- 1Laboratory of Molecular Therapy of Cancer, Cancer Research Institute, Tomsk National Research Medical Center, Russian Academy of Sciences, Tomsk, Russia
- 2Laboratory of Translational Cellular and Molecular Biomedicine, National Research Tomsk State University, Tomsk, Russia
- 3Laboratory of Molecular Oncology and Immunology, Cancer Research Institute, Tomsk National Research Medical Center, Russian Academy of Sciences, Tomsk, Russia
- 4Department of Gynecology, Cancer Research Institute, Tomsk National Research Medical Center, Russian Academy of Sciences, Tomsk, Russia
Immunotherapy represents a groundbreaking therapeutic approach, based on the immune system’s intrinsic capacity to interfere with tumor progression, that opens the horizons in the treatment of endometrial cancer. However, the clinical efficacy of immunotherapy is hampered by the development of resistance in patients. The resistance to immunotherapy is multifactorial mechanism, encompassed genetic and epigenetic alterations in tumor cells modulating immune checkpoint molecules, resulted in escaping immune surveillance. The tumor microenvironment can orchestrate an immunosuppressive milieu, attenuating the immune response and facilitating tumor progression. To overcome immunotherapeutic resistance in endometrial cancer we must bring to light the mechanisms of intricate interplay between neoplastic cells, the host immune system, and the tumor microenvironment. The identification of predictive biomarkers for immunotherapeutic response and the innovative agents capable of reversing resistance pathways must be developed. Our review summarizes accumulated data on the role of cells of the tumor microenvironment and their regulatory molecules in the mechanisms underlying therapeutic effects of immune checkpoint inhibitors, including resistance to therapy. Major question we raise here – which group of patients is the most favorable to achieve durable immunotherapy response in endometrial cancer?
1 Introduction
Endometrial cancer (EC) represents a significant public health challenge globally. Based on the World Cancer Research Fund International data (2020), EC ranks sixth most prevalent cancer in women and the fifteenth in the whole population. Approximately 417,000 new EC cases and 97,370 related fatalities are revealed annually worldwide (1). There is an observed upward trend in both the incidence and mortality rates associated with EC. By 2044, the incidence of EC is projected to be exceeded 600,000 cases annually, that will account around 48% increase starting from 2019 (2, 3).
Therapeutic interventions in the early-stage EC can be markedly efficacious. The five-year survival rate for patients diagnosed at stage I is approximately 96%, at stage II – between 80-90%. However, the survival rate significantly declined to 20-25% in metastatic EC (4). The conventional regimen for the treatment of advanced stage EC includes a combination therapy with paclitaxel and carboplatin, which allowed to achieve a median overall survival (OS) equal to 37 months and an objective response rate of 52%. Disease progression is observed in nearly half of the patients with advanced EC within the first-year post-treatment. At the same time, second-line chemotherapeutic treatment does not demonstrate substantial efficacy (5). In light of these challenges, immunotherapy – specifically, immune checkpoint inhibitors (ICIs) – emerges as a promising approach to amplify the effectiveness of the conventional treatment in endometrial cancer (6, 7) (Table 1). PD-1/PD-L1 axis plays essential role in immune escape mechanisms and is reported the most effective target for ICI therapy (8). Herewith, the expression and activity of PD-L1 in tumor is under rigorous control accompanied by the complex molecular mechanisms including genomic amplification, epigenetic regulation, transcriptional regulation, translational regulation and posttranslational modification (9, 10).
The effectiveness of immunotherapy in monotherapy and in combination varies from 26.7% to 63.6%, respectively. Data on clinical trials involved in studying ICI effects were extensively collected in systemic review (11). Predicting tumor response to ICIs is possible due to an effective differentiation between so-named “cold” (immune-desert) and “hot” (immune-inflamed) tumor phenotypes. Tumor intrinsic factors and tumor immune microenvironment characteristics underlie the concept of “cold” and “hot” tumors. Key factors related to the durable response rate and efficiency of ICIs in EC include high levels of T cell infiltration, increased PD-L1 expression, and high tumor mutation burden (TMB) or MSI-H/dMMR status of tumors (12).
Despite the progress made in improving the immunotherapy efficacy for patients with advanced or recurrent EC, there is still a considerable proportion of patients who do not respond to the treatment. It is necessary to search for and implement highly accurate biomarkers for immunotherapy application in patients with EC. In the present review, we collected and described data accumulated for several factors, which allow predicting the response to immunotherapy in EC patients: genetic alterations, transcriptomic signatures of immune cells, transcriptomic immune-related signatures and proteomic signatures.
2 Genetic alterations
The integration of molecular genetics into clinical oncology has revolutionized the classification of molecular subtypes in tumors, including EC. Pioneering work made by Levine et al. resulted in the stratification of EC into four genetic subtypes with distinct prognostic implications (13). These subtypes are defined as follows: POLE mut (10%), with a high mutation rate in DNA polymerase epsilon; MSI-high (20%), indicating microsatellite instability; TP53 wt (52%) with wild-type p53 status; and TP53 mut (18%), with TP53 gene mutations. POLE mut shows elevated T effector and interferon (IFN) signatures, suggesting a strong immune response and reduced innate resistance to anti-PD-1 therapy, making it potentially more responsive to immunotherapy. In contrast, TP53 wt has lower T effector and IFN-related gene expression, possibly indicating resistance to ICIs. This highlights the importance of molecular profiling not only for prognosis, but also in predicting immunotherapy responses (14).
Expanding this genetic framework, molecular subtypes of EC based on immune suppression gene signatures were determined in recent study (15). These signatures overlap pathways involved in T cell exhaustion, Treg function, myeloid-derived suppressor cell (MDSC) activity, transforming growth factor-beta (TGF-β) signaling, and IFN-γ signaling. This analysis revealed four immune subtypes: the immune-activated subtype, characterized by strong expression of immune cell markers and immune checkpoint molecules, reflecting a robust anti-tumor immune environment; the immune-deficient subtype, defined by low immune gene expression and related to poorer clinical outcomes; the IFN-γ dominant subtype, marked by high expression of genes driving IFN-γ signaling, a key mediator of immune response; and the TGF-β dominant subtype, distinguished by elevated expression of genes of TGF-β signaling pathway, known for its role in immune modulation (15).
Several mutations found in tumor tissues of EC patients were demonstrated to be associated with response to immune checkpoint blockade (ICB) therapy. Thus, mutations in a POLE gene in patients with EC may result in the overexpression of PD-1 in tumor-infiltrating lymphocytes. This indicates that mutations in POLE can be predictive for the effectiveness of anti-PD-1 therapy in EC (16). Two cases of advanced ultra/hypermutated EC with mutations in POLE were described. These two patients who underwent radiotherapy plus chemotherapy followed by administration of nivolumab obtained a sustained clinical efficacy with a duration of seven months (17). The most common mutations indicative for EC patients were mutations in genes PTEN, ARID1A, and PIK3CA (18).
The correlation between mutations in ARID1A, a gene implicated in chromatin remodeling, and improved outcomes after ICB therapy is well-documented across various cancer types including EC (19). A comprehensive study across multiple cancer types demonstrated better survival rates in EC patients with ARID1A mutations who undergone ICB therapy (20). However, this study included only a small cohort (N=10) that was insufficient to establish a significant survival benefit. ARID1A mutations was associated with Treg infiltration and enhanced type-I IFN response pathway, and authors supposed that it could improve the immune response and survival rates in EC patients (20). ARID1A directly interacts with DNA mismatch repair protein MSH2, a key component of the DNA mismatch repair (MMR) system (21). The absence of ARID1A disrupts MSH2 functionality, resulting in a high frequency of microsatellite instability (MSI-high). This process is often accompanied by an upregulation of PD-L1, and tumors harboring ARID1A mutations typically respond to the treatment with ICIs better than tumors without mutations in ARID1A (22, 23). However, this correlation does not produce a desired effect in microsatellite stable (MSS) endometrial carcinoma, where ARID1A deficiency does not lead to notable PD-L1 expression or anti-tumor immune infiltration (24). Authors of recent study concluded that the predictive value of ARID1A mutations for ICI therapy response can be limited in EC due to the insufficiency of ARID1A-negative tumors with MSI-high status (24). This is out of sync with studies on other cancer types that defined mutation in ARID1A as a predictive biomarker for ICI response.
A mutational signature of a DNA polymerase delta catalytic subunit gene (POLD1), specifically the p.D402N variant, was identified in the tumor tissue of patient with EC (25). A comprehensive whole-exome and transcriptomic analysis of tumor tissues revealed a pronounced hypermutated state of tumors along with a T cell-inflamed gene expression profile in patients with EC. These features collectively served as prognostic indicator for the efficacy of pembrolizumab therapy (25). In a single-arm Phase 2 clinical trial of nivolumab, patients with dMMR (deficient DNA mismatch repair) uterine or ovarian cancers who had MEGF8 or SETD1B somatic mutations experienced better 24-week PFS (26).
These findings highlight the complex interplay between genetic alterations and the immune landscape in EC. The molecular deciphering of heterogeneous EC subtypes do not only help in prognostication but also paves the way for personalized immunotherapeutic approaches, which will potentially improve patient outcomes.
3 Transcriptomic signatures of immune cells
Among various predictors of immunotherapy response in EC, gene signatures related to immune cell types are the most prevalent. In analysis of 539 EC specimens, a prognostic model was built based on four ICI-associated genes: LINC01871, CXCL13, IGKJ5, and LINC01281 (27). Tumor tissues from patients with a lower risk score had an abundant infiltration with immune effector cells and were classified as having an immune-inflamed phenotype. These patients also showed high immunophenoscore (specifically, high CTLA4 and PD1 scores), indicating a likely better response to immunotherapy (27). Another study found that EC patients with a high novel immune risk score (NIRS), included CTSW, CD3D, and CD48 genes, exhibited diminished levels of immune cell infiltration, fewer gene mutations, and lower expression of various immune checkpoints (28). Authors suggested that high NIRS that are related to diminished CD8+ T cell infiltration can be indicative for a decreased responsiveness to immunotherapy (28).
A subset of tumor-infiltrating CXCL8hiIL1Bhi macrophages was identified as a predictor for the durable response to immunotherapy in EC (29). A prognostic signature was developed using six genes from this macrophage subset – SCL8A1, TXN, ANXA5, CST3, CD74, and NANS. Patients with high-risk score had shorter survival and worse response to immunotherapy (29). The existence of dysfunctional (CD8+PD-1+) or terminally dysfunctional (CD8+PD-1+TOX+) T cells, along with their interaction with PD-L1+ cells, independently predicted a 24-month PFS in patients with dMMR uterine or ovarian cancers treated with nivolumab (26). In another study, CXCL9-secreting antigen-presenting and CXCL13-secreting follicular dendritic cells contributed to the formation of an immune-activated milieu in EC via the maintaining tertiary lymphoid structures (TLSs) (30). Complementary study indicated that the detection of TLSs within hematoxylin-eosin-stained sections correlated with improved PFS in patients with advanced dMMR endometrial cancer undergone second-line treatment with Durvalumab (31). To substantiate these preliminary findings and to delineate further the factors that can predict the response to Durvalumab, particularly in first-line settings, the analysis of larger patient cohorts can be suggested. Subsequent study revealed that the presence of cytotoxic T-cell response elements within the TME, including elevated IFN-γ and T-cell inflamed scores accompanied with high HLA class II expression, was related to an extended duration of response with pembrolizumab and lenvatinib in serous endometrial cancer (32). Another cells that may have clinical relevance in the context of anti-PD-1 therapy is T follicular helper (Tfh) (33). Classifying patients based on Tfh presence can predict the effectiveness of ICIs. Thus, high Tfh score was associated with favorable outcomes following anti-PD-1 therapy. A notably greater incidence of complete or partial response was observed in patients with abundant Tfh infiltration compared to those with minimal Tfh infiltration. Hereby, the stratification of patients according to Tfh levels can constitute an optimal way for enhancing the accuracy for administration of anti-PD-1 treatment in EC (33). In patients with MSI-H endometrial cancer treated with pembrolizumab, a significant increase in fibroblast and endothelial cell transcriptomic signatures was observed in non-responders compared to responders (34, 35). Specifically, the expression of TAGLN (fibroblast gene) and endothelial cell genes (EMCN, KDR, MMRN1, MYCT1, PEAR1, PTPRB, and TEK) was elevated as response decreased (34). Imaging mass cytometry analysis demonstrated similar trend of higher population of activated fibroblasts (SMA+, MFAP5+) and endothelial cells (CD31+) as well as regulatory T cells (CD4+FOXP3+) in non-responders (35).
Another study found that the effectiveness of immunotherapy is linked to both the presence and the functional state of certain immune cell markers (36).
Thus, a balance in the composition and the activation state of the TME can be strongly associated with the response to immunotherapy in EC, and foremost in MSI-H tumors. A durable response to ICI were detected in patients with the TME strongly accumulated by Tfh, cytotoxic T-cell, dysfunctional (CD8+PD-1+) or terminally dysfunctional (CD8+PD-1+TOX+) T cells, CXCL8hiIL1Bhi macrophage subsets, and TLS presence. Tumor infiltration with activated fibroblasts (SMA+, MFAP5+) and endothelial cells (CD31+) as well as regulatory T cells (CD4+FOXP3+) was an indicator for marginal immunotherapy efficacy (Figure 1).
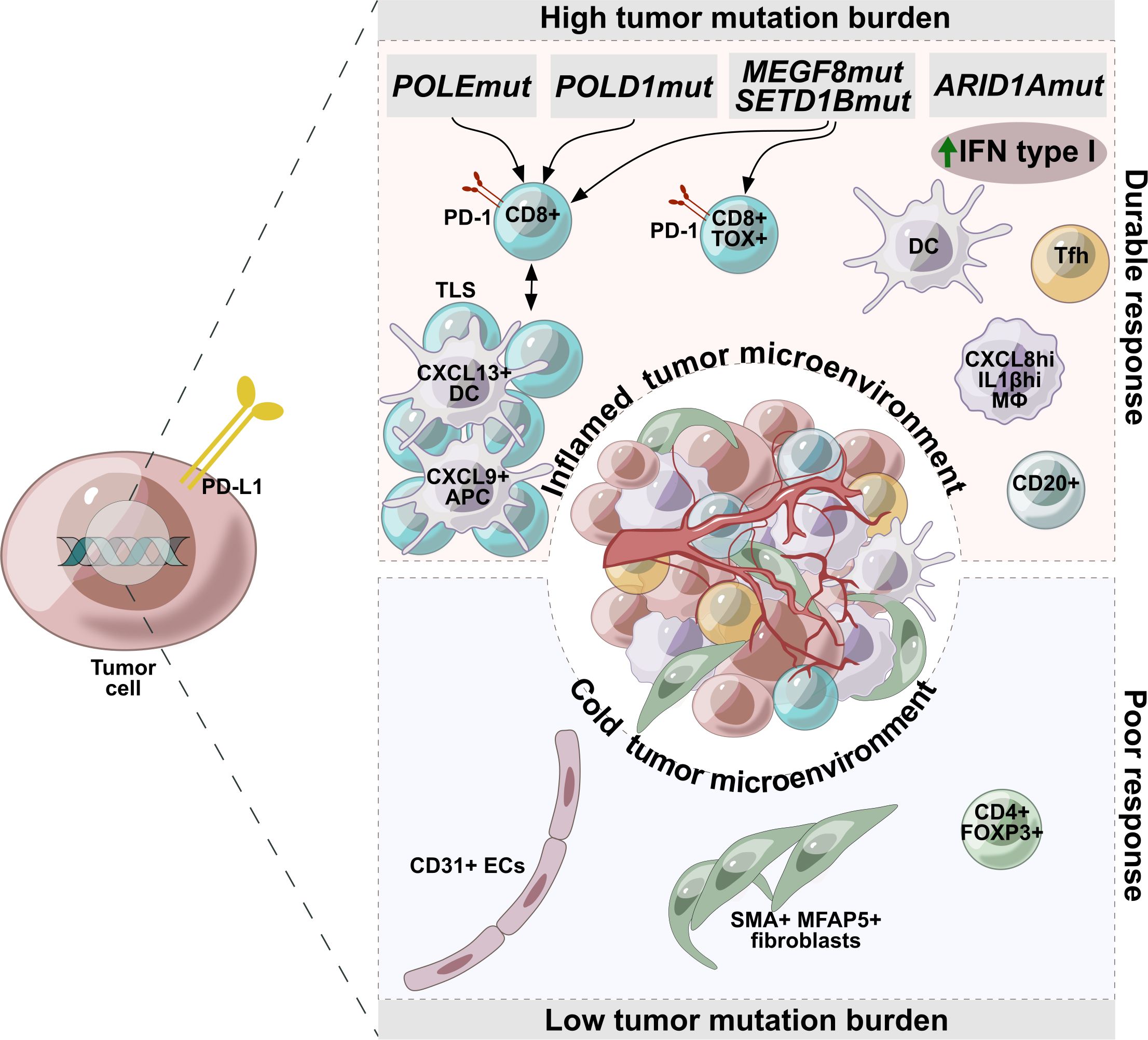
Figure 1. Factors associated with durable or poor response to immunotherapy in endometrial cancer. Tumors with high mutational burden are characterized by accumulation of Tfh, cytotoxic T-cell, dysfunctional (CD8+PD-1+) or terminally dysfunctional (CD8+PD-1+TOX+) T cells, CXCL8hiIL1Bhi macrophage subsets, and TLS presence, making these tumors potentially more sensitive to immunotherapy. Tumors with a low mutational burden are characterized by a cold microenvironment and are infiltrated with activated fibroblasts (SMA+, MFAP5+), endothelial cells (CD31+), regulatory T cells (CD4+FOXP3+), which are indicators of low efficacy of immunotherapy.
4 Transcriptomic immune-related signatures
Some gene signatures comprise genes which don’t directly belong to immune cell markers, but are associated with immune cell response. A prognostic model constructed using five genes associated with metabolic processes (INPPP5K, PLPP2, MBOAT2, DDC, and ITPKA) was related to immune cell infiltration and immune system functions in EC (37). It was correlated with the expression of immune checkpoints (CD279, CTLA4, and CD40) and predicted clinical outcomes of ICI therapy (37).
Another immune-related prognostic signature (IRPS) was designed based on the expression of two genes, CCL13 and KLRC1. It was observed that patients exhibiting a lower IRPS demonstrated more favorable outcome from immunotherapy and more robust response to the treatment following various chemotherapeutic regimens (38). Authors utilized a panel of 34 immune cell dysfunction-related genes (ICDRGs) to calculate the Immune Phenotype Score (IPS), which helped to predict the clinical efficacy of ICIs in various EC patient cohorts (36). The findings indicated that patients with EC who had lower ICDRG score exhibited higher IPS, suggesting a greater sensitivity to immunotherapy compared to those with higher ICDRG score. In this study, the authors did not describe specific cell types associated with response to immunotherapy (36).
An immune signature, included following genes CBLC, PLA2G2A, TNF, NR3C1, APOD, TNFRSF18, and LTB, was an independent prognostic criterion for OS and outperformed conventional methods for staging of EC (39). Based on this gene signature, patients categorized at low-risk group had an increased sensitivity to ICI therapy (39). The majority of the genes composing this signature play essential roles in controlling the immune response. Specifically, c-Cbl (CBLC gene) is found in different immune cells, including macrophages, where it binds to PD-1 and marks it for ubiquitination, that leads to proteasomal degradation of the latter (40). This process reduces PD-1 expression on macrophages and aggravates their ability to phagocyte tumor cells, thereby inhibiting tumor growth (40). Phospholipase A2, membrane associated (PLA2G2A) is implicated in supporting an innate Th2-type immunity, which in turn boosts the early production of B-1 B cells (41).
A comprehensive 12-gene signature included DRAM1, ELAPOR1, MAPT, TRIM58, UCHL1, CDKN2A, CYFIP2, AKT2, LINC00618, TTPA, TRIM46, and NOS2, and belonged to Programmed Cell Death (PCD) pathways was used to estimate the response to immunotherapy in patients with EC. It was supposed that patients with increased PCD score may exhibit an attenuated response to immunotherapeutic modalities (42). The expression of TRIM58, which is a part of above-mentioned gene signature, positively correlated to the abundant accumulation of M2 macrophages and resting mast cells in the TME, and inversely correlated to the presence of Tfh in the TME in KRAS-mutant lung adenocarcinoma (43). An ubiquitin carboxy-terminal hydrolase L1 (UCHL1) expressed by macrophages facilitated the destruction of the phosphatidylinositol-4,5-bisphosphate 3-kinase, catalytic subunit alpha (PI3CA), via autophagic processes in vivo. This process resulted in the augmentation of the AKT pathway activity, which in turn, fostered the differentiation of macrophages towards the pro-inflammatory M1 phenotype (44). Cyclin-dependent kinase inhibitor 2A (CDKN2A) loss-of-function is associated with poorer clinical outcome in patients with advanced NSCLC treated with ICIs (45).
Interesting was the point that the long non-coding RNAs (lncRNAs) associated with cuproptosis, a process occurring by combining copper ions with the lipid-acylated components leading to cell death, can affect immune-related functions and the efficacy of immunotherapy (46). Recent study has demonstrated that molecular phenotypes related to cuproptosis, together with the scoring system known as CuproScore, can help in prognosis for patients with colorectal cancer and to predict the response to immunotherapy (47). These phenotypes included FDX1, LIPT1, LIAS, DLD, DBT, GCSH, DLST, DLAT, PDHA1, PDHB, SLC31A1, ATP7A, ATP7B, CDKN2A, GLS, and MTF1 (47). Patients with gastric cancer categorized in the low cuproptosis-related prognosis signature subgroup exhibited elevated levels of tumor mutation burden (TMB), a higher incidence of MSI-H, and increased expression of PD-L1 in tumor (48). These factors were associated with a more favorable response to immunotherapy (48). Another study demonstrated that the subgroup of patients with low G Protein-Coupled Receptor (GPR) score (GPR-related genes) and high TME score had the highest proportion (31%) of patients responding to ICB therapy compared to those with high GPR and low TME (18). This subgroup also showed increased expression of several inhibitory immune markers, including CTLA4, LAG3, and PDCD1, as well as human leukocyte antigen (HLA) markers (18). Recent study indicated that the expression of CCNE1 was significantly associated with TMB, MSI, the presence of neoantigens, and Immune Checkpoints in EC. This association could influence the responsiveness to immunotherapy treatment (49).
Thus, some essential genes play a fundamental role in maintaining the equilibrium in the TME, that is critical in the defining immunotherapy efficacy.
5 Proteomic signatures
One more prognostic model was constructed based on the protein expression of X1433EPSILON, Chk2-pT68, ER alpha, Fibronectin, PR, EPPK1, Annexin 1, Myosin IIA, and p16INK4a in EC tumor tissue (50). The expression of X1433EPSILON, ER-alpha, PR, Annexin 1, and Myosin IIA was associated with a better outcome, whereas the expression of Chk2-pT68, Fibronectin, EPPK1, and p16INK4a was associated with an unfavorable prognosis. The low-risk group exhibited higher proportions of CD8+ T cells, Tfh cells, and Tregs in the TME. Authors suggested that patients in the low-risk category were more likely to respond to immunotherapy compared to those in the high-risk group (50). Markers of immunotherapy response that are based on the immunohistochemical detection of particular molecules or cellular types were also revealed. In our recent study, we identified that in the TME of pMMR endometrial cancer, both the percentage of CD20+ B lymphocytes and the ratio of CD8-to-CD20 lymphocytes indicated responders to immune-targeted therapy and predicted favorable OS (51). In dMMR EC, non-responders generally had lower levels of CD8+ T cells, no terminally differentiated T cells, absent mature TLS and dendritic cells (52). A combination of four specific immune features (absence of PD-L1 and expression of TIM-3, IDO1, and LAG-3) accurately predicted ICI response (52).
6 Conclusions and future perspectives
Last years manifested emergency in patient stratification for the immunotherapy administration. For that, novel accurate predictive biomarkers must be identified. The data we collected in this review suggest that cells of the TME are critical in achieving high efficacy of immunotherapy in endometrial cancer. Thus, tumor infiltration with Tfh, cytotoxic T-cell, dysfunctional (CD8+PD-1+) or terminally dysfunctional (CD8+PD-1+TOX+) T cells, CXCL8hiIL1Bhi macrophages, as well as TLS presence, indicating “hot” tumor microenvironment, may be a strong indicator for beneficial immunotherapy response. Simultaneously, high TMB accompanied by mutations in critical genes increases the chance for a positive response to ICIs in patients with endometrial cancer. Systemic factors are also likely to make a significant contribution to the prognosis of disease progression. However, the literature lacks comprehensive studies on the predictive value of systemic factors in EC. Only the correlation of neutrophil-to-lymphocyte ratio (NLR) with the effectiveness of immunotherapies such as pembrolizumab, the combination of lenvatinib and pembrolizumab, nivolumab, and atezolizumab was studied. A pre-treatment NLR below 6 was associated with improved OS in recurrent EC patients received immunotherapy (53).
In recent years, using high-tech sequencing methods, various transcriptional signatures have been developed that reflect the functional activation of a number of cells in the TME. However, the role of gene signatures is controversial, since the use of sequencing methods involves the introduction of errors associated with the processing of big data, and, therefore, errors in the true predictive value of gene signatures.
We assure that to use described biomarker in clinics, the investigations on its predictive value should be started from the beginning of the clinical trial, followed by a stratified randomization process that comprehensively addresses the status of this biomarker. Moreover, to uncover applicable biomarker that able to predict immunotherapy effectiveness properly, precise molecular and cellular mechanisms underlying either tumor progression or tumor regression during treatment should be thoroughly investigated.
It should be noted that endometrial tumors, for which the administration of ICIs is applicable, accounts only 20-25% among all cases of EC. It is related to the nature of tumor and character of the TME. This fact indicates the exigence in the searching therapeutic strategies for proficient MMR tumors, as well as increasing the immunogenicity of tumor or the transition of “cold” into “hot” tumors. According to these demands, multiple clinical trials and pre-clinical studies demonstrated the success in using immunotherapy in combination with other types of therapies. These approaches include the combination immunotherapy, the use of immune checkpoint inhibitors with chemotherapy, with angiogenesis inhibitors, with radiotherapy, or epigenetic drugs. For example, pembrolizumab plus lenvatinib (a multitargeted tyrosine kinase inhibitor of vascular endothelial growth factor receptors 1 through 3, fibroblast growth factor receptors 1 through 4, platelet-derived growth factor receptor α, RET, and KIT) demonstrated longer PFS and OS than chemotherapy among patients with advanced EC that was not MSI-H/dMMR, followed by the approval of this combination by the FDA for this group of patients (54, 55).
Substantial reprogramming of the TME by several cytotoxic chemotherapeutic drugs and radiotherapy regiments was demonstrated to induce various immunostimulatory effects. Thus, chemotherapy may activate cytotoxic immune cells, enhance the presentation of tumor cell-specific antigens, and induce immunogenic cell death (56). Some preclinical models demonstrated convincingly that the efficacy of certain chemotherapeutic agents is higher in immunocompetent mice than in their immunodeficient counterparts (57). Moreover, immune stimulatory effects of chemotherapy can contribute to the transformation of “cold” to “hot” tumors in response to ICIs (58). All these evidences triggered clinical trials based on using combination chemotherapy and ICIs in EC patients (59, 60).
The mechanism of radiotherapy is associated with releasing tumor neoantigens and activation of adaptive immune response both locally in irradiated tumor site and in non-irradiated tumor metastases, known as abscopal effect, inducing immunogenic tumor cell death in the distant sites (61). Clinical trials with combination therapy based on ICIs and radiotherapy are limited for metastatic EC, but can be promising strategy to complementary control distant metastatic spread (61).
The leading-edge approach for treating EC is developing based on epigenetic modification inhibitors that are aimed to increase the immunogenicity of tumors and may help to facilitate the turning tumors from a “cold” into a “hot” ones (12, 58). The application of therapeutic epigenetic regulation combined with ICIs is under the clinical trials for several cancers, but not for endometrial cancer (10).
In summary, to improve the accuracy of the effectiveness of reported combinatorial approaches, it is urgently needed to unravel the intricate mechanisms of tumor immune microenvironment involvement in the pathogenesis of EC, and, more thoroughly, the mechanisms of the transition of “hot” to “cold” endometrial tumors. The inclusion of rigorous biomarker evaluation into clinical trials is required for the efficacious introduction of brand-new immunotherapeutic strategies and combinatory approaches targeting endometrial cancer. Hereby, the responsibility for the success of oncoming clinical trials goes outside the framework of simple evaluation of the efficacy of monotherapy or combination therapy; it encompasses an understanding of complex molecular ground that contributes to this success.
Author contributions
LT: Writing – original draft, Writing – review & editing. IL: Writing – original draft. NE: Writing – original draft. AM: Writing – original draft. EL: Writing – original draft. AK: Writing – original draft. MS: Writing – review & editing. LK: Writing – review & editing.
Funding
The author(s) declare financial support was received for the research, authorship, and/or publication of this article. This research was funded by Russian Science Foundation, grant number 20-75-10033-P.
Acknowledgments
We would like to thank Elena Shmakova for preparing the figure.
Conflict of interest
The authors declare that the research was conducted in the absence of any commercial or financial relationships that could be construed as a potential conflict of interest.
Generative AI statement
The author(s) declare that no Generative AI was used in the creation of this manuscript.
Publisher’s note
All claims expressed in this article are solely those of the authors and do not necessarily represent those of their affiliated organizations, or those of the publisher, the editors and the reviewers. Any product that may be evaluated in this article, or claim that may be made by its manufacturer, is not guaranteed or endorsed by the publisher.
References
1. Siegel RL, Miller KD, Wagle NS, Jemal A. Cancer statistics, 2023. CA Cancer J Clin. (2023) 73:17–48. doi: 10.3322/caac.21763
2. Gu B, Shang X, Yan M, Li X, Wang W, Wang Q, et al. Variations in incidence and mortality rates of endometrial cancer at the global, regional, and national levels, 1990-2019. Gynecol Oncol. (2021) 161:573–80. doi: 10.1016/j.ygyno.2021.01.036
3. Yang L, Yuan Y, Zhu R, Zhang X. Time trend of global uterine cancer burden: an age-period-cohort analysis from 1990 to 2019 and predictions in a 25-year period. BMC Womens Health. (2023) 23:384. doi: 10.1186/s12905-023-02535-5
4. Mazidimoradi A, Momenimovahed Z, Khalajinia Z, Allahqoli L, Salehiniya H, Alkatout I. The global incidence, mortality, and burden of uterine cancer in 2019 and correlation with SDI, tobacco, dietary risks, and metabolic risk factors: An ecological study. Health Sci Rep. (2024) 7:e1835. doi: 10.1002/hsr2.1835
5. Romero D. Survival benefit with second-line combination in endometrial cancer. Nat Rev Clin Oncol. (2022) 19:149. doi: 10.1038/s41571-022-00604-6
6. Banerjee S, Hasson SP. Top advances of the year: Immunotherapy in endometrial cancer. Cancer. (2024) 130:3082–6. doi: 10.1002/cncr.35417
7. Hamoud BH, Sima RM, Vacaroiu IA, Georgescu MT, Bobirca A, Gaube A, et al. The evolving landscape of immunotherapy in uterine cancer: A comprehensive review. Life (Basel). (2023) 13:1502. doi: 10.3390/life13071502
8. Mamat Yusof MN, Chew KT, Kampan N, Abd Aziz NH, Md Zin RR, Tan GC, et al. PD-L1 expression in endometrial cancer and its association with clinicopathological features: A systematic review and meta-analysis. Cancers (Basel). (2022) 14:3911. doi: 10.3390/cancers14163911
9. Ju X, Zhang H, Zhou Z, Wang Q. Regulation of PD-L1 expression in cancer and clinical implications in immunotherapy. Am J Cancer Res. (2020) 10:1–11.
10. Yang J, Xu J, Wang W, Zhang B, Yu X, Shi S. Epigenetic regulation in the tumor microenvironment: molecular mechanisms and therapeutic targets. Signal Transduct Target Ther. (2023) 8:210. doi: 10.1038/s41392-023-01480-x
11. Maiorano BA, Maiorano MFP, Cormio G, Maglione A, Lorusso D, Maiello E. How immunotherapy modified the therapeutic scenario of endometrial cancer: A systematic review. Front Oncol. (2022) 12:844801. doi: 10.3389/fonc.2022.844801
12. Wu B, Zhang B, Li B, Wu H, Jiang M. Cold and hot tumors: from molecular mechanisms to targeted therapy. Signal Transduct Target Ther. (2024) 9:274. doi: 10.1038/s41392-024-01979-x
13. Cancer Genome Atlas Research Network, Kandoth C, Schultz N, Cherniack AD, Akbani R, Liu Y, et al. Integrated genomic characterization of endometrial carcinoma. Nature. (2013) 497:67–73. doi: 10.1038/nature12113
14. Jiang F, Jiang S, Cao D, Mao M, Xiang Y. Immunologic signatures across molecular subtypes and potential biomarkers for sub-stratification in endometrial cancer. Int J Mol Sci. (2023) 24:1791. doi: 10.3390/ijms24021791
15. Yang Y, Wu SF, Bao W. Molecular subtypes of endometrial cancer: Implications for adjuvant treatment strategies. Int J Gynecol Obstet. (2024) 164:436–59. doi: 10.1002/ijgo.14969
16. Howitt BE, Shukla SA, Sholl LM, Ritterhouse LL, Watkins JC, Rodig S, et al. Association of polymerase e-mutated and microsatellite-instable endometrial cancers with neoantigen load, number of tumor-infiltrating lymphocytes, and expression of PD-1 and PD-L1. JAMA Oncol. (2015) 1:1319–23. doi: 10.1001/jamaoncol.2015.2151
17. Santin AD, Bellone S, Buza N, Choi J, Schwartz PE, Schlessinger J, et al. Regression of chemotherapy-resistant Polymerase epsilon (POLE) ultra-mutated and MSH6 hyper-mutated endometrial tumors with nivolumab. Clin Cancer Res. (2016) 22:5682–7. doi: 10.1158/1078-0432.CCR-16-1031
18. Chen S, Luo X, Yang B, Zhuang J, Guo J, Zhu Y, et al. The combined signatures of G protein-coupled receptor family and immune landscape provide a prognostic and therapeutic biomarker in endometrial carcinoma. J Cancer Res Clin Oncol. (2023) 149:14701–19. doi: 10.1007/s00432-023-05270-4
19. Zhou H, Sun D, Song S, Niu Y, Zhang Y, Lan H, et al. Efficacy of immunotherapy in ARID1A-mutant solid tumors: a single-center retrospective study. Discovery Onc. (2024) 15:213. doi: 10.1007/s12672-024-01074-1
20. Wu Z, Wang Q, Liu X, Zheng J, Ji L, Li X. Identification and validation of a novel multiomics signature for prognosis and immunotherapy response of endometrial carcinoma. J Oncol. (2022) 2022:8998493. doi: 10.1155/2022/8998493
21. Shen J, Ju Z, Zhao W, Wang L, Peng Y, Ge Z, et al. ARID1A deficiency promotes mutability and potentiates therapeutic antitumor immunity unleashed by immune checkpoint blockade. Nat Med. (2018) 24:556–62. doi: 10.1038/s41591-018-0012-z
22. Kim YB, Ahn JM, Bae WJ, Sung CO. Lee D Functional loss of ARID1A is tightly associated with high PD-L1 expression in gastric cancer. Int J Cancer. (2019) 145:916–26. doi: 10.1002/ijc.32140
23. Li L, Li M, Jiang Z, Wang X. ARID1A mutations are associated with increased immune activity in gastrointestinal cancer. Cells. (2019) 8:678. doi: 10.3390/cells8070678
24. Yamashita H, Nakayama K, Kanno K, Ishibashi T, Ishikawa M, Iida K, et al. Evaluation of ARID1A as a potential biomarker for predicting response to immune checkpoint inhibitors in patients with endometrial cancer. Cancers. (2024) 16:1999. doi: 10.3390/cancers16111999
25. Wei CH, Wang EW, Ma L, Zhou Y, Zheng L, Hampel H, et al. POLD1 DEDD motif mutation confers hypermutation in endometrial cancer and durable response to pembrolizumab. Cancers. (2023) 15:5674. doi: 10.3390/cancers15235674
26. Friedman CF, Manning-Geist BL, Zhou Q, Soumerai T, Holland A, Da Cruz Paula A, et al. Nivolumab for mismatch-repair-deficient or hypermutated gynecologic cancers: a phase 2 trial with biomarker analyses. Nat Med. (2024) 30:1330–8. doi: 10.1038/s41591-024-02942-7
27. Zhu H, Luo F, He W, Zhang S, Chen J, Sun L, et al. Identification of an immune cell infiltration-related prognostic model to guide immunotherapy in endometrial carcinoma. Clin Exp Obstet Gynecol. (2023) 50:204. doi: 10.31083/j.ceog5010204
28. Zhang G, Yin Z, Fang J, Wu A, Chen G, Cao K. Construction of the novel immune risk scoring system related to CD8+ T cells in uterine corpus endometrial carcinoma. Cancer Cell Int. (2023) 23:124. doi: 10.1186/s12935-023-02966-y
29. Wu Q, Jiang G, Sun Y, Li B. Reanalysis of single-cell data reveals macrophage subsets associated with the immunotherapy response and prognosis of patients with endometrial cancer. Exp Cell Res. (2023) 430:113736. doi: 10.1016/j.yexcr.2023.113736
30. Kodama M, Nagase Y, Aimono E, Nakamura K, Takamatsu R, Abe K, et al. CXCL9 and CXCL13 coordinately shape the immune-activated microenvironment of endometrial cancer via tertiary lymphoid structure formation. Res Square. (2023). doi: 10.21203/rs.3.rs-2503389/v1
31. Zhou Z, Song Y, Song Z, Zhu X, Han S. 47TiP - A real-world study of multiparametric prediction of the efficacy of immune checkpoint inhibitors in combination with chemotherapy for advanced non-small cell lung cancer. Immuno-Oncol Technol. (2023) 20:100515. doi: 10.1016/j.iotech.2023.100515
32. Carballo EV, Wu S, Angara K, Penn CA, Herzog TJ, Thaker P, et al. Examining the immune tumor microenvironment of endometrial cancer patients treated with pembrolizumab plus Lenvatinib. Gynecol Oncol Rep. (2023) 48:S1. doi: 10.1016/S2352-5789(23)00222-9
33. Chen Y, You S, Li J, Zhang Y, Kokaraki G, Epstein E, et al. Follicular helper T-cell-based classification of endometrial cancer promotes precise checkpoint immunotherapy and provides prognostic stratification. Front Immunol. (2022) 12:788959. doi: 10.3389/fimmu.2021.788959
34. How J, Dang M, Euscher E, Yates M, Peng W, Patel S, et al. The tumor immune microenvironment shapes response to pembrolizumab in microsatellite instability-high endometrial cancer. Gynecol Oncol. (2021) 162:S308. doi: 10.1016/S0090-8258(21)01235-X
35. How JA, Dang M, Ferri-Borgogno S, Euscher E, Yates MS, Peng W, et al. Abstract 1248: Predictors of innate resistance to pembrolizumab in patients with microsatellite instability-high endometrial cancer. Cancer Res. (2022) 82:1248. doi: 10.1158/1538-7445.AM2022-1248
36. Liu Z, Luo Y, Su L, Hu X. Identification of immunogenic cell death-related subtypes used for predicting survival and immunotherapy of endometrial carcinoma through a bioinformatics analysis. Medicine. (2023) 102:e34571. doi: 10.1097/MD.0000000000034571
37. Huang H, Cai X, Lin J, Wu Q, Zhang K, Lin Y, et al. A novel five-gene metabolism-related risk signature for predicting prognosis and immune infiltration in endometrial cancer: A TCGA data mining. Comput Biol Med. (2023) 155:106632. doi: 10.1016/j.compbiomed.2023.106632
38. Liu J, Wang Y, Mei J, Nie S, Zhang Y. Identification of a novel immune landscape signature for predicting prognosis and response of endometrial carcinoma to immunotherapy and chemotherapy. Front Cell Dev Biol. (2021) 9:671736. doi: 10.3389/fcell.2021.671736
39. Meng Y, Yang Y, Zhang Y, Yang X, Li X, Hu C. The role of an immune signature for prognosis and immunotherapy response in endometrial cancer. Am J Transl Res. (2021) 13:532–48.
40. Lyle C, Richards S, Yasuda K, Napoleon MA, Walker J, Arinze N, et al. c-Cbl targets PD-1 in immune cells for proteasomal degradation and modulates colorectal tumor growth. Sci Rep. (2019) 9:20257. doi: 10.1038/s41598-019-56208-1
41. Shinton SA, Brill-Dashoff J, Hayakawa K. Pla2g2a promotes innate Th2-type immunity lymphocytes to increase B1a cells. Sci Rep. (2022) 12:14899. doi: 10.1038/s41598-022-18876-4
42. Meng J, Zong C, Wang M, Chen Y, Zhao S. Constructing a prognostic model of uterine corpus endometrial carcinoma and predicting drug-sensitivity responses using programmed cell death-related pathways. J Cancer. (2024) 15:2948–59. doi: 10.7150/jca.92201
43. Chen X, Wang Y, Qu X, Bie F, Wang Y, Du J. TRIM58 is a prognostic biomarker remodeling the tumor microenvironment in KRAS-driven lung adenocarcinoma. Future Oncol. (2021) 17:565–79. doi: 10.2217/fon-2020-0645
44. Huang Y, He S, Chen Y, Sheng J, Fu Y, Du X, et al. UCHL1 promoted polarization of M1 macrophages by regulating the PI3K/AKT signaling pathway. J Inflammation Res. (2022) 15:735–46. doi: 10.2147/JIR.S343487
45. Gutiontov SI, Turchan WT, Spurr LF, Rouhani SJ, Chervin CS, Steinhardt G, et al. CDKN2A loss-of-function predicts immunotherapy resistance in non-small cell lung cancer. Sci Rep. (2021) 11:20059. doi: 10.1038/s41598-021-99524-1
46. Zhang X, Ye Z, Xiao G, He T. Prognostic signature construction and immunotherapy response analysis for Uterine Corpus Endometrial Carcinoma based on cuproptosis-related lncRNAs. Comput Biol Med. (2023) 159:106905. doi: 10.1016/j.compbiomed.2023.106905
47. Shao Y, Fan X, Yang X, Li S, Huang L, Zhou X, et al. Impact of Cuproptosis-related markers on clinical status, tumor immune microenvironment and immunotherapy in colorectal cancer: A multi-omic analysis. Comput Struct Biotechnol J. (2023) 21:3383–403. doi: 10.1016/j.csbj.2023.06.011
48. Wang XX, Deng SZ, Wu LH, Liu QQ, Zheng G, Du K, et al. Cuproptosis-mediated patterns characterized by distinct tumor microenvironment and predicted the immunotherapy response for gastric cancer. ACS Omega. (2023) 8:10851–62. doi: 10.1021/acsomega.2c07052
49. Zheng X, Chen L, Liu W, Zhao S, Yan Y, Zhao J, et al. CCNE1 is a predictive and immunotherapeutic indicator in various cancers including UCEC: a pan-cancer analysis. Hereditas. (2023) 160:13. doi: 10.1186/s41065-023-00273-0
50. Lai J, Xu T, Yang H. Protein-based prognostic signature for predicting the survival and immunotherapeutic efficiency of endometrial carcinoma. BMC Cancer. (2022) 22:325. doi: 10.1186/s12885-022-09402-w
51. Maltseva A, Kalinchuk A, Chernorubashkina N, Sisakyan V, Lots I, Gofman A, et al. Predicting response to immunotargeted therapy in endometrial cancer via tumor immune microenvironment: A multicenter, observational study. Int J Mol Sci. (2024) 25:3933. doi: 10.3390/ijms25073933
52. Grau Bejar JF, Yaniz Galende E, Zeng Q, Genestie C, Rouleau E, de Bruyn M, et al. Immune predictors of response to immune checkpoint inhibitors in mismatch repair-deficient endometrial cancer. J Immunother Cancer. (2024) 12:e009143. doi: 10.1136/jitc-2024-009143
53. Barrington DA, Calo C, Baek J, Brown M, Wagner V, Gonzalez L, et al. Beyond mismatch repair deficiency? Pre-treatment neutrophil-to-lymphocyte ratio is associated with improved overall survival in patients with recurrent endometrial cancer treated with immunotherapy. Gynecol Oncol. (2022) 166:522–9. doi: 10.1016/j.ygyno.2022.07.010
54. Makker V, Colombo N, Casado Herráez A, Santin AD, Colomba E, Miller DS, et al. Lenvatinib plus pembrolizumab for advanced endometrial cancer. N Engl J Med. (2022) 386:437–48. doi: 10.1056/NEJMoa2108330
55. Makker V, Colombo N, Casado Herráez A, Monk BJ, Mackay H, Santin AD, et al. Lenvatinib plus pembrolizumab in previously treated advanced endometrial cancer: updated efficacy and safety from the randomized phase III study 309/KEYNOTE-775. J Clin Oncol. (2023) 41:2904–10. doi: 10.1200/JCO.22.02152
56. Principe DR, Kamath SD, Korc M, Munshi HG. The immune modifying effects of chemotherapy and advances in chemo-immunotherapy. Pharmacol Ther. (2022) 236:108111. doi: 10.1016/j.pharmthera.2022.108111
57. Zitvogel L, Pitt JM, Daillère R, Smyth MJ, Kroemer G. Mouse models in oncoimmunology. Nat Rev Cancer. (2016) 16:759–73. doi: 10.1038/nrc.2016.91
58. Liu YT, Sun ZJ. Turning cold tumors into hot tumors by improving T-cell infiltration. Theranostics. (2021) 11:5365–86. doi: 10.7150/thno.58390
59. Bogani G, Monk BJ, Powell MA, Westin SN, Slomovitz B, Moore KN, et al. Adding immunotherapy to first-line treatment of advanced and metastatic endometrial cancer. Ann Oncol. (2024) 35:414–28. doi: 10.1016/j.annonc.2024.02.006
60. Eskander RN, Sill MW, Beffa L, Moore RG, Hope JM, Musa FB, et al. Pembrolizumab plus chemotherapy in advanced endometrial cancer. N Engl J Med. (2023) 388:2159–70. doi: 10.1056/NEJMoa2302312
Keywords: endometrial cancer, immunotherapy, immune checkpoint inhibitors, tumor microenvironment, predictive biomarkers
Citation: Tashireva LA, Larionova IV, Ermak NA, Maltseva AA, Livanos EI, Kalinchuk AY, Stakheyeva MN and Kolomiets LA (2025) Predicting immunotherapy efficacy in endometrial cancer: focus on the tumor microenvironment. Front. Immunol. 15:1523518. doi: 10.3389/fimmu.2024.1523518
Received: 06 November 2024; Accepted: 24 December 2024;
Published: 20 January 2025.
Edited by:
Sergei Kusmartsev, University of Florida, United StatesReviewed by:
Debasish Kumar Dey, University of Oklahoma, United StatesZhongkun Zhang, Southeast University, China
Copyright © 2025 Tashireva, Larionova, Ermak, Maltseva, Livanos, Kalinchuk, Stakheyeva and Kolomiets. This is an open-access article distributed under the terms of the Creative Commons Attribution License (CC BY). The use, distribution or reproduction in other forums is permitted, provided the original author(s) and the copyright owner(s) are credited and that the original publication in this journal is cited, in accordance with accepted academic practice. No use, distribution or reproduction is permitted which does not comply with these terms.
*Correspondence: Liubov A. Tashireva, dGFzaGlyZXZhQG9uY29sb2d5LnRvbXNrLnJ1