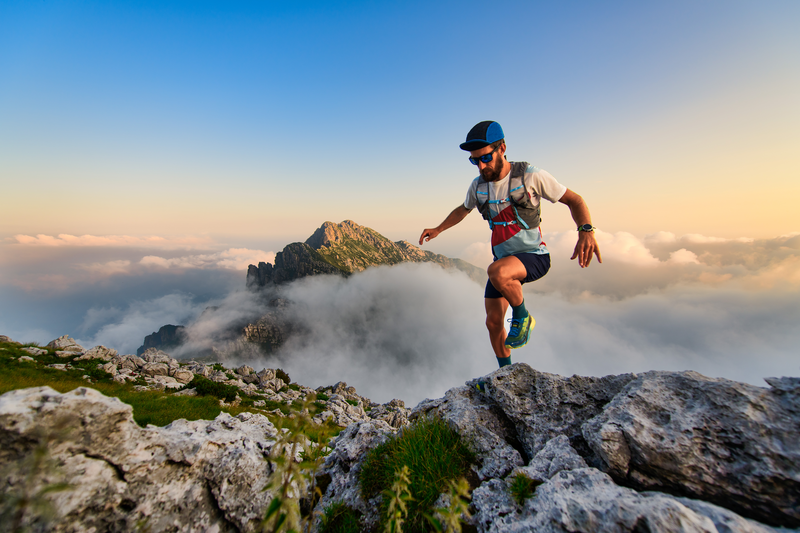
95% of researchers rate our articles as excellent or good
Learn more about the work of our research integrity team to safeguard the quality of each article we publish.
Find out more
OPINION article
Front. Immunol. , 20 December 2024
Sec. Multiple Sclerosis and Neuroimmunology
Volume 15 - 2024 | https://doi.org/10.3389/fimmu.2024.1524432
This article is part of the Research Topic Remedying the injured brain in cognitive impairment: potential neuroimmune communication signaling and therapeutic opportunities View all 5 articles
Alzheimer’s disease (AD) holds global significance as a neurodegenerative disorder with a complex etiology still partly understood. Recent advancements in genetic and molecular research have underscored the intricate interplay of cellular pathways and gene networks, alongside feedforward and feedback regulatory mechanisms, which may differentially impact various pathogenic phenotypes and cellular stages of AD (1). Besides the strategies of neurotransmitter modulator interference and amyloid beta peptide (Aβ)-targeted plaque clearance, neuroinflammatory inhibitors have been widely explored as potential therapeutic approaches for AD, targeting toll-like receptors (TLRs), nuclear factor kappa-light-chain-enhancer of activated B cells (NF-κB), NOD-, LRR- and pyrin domain-containing protein 3 (NLRP3) inflammasome, among others (2–5). Notably, microglia play a pivotal role in clearing Aβ and damaged neuronal cells, crucial for maintaining the balance between pro- and anti-inflammatory processes during neuroinflammation. Their close association with tau phosphorylation, synapse loss, and cognitive decline makes these pathological processes central to AD research (6–11).
MicroRNA (miRNA) modulation of neuroinflammation emerges as a critical regulator in maintaining microglial homeostasis by altering immunoinflammatory-related gene and protein expression. Dysregulation of this process can induce a variety of AD-associated pathological processes, including Aβ metabolism (12, 13), tau phosphorylation (14, 15), neuronal damage (16, 17), and synapse dysfunction (18, 19). The full scope of miRNA mechanisms in regulating neuroinflammation and related disorders remains under exploration. Our research highlights the crucial influence of a newly identified miRNA, miR-25802, on pathological neuroinflammation in AD (20–23). This discussion delves into the role of miR-25802 in AD pathology and its potential as a biomarker and target for future miRNA-based AD therapies (Figure 1).
Figure 1. Role of miR-25802 in AD neuroinflammatory pathology. AD, Alzheimer’s disease; Btk, Bruton tyrosine kinase; CBP/P300, CREB-binding protein/p300; IKKα, IκB kinase α; IKKβ, IκB kinase β; IKKγ, IκB kinase γ; IL-1β, Interleukin-1β; IL-6, Interleukin-6; iNOS, Inducible nitric oxide synthase; IκB, Inhibitor of nuclear factor kappa B; KLF4, Kruppel-like factor 4; MCPIP, Monocyte chemotactic protein-induced protein; TNFα, Tumor necrosis factor-alpha.
The novel base sequences miR-25802 were identified in the AD mouse brain utilizing high-throughput sequencing (20–23). miR-25802 expression is conserved across species and relies on the canonical miRNA enzymes Dicer and Drosha for biogenesis. Notably, significant upregulation of miR-25802 in the plasma of AD patients hints at potential diagnostic utility, and its upregulation in brain regions associated with learning and memory across various AD mouse models suggests a crucial role in AD pathology. The peak upregulation of miR-25802 occurs in AD models from 5 to 7 months, which aligns with the early microglial activation in AD (24).
Subsequent study addresses the functional implications of miR-25802 in AD-related neuroinflammation. First, miR-25802 promotes the activation of pro-inflammatory microglial cells, leading to increased release of pro-inflammatory cytokines such as tumor necrosis factor-alpha (TNF-α), interleukin-6 (IL-6), inducible nitric oxide synthase (iNOS), and interleukin-1-beta (IL-1β), while the inhibition of miR-25802 shifts microglia toward an anti-inflammatory phenotype, characterized by M2 markers such as arginase 1 (Arg1), macrophage mannose receptor 1(CD206), interleukin 4 (IL-4), and transforming growth factor beta (TGF-β), ultimately alleviating the inflammatory response. Second, in vivo functional evidence underscored the significant impact of modulating miR-25802 expression on AD pathology. Overexpression of miR-25802 via adeno-associated virus type 9 (AAV9) in 5×familial AD (5×FAD) mice exacerbated spatial learning and memory abilities, Aβ deposition, and microglial activation. Conversely, inhibiting miR-25802 improved cognitive impairment, reduced Aβ deposition, and attenuated microglial activation in AD mice. These effects of miR-25802 depend on its regulation of Kruppel-like factor 4 (KLF4), a direct target of miR-25802 involved in microglial M1/M2 phenotype conversion and neuroinflammatory responses.
Using bioinformatics, a dual-luciferase reporter assay, gain- and loss-of-function experiments, quantitative real-time polymerase chain reaction (qRT-PCR), and Western blot analyses, KLF4 was identified as a mediator of the effect of miR-25802 on microglia-mediated neuroinflammation in AD, both in vitro and in vivo. Overexpression or inhibition of miR-25802 post-transcriptionally regulated KLF4 expression. Consistently, KLF4 overexpression or silencing in 5×FAD mouse brain reversed the pathological characteristics associated with miR-25802 upregulation or inhibition, including cognitive impairment, Aβ deposition, microglial activation, and inflammatory reactions. KLF4 is a multifunctional transcription factor known to regulate inflammation in the peripheral tissue (25–27). Reports indicate that KLF4 suppresses the polarization of pro-inflammatory M1 macrophages while promoting anti-inflammatory M2 polarization (25, 28, 29). In line with these findings, silencing or overexpression of KLF4 had enhanced or inhibitory effects on the NF-κB cascades. By inhibiting KLF4 in microglia, miR-25802 encouraged microglial differentiation into the pro-inflammatory M1 phenotype, leading to excessive activation of the NF-κB signaling pathway and exacerbating the inflammatory response (20).
NF-κB plays a central role in microglial activation and inflammatory factor release in the brain of AD patients, mediating the downstream neuroinflammatory response triggered by Aβ deposition (30–32). Interestingly, miR-25802 has a genetic affinity with let-7a-2-3p, a member of the let-7 family identified with protective effects in immunoinflammatory disorders like stroke, AD, and multiple sclerosis. Upregulation of let-7 in macrophages promotes an anti-inflammatory phenotype by reducing expression of the transcription factor CCAAT/enhancer binding protein delta (C/ebp-δ) (33). Other inflammation-related targets of let-7 include IL6 and toll-like receptor 4 (Tlr4) (34, 35). NF-κB inhibits let-7-mediated anti-inflammatory actions via inflammatory feedforward loops involving RNA-binding protein Lin28 or IL-6 signaling (36, 37). Combining gene sequence potential analysis with experimental evidence, the involvement of miR-25802/KLF4/NF-κB signaling in microglia-mediated neuroinflammation in AD has been established.
Recent studies have substantiated significant dysregulation of miRNA expression in the body fluids of AD patients (38, 39), which can be predictive of mild cognitive impairment (40), indicating its potential as a promising biomarker for early AD diagnosis. Notably, the emergence of biological miRNA sensing platforms has opened a new avenue for early AD detection, enabling real-time, sensitive detection of miRNAs in body fluids through electrochemical, fluorescence, and surface plasmon resonance biosensing technologies (41, 42). Our previous studies have revealed remarkable downregulations of miR-200a-3p (43) and miR-148a-3p (17) in the blood of AD patients, both playing protective roles in AD. As potential biomarkers, miR-200a-3p and miR-148a-3p have been successfully incorporated into biosensors to facilitate early detection of AD (44–46). In vivo models hypothesize the abnormal upregulation of miR-25802 contributes to neuroinflammation and cognitive deficits in the early stages of AD (20–23). Receiver operation curve analysis in the plasma of AD patients shows that miR-25802 expression levels have high diagnostic efficacy for cognitive dysfunction, suggesting its potential as an AD biomarker. Given the strong correlation between miR-25802 and AD progression, the early development of miR-25802-based diagnostic devices for AD is desirable.
Regarding miRNA-based treatment, preclinical studies have achieved precise gene expression regulation and cognitive improvement through the introduction of exogenous miRNAs using various miRNA delivery approaches, including viral vectors (12), liposomes (47), nanoparticles (48, 49), and exosomes (50). Among these, viral delivery systems, particularly due to their high efficiency in facilitating cellular RNA uptake, have attracted significant attention (51). For example, adeno-associated virus (AAV) type 5 serves as a delivery vector for AMT-130, a miRNA-based therapy currently in phase 2 clinical trials targeting Huntington’s disease (52, 53). Additionally, recombinant AAV9, a highly effective platform for central nervous system delivery, has been approved by the FDA for gene therapy in spinal muscular atrophy (54).
Importantly, our findings demonstrate that lateral ventricular injection of AAV9-encapsulated miR-25802 sponges in 5×FAD mice improved learning and memory abilities (20–23). Conversely, AAV9-coated KLF4 shRNA functioned to suppress the enhancement of learning and memory induced by miR-25802 sponges. This suggests that regulating miR-25802 expression or targeting its associated signaling pathways through AAV9 delivery might offer novel therapeutic approaches for AD, potentially counteracting disease progression and ameliorating cognitive deficits. Besides, the primary challenge in AD treatment remains the development of ncRNA-based therapies capable of effectively crossing the blood-brain barrier (55). Non-viral vectors have been investigated for miRNA-based treatment, particularly by packaging negatively charged miRNAs into liposome nanoparticles. This approach not only protects miRNAs from degradation by endogenous nucleases but also enables cell type-specific targeted delivery (56–58), presenting new opportunities for the development of suitable brain delivery systems for miR-25802.
Microglia-driven neuroinflammation is implicated in both the initiation and progression of AD. Microglia activation serves as a defense mechanism for the brain against detrimental pathogens. However, this activation also incites inflammatory reactions that exacerbate the brain injury. Intriguingly, studies have predicted that more than 50% of mRNAs host miRNAs, allowing them to regulate an array of biological processes, encompassing oxidative stress, inflammation, and apoptosis (59). Specific miRNAs have emerged as potential mediators of neuroinflammation. For instance, miR-155 and miR-223 bind to TLRs to modulate the inflammatory response and influence the production of inflammatory mediators by sponging off suppressors of cytokine signaling in transgenic AD mouse models (60–62). Additionally, miRNAs like miR-146a, miR-873, and miR-34a are involved in neuropathology and neuroinflammation, regulated by NF-ĸB-associated signaling (63–65). Our research indicates that miR-25802, highly expressed in the brain and microglia across various AD models, is a functional RNA molecule devoid of coding capabilities but capable of regulating KLF4 expression and function through NF-κB signaling in neuroinflammation. The let-7 family, consisting of 8–12 members, has been reported to be dysregulated in AD compared to normal controls (66). miR-25802 exhibits high sequence similarity to the let-7 family, whose meta-analysis as biomarkers for early AD detection aligns with our identification of miR-25802 as an inflamma-miRNA based on microglial regulation in AD pathology.
miRNAs may emerge as therapeutic interventions for AD in the future. Currently, ncRNA-based therapies have been developed for complex, intractable diseases such as cardiovascular diseases and cancers (67, 68). In our prior studies, miR-25802 mimics, inhibitors, and sponges were designed based on functional modifications or complementary sequences. miR-25802 mimics, derived from exogenous synthesis and composed of small double-stranded RNA molecules, exacerbated microglial activation via aberrant regulation of the KLF4/NF-κB pathways both in vitro and in vivo. Conversely, miR-25802 inhibitors and sponges shielded anti-inflammatory microglial conversion by restoring KLF4/NF-κB inflammatory signaling in vitro and in vivo, respectively. Furthermore, intracranial injection of these mimics of miR-25802 into wild-type or 5×FAD mice resulted in no significant systemic discomfort or exercise capacity alterations during the one-month observation period. These findings suggest the miR-25802/KLF4/NF-κB pathway as a viable therapeutic target for AD.
Our opinion delves into the biological function, underlying mechanisms, and potential clinical application of miR-25802, an innovative non-coding RNA sequence, within the context of AD. miR-25802 orchestrates the KLF4/NF-κB pathway in microglia-mediated neuroinflammation, facilitating cognitive impairments and Aβ toxicity, highlighting the potential of targeting miR-25802 and its regulatory network as an emerging frontier in AD treatment. Future research on miR-25802 will be further advanced through potential interdisciplinary collaborations, including neuroscience, bioengineering, and clinical medicine. Initially, identifying the drivers of miR-25802 upregulation in AD and other specific mRNA targets using tissue-specific knockout technologies will be crucial. Additionally, clinical research necessitates exploring the diagnostic and differential diagnostic implications of the miR-25802/KLF4 axis across a broader patient cohort. Furthermore, most miRNA-centric diagnostic tools and therapeutic interventions for AD are still in their initial stage. Regarding miR-25802, biological macromolecular inhibitors, employing oligonucleotide analogues as scaffolds, may afford benefits such as high selectivity and efficiency; however, they also pose challenges like immunogenicity and multiple conformations. Alternatively, the development of small molecule inhibitors targeting miR-25802 and its signaling pathway boasts its own strengths, including robust cell membrane penetration, resistance to intracellular enzymatic degradation, and reduced synthesis costs. In summary, achieving a profound understanding of the mechanism of miR-25802 in AD, coupled with the creation of more effective delivery systems or targeted small molecule drugs, will pave the way for translating miR-25802 research from the laboratory to clinical practice.
KZ: Writing – original draft. ZL: Writing – original draft. LZ: Visualization, Writing – original draft. ZC: Visualization, Writing – original draft. RL: Conceptualization, Funding acquisition, Supervision, Writing – review & editing.
The author(s) declare financial support was received for the research, authorship, and/or publication of this article. This work was supported by the National Natural Science Foundation of China (No. 82473904) and High-level Foreign Expert Projects (No. DL2023194004L).
The authors declare that the research was conducted in the absence of any commercial or financial relationships that could be construed as a potential conflict of interest.
The author(s) declared that they were an editorial board member of Frontiers, at the time of submission. This had no impact on the peer review process and the final decision.
The author(s) declare that no Generative AI was used in the creation of this manuscript.
All claims expressed in this article are solely those of the authors and do not necessarily represent those of their affiliated organizations, or those of the publisher, the editors and the reviewers. Any product that may be evaluated in this article, or claim that may be made by its manufacturer, is not guaranteed or endorsed by the publisher.
1. Murdock MH, Tsai LH. Insights into Alzheimer’s disease from single-cell genomic approaches. Nat Neurosci. (2023) 26:181–95. doi: 10.1038/s41593-022-01222-2
2. Cummings JL, Osse AML, Kinney JW. Alzheimer’s disease: novel targets and investigational drugs for disease modification. Drugs. (2023) 83:1387–408. doi: 10.1007/s40265-023-01938-w
3. Rangasamy SB, Jana M, Roy A, Corbett GT, Kundu M, Chandra S, et al. Selective disruption of TLR2-MyD88 interaction inhibits inflammation and attenuates Alzheimer’s pathology. J Clin Invest. (2018) 128:4297–312. doi: 10.1172/JCI96209
4. Sun E, Motolani A, Campos L, Lu T. The pivotal role of NF-kB in the pathogenesis and therapeutics of alzheimer’s disease. Int J Mol Sci. (2022) 23:8972. doi: 10.3390/ijms23168972
5. McManus RM, Latz E. NLRP3 inflammasome signaling in Alzheimer’s disease. Neuropharmacology. (2024) 252:109941. doi: 10.1016/j.neuropharm.2024.109941
6. Arranz AM, De Strooper B. The role of astroglia in Alzheimer’s disease: pathophysiology and clinical implications. Lancet Neurol. (2019) 18:406–14. doi: 10.1016/S1474-4422(18)30490-3.E
7. Ralvenius WT, Andresen JL, Huston MM, Penney J, Bonner JM, Fenton OS, et al. Nanoparticle-Mediated Delivery of Anti-PU.1 siRNA via Localized Intracisternal Administration Reduces Neuroinflammation. Adv Mater. (2024) 36:e2309225. doi: 10.1002/adma.202309225
8. Rego S, Sanchez G, Da Mesquita S. Current views on meningeal lymphatics and immunity in aging and Alzheimer’s disease. Mol Neurodegener. (2023) 18:55. doi: 10.1186/s13024-023-00645-0
9. Agarwal D, Sandor C, Volpato V, Caffrey TM, Monzón-Sandoval J, Bowden R, et al. A single-cell atlas of the human substantia nigra reveals cell-specific pathways associated with neurological disorders. Nat Commun. (2020) 11:4183. doi: 10.1038/s41467-020-17876-0
10. Chen Y, Colonna M. Microglia in Alzheimer’s disease at single-cell level. Are there common patterns in humans and mice? J Exp Med. (2021) 218:e20202717. doi: 10.1084/jem.20202717
11. Li X, Li C, Zhang W, Wang Y, Qian P, Huang H. Inflammation and aging: signaling pathways and intervention therapies. Signal Transduct Target Ther. (2023) 8:239. doi: 10.1038/s41392-023-01502-8
12. Yin Z, Herron S, Silveira S, Kleemann K, Gauthier C, Mallah D, et al. Identification of a protective microglial state mediated by miR-155 and interferon-γ signaling in a mouse model of Alzheimer’s disease. Nat Neurosci. (2023) 26:1196–207. doi: 10.1038/s41593-023-01355-y
13. Sun T, Zhao K, Liu M, Cai Z, Zeng L, Zhang J, et al. miR-30a-5p induces Aβ production via inhibiting the nonamyloidogenic pathway in Alzheimer’s disease. Pharmacol Res. (2022) 178:106153. doi: 10.1016/j.phrs.2022.106153
14. Kodama L, Guzman E, Etchegaray JI, Li Y, Sayed FA, Zhou L, et al. Microglial microRNAs mediate sex-specific responses to tau pathology. Nat Neurosci. (2020) 23:167–71. doi: 10.1038/s41593-019-0560-7
15. Jiang H, Liu J, Guo S, Zeng L, Cai Z, Zhang J, et al. miR-23b-3p rescues cognition in Alzheimer’s disease by reducing tau phosphorylation and apoptosis via GSK-3β signaling pathways. Mol Ther Nucleic Acids. (2022) 28:539–57. doi: 10.1016/j.omtn.2022.04.008
16. Wei H, Zhu Z, Xu Y, Lin L, Chen Q, Liu Y, et al. Microglia-derived exosomes selective sorted by YB-1 alleviate nerve damage and cognitive outcome in Alzheimer’s disease. J Transl Med. (2024) 22:466. doi: 10.1186/s12967-024-05256-x
17. Zeng L, Jiang H, Ashraf GM, Liu J, Wang L, Zhao K, et al. Implications of miR-148a-3p/p35/PTEN signaling in tau hyperphosphorylation and autoregulatory feedforward of Akt/CREB in Alzheimer’s disease. Mol Ther Nucleic Acids. (2022) 27:256–75. doi: 10.1016/j.omtn.2021.11.019
18. Li X, Chen SC, Ip JPK. Diverse and composite roles of miRNA in non-neuronal cells and neuronal synapses in Alzheimer’s disease. Biomolecules. (2022) 12:1505. doi: 10.3390/biom12101505
19. Dalal S, Ramirez-Gomez J, Sharma B, Devara D, Kumar S. MicroRNAs and synapse turnover in Alzheimer’s disease. Ageing Res Rev. (2024) 99:102377. doi: 10.1016/j.arr.2024.102377
20. Zhao K, Liu J, Sun T, Zeng L, Cai Z, Li Z, et al. The miR-25802/KLF4/NF-κB signaling axis regulates microglia-mediated neuroinflammation in Alzheimer’s disease. Brain Behav Immun. (2024) 118:31–48. doi: 10.1016/j.bbi.2024.02.016
21. Liu R, Li Z, Zhao K, Liu M, Zeng L, Sun T, et al. Biomarker miR-25802 cluster for inflammation-related diseases and application of biomarker miR-25802 cluster (2022). Available online at: https://patentscope2.wipo.int/search/zh/detail.jsf?docId=CN397428498 (accessed December 14, 2024).
22. Liu R, Li Z, Zhao K, Liu M, Zeng L. Biomarker Mir-25802 Cluster for inflammation-related diseases, and use thereof. PCT/CN2023/108782 W.O (2024). Available online at: https://patentscope.wipo.int/search/zh/detail.jsf?docId=WO2024012600 (accessed December 14, 2024).
23. Liu R, Li Z, Zhao K, Liu M, Zeng L. Biomarker Mir-25802 Cluster for inflammation-related diseases, and use thereoff (2024). Available online at: https://patentcenter.uspto.gov/applications/18713459?application= (accessed December 14, 2024).
24. Leng F, Edison P. Neuroinflammation and microglial activation in Alzheimer disease: where do we go from here? Nat Rev Neurol. (2021) 17:157–72. doi: 10.1038/s41582-020-00435-y
25. Kapoor N, Niu J, Saad Y, Kumar S, Sirakova T, Becerra E, et al. Transcription factors STAT6 and KLF4 implement macrophage polarization via the dual catalytic powers of MCPIP. J Immunol. (2015) 194:6011–23. doi: 10.4049/jimmunol.1402797
26. Ma C, Xia R, Yang S, Liu L, Zhang J, Feng K, et al. Formononetin attenuates atherosclerosis via regulating interaction between KLF4 and SRA in apoE-/- mice. Theranostics. (2020) 10:1090–106. doi: 10.7150/thno.38115
27. Cao Y, Xiong J, Guan X, Yin S, Chen J, Yuan S, et al. Paeoniflorin suppresses kidney inflammation by regulating macrophage polarization via KLF4-mediated mitophagy. Phytomedicine. (2023) 16:154901. doi: 10.1016/j.phymed.2023.154901
28. Li Z, Martin M, Zhang J, Huang HY, Bai L, Zhang J, et al. Krüppel-like factor 4 regulation of cholesterol-25-hydroxylase and liver X receptor mitigates atherosclerosis susceptibility. Circulation. (2017) 136:1315–30. doi: 10.1161/CIRCULATIONAHA.117.027462
29. Wen Y, Lu X, Ren J, Privratsky JR, Yang B, Rudemiller NP, et al. KLF4 in macrophages attenuates TNFα-mediated kidney injury and fibrosis. J Am Soc Nephrol. (2019) 30:1925–38. doi: 10.1681/ASN.2019020111
30. Lian H, Yang L, Cole A, Sun L, Chiang AC, Fowler SW, et al. NFκB-activated astroglial release of complement C3 compromises neuronal morphology and function associated with Alzheimer’s disease. Neuron. (2015) 85:101–15. doi: 10.1016/j.neuron.2014.11.018
31. Sun J, Zhang Y, Kong Y, Ye T, Yu Q, Kumaran Satyanarayanan S, et al. Microbiota-derived metabolite Indoles induced aryl hydrocarbon receptor activation and inhibited neuroinflammation in APP/PS1 mice. Brain Behav Immun. (2022) 106:76–88. doi: 10.1016/j.bbi.2022.08.003
32. Rahimifard M, Maqbool F, Moeini-Nodeh S, Niaz K, Abdollahi M, Braidy N, et al. Targeting the TLR4 signaling pathway by polyphenols: A novel therapeutic strategy for neuroinflammation. Ageing Res Rev. (2017) 36:11–9. doi: 10.1016/j.arr.2017.02.004
33. Wang L, Liu T, Chen G, Li Y, Zhang S, Mao L, et al. Exosomal microRNA let-7-5p from Taenia pisiformisCysticercus Prompted Macrophage to M2 Polarization through Inhibiting the Expression of C/EBP δ. Microorganisms. (2021) 9:1403. doi: 10.3390/microorganisms9071403
34. Di T, Yang Y, Fu C, Zhang Z, Qin C, Sai X, et al. Let-7 mediated airway remodeling in chronic obstructive pulmonary disease via the regulation of IL-6. Eur J Clin Invest. (2021) 51:e13425. doi: 10.1111/eci.13425
35. Chen XM, Splinter PL, O’Hara SP, LaRusso NF. A cellular micro-RNA, let-7i, regulates Toll-like receptor 4 expression and contributes to cholangiocyte immune responses against Cryptosporidium parvum infection. J Biol Chem. (2007) 282:28929–38. doi: 10.1074/jbc.M702633200
36. Kang M, Lee KH, Lee HS, Jeong CW, Ku JH, Kim HH, et al. Concurrent treatment with simvastatin and NF-κB inhibitor in human castration-resistant prostate cancer cells exerts synergistic anti-cancer effects via control of the NF-κB/LIN28/let-7 miRNA signaling pathway. PloS One. (2017) 12:e0184644. doi: 10.1371/journal.pone.0184644
37. Cao Q, Li YY, He WF, Zhang ZZ, Zhou Q, Liu X, et al. Interplay between microRNAs and the STAT3 signaling pathway in human cancers. Physiol Genomics. (2013) 45:1206–14. doi: 10.1152/physiolgenomics.00122.2013
38. Swarbrick S, Wragg N, Ghosh S, Stolzing A. Systematic review of miRNA as biomarkers in Alzheimer’s disease. Mol Neurobiol. (2019) 56:6156–67. doi: 10.1007/s12035-019-1500-y
39. Han SW, Pyun JM, Bice PJ, Bennett DA, Saykin AJ, Kim SY, et al. miR-129-5p as a biomarker for pathology and cognitive decline in Alzheimer’s disease. Alzheimers Res Ther. (2024) 16:5. doi: 10.1186/s13195-023-01366-8
40. Kumar A, Su Y, Sharma M, Singh S, Kim S, Peavey JJ, et al. MicroRNA expression in extracellular vesicles as a novel blood-based biomarker for Alzheimer’s disease. Alzheimers Dement. (2023) 19:4952–66. doi: 10.1002/alz.13055
41. Pishbin E, Sadri F, Dehghan A, Kiani MJ, Hashemi N, Zare I, et al. Recent advances in isolation and detection of exosomal microRNAs related to Alzheimer’s disease. Environ Res. (2023) 227:115705. doi: 10.1016/j.envres.2023.115705
42. Pereira RL, Oliveira D, Pêgo AP, Santos SD, Moreira FTC. Electrochemical miRNA-34a-based biosensor for the diagnosis of Alzheimer’s disease. Bioelectrochemistry. (2023) 154:108553. doi: 10.1016/j.bioelechem.2023.108553
43. Wang L, Liu J, Wang Q, Jiang H, Zeng L, Li Z, et al. MicroRNA-200a-3p mediates neuroprotection in alzheimer-related deficits and attenuates amyloid-beta overproduction and tau hyperphosphorylation via coregulating BACE1 and PRKACB. Front Pharmacol. (2019) 10:806. doi: 10.3389/fphar.2019.00806
44. Na HK, Wi JS, Son HY, Ok JG, Huh YM, Lee TG. Discrimination of single nucleotide mismatches using a scalable, flexible, and transparent three-dimensional nanostructure-based plasmonic miRNA sensor with high sensitivity. Biosens Bioelectron. (2018) 113:39–45. doi: 10.1016/j.bios.2018.04.033
45. Wang Y, Howes PD, Kim E, Spicer CD, Thomas MR, Lin Y, et al. Duplex-specific nuclease-amplified detection of microRNA using compact quantum dot-DNA conjugates. ACS Appl Mater Interf. (2018) 10:28290–300. doi: 10.1021/acsami.8b07250
46. Park JS, Kim ST, Kim SY, Jo MG, Choi MJ, Kim MO. A novel kit for early diagnosis of Alzheimer’s disease using a fluorescent nanoparticle imaging. Sci Rep. (2019) 9:13184. doi: 10.1038/s41598-019-49711-y
47. Su D, Chen Z, An X, Yang J, Yang J, Wang X, et al. MicroRNA-195 liposomes for therapy of Alzheimer’s disease. J Control Release. (2024) 365:583–601. doi: 10.1016/j.jconrel.2023.12.003
48. Ouyang Q, Liu K, Zhu Q, Deng H, Le Y, Ouyang W, et al. Brain-penetration and neuron-targeting DNA nanoflowers co-delivering miR-124 and rutin for synergistic therapy of Alzheimer’s disease. Small. (2022) 18:e2107534. doi: 10.1002/smll.202107534
49. Israel LL, Sun T, Braubach O, Cox A, Shatalova ES, Rashid HM, et al. [amp]]beta;-Amyloid targeting nanodrug for neuron-specific delivery of nucleic acids in Alzheimer’s disease mouse models. J Control Release. (2023) 361:636–58. doi: 10.1016/j.jconrel.2023.08.001
50. Chen C, Bao Y, Xing L, Jiang C, Guo Y, Tong S, et al. Exosomes derived from M2 microglial cells modulated by 1070-nm light improve cognition in an Alzheimer’s disease mouse model. Adv Sci (Weinh). (2023) 10:e2304025. doi: 10.1002/advs.202304025
51. Gao J, Gunasekar S, Xia ZJ, Shalin K, Jiang C, Chen H, et al. Gene therapy for CNS disorders: modalities, delivery and translational challenges. Nat Rev Neurosci. (2024) 25:553–72. doi: 10.1038/s41583-024-00829-7
52. Estevez-Fraga C, Tabrizi SJ, Wild EJ. Huntington’s disease clinical trials corner: March 2024. J Huntingtons Dis. (2024) 13:1–14. doi: 10.3233/JHD-240017
53. Estevez-Fraga C, Tabrizi SJ, Wild EJ. Huntington’s disease clinical trials corner: November 2022. J Huntingtons Dis. (2022) 11:351–67. doi: 10.3233/JHD-229006
54. Mendell JR, Al-Zaidy S, Shell R, Arnold WD, Rodino-Klapac LR, Prior TW, et al. Single-dose gene-replacement therapy for spinal muscular atrophy. N Engl J Med. (2017) 377:1713–22. doi: 10.1056/NEJMoa1706198
55. Min HS, Kim HJ, Naito M, Ogura S, Toh K, Hayashi K, et al. Systemic brain delivery of antisense oligonucleotides across the blood-brain barrier with a glucose-coated polymeric nanocarrier. Angew Chem Int Ed Engl. (2020) 59:8173–80. doi: 10.1002/anie.201914751
56. Roberts TC, Langer R, Wood MJA. Advances in oligonucleotide drug delivery. Nat Rev Drug Discovery. (2020) 19:673–94. doi: 10.1038/s41573-020-0075-7
57. Blanco E, Shen H, Ferrari M. Principles of nanoparticle design for overcoming biological barriers to drug delivery. Nat Biotechnol. (2015) 33:941–51. doi: 10.1038/nbt.3330
58. Lee SWL, Paoletti C, Campisi M, Osaki T, Adriani G, Kamm RD, et al. MicroRNA delivery through nanoparticles. J Control Release. (2019) 313:80–95. doi: 10.1016/j.jconrel.2019.10.007
59. Mushtaq G, Greig NH, Anwar F, Zamzami MA, Choudhry H, Shaik MM, et al. miRNAs as circulating biomarkers for Alzheimer’s disease and Parkinson’s disease. Med Chem. (2016) 12:217–25. doi: 10.2174/1573406411666151030112140
60. Fabbri M. TLRs as miRNA receptors. Cancer Res. (2012) 72:6333–7. doi: 10.1158/0008-5472.CAN-12-3229
61. Guedes JR, Custódia CM, Silva RJ, de Almeida LP, Pedroso de Lima MC, Cardoso AL. Early miR-155 upregulation contributes to neuroinflammation in Alzheimer’s disease triple transgenic mouse model. Hum Mol Genet. (2014) 23:6286–301. doi: 10.1093/hmg/ddu348
62. Duan R, Wang SY, Wei B, Deng Y, Fu XX, Gong PY, et al. Angiotensin-(1-7) Analogue AVE0991 Modulates Astrocyte-Mediated Neuroinflammation via lncRNA SNHG14/miR-223-3p/NLRP3 Pathway and Offers Neuroprotection in a Transgenic Mouse Model of Alzheimer’s Disease. J Inflammation Res. (2021) 14:7007–19. doi: 10.2147/JIR.S343575
63. Nakano M, Kubota K, Kobayashi E, Chikenji TS, Saito Y, Konari N, et al. Bone marrow-derived mesenchymal stem cells improve cognitive impairment in an Alzheimer’s disease model by increasing the expression of microRNA-146a in hippocampus. Sci Rep. (2020) 10:10772. doi: 10.1038/s41598-020-67460-1
64. Liu X, He F, Pang R, Zhao D, Qiu W, Shan K, et al. Interleukin-17 (IL-17)-induced microRNA 873 (miR-873) contributes to the pathogenesis of experimental autoimmune encephalomyelitis by targeting A20 ubiquitin-editing enzyme. J Biol Chem. (2014) 289:28971–86. doi: 10.1074/jbc.M114.577429
65. Periyasamy P, Thangaraj A, Bendi VS, Buch S. HIV-1 Tat-mediated microglial inflammation involves a novel miRNA-34a-NLRC5-NFκB signaling axis. Brain Behav Immun. (2019) 80:227–37. doi: 10.1016/j.bbi.2019.03.011
66. Cătană CS, Marta MM, Văleanu M, Dican L, Crişan CA. Human leukocyte antigen and microRNAs as key orchestrators of mild cognitive impairment and Alzheimer’s disease: A systematic review. Int J Mol Sci. (2024) 25:8544. doi: 10.3390/ijms25158544
67. Shah AM, Giacca M. Small non-coding RNA therapeutics for cardiovascular disease. Eur Heart J. (2022) 43:4548–61. doi: 10.1093/eurheartj/ehac463
Keywords: Alzheimer’s disease, microRNA-25802, miRNA-based therapy, neuroinflammation, microglia
Citation: Zhao K, Li Z, Zeng L, Cai Z and Liu R (2024) MiR-25802: a potential target for treating Alzheimer’s disease by regulating neuroinflammation. Front. Immunol. 15:1524432. doi: 10.3389/fimmu.2024.1524432
Received: 07 November 2024; Accepted: 10 December 2024;
Published: 20 December 2024.
Edited by:
Davide Cossu, Juntendo University, JapanReviewed by:
Chunmei Liang, Affiliated Hospital of Guangdong Medical University, ChinaCopyright © 2024 Zhao, Li, Zeng, Cai and Liu. This is an open-access article distributed under the terms of the Creative Commons Attribution License (CC BY). The use, distribution or reproduction in other forums is permitted, provided the original author(s) and the copyright owner(s) are credited and that the original publication in this journal is cited, in accordance with accepted academic practice. No use, distribution or reproduction is permitted which does not comply with these terms.
*Correspondence: Rui Liu, bGl1cnVpQGltYi5wdW1jLmVkdS5jbg==
Disclaimer: All claims expressed in this article are solely those of the authors and do not necessarily represent those of their affiliated organizations, or those of the publisher, the editors and the reviewers. Any product that may be evaluated in this article or claim that may be made by its manufacturer is not guaranteed or endorsed by the publisher.
Research integrity at Frontiers
Learn more about the work of our research integrity team to safeguard the quality of each article we publish.