neuroendocrine. However, with the extensive use of cisapride, problems such as prolonged Q-T interval and torsion-tip ventricular tachycardia can occur, and it is now replaced by the safer fourth-generation gastroenterokinetic drug moxapride. Komura, M., showed that mosapride reduced the incidence of gastroesophageal reflux in patients with neurologic impairment (38). In addition, there are a number of newly developed gastrointestinal motility drugs on the rise,
In recent years, Elmokadem, EM et al. proposed that the ratio of itopride to metoclopramide group could significantly increase the proportion of enteral nutritional feed, calories and protein in patients after one week (p = 0.001), (p = 0.002), (p = 0.01) (39). Whether erythromycin derivative therapy enhances gastrointestinal motility remains controversial (40, 41). In addition to gastrointestinal motivity drugs, it is also important to protect gastric mucosa, representative drugs are mainly omeprazole (OM), OM can effectively reduce the severity of stress ulcer in TBI patients by reducing the expression rate of ET-1 in tissues, iNOS and MIP-1alpha activity (16). Zhao, ZL study showed that TBI patients taking short-term high doses of glucocorticoids had an increased risk of death and a clear trend of clinical improvement compared with patients taking long-term low doses of glucocorticoids (42, 43). Ozay, R’s study also confirmed that omeprazole and methylprednisolone were equally effective in protecting the brain from oxidative stress and early apoptosis of TBI cells (44). The study by Palm, Nicole M, et al. suggests that trauma patients at risk of stress gastropathy can discontinue preventive medications once they tolerate enteral nutrition (45), so early enteral nutrition is a top priority for TBI patients.
TBI patients are generally at risk of poor eating or disorder, high energy consumption, digestive system dysfunction, high aspiration risk, abnormal glucose and lipid metabolism, intestinal flora disorders, and poor gastrointestinal tolerance, etc. Poor nutrition management will increase the risk of poor prognosis (46). Yang, L’s study pointed out that nutritional support for TBI patients can effectively shorten the length of hospital stay, reduce the infection rate, and play a positive role in promoting the rehabilitation of TBI patients (47). Parenteral nutrition (PN) and enteral nutrition (EN) are two methods mainly used for nutritional support. Qin, Y et al. found that the use of PN was more likely to induce peptic ulcer by comparing EN and PN. Chiang, YH pointed out that compared with EN patients, the risk ratio of non-EN TBI patients was 14.63 (95% CI 8.58-24.91), which may be similar to that of EN patients (48). This may be related to the fact that EN can increase gastrointestinal blood flow, restore nitrogen balance in the body, repair intestinal mucosal barrier structure, improve gastrointestinal motivity, reduce intestinal flora and endotoxin translocation, and improve immune function (49). Therefore, in recent years, EN, especially early EN, has attracted more and more attention due to its advantages of simplicity, convenience, low economic cost, and good effect and benefit, and has become the first choice for nutritional support for TBI patients (50, 51). Early EN refers to enteral nutrition treatment carried out within 24-48h of admission, and EEN is also recommended in ESICM Clinical Practice Guidelines published in 2017 (52). The study of Chourdakis, M showed that compared with delayed enteral nutrition, early EN can have a beneficial effect on hormone levels in patients with TBI, thereby improving prognosis (53).
Interdisciplinary collaboration has been shown to have a positive impact on the recovery of gastrointestinal function in patients with TBI. According to a number of studies, combined intervention using multidisciplinary teams (including neurology, rehabilitation, and nutrition) can significantly improve gastrointestinal dysfunction in patients with TBI, reduce the incidence of gastrointestinal complications, and improve overall survival. The Department of Nutrition can maximize the recovery of intestinal barrier function, optimize immune function, reduce inflammatory response, and improve overall prognosis by adopting a personalized feeding regimen in enteral nutrition. Studies have shown that personalized enteral nutrition programs reduce the incidence of gastrointestinal complications and shorten the length of hospital stay in patients with TBI (54).
The addition of various nutrients to enteral nutrition is also particularly important in improving digestive function in TBI patients (55, 56). In the study of Zhang, X, adding probiotics to enteral nutrition to treat TBI rats found that it could reduce the expression of dopamine receptor (DRs) in intestinal mucosa and prevent damage to intestinal mucosal barrier (IMB) (57). Sun, B et al. used Lactobacillus acidophilus to restore damaged Cajal interstitial cells (ICC) and damaged ICC network in TBI mice to prevent digestive disorders such as TBI-mediated inhibition of intestinal smooth muscle contractions (58). The Yi LJ study showed that supplementing EN with probiotics in early enteral nutrition effectively reduced the risk of infection, mortality, and gastrointestinal complications (59). Karakayal, EM et al. significantly improved outcomes in rats by reducing oxidative stress, apoptosis and gliosis and increasing vascular distribution through probiotic treatment (60). Cui yang et al. can induce immune tolerance by increasing Treg differentiation through oral brain protein combined with probiotics, thereby reducing secondary inflammatory injury after craniocerebral injury (61). Studies have shown that adding omega-3 fatty acids, curcumin, resveratrol, apigenin, vitamins and minerals to enteral nutrition can repair intestinal function, and ketogenic diet can improve the prognosis of patients with TBI (62). The addition of dietary fiber (DF) in enteral nutrition can produce short chainratty acids (SCFAs) under the action of human intestinal flora, thus improving digestive tract symptoms such as constipation and diarrhea in patients (63). The study of Yagmurdur, H also supports that dietary fiber should be increased while EN. Kurtz, P showed that multimodal monitoring of nutrition therapy, blood glucose control and brain microdialysis (CMD) could be used as an integrated approach to better manage TBI patients during enteral nutrition and diet adjustment.
After TBI, the brain stem, cerebellum and thalamus are damaged or intracranial high pressure leads to the inability to complete swallowing normally, resulting in swallowing disorder. In addition, dysconsciousness and cognitive decline in TBI patients may also affect swallowing function. The characteristics of traditional food do not fully meet the requirements of swallowing disorder patients, cannot cooperate with swallowing related rehabilitation training, daily meals are easy to cause coughing and aspiration. Therefore, relevant studies suggest that food traits can be changed to establish a safe and scientific diet training suitable for patients with dysphagia (64). At present, the main mainstream dysphagia training program is the International Dysphagia Diet Standardization Initiative (IDDSI), which was formulated in 2015 for thickening liquid food and daily rehabilitation training for patients with dysphagia disorders (65). Su, M et al. confirmed the feasibility of IDDSI framework in clinical and bedside applications by conducting a trial on 26 patients with dysphagia (66). An, S et al. further elaborated that the type of thickening thing seems to have more influence on the recovery of swallowing function (67). In addition to thickening liquid, some studies have pointed out that the recovery of swallowing function is also affected by the taste of sour, sweet, bitter and hot (68).
The joint rehabilitation department can improve the prognosis of patients through rehabilitation. Xing, X et al. used electroacupuncture to treat TBI patients and found that GIF, D-lactic acid (D-lac), diamine oxidase (DAO), lipolysaccharide (LPS), IAP and abdominal circumference were all lower in the acupuncture group at day 7, and compared with day 1 and day 3, The changes of the above indexes were similar (all p < 0.05). Therefore, early electroacupuncture treatment can improve digestive disorders in TBI patients. Therefore, patients with TBI need to work together with neurologists, gastroenterologists, rehabilitation doctors, dietitians, psychiatrists, etc. It is also very important for the neurosurgery department to evaluate whether early enteral nutrition can be implemented, the nutrition department to provide personalized nutritional meals, and the rehabilitation department to cooperate with the swallowing function and other rehabilitation exercise psychiatrists to pay attention to the psychological state of patients and provide necessary psychological support, so as to jointly achieve the goal of helping patients to obtain the best treatment effect and quality of life (48, 69).Therefore, promoting collaboration among interdisciplinary teams will be an integral part of TBI therapy in the future.
Prognosis and long-term outcomes of digestive disorders post-TBI
The recovery of digestive system after TBI is affected by various factors, the first of which is individual differences. Weijun Fu’s study showed that female, severe GCS score, frontal injury, abnormal blood sodium, pulmonary infection, and intracranial infection are risk factors for recovery of digestive system disorders. Digestive dysfunction in people with TBI is not a short-term consequence, but rather a long-term one, and increases in incidence and severity over time (70). The majority of patients admitted to TBI for one year of rehabilitation services detected H. pylori infection. A 1-year follow-up of TBI with digestive disease found that the mortality rate was about 3 times higher than that of normal patients (71). Lower digestive tract dysfunction is a long-term and serious complication after TBI. The strong and rapid inflammatory response after TBI is not limited to the brain. With the increase of intestinal permeability, harmful substances such as intestinal bacteria enter the blood and lymphatic circulation, which can induce systemic inflammatory response and even threaten life. Mercado, NM et al., through a mouse model, found that TBI can increase systemic inflammatory response through peripheral blood albumin levels (72). Faries, PL et al. found that changes in intestinal permeability after severe trauma can further affect systemic inflammatory response syndrome (SIRS) and multiple organ failure (MOF) in patients (73). Finally, it is very likely that TBI is not a threat to patients’ lives, but the systemic inflammatory response and MODS induced by digestive disorders (74, 75).
On the other hand, digestive disorders can aggravate the original TBI brain injury and even cause more serious chronic neurological damage (76). Therefore, with the continuous improvement of people’s requirements for quality of life, most studies not only focus on early gastrointestinal and digestive disorders after DTI, but also focus on long-term longitudinal studies (77, 78). Celorrio, M., demonstrated in the study that antibiotic exposure 1 week after TBI reduced cortical infiltration of Ly6Chigh monocytes, increased microglial pro-inflammatory markers, and decreased T-lymphocyte infiltration, and that this effect could persist 1 month after injury. At the same time, the dysfunction of gut microbes can seriously aggravate the loss of neurons in the injured hippocampus and reduce the activation of microglia, which is accompanied by changes in the fear memory response (79), which may be related to the occurrence of mental diseases such as depression and cognitive disorders. li beixu et al. retrospectively analyzed 1027 cases of craniocerebral trauma caused by traffic accidents. The severity and area of craniocerebral trauma were closely related to the incidence of organic personality disorders (80). Studies by Chiu, LS et al. indicate that the incidence and severity of TBI is associated with an increased susceptibility to developing neurodegenerative diseases such as Parkinson’s or Alzheimer’s disease (81). So treatment requires long-term care and rehabilitation for the cognitive impairment and other clinical symptoms that people with TBI may experience. Table 1 summarizes animal experiments, clinical studies, and cohort studies on the effects of TBI on digestive system dysfunction.
Current challenges and future directions in TBI-related digestive research
At present, the molecular mechanism of digestive system dysfunction induced by TBI is still not fully understood. In particular, the gut-brain axis (GBA), intestinal flora and systemic inflammation have not been fully explored. In the future, by utilizing high-throughput sequencing, gene-editing techniques, immune response and metabolic pathways, as well as animal models and clinical validation, more can be revealed about the molecular mechanisms by which TBI affects digestive function.
The diagnostic criteria for TBI-related digestive dysfunction are still inconsistent, which brings certain difficulties to clinical diagnosis and treatment. Most of the existing studies on TBI-related digestive dysfunction focus on the early stage after craniocerebral injury. There are still significant challenges in areas such as precision treatment strategies for TBI patients and the collection of long-term clinical data, and by filling these research gaps, we can provide more effective treatment options for TBI patients and mitigate the long-term impact of digestive complications on patient health.
In recent years, more and more studies have begun to focus on the neurological degeneration and psychiatric problems caused by long-term digestive disorders. Long-term follow-up data will help to better understand the natural history and prognosis of this complication. However, in Fang, Y’s study pointed out that the effective new techniques and methods required by IDDSI were not applied in time to patients with feeding difficulties after TBI (82). Therefore, cooperation is still needed in drug therapy, rehabilitation training, nutritional support and other aspects, and clinical application is still facing great challenges. Since the occurrence and presentation of digestive disorders after TBI vary from individual to individual, it is necessary to develop an individualized treatment plan for each patient’s specific situation. First, the individualized nutritional formula has more significant advantages than the conventional nutritional formula for TBI patients, which can reduce inflammation, improve the immune level of patients, improve intestinal mucosal barrier function, and have good gastrointestinal tolerance and low incidence of adverse reactions (83). Secondly, while improving the efficacy of treatment and the satisfaction of patients, individualized treatment helps to improve the self-management ability of patients, so as to participate more actively in the treatment process and better improve the prognosis (84).
Related studies have shown that fecal microbiota transplant-mediated Ghrelin recovery improves neural function after TBI by zhang yamei et al. (85). Berry, JAD et al. improved TBI patients’ ability to recover normal intestinal function and defecation through mesenteric elevation. Young, PJ, et al., reduced in-hospital mortality in TBI patients with selective digestive tract purification (SDD) (86). Traditional Chinese medicine treatment can also be used to effectively improve digestive disorders in TBI patients (87, 88) Wei, C The use of ventilation Huoxuet Decoction (TQHXD) in the treatment of TBI mice showed that TQHXD could significantly improve the differentiation of cluster 36 (CD36)/15-lipoxygenase (15-LO)/nuclear receptor subfamily of 4 group A members 1 (NR4A1) signaling is expressed in mouse colon tissue to improve digestive dysfunction in TBI mice (89). Baudo, G developed in mice a Lipo-Dex (liposome nanocoliths coated with dexamethasone) that selectively targets the injured brain, thereby reducing lesion volume, cell death, astrogliosis, release of pro-inflammatory cytokines, and activation of microglia, thereby improving prognosis (90).
Conclusion
This paper discusses the relevant pathophysiology, diagnostic methods, and management strategies for digestive disorders following TBI. Future research is expected to further clarify diagnostic criteria and pathogenesis, particularly within the context of the GBA. This may offer new insights for clinical treatment of these disorders (91, 92). Effective treatment requires a multidisciplinary approach to develop more personalized plans (35), with clinicians making precise diagnoses based on specific patient symptoms and signs, supplemented by laboratory tests and imaging studies. An individualized treatment plan that includes medication, nutritional support, and rehabilitation training should be developed. Early and accurate assessment of TBI patients is crucial to ensure optimal recovery and minimize complications such as digestive disorders (93). Additionally, ongoing care measures can further support the rehabilitation of TBI patients post-discharge, helping to monitor long-term gastrointestinal dysfunction and other related issues. This is vital for the comprehensive study of TBI patients (94). In summary, future collaborative, multidisciplinary research aimed at addressing this complication could reduce mortality rates in TBI patients and enhance their quality of life.
Author contributions
YL: Writing – original draft. CH: Writing – original draft. CW: Writing – original draft. RC: Writing – original draft. YZ: Writing – original draft. QZ: Writing – original draft. BS: Writing – original draft. YH: Writing – review & editing. SL: Writing – original draft, Writing – review & editing.
Funding
The author(s) declare that financial support was received for the research, authorship, and/or publication of this article. This study was supported by the Research and Translation Application of the Capital’s Unique Clinical Diagnosis and Treatment Technology (Z221100007422024) and the Special Scientific Research Project for Health Development in the Capital (2021zx-08).
Acknowledgments
We thank BioRender.com for the support of the diagram drawing.
Conflict of interest
The authors declare that the research was conducted in the absence of any commercial or financial relationships that could be construed as a potential conflict of interest.
Generative AI statement
The author(s) declare that no Generative AI was used in the creation of this manuscript.
Publisher’s note
All claims expressed in this article are solely those of the authors and do not necessarily represent those of their affiliated organizations, or those of the publisher, the editors and the reviewers. Any product that may be evaluated in this article, or claim that may be made by its manufacturer, is not guaranteed or endorsed by the publisher.
References
1. Capizzi A, Woo J, Verduzco-Gutierrez M. Traumatic brain injury: an overview of epidemiology, pathophysiology, and medical management. Med Clinics North America. (2020) 104:213–38. doi: 10.1016/j.mcna.2019.11.001
2. Huang J. Traumatic brain injury. Neurol Res. (2013) 35:2–221. doi: 10.1179/1743132813Y.0000000178
3. Zhang J, Li Z, Chandrasekar A, Li S, Ludolph A, Boeckers T, et al. Fast maturation of splenic dendritic cells upon TBI is associated with FLT3/FLT3L signaling. Front Immunol. (2022) 13:824459. doi: 10.3389/fimmu.2022.824459
4. Feighery L, Smyth A, Keely S, Baird A, O’Connor W, Callanan J, et al. Increased intestinal permeability in rats subjected to traumatic frontal lobe percussion brain injury. J trauma. (2008) 64:131–7. doi: 10.1097/TA.0b013e3181568d9f
5. Hang C, Shi J, Li J, Wu W, Yin H. Alterations of intestinal mucosa structure and barrier function following traumatic brain injury in rats. World J Gastroenterol. (2003) 9:81–2776. doi: 10.3748/wjg.v9.i12.2776
6. Li S, Qiu N, Ni A, Hamblin M, Yin K. Role of regulatory non-coding RNAs in traumatic brain injury. Neurochem Int. (2024) 172:105643. doi: 10.1016/j.neuint.2023.105643
7. Lu W, Rhoney D, Boling W, Johnson J, Smith T. A review of stress ulcer prophylaxis in the neurosurgical intensive care unit. Neurosurgery. (1997) 41:416–25. doi: 10.1097/00006123-199708000-00017
8. Saxe J, Ledgerwood A, Lucas C, Lucas W. Lower esophageal sphincter dysfunction precludes safe gastric feeding after head injury. J trauma. (1994) 37:581–4. doi: 10.1097/00005373-199410000-00010
9. Pedoto M, O’Dell M, Thrun M, Hollifield D. Superior mesenteric artery syndrome in traumatic brain injury: two cases. Arch Phys Med Rehabil. (1995) 76(9):871–5. doi: 10.1016/S0003-9993(95)80555-9
10. Brown T, Davidson P, Larson G. Acute gastritis occurring within 24 hours of severe head injury. Gastrointest Endosc. (1989) 35:37–40. doi: 10.1016/S0016-5107(89)72683-3
11. Varela-Trinidad G, Dominguez-Diaz C, Solorzano-Castanedo K, Iniguez-Gutierrez L, Hernandez-Flores T, Fafutis-Morris M. Probiotics: protecting our health from the gut. Microorganisms. (2022) 10:1428. doi: 10.3390/microorganisms10071428
12. Kao C, ChangLai S, Chieng P, Yen T. Gastric emptying in head-injured patients. Am J Gastroenterol. (1998) p:12–1108. doi: 10.1111/j.1572-0241.1998.00338.x
13. ReintamBlaser A, Malbrain M, Starkopf J, Fruhwald S, Jakob S, DeWaele J, et al. Gastrointestinal function in intensive care patients: terminology, definitions and management. Recommendations ESICM Working Group Abdominal Problems. Intensive Care Med. (2012) 38:94–384. doi: 10.1007/s00134-011-2459-y
14. Zhang D, Fu R, Li Y, Li H, Li Y, Li H. Comparison of the clinical characteristics and prognosis of primary versus secondary acute gastrointestinal injury in critically ill patients. J Intensive Care. (2017) 5:26. doi: 10.1186/s40560-017-0221-4
15. Kumaria A, Kirkman M, Scott R, Dow G, Leggate A, Macarthur D, et al. A reappraisal of the pathophysiology of cushing ulcer: A narrative review. J Neurosurg Anesthesiol. (2024) 36:211–17. doi: 10.1097/ANA.0000000000000918
16. Hsieh J, Howng S, Huang T, Wang J, Chen F. Endothelin-1, inducible nitric oxide synthase and macrophage inflammatory protein-1alpha in the pathogenesis of stress ulcer in neurotraumatic patients. J trauma. (2006) 61:8–873. doi: 10.1097/01.ta.0000195986.50315.4f
17. Nicholson S, Burmeister D, Johnson T, Zou Y, Lai Z, Scroggins S, et al. A prospective study in severely injured patients reveals an altered gut microbiome is associated with transfusion volume. J Trauma acute Care surgery. (2019) 86:573–82. doi: 10.1097/TA.0000000000002201
18. Herbert M, Holzer P. Standardized concept for the treatment of gastrointestinal dysmotility in critically ill patients–current status and future options. Clin Nutr (Edinburgh Scotland). (2008) 27:25–41. doi: 10.1016/j.clnu.2007.08.001
19. Li Z, Ji X, Zheng T, Zhang Y, Li Z. Effect of long-term and short-term mild hypothermia in severe traumatic brain injury: a comparative study. Zhonghua Wei Zhong Bing Ji Jiu Yi Xue. (2019) 31:1440–44. doi: 10.3760/cma.j.issn.2095-4352.2019.12.002
20. Adike A, Quigley E. Gastrointestinal motility problems in critical care: a clinical perspective. J Dig Dis. (2014) 15:44–335. doi: 10.1111/1751-2980.12147
21. Mutlu G, Mutlu E, Factor P. Prevention and treatment of gastrointestinal complications in patients on mechanical ventilation. Am J Respir medicine: drugs devices other interventions. (2003) 2:395–411. doi: 10.1007/BF03256667
22. Hanscom M, Loane D, Shea-Donohue T. Brain-gut axis dysfunction in the pathogenesis of traumatic brain injury. J Clin Invest. (2021) 131:e143777. doi: 10.1172/JCI143777
23. Purkayastha S, Stokes M, Bell K. Autonomic nervous system dysfunction in mild traumatic brain injury: a review of related pathophysiology and symptoms. Brain Inj. (2019) 33:1129–36. doi: 10.1080/02699052.2019.1631488
24. Bansal V, Costantini T, Ryu S, Peterson C, Loomis W, Putnam J, et al. Stimulating the central nervous system to prevent intestinal dysfunction after traumatic brain injury. J trauma. (2010) 68:64–1059. doi: 10.1097/TA.0b013e3181d87373
25. Zhou C, Li S, Qiu N, Sun P, Hamblin M, Dixon C, et al. Loss of microRNA-15a/16-1 function promotes neuropathological and functional recovery in experimental traumatic brain injury. JCI Insight. (2024) 9:e178650. doi: 10.1172/jci.insight.178650
26. Frohlich A, OldeHeuvel F, Rehman R, Krishnamurthy S, Li S, Li Z, et al. Neuronal nuclear calcium signaling suppression of microglial reactivity is mediated by osteoprotegerin after traumatic brain injury. J Neuroinflammation. (2022) 19:279. doi: 10.1186/s12974-022-02634-4
27. Cannon A, Anderson L, Galicia K, Murray M, Kamran A, Li X, et al. Traumatic brain injury-induced inflammation and gastrointestinal motility dysfunction. Shock (Augusta Ga.). (2023) 59:621–26. doi: 10.1097/SHK.0000000000002082
28. Qi L, Cui X, Dong W, Barrera R, Nicastro J, Coppa G, et al. Ghrelin attenuates brain injury after traumatic brain injury and uncontrolled hemorrhagic shock in rats. Mol Med (Cambridge Mass.). (2012) 18:93–186. doi: 10.2119/molmed.2011.00390
29. Ling H, Li W, Zhou M, Tang Y, Chen Z, Hang C. Expression of intestinal myeloid differentiation primary response protein 88 (Myd88) following experimental traumatic brain injury in a mouse model. ;. (2013) p:e227–34. doi: 10.1016/j.jss.2012.03.030
30. Li S, OldeHeuvel F, Rehman R, Aousji O, Froehlich A, Li Z, et al. Interleukin-13 and its receptor are synaptic proteins involved in plasticity and neuroprotection. Nat Commun. (2023) 14:200. doi: 10.1038/s41467-023-35806-8
31. OldeHeuvel F, Holl S, Chandrasekar A, Li Z, Wang Y, Rehman R, et al. STAT6 mediates the effect of ethanol on neuroinflammatory response in TBI. Brain behavior immunity. (2019) 81:228–46. doi: 10.1016/j.bbi.2019.06.019
32. You W, Zhu Y, Wei A, Du J, Wang Y, Zheng P, et al. Traumatic brain injury induces gastrointestinal dysfunction and dysbiosis of gut microbiota accompanied by alterations of bile acid profile. J Neurotrauma. (2022) 39:227–37. doi: 10.1089/neu.2020.7526
33. Huber-Lang M, Lambris J, Ward P. Innate immune responses to trauma. Nat Immunol. (2018) 19:327–41. doi: 10.1038/s41590-018-0064-8
34. Stephens M, vonderWeid P. Lipopolysaccharides modulate intestinal epithelial permeability and inflammation in a species-specific manner. Gut Microbes. (2020) 11:421–32. doi: 10.1080/19490976.2019.1629235
35. Celorrio M, Shumilov K, Friess S. Gut microbial regulation of innate and adaptive immunity after traumatic brain injury. Neural Regener Res. (2024) 19:272–76. doi: 10.4103/1673-5374.379014
36. Chen X, Chai Y, Wang S, Wang J, Yue S, Jiang R, et al. Risk factors for corticosteroid insufficiency during the sub-acute phase of acute traumatic brain injury. Neural Regener Res. (2020) 15:1259–65. doi: 10.4103/1673-5374.272611
37. Schapira M, Henrion J, Heller F. The current status of gastric prokinetic drugs. Acta Gastroenterol Belg. (1990) 53:57–446.
38. Komura M, Kanamori Y, Tanaka Y, Kodaka T, Sugiyama M, Terawaki K, et al. Mosapride for gastroesophageal reflux disease in neurologically impaired patients. ;. (2017) p:347–51. doi: 10.1111/ped.13144
39. Elmokadem E, ElBorolossy R, Bassiouny A, Hanna M, Darweesh E, Sabri N. The efficacy and safety of itopride in feeding intolerance of critically ill patients receiving enteral nutrition: a randomized, double-blind study. BMC Gastroenterol. (2021) 21:126. doi: 10.1186/s12876-021-01712-w
40. Shaikh N, Nainthramveetil M, Nawaz S, Hassan J, Shible A, Karic E, et al. Optimal dose and duration of enteral erythromycin as a prokinetic: A surgical intensive care experience. Qatar Med J. (2020) 2020:36. doi: 10.5339/qmj.2020.36
41. ElHennawy A, Sparks J, Armentrout D, Huseby V, Berseth C. Erythromycin fails to improve feeding outcome in feeding-intolerant preterm infants. J Pediatr Gastroenterol Nutr. (2003) 37(3):281–6. doi: 10.1097/00005176-200309000-00015
42. Zhao Z, Chen X, Zhu H, Zhang B, Chai Y, Li X, et al. Effects of glucocorticoids on traumatic brain injury related critical illness-related corticosteroid insufficiency. Chin Med J (Engl). (2013) 126:61–3754. doi: 10.3760/cma.j.issn.0366-6999.20130116
43. Alderson P, Roberts I. Corticosteroids in acute traumatic brain injury: systematic review of randomised controlled trials. BMJ (Clinical Res ed.). (1997) 314:9–1855. doi: 10.1136/bmj.314.7098.1855
44. Ozay R, Turkoglu M, Gurer B, Dolgun H, Evirgen O, Erguder B, et al. The protective effect of omeprazole against traumatic brain injury: an experimental study. ;. (2017) p:634–43. doi: 10.1016/j.wneu.2017.04.136
45. Palm N, McKinzie B, Ferguson P, Chapman E, Dorlon M, Eriksson E, et al. Pharmacologic stress gastropathy prophylaxis may not be necessary in at-risk surgical trauma ICU patients tolerating enteral nutrition. J Intensive Care Med. (2018) 33:424–29. doi: 10.1177/0885066616678385
46. Lee H, Oh B. Nutrition management in patients with traumatic brain injury: A narrative review. Brain NeuroRehabilitation. (2022) 15:e4. doi: 10.12786/bn.2022.15.e4
47. Yang L, Liao D, Hou X, Wang Y, Yang C. Systematic review and meta-analysis of the effect of nutritional support on the clinical outcome of patients with traumatic brain injury. Ann Palliat Med. (2021) 10:11960–69. doi: 10.21037/apm-21-3071
48. Chiang Y, Chao D, Chu S, Lin H, Huang S, Yeh Y, et al. Early enteral nutrition and clinical outcomes of severe traumatic brain injury patients in acute stage: a multi-center cohort study. J Neurotrauma. (2012) 29:75–80. doi: 10.1089/neu.2011.1801
49. Araujo-Junqueira L, De-Souza D. Enteral nutrition therapy for critically ill adult patients; critical review and algorithm creation. Nutr Hosp. (2012) 27:999–1008. doi: 10.3305/nh.2012.27.4.5840
50. JustoMeirelles C, deAguilar-Nascimento J. Enteral or parenteral nutrition in traumatic brain injury: a prospective randomised trial. Nutr Hosp. (2011) 26:4–1120. doi: 10.1590/S0212-16112011000500030
51. Yanagawa T, Bunn F, Roberts I, Wentz R, Pierro A. Nutritional support for head-injured patients. Cochrane Database System. Rev. (2002) 2002:CD001530. doi: 10.1002/14651858.CD001530
52. ReintamBlaser A, Starkopf J, Alhazzani W, Berger M, Casaer M, Deane A, et al. Early enteral nutrition in critically ill patients: ESICM clinical practice guidelines. Intensive Care Med. (2017) 43(3):380–98. doi: 10.1007/s00134-016-4665-0
53. Chourdakis M, Kraus M, Tzellos T, Sardeli C, Peftoulidou M, Vassilakos D, et al. Effect of early compared with delayed enteral nutrition on endocrine function in patients with traumatic brain injury: an open-labeled randomized trial. JPEN J Parenter Enteral Nutr. (2012) 36(1):108–16. doi: 10.1177/0148607110397878
54. Wang Y, Wang D, Fu J, Liu X, Liu Y. Clinical observation on application of different enteral nutrition preparations in patients with severe traumatic brain injury. Zhonghua Wei Zhong Bing Ji Jiu Yi Xue. (2019) 31:209–13. doi: 10.3760/cma.j.issn.2095-4352.2019.02.017
55. Aydin S, Ulusoy H, Usul H, Yulug E, Cobanoglu U, Aydin K, et al. Effects of early versus delayed nutrition on intestinal mucosal apoptosis and atrophy after traumatic brain injury. Surg Today. (2005) 35:9–751. doi: 10.1007/s00595-005-3034-3
56. Wan G, Wang L, Zhang G, Zhang J, Lu Y, Li J, et al. Effects of probiotics combined with early enteral nutrition on endothelin-1 and C-reactive protein levels and prognosis in patients with severe traumatic brain injury. J Int Med Res. (2020) 48:300060519888112. doi: 10.1177/0300060519888112
57. Zhang X, Jiang X. Effects of enteral nutrition on the barrier function of the intestinal mucosa and dopamine receptor expression in rats with traumatic brain injury. JPEN. J parenteral enteral Nutr. (2015) 39:23–114. doi: 10.1177/0148607113501881
58. Sun B, Hu C, Fang H, Zhu L, Gao N, Zhu J. The Effects of Lactobacillus acidophilus on the Intestinal Smooth Muscle Contraction through PKC/MLCK/MLC Signaling Pathway in TBI Mouse Model. PloS One. (2015) 10:e0128214. doi: 10.1371/journal.pone.0128214
59. Yi L, Tian X, Shi B, Pi Y, Chen W. Early enteral nutrition supplemented with probiotics improved the clinical outcomes in severe head injury: Some promising findings from Chinese patients. Med (Madr). (2019) 98:e15426. doi: 10.1097/MD.0000000000015426
60. Karakayali E, Kocamaz E, Alpay S, Onal T, Oztatlici M, Durusma R, et al. Histological and electroencephalographic demonstration of probiotic effect for reduce of oxidative stress and apoptosis in experimental traumatic brain injury. Ulusal travma ve acil cerrahi dergisi = Turkish J Trauma Emergency surgery: TJTES. (2023) 29:1203–11. doi: 10.14744/tjtes.2023.80743
61. Cui Y, Xu L, Wang F, Wang Z, Tong X, Yan H. Orally administered brain protein combined with probiotics increases treg differentiation to reduce secondary inflammatory damage following craniocerebral trauma. Front Immunol. (2022) 13:928343. doi: 10.3389/fimmu.2022.928343
62. Lai J, Chen X, Lin S, Chen C, Zheng X. Progress in research on the role of clinical nutrition in treating traumatic brain injury affecting the neurovascular unit. Nutr Rev. (2023) 81:1051–62. doi: 10.1093/nutrit/nuac099
63. Dalile B, VanOudenhove L, Vervliet B, Verbeke K. The role of short-chain fatty acids in microbiota-gut-brain communication. Nat Rev Gastroenterol hepatology. (2019) 16:461–78. doi: 10.1038/s41575-019-0157-3
64. Steele C, Alsanei W, Ayanikalath S, Barbon C, Chen J, Cichero J, et al. The influence of food texture and liquid consistency modification on swallowing physiology and function: a systematic review. Dysphagia. (2015) 30:2–26. doi: 10.1007/s00455-014-9578-x
65. Wong M, Chan K, Wong T, Tang H, Chung H, Kwan H. Quantitative textural and rheological data on different levels of texture-modified food and thickened liquids classified using the international dysphagia diet standardisation initiative (IDDSI) guideline. Foods (Basel Switzerland). (2023) 12:3765. doi: 10.3390/foods12203765
66. Su M, Zheng G, Chen Y, Xie H, Han W, Yang Q, et al. Clinical applications of IDDSI framework for texture recommendation for dysphagia patients. J Texture Stud. (2018) 49:2–10. doi: 10.1111/jtxs.12306
67. An S, Lee W, Yoo B. Comparison of national dysphagia diet and international dysphasia diet standardization initiative levels for thickened drinks prepared with a commercial xanthan gum-based thickener used for patients with dysphagia. Prev Nutr Food science. (2023) 28:83–8. doi: 10.3746/pnf.2023.28.1.83
68. Pena-Chavez R, Schaen-Heacock N, Hitchcock M, Kurosu A, Suzuki R, Hartel R, et al. Effects of food and liquid properties on swallowing physiology and function in adults. Dysphagia. (2023) 38:785–817. doi: 10.1007/s00455-022-10525-2
69. Pelizzari L, Antoniono E, Giraudo D, Ciardi G, Lamberti G. Fecal Incontinence after Severe Brain Injury: A Barrier to Discharge after Inpatient Rehabilitation? Neurol Int. (2023) 15:1339–51. doi: 10.3390/neurolint15040084
70. Shem K, Englander J. Symptomatic Helicobacter pylori infection in a neurorehabilitation population. Arch Phys Med Rehabil. (1998) 79(10):1297–300. doi: 10.1016/S0003-9993(98)90278-7
71. Harrison-Felix C, Whiteneck G, Devivo M, Hammond F, Jha A. Causes of death following 1 year postinjury among individuals with traumatic brain injury. J Head Trauma rehabilitation. (2006) 21:22–33. doi: 10.1097/00001199-200601000-00003
72. Mercado N, Zhang G, Ying Z, Gomez-Pinilla F. Traumatic brain injury alters the gut-derived serotonergic system and associated peripheral organs. Biochim Biophys Acta Mol basis disease. (2022) 1868:166491. doi: 10.1016/j.bbadis.2022.166491
73. Faries P, Simon R, Martella A, Lee M, Machiedo G. Intestinal permeability correlates with severity of injury in trauma patients. J trauma. (1998) 44:1031–5. doi: 10.1097/00005373-199806000-00016
74. Katzenberger R, Ganetzky B, Wassarman D. The gut reaction to traumatic brain injury. Fly (Austin). (2015) 9:68–74. doi: 10.1080/19336934.2015.1085623
75. Sundman M, Chen N, Subbian V, Chou Y. The bidirectional gut-brain-microbiota axis as a potential nexus between traumatic brain injury, inflammation, and disease. Brain behavior immunity. (2017) 66:31–44. doi: 10.1016/j.bbi.2017.05.009
76. Aadal L, Mortensen J, Kellenberger S, Nielsen J. Lower bowel dysfunction following acquired brain injury: A challenge during rehabilitation. Gastroenterol nursing: Off J Soc Gastroenterol Nurses Associates. (2019) 42:12–9. doi: 10.1097/SGA.0000000000000352
77. Urban R, Pyles R, Stewart C, Ajami N, Randolph K, Durham W, et al. Altered fecal microbiome years after traumatic brain injury. J Neurotrauma. (2020) 37:1037–51. doi: 10.1089/neu.2019.6688
78. Sabet N, Soltani Z, Khaksari M. Multipotential and systemic effects of traumatic brain injury. J Neuroimmunol. (2021) 357:577619. doi: 10.1016/j.jneuroim.2021.577619
79. Celorrio M, Abellanas M, Rhodes J, Goodwin V, Moritz J, Vadivelu S, et al. Gut microbial dysbiosis after traumatic brain injury modulates the immune response and impairs neurogenesis. Acta Neuropathol Commun. (2021) 9:40. doi: 10.1186/s40478-021-01137-2
80. Li B, Fang Y, Lin J, Chen X, Li C, He M. Forensic psychiatric analysis of organic personality disorders after craniocerebral injury in Shanghai, China. Front Psychiatry. (2022) 13:944888. doi: 10.3389/fpsyt.2022.944888
81. Chiu L, Anderton R. The role of the microbiota-gut-brain axis in long-term neurodegenerative processes following traumatic brain injury. Eur J Neurosci. (2023) 57:400–18. doi: 10.1111/ejn.15892
82. Fang Y, Ma Y, He H, Chen T, Fu J, Zhu J. Preventive strategies for feeding intolerance among patients with severe traumatic brain injury: A cross-sectional survey. Int J Nurs Sci. (2022) 9:278–85. doi: 10.1016/j.ijnss.2022.06.014
83. Zhu C, Zhang Y, Li W, Li Q. Clinical observation of individualized nutritional formula on inflammation index, immune status and gastrointestinal tolerance in patients with severe head injury. Pak J Med Sci. (2021) 37:952–58. doi: 10.12669/pjms.37.4.3987
84. Clausen T, Bullock R. Medical treatment and neuroprotection in traumatic brain injury. Curr Pharm Des. (2001) 7:32–1517. doi: 10.2174/1381612013397267
85. Zhang Y, Liu J, Liu X, Zhou Y, Geng J, Shi Z, et al. Fecal microbiota transplantation-mediated ghrelin restoration improves neurological functions after traumatic brain injury: evidence from 16S rRNA sequencing and in vivo studies. Mol Neurobiol. (2024) 61:919–34. doi: 10.1007/s12035-023-03595-2
86. Young P, Devaux A, Li Q, Billot L, Davis J, Delaney A, et al. Selective digestive tract decontamination in critically ill adults with acute brain injuries: a post hoc analysis of a randomized clinical trial. Intensive Care Med. (2024) 50:56–67. doi: 10.1007/s00134-023-07261-y
87. Xing X, Jiang R, Lei S, Xu Q, Zhu M, Zhi Y, et al. Electroacupuncture in the treatment of acute gastrointestinal injury in patients with severe traumatic brain injury: a prospective randomized controlled trial. Zhonghua Wei Zhong Bing Ji Jiu Yi Xue. (2021) 33:95–9. doi: 10.3760/cma.j.cn121430-20200804-00562
88. Zhao P, Huang Z, Xu S, Zhang Y. Adjuvant treatment with Angong Niuhuang pills in treating traumatic brain damage: a meta-analysis of randomized controlled trials. Ann Palliat Med. (2021) 10:1569–77. doi: 10.21037/apm-20-1331
89. Wei C, Zhu F, Yu J, Gao F, Yuan Y, Zhang Y, et al. Tongqiao Huoxue Decoction ameliorates traumatic brain injury-induced gastrointestinal dysfunction by regulating CD36/15-LO/NR4A1 signaling, which fails when CD36 and CX3CR1 are deficient. CNS Neurosci Ther. (2023) 29 Suppl 1:161–84. doi: 10.1111/cns.14247
90. Baudo G, Flinn H, Holcomb M, Tiwari A, Soriano S, Taraballi F, et al. Sex-dependent improvement in traumatic brain injury outcomes after liposomal delivery of dexamethasone in mice. Bioeng Transl Med. (2024) 9:e10647. doi: 10.1002/btm2.10647
91. Guangliang H, Tao W, Danxin W, Lei L, Ye M. Critical knowledge gaps and future priorities regarding the intestinal barrier damage after traumatic brain injury. World Neurosurg. (2024) 188:136–49. doi: 10.1016/j.wneu.2024.05.105
92. Du Q, Li Q, Liao G, Li J, Ye P, Zhang Q, et al. Emerging trends and focus of research on the relationship between traumatic brain injury and gut microbiota: a visualized study. Front Microbiol. (2023) 14:1278438. doi: 10.3389/fmicb.2023.1278438
93. Venegoni W, Shen Q, Thimmesch A, Bell M, Hiebert J, Pierce J. The use of antioxidants in the treatment of traumatic brain injury. J Adv Nurs. (2017) 73:1331–38. doi: 10.1111/jan.13259
Keywords: trauma, TBI, post-traumatic syndromes, gut-brain axis, digestive system disorders, neuroinflammatory, enteral nutrition
Citation: Lin Y, Hou C, Wang C, Chen R, Zhu Y, Zhou Q, Shao B, Huang Y and Li S (2024) Research progress on digestive disorders following traumatic brain injury. Front. Immunol. 15:1524495. doi: 10.3389/fimmu.2024.1524495
Received: 07 November 2024; Accepted: 09 December 2024;
Published: 20 December 2024.
Edited by:
Zhuang Sha, The Affiliated Hospital of Xuzhou Medical University, ChinaCopyright © 2024 Lin, Hou, Wang, Chen, Zhu, Zhou, Shao, Huang and Li. This is an open-access article distributed under the terms of the Creative Commons Attribution License (CC BY). The use, distribution or reproduction in other forums is permitted, provided the original author(s) and the copyright owner(s) are credited and that the original publication in this journal is cited, in accordance with accepted academic practice. No use, distribution or reproduction is permitted which does not comply with these terms.
*Correspondence: Shun Li, bGlzMjRAdXBtYy5lZHU=; Yi Huang, NzgzNDgxNDVAcXEuY29t
†These authors have contributed equally to this work