- 1Cancer Center, Union Hospital, Tongji Medical College, Huazhong University of Science and Technology, Wuhan, China
- 2Institute of Radiation Oncology, Union Hospital, Tongji Medical College, Huazhong University of Science and Technology, Wuhan, China
- 3Hubei Key Laboratory of Precision Radiation Oncology, Wuhan, China
- 4Department of Pediatric Surgery, Union Hospital, Tongji Medical College, Huazhong University of Science and Technology, Wuhan, China
Lung cancer, particularly non-small cell lung cancer (NSCLC), remains a leading cause of cancer-related deaths, with conventional treatments offering limited effectiveness in advanced stages, due to distant metastases and treatment resistance. Recent advancements in immunotherapy, specifically immune checkpoint inhibitors (ICIs), have shown promise, but their efficacy as standalone therapies are often insufficient. This has led to increased interest in combining ICIs with radiotherapy, known as radioimmunotherapy (iRT), to enhance treatment outcomes. This review explores the mechanisms that underlie the synergy between radiotherapy and immunotherapy. Radiotherapy can induce the “abscopal effect”, eliciting systemic immune responses that reduce tumor burdens outside the treated area. It also increases the expression of major histocompatibility complex class I (MHC-I) on tumor cells, improving immune recognition. Furthermore, radiotherapy can modify the tumor microenvironment by inducing metabolic reprogramming to bolster anti-tumor immunity. We discuss strategies for optimizing iRT, including considerations of radiation doses, fractionation schedules, and treatment site selection, which significantly influence immune responses by enhancing MHC-I expression or promoting T-cell infiltration. Clinical evidence supports the efficacy of iRT in NSCLC and other cancers, though challenges in standardizing treatment protocols and managing side effects persist. Overall, radioimmunotherapy presents a promising approach to improving NSCLC treatment outcomes. Ongoing research into its mechanisms and the refinement of treatment may reshape clinical practice, offering more effective and personalized options for patients with advanced lung cancer. Further studies are essential to validate these findings and optimize therapeutic protocols.
Introduction
Lung cancer is one of the most common and deadliest malignant tumors worldwide, with an incidence and mortality rate of 11% and 20% in the United States in 2024, respectively, and this disease shows an increasing trend in incidence among females in China (1, 2). Pathologically, it can be broadly categorized into small cell lung cancer (SCLC) and non-small cell lung cancer (NSCLC), with NSCLC accounting for approximately 85% (3). NSCLC includes histological types such as adenocarcinoma and squamous cell carcinoma, with adenocarcinoma being the most prevalent histological subtype (4). In recent years, cancer has become a leading cause of death in China, and the 5-year survival rate for advanced NSCLC remains low (5, 6). Due to the high incidence and low survival rates of NSCLC in China, significant advancements have been made in the treatment of NSCLC in recent years. Surgery remains the primary treatment modality for early to mid-stage NSCLC, while chemotherapy and radiation therapy are recommended as conventional treatments for locally advanced or metastatic NSCLC. The development of targeted therapies has shifted treatment strategies from traditional chemotherapy and radiation towards gene mutation-directed targeted therapies. For example, NSCLC with EGFR gene-sensitive mutations can be treated with tyrosine kinase inhibitors (TKIs) (7, 8). Tumor therapies are evolving rapidly, and combination therapy strategies are becoming an emerging trend (9). In recent years, with the breakthrough progress in immunotherapy, it has also been gradually employed in the treatment of lung cancer patients, notably through the use of immune checkpoint inhibitors (ICIs). However, studies have shown that the efficacy of using ICIs alone may not be satisfactory (10, 11). The response rate of ICI monotherapy in solid tumors is only about 20% due to the development of primary or secondary resistance, the specific mechanisms of which involve the loss of antigens from tumor cells, the infiltration of immunosuppressive cells and the formation of an immunosuppressive microenvironment. Consequently, various methods have been explored to enhance the effectiveness of immunotherapy. In 2015, a study of 41 patients with metastatic solid tumors showed that local radiotherapy combined with granulocyte-macrophage colony-stimulating factor (GMCSF) produced an abscopal effect in 27% of patients, which demonstrates the synergistic effect of immunotherapy and radiotherapy (12). Since then, there has been a growing interest in ICIs combined with radiation therapy (RT), known as radioimmunotherapy (iRT), and many clinical trials have achieved encouraging conclusions (13–15).
The mechanism of radiotherapy combined with immunotherapy
Radiotherapy remains one of the essential treatment modalities for malignant tumors (16). “Abscopal effect (AE)” refers to the phenomenon that when a tumor receives local radiotherapy, the distal unirradiated tumor also shrinks or disappears. This observation is attributed to the systemic immune response triggered by local radiotherapy (17, 18). This potential systemic anti-tumor response provides robust evidence for the combination of radiotherapy and immunotherapy. To fully harness the clinical potential of the anti-tumor immune response induced by radiotherapy, it is crucial to understand the mechanism of action of radioimmunotherapy (iRT) (Figure 1).
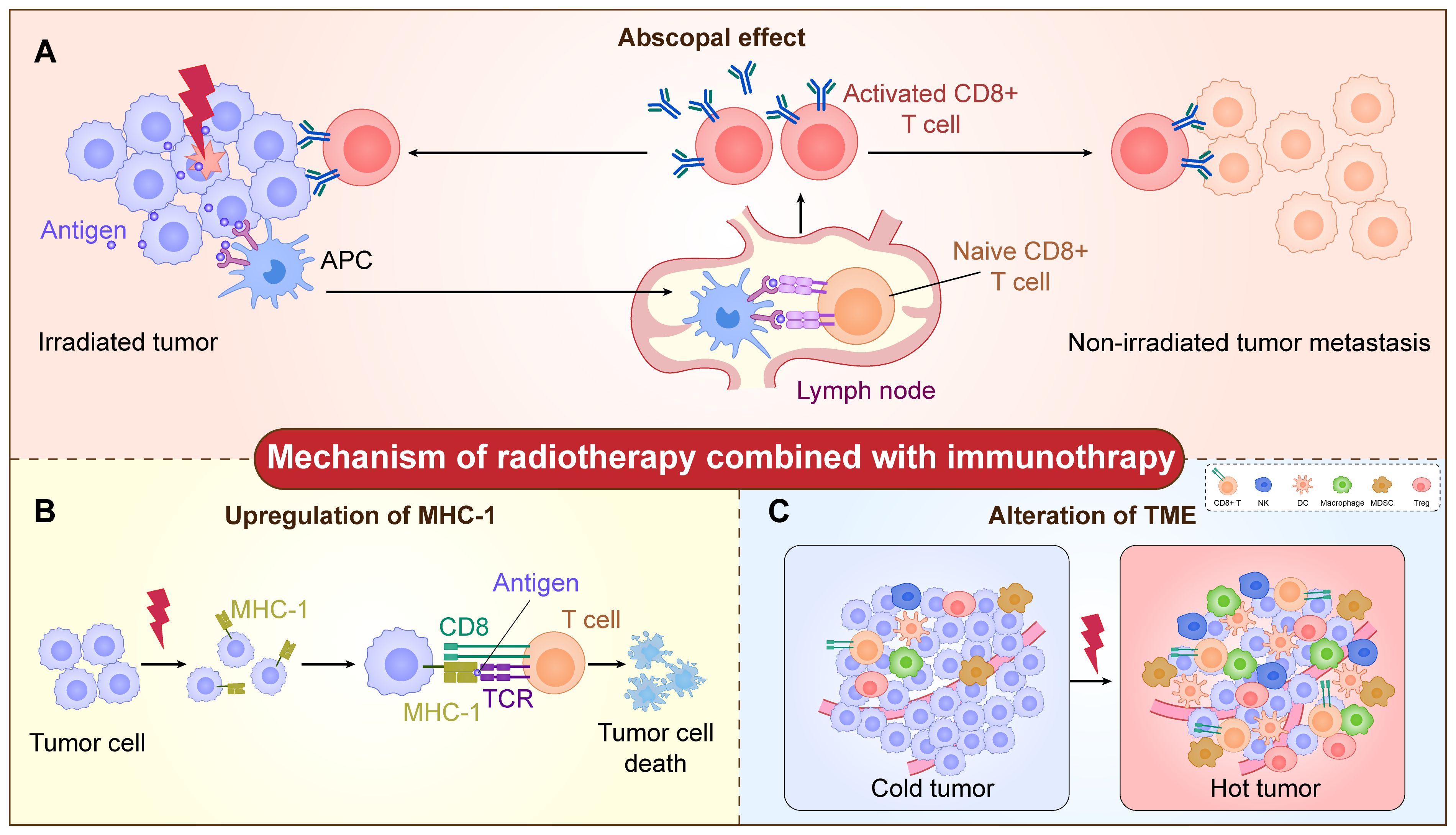
Figure 1. The mechanism of radiotherapy combined with immunotherapy. (A) The radiation increases tumor antigen exposure. Antigens from damaged tumor cells can be taken up by antigen-presenting cells (APCs) and presented to native T cells, which leads to the activation of T cells. Subsequently, the activated T cells targeting tumor-specific antigens infiltrate into both the primary tumor and unirradiated metastatic lesions, thereby triggering the abscopal effect. (B) The radiation increases the expression of MHC-I on the tumor cell surface and modulates the process of antigen presentation. The MHC-I-positive tumor cells are then recognized and cleared by CD8+ T cells. (C) The radiation increases the infiltration of immune cells, including CD8+ T cells, NK cells, DC cells, macrophages, Treg-cells, MDSCs, et al., which changes the microenvironment from “cold tumor” into “hot tumor”.
The “abscopal effect” of radiotherapy
The first clinical case of the AE was reported by Nobler in 1963. A patient with malignant lymphoma received radiotherapy to the abdomen, and the distant bilateral pleural effusions subsided (19). Later, the AE was observed in a series of tumors including non-small cell lung cancer, breast cancer, thymic cancer and melanoma. For example, a lung adenocarcinoma patient with brain metastases underwent radiotherapy to the metastatic lesion. The size of the primary lung lesion was reduced by 40% after completing whole‐brain irradiation (20). The mechanisms of the AE involve complex immune system activation, alterations in the tumor microenvironment, and the interaction of multiple molecular and cellular signals. Radiotherapy-induced immunogenic cell death (ICD) of tumor cells is one of the core mechanisms of AE. ICD refers to the specific way in which tumor cells die in response to injuries such as radiotherapy, releasing a series of damage-associated molecular patterns (DAMPs). These DAMPs have potent immunostimulatory functions and can activate both innate and adaptive immune responses, which in turn trigger systemic anti-tumor effects (20). In addition, radiotherapy induces the conversion of tumor-associated macrophages (TAM) from M2 (pro-tumor) to M1 (anti-tumor) and removes immunosuppressive cells, all of which contribute to the development of AE.
Radiation therapy increases the expression of MHC class I molecules on the surface of tumor cells
In recent years, with the breakthrough progress in immunotherapy, immune checkpoint inhibitor (ICI) therapy has shown promising clinical responses in the treatment of various cancers. The initiation of an immune response begins with the recognition of tumor-specific antigens by major histocompatibility complex class I (MHC-I) molecules. T cells play a central role in the host immune system. Upon interaction of MHC-I and T cell receptors, the immune response is initiated with certain other additional stimuli. As a result, MHC class I-positive tumor cells are recognized and cleared by T lymphocytes or other immune cells such as macrophages (21). The loss or downregulation of MHC-I will make the cancer less visible to the immune system. Thus, MHC-I expression deficiency is associated with resistance to checkpoint blockade and overt immunotherapy (22). In addition, during immunotherapy, regression of MHC-I-high metastases and progression of MHC-I-low metastases were observed. Furthermore, defects in the IFN response pathway, which regulates MHC-I levels, have also been correlated with resistance to checkpoint immunotherapy (23). The above evidence suggests that MHC-I loss significantly reduces ICI efficacy (24–26). Radiation therapy not only increases the expression of MHC-I but also modulates the process of antigen presentation on the cell surface, enhancing the sensitivity of immune cells to tumor cells, triggering anti-tumor immunity (27, 28).
Radiation therapy alters the tumor microenvironment
In addition to mediating DNA damage-induced cancer cell death, radiation therapy can modulate tumor immunogenicity and adjuvant properties by triggering the release of pro- and anti-inflammatory mediators, increasing immune stimulation and infiltration of immune-suppressing cells, thus transforming a “cold tumor” into a “hot tumor” and increasing tumor susceptibility to immunotherapy (29). Ionizing radiation-induced release of double-stranded DNA (dsDNA) and mitochondrial DNA (mtDNA) can activate the cGAS-STING signaling pathway and the type I IFN response, which are important for DC and T cell initiation (30, 31). Moreover, radiotherapy stimulates the release of chemokines such as CXCL9, CXCL10 and CXCL16 from tumor cells and stromal cells, which increase the infiltration of intratumoral NK, DC, and T cells, leading to enhanced anti-tumor immunity (32). However, radiation can also lead to the accumulation of immunosuppressive cell populations such as Treg and Myeloid-derived suppressor cells (MDSCs) in the tumor. The apoptotic cells that appear after RT can activate M2-type macrophages to secrete a range of anti-inflammatory cytokines such as TGF-β and IL-10, which limits the positive immunomodulatory effects of radiotherapy (33). Therefore, the effect of radiotherapy on immunotherapy is a “double-edged sword”, and it is necessary to comprehensively consider the effects of radiotherapy on cancer cells, immune cells, and various mesenchymal cells in the microenvironment, to achieve truly accurate iRT.
Metabolic reprogramming is the capacity of a cell to adjust its metabolism in response to various stimuli and stressors, allowing it to withstand external pressures and acquire new functions (34). It has been demonstrated that radiotherapy-mediated metabolic reprogramming is involved in immune regulation (35). Radiotherapy enhances the glycolysis of tumor cells (36). High glucose consumption leads to lactate production and secretion into the tumor microenvironment. Lactate exerts immunosuppressive effects by decreasing NK cell cytokinesis, increasing PD-1 expression, and activating Treg cells (37). Lactate also promotes the recruitment of MDSCs in tumor and spleen and induces “M2-like” polarization in tumor-associated macrophages (38). On the other hand, radiotherapy induces ferroptosis of tumor cells by promoting lipid peroxidation (39). Ferroptosis enhances the ability of innate immune cells to recognize tumor cells and initiates the adaptive immune response, which improves the “immune desert status” of TME. As a potential form of ICD, ferroptosis not only drives macrophage polarization toward the M1 phenotype, but also promotes intra-tumoral T cell infiltration and activation, and can even trigger a vaccine-like response, thus activating the anti-tumor immune responses (40). Taken together, radiotherapy-induced metabolic alterations play a complex and important role in immune regulation, and a better understanding of these mechanisms is essential for the development of more effective therapeutic strategies.
Combining radiotherapy with immunotherapy in clinical practice
Based on the regulatory effects of radiotherapy on the immune system, the current radioimmunotherapy (iRT) treatment approach has been widely used in the clinical treatment of various types of cancers. Radiotherapy combined with PD-1/PD-L1 antibody drugs has achieved significant success in malignant melanoma, non-small cell lung cancer (NSCLC), head and neck squamous cell carcinoma, and some solid tumors. Additionally, radiotherapy combined with CTLA-4 inhibitors has been extensively studied and applied in melanoma, lung cancer, and other cancers (41, 42). To maximize the advantages of the iRT treatment approach in clinical settings, it is essential to thoroughly investigate the factors influencing treatment efficacy. Determining the radiotherapy dose and fractionation, selecting the irradiation site, timing of radiotherapy, and choice of immunotherapy all need to be optimized through clinical practice to identify the best combinations (43).
Selection of radiotherapy regimens
The immune response induced by radiotherapy exhibits a “dose-dependent” nature (4), and extensive research has been conducted in clinical settings regarding the selection of radiotherapy doses and fractionation schemes. Many studies have shown that for activation of anti-tumor CD8+ T cell responses, a single high dose or large fractionated radiation is superior to conventional fractionated regimens (4). High-dose radiation induces enhanced double-strand breaks, leading to increased tumor cell death and release of tumor antigens and cytokines (e.g., IFN-γ), which promotes activation of cytotoxic CD8+ T cells (33). However, high-dose radiotherapy (≥15 Gy) might increase the proportion of Treg cells in the spleen, thereby suppressing anti-tumor immune responses (44). Therefore, the hypofractioned radiotherapy modality may be considered. A non-randomized observational study demonstrated that SBRT (48 Gy/6-8 F) induced peripheral blood T-cell activation in patients with early-stage NSCLC, mainly in increased PD-1 expression and proliferation (45). Allen et al. compared immune responses and tumor control rates with high-dose hypofractioned (8 Gy x 2) or low-dose daily fractionated (2 Gy x 10) IR combined with PD-1. The results showed that 8 Gy x 2 IR preserved peripheral and tumor-infiltrating CD8+ T lymphocyte activation and reduced MDSCs accumulation in comparison to 2 Gy x 10 IR (46). Furthermore, a clinical study conducted in advanced NSCLC showed that high-dose, hypofractioned SBRT (50 Gy/4 F) was superior to conventional radiotherapy (45 Gy/15 F) when combined with a PD-1 inhibitor in several efficacy endpoints (47). The above studies suggest that high-dose hypofractioned IR can preserve or enhance anti-tumor immunity and thus control primary and distant tumors. However, more efforts are needed to determine the optimal radiotherapy regimen.
In addition to the selection of radiotherapy doses and fractionation schemes, determining the irradiation site is also crucial. Lymphocyte reduction is one of the most common side effects of radiotherapy, and ensuring the integrity of the immune system is essential for effective tumor treatment. Therefore, it is important to protect lymphocytes during radiotherapy and immunotherapy to minimize damage to immune cells (48). For non-small cell lung cancer (NSCLC), in clinical practice, the primary tumor site is typically given a total dose of 50-70 Gy, while draining lymph nodes are usually irradiated with doses ranging from 45-50 Gy (49). However, since draining lymph nodes are crucial sites for T lymphocyte activation and aggregation, irradiation of tumor-free lymph nodes may inhibit the activation of anti-tumor immune responses. Furthermore, immune cells in the bone marrow and circulating in the bloodstream are highly sensitive to radiation therapy. Therefore, during radiotherapy, efforts should be made to avoid or minimize irradiation of the bone marrow and blood vessels (33).
In order to achieve the best therapeutic outcomes with radioimmunotherapy, the debate within the academic community revolves around whether these treatments should be administered synchronously or sequentially. There are numerous ongoing clinical trials exploring various combinations. For example, the PACIFIC study, which was used to evaluate the efficacy of using immunotherapy (primarily Durvalumab) after radiation therapy in patients with unresectable stage III non-small cell lung cancer (NSCLC), showed that rapid follow-up immunotherapy after radiation therapy significantly prolongs progression disease-free survival (PFS) and improves the overall survival (OS) of patients, with a manageable safety and tolerability profile (50). Another real-world study on stage III NSCLC using durvalumab consolidation after chemoradiotherapy showed a 14.3% incidence of grade 3 radiation pneumonitis in the experimental group compared to 2.5% in the observation group (51). Furthermore, results from the phase I clinical trial KEYNOTE-001 indicate that patients who received any form of radiotherapy before undergoing pembrolizumab immunotherapy experienced prolonged PFS (52). However, in patients with metastatic head and neck squamous cell carcinoma (HNSCC), simultaneous administration of nivolumab with stereotactic body radiotherapy (SBRT) did not improve objective response rates (ORR) compared to nivolumab monotherapy (53). A large number of relevant clinical trials are currently underway. For instance, A single-arm phase II trial called “Chemotherapy and Immunotherapy Induction Followed by Hypo-radiotherapy and Immunotherapy Maintenance in Locally Advanced NSCLC” (NCT05784142) was designed to determine the efficacy and safety of combining immunotherapy in association with standard chemotherapy and subsequently with hypoRT, followed by a treatment of maintenance with only immunotherapy. The investigators want to exploit the survival benefit effect of immuno-chemotherapy plus sequential hypoRT in LA-NSCLC. Another phase III clinical study “A Randomized Trial of Consolidative Immunotherapy With vs Without Thoracic Radiotherapy and/or Stereotactic Body Radiation Therapy (SBRT) After First-Line Systemic Therapy for Metastatic NSCLC” (NCT03867175) aims to compare progression-free survival of patients randomized to radiation and consolidative immunotherapy against those receiving consolidative immunotherapy alone. And to find out how effective immunotherapy and stereotactic radiotherapy are in treating patients with stage IV non-small cell lung cancer compared to immunotherapy alone after first-line systemic therapy (therapy that goes throughout the body). As a result, the optimal sequence for combining radiotherapy and immunotherapy remains undetermined, and large-scale clinical trials are still underway to investigate the optimal timing of administration of radiation and immunotherapy to improve oncologic outcomes, which may lead to new thinking in clinical care.
Selection of immunotherapy regimens
Radiotherapy can induce anti-tumor immune responses, and a commonly studied approach is the combination of radiotherapy with immune checkpoint inhibitors (ICIs), with the most common being PD-1/PD-L1 and CTLA-4 inhibitors.
PD-1/PD-L1 immune checkpoint inhibitors
Under normal circumstances, the binding of PD-L1 on the surface of tissue cells with PD-1 on the surface of lymphocytes can inhibit lymphocyte function, playing a role in immune tolerance. However, many tumor cells also express PD-L1 on their surface, which can bind to PD-1 on corresponding lymphocytes, inhibiting lymphocyte function, cytokine release, and inducing lymphocyte apoptosis. This process leads to immune escape by tumor cells (54). PD-1/PD-L1 inhibitors work by disrupting these mechanisms to achieve the desired effects in cancer immunotherapy.
CTLA-4 immune checkpoint inhibitors
CTLA-4, expressed on the surface of activated T lymphocytes, can competitively bind to B7 molecules with CD28, inhibiting T cell activation and the secretion of interleukin IL-2. Additionally, CTLA-4 can bind to CD80/CD86 to inhibit T cell activation. These actions suppress the body’s anti-tumor immune response (55, 56). CTLA-4 inhibitors work by enhancing anti-tumor effects and boosting intra-tumoral immune responses through the mechanisms described above.
In the treatment of metastatic NSCLC, a retrospective analysis has indicated that the combination of PD-1/PD-L1 immune checkpoint inhibitors with SBRT is significantly superior to the combination of CTLA-4 immune checkpoint inhibitors with SBRT. However, this view still requires support from prospective research data (41). The CheckMate-73L study has highlighted that in the treatment of unresectable stage III NSCLC patients, the safety of the PD-1/PD-L1+CTLA-4 dual immunotherapy regimen compared to PD-1/PD-L1 inhibitor monotherapy as consolidation therapy after concurrent chemoradiotherapy was evaluated. Based on the phase II results, the dual immunotherapy group exhibited a higher incidence of grade 3 adverse events, leading to a higher discontinuation rate compared to the monotherapy group (57). In summary, compared to CTLA-4 inhibitors, PD-1/PD-L1 inhibitors in combination with radiotherapy typically have lower immune-related toxicity, are easier to administer and more highly targeted. Radiotherapy increases tumor antigen release, PD-1/PD-L1 inhibitors can deregulate T-cell suppression and synergistically enhance anti-tumor immune responses. In addition, in non-small cell lung cancer, a large number of clinical trial data support that the combination of PD-1/PD-L1 inhibitors and radiotherapy improves overall survival (OS) and progression-free survival (PFS), and significantly improves patients’ quality of life. Therefore, PD-1/PD-L1 inhibitors are generally favored for their established efficacy in NSCLC.
Safety of radiotherapy combined with immunotherapy
The damage caused by radiotherapy is mainly attributed to the radiation site, which may result in corresponding injuries to the brain, heart, lungs, liver, and other areas. One of the most severe injuries is radiation-induced lung injury (RILI), including radiation pneumonitis and radiation pulmonary fibrosis (58). On the other hand, immunotherapy-related injuries encompass checkpoint inhibitor pneumonitis, colitis, hepatitis, immune checkpoint inhibitor-related endocrine disorders, and skin toxicity. However, it is important to draw our attention to the fact that both radiotherapy and ICIs can lead to lung inflammation, which may be an increased risk when combined, and that ICIs may trigger immune-mediated colitis manifested by diarrhea and intestinal discomfort (59). The combination of the two may lead to a wider range of inflammatory responses, requiring detection and early intervention to effectively control and minimize the occurrence of these adverse effects. Monitoring biomarkers of toxicity is essential for managing adverse effects in radiotherapy and immunotherapy, for example, by monitoring biomarkers such as circulating lymphocytes, immune-related toxicity can be recognized early and radiotherapy and immunotherapy doses can be adjusted appropriately, depending on the patient’s response and biomarker levels (60). Therefore, assessing the safety of radiotherapy combined with immunotherapy (iRT) treatment is crucial for clinical application, with radiation pneumonitis being a key concern in clinical research.
However, currently, there is no evidence indicating that the toxicity of iRT is significantly higher than the toxicity of using ICIs or RT alone (61, 62). Moreover, data from numerous clinical studies suggest that iRT typically results in grades 1 to 2 toxicity, including fatigue, skin reactions, gastrointestinal symptoms, and respiratory symptoms, which can usually be effectively managed with symptomatic treatment and supportive care. However, grade 3 (requiring medical support) or life-threatening grade 4 toxicities such as severe pneumonia or colitis are relatively rare (63). Additionally, existing clinical data indicate that iRT may be well tolerated and associated with acceptable toxicity across different types of cancer (64). Therefore, more clinical data are needed to evaluate the survival benefits and safety of iRT.
Clinical advances of radiotherapy combined with immunotherapy in NSCLC
With the simultaneous exploration of multiple treatment modalities, the efficacy of targeted/immunotherapy in the perioperative period is gradually becoming prominent. Several clinical trials have been conducted in different stages of non-small cell lung cancer (Table 1).
iRT Treatment for early-stage NSCLC
The primary treatment for patients with early-stage NSCLC is surgical resection, but some patients still develop recurrent metastases after surgery, and many clinical trials have attempted to improve prognosis by adding adjuvant or neoadjuvant therapy. Stereotactic ablative radiotherapy (SABR) is the standard treatment for inoperable early-stage NSCLC, but regional and distant recurrent metastases are also common. Several studies have shown that immunotherapy reduces recurrence and improves patient survival after radiotherapy, and a randomized phase II trial by Joe Y Chang et al. demonstrated a significant improvement in the 4-year EFS rate and tolerable toxicity with the combination of immunotherapy and SABR (65). Another randomized phase I trial on synchronous or sequential treatment with SBRT also suggested that multimodal therapy could control metastasis and improve overall survival (66). Thus, iRT can be used in early-stage NSCLC to improve postoperative recurrence and prolong survival. The phase II clinical study ASTEROID was designed to evaluate the efficacy and safety of sequential Durvalumab treatment after SBRT in NSCLC patients with cT1~2N0M0, and the preliminary results confirmed the feasibility of SBRT in combination with Durvalumab treatment (67).
iRT treatment for locally advanced NSCLC
Due to the insidious nature of NSCLC, a majority of patients awaiting diagnosis clinically are in the advanced stages, with locally advanced NSCLC accounting for approximately 30% of all NSCLC cases. The Durvalumab treatment arm of the PACIFIC study demonstrated significantly prolonged median overall survival (OS) and progression-free survival (PFS), with a higher 4-year PFS rate. This study established concurrent chemoradiotherapy combined with immune consolidation therapy as the standard treatment for inoperable locally advanced NSCLC (43). The LUN14-179 study (68) and the DETERRED study (69) also confirmed the effectiveness of this treatment approach. Additionally, the DOLPHIN study (70) and the PACIFIC-6 study (71) noted that Durvalumab immunotherapy combined with radical radiotherapy was synergistic and tolerable in patients with unresectable locally advanced NSCLC.
In summary, the strategy of immune consolidation therapy following concurrent chemoradiotherapy has significantly improved the progression-free survival (PFS) and overall survival (OS) of patients with inoperable locally advanced NSCLC. However, clinicians still need to be highly aware of the adverse effects associated with this mode of treatment.
iRT treatment for advanced NSCLC
In the treatment of advanced NSCLC, the focus of clinical research is on the combination of SBRT with immune consolidation therapy. In a secondary analysis of KEYNOTE-001, it was found that patients with advanced NSCLC who had received any area or extracranial radiotherapy in addition to Pembrolizumab treatment showed significant improvements in overall survival (OS) and progression-free survival (PFS) compared to those who had only received Pembrolizumab (72). In addition to this, in a phase III placebo-controlled PACIFIC trial, researchers found that consolidation of durvalumab treatment after radiotherapy significantly improved PFS and OS with a manageable safety profile (73). The SICI study also indicated that in patients with advanced NSCLC, our combination of durvalumab, tremelimumab and SBRT use is safe and feasible for primary tumors (74).
With the advancement of radiotherapy technology, the application of Stereotactic Body Radiation Therapy (SBRT) in advanced NSCLC is becoming increasingly widespread. Theelen conducted a combined analysis of data from the PEMBRO-RT and MDACC clinical studies involving the treatment of advanced NSCLC patients with radiotherapy combined with Pembrolizumab. The results demonstrated that SBRT combined with Immune Checkpoint Inhibitors (ICIs) significantly improved the prognosis of patients with advanced NSCLC (75).
Conclusion and future perspectives
According to current clinical data, the combination of radiotherapy and immunotherapy is considered to be an effective treatment for NSCLC. Several studies have shown that iRT can significantly prolong the overall survival (OS) and progression-free survival (PFS) of patients with advanced NSCLC with an acceptable safety profile, and not only improve local tumor control, but also induce systemic immune response and control distant metastasis through the “abscopal effect”. However, the most effective combination and sequence of iRT in non-small cell lung cancer (NSCLC) requires comprehensive clinical trial data to guide clinical protocols.
iRT is regarded as a potential breakthrough in the field of tumor therapy, which can improve the therapeutic effect and reduce the therapeutic resistance. In the direction of future development, on the one hand, biomarkers predicting the combined application of radiation therapy and immunotherapy can be investigated, which may involve factors such as the immune characteristics of the tumor microenvironment and the genetic variation of tumor cells (76). On the other hand, the application of iRT in other solid tumors can be explored, including but not limited to lung cancer, melanoma, pancreatic cancer. Through clinical trials and studies, the efficacy of combination therapy in different cancer types can be verified and the best treatment options can be explored (Reardon, 2024)1. There is also a need to explore new therapeutic strategies, such as enhancing the effect of immunotherapy through gene editing technology, or using nanotechnology to improve the targeting of radiation therapy (Liu et al., 2024)2. The research and practice of the above development directions can further promote the development of the combined application of radiation therapy and immunotherapy, provide more effective treatment options for tumor patients, and bring new breakthroughs in the field of solid tumor treatment.
Author contributions
HX: Writing – original draft, Writing – review & editing. YC: Funding acquisition, Resources, Supervision, Writing – review & editing. YZ: Conceptualization, Visualization, Writing – review & editing.
Funding
The author(s) declare financial support was received for the research, authorship, and/or publication of this article. This work was supported by the National Natural Science Foundation of China (82303828).
Conflict of interest
The authors declare that the research was conducted in the absence of any commercial or financial relationships that could be construed as a potential conflict of interest.
Generative AI statement
The author(s) declare that no Generative AI was used in the creation of this manuscript.
Publisher’s note
All claims expressed in this article are solely those of the authors and do not necessarily represent those of their affiliated organizations, or those of the publisher, the editors and the reviewers. Any product that may be evaluated in this article, or claim that may be made by its manufacturer, is not guaranteed or endorsed by the publisher.
Abbreviations
NSCLC, Non-small cell lung cancer; ICI, Immune checkpoint inhibitor; iRT, Radioimmunotherapy; MHC-I, Major histocompatibility complex class I; TKIs, Tyrosine kinase inhibitors; RT, radiation therapy; AE, abscopal effect; ICD, Immunogenic cell death; Tregs, immunosuppressive regulatory T cells; TAMs, Tumor-associated macrophages; MDSCs, Myeloid-derived suppressor cells; SBRT, Stereotactic body radiotherapy; PFS, Progression-free survival; HNSCC, Head and neck squamous cell carcinoma; ORR, Objective response rates; RILI, Radiation-induced lung injury; DFS, Disease-free survival; OS, Overall survival.
Footnotes
- ^ Reardon S. (2024). First cell therapy for solid tumours heads to the clinic: what it means for cancer treatment. Nature. (Ahead of print). doi: 10.1038/ d41586-024-00673-w
- ^ Liu H, Guo Z, Wang P. (2024). Genetic expression in cancer research: Challenges and complexity. Gene Rep. (Ahead of print). doi: 10.1016/j.genrep.2024.102042
References
1. Siegel RL, Giaquinto AN, Jemal A. Cancer statistics. CA Cancer J Clin. (2024) 74:12–49. doi: 10.3322/caac.21820
2. Teng Y, Xia C, Cao M, Yang F, Yan X, He S, et al. Lung cancer burden and trends from 2000 to 2018 in China: Comparison between China and the United States. Chin J Cancer Res. (2023) 35:618–26. doi: 10.21147/j.issn.1000-9604.2023.06.06
3. Li C, Lei S, Ding L, Xu Y, Wu X, Wang H, et al. Global burden and trends of lung cancer incidence and mortality. Chin Med J (Engl). (2023) 136:1583–90. doi: 10.1097/CM9.0000000000002529
4. Zhang Z, Liu X, Chen D, Yu J. Radiotherapy combined with immunotherapy: the dawn of cancer treatment. Signal Transduct Target Ther. (2022) 7:258. doi: 10.1038/s41392-022-01102-y
5. Lahiri A, Maji A, Potdar PD, Singh N, Parikh P, Bisht B, et al. Lung cancer immunotherapy: progress, pitfalls, and promises. Mol Cancer. (2023) 22:40. doi: 10.1186/s12943-023-01740-y
6. Qi J, Li M, Wang L, Hu Y, Liu W, Long Z, et al. National and subnational trends in cancer burden in China, 2005-20: an analysis of national mortality surveillance data. Lancet Public Health. (2023) 8:e943–55. doi: 10.1016/S2468-2667(23)00211-6
7. Osmani L, Askin F, Gabrielson E, Li QK. Current WHO guidelines and the critical role of immunohistochemical markers in the subclassification of non-small cell lung carcinoma (NSCLC): Moving from targeted therapy to immunotherapy. Semin Cancer Biol. (2018) 52:103–9. doi: 10.1016/j.semcancer.2017.11.019
8. Imyanitov EN, Iyevleva AG, Levchenko EV. Molecular testing and targeted therapy for non-small cell lung cancer: Current status and perspectives. Crit Rev Oncol Hematol. (2021) 157:103194. doi: 10.1016/j.critrevonc.2020.103194
9. Sonkin D, Thomas A, Teicher BA. Cancer treatments: Past, present, and future. Cancer Genet. (2024) 286-287:18–24. doi: 10.1016/j.cancergen.2024.06.002
10. Weiner LM. Cancer immunotherapy–the endgame begins. N Engl J Med. (2008) 358:2664–5. doi: 10.1056/NEJMp0803663
11. Shankar B, Zhang J, Naqash AR, Forde PM, Feliciano JL, Marrone KA, et al. Multisystem immune-related adverse events associated with immune checkpoint inhibitors for treatment of non-small cell lung cancer. JAMA Oncol. (2020) 6:1952–6. doi: 10.1001/jamaoncol.2020.5012
12. Golden EB, Chhabra A, Chachoua A, Adams S, Donach M, Fenton-Kerimian M, et al. Local radiotherapy and granulocyte-macrophage colony-stimulating factor to generate abscopal responses in patients with metastatic solid tumours: a proof-of-principle trial. Lancet Oncol. (2015) 16:795–803. doi: 10.1016/S1470-2045(15)00054-6
13. Wang H, Yao Z, Kang K, Zhou L, Xiu W, Sun J, et al. Preclinical study and phase II trial of adapting low-dose radiotherapy to immunotherapy in small cell lung cancer. Med. (2024) 5:1237–1254.e9. doi: 10.1016/j.medj.2024.06.002
14. Mortensen REJ, Holmström MO, Lisle TL, Hasselby JP, Willemoe GL, Met Ö, et al. Pre-existing TGF-β-specific T-cell immunity in patients with pancreatic cancer predicts survival after checkpoint inhibitors combined with radiotherapy. J Immunother Cancer. (2023) 11:e006432. doi: 10.1136/jitc-2022-006432
15. Guan J, Sun K, Guerrero CA, Zheng J, Xu Y, Mathur S, et al. A phase 2 study of in situ oncolytic virus therapy and stereotactic body radiation therapy followed by pembrolizumab in metastatic non-small cell lung cancer. Int J Radiat Oncol Biol Phys. (2024) 118:1531–40. doi: 10.1016/j.ijrobp.2023.08.044
16. Citrin DE. Recent developments in radiotherapy. N Engl J Med. (2017) 377:1065–75. doi: 10.1056/NEJMra1608986
17. Janopaul-Naylor JR, Shen Y, Qian DC, Buchwald ZS. The abscopal effect: A review of pre-clinical and clinical advances. Int J Mol Sci. (2021) 22:11061. doi: 10.3390/ijms222011061
18. Nelson BE, Adashek JJ, Lin SH, Subbiah V. The abscopal effect in patients with cancer receiving immunotherapy. Med. (2023) 4:233–44. doi: 10.1016/j.medj.2023.02.003
19. van der Woude LL, Gorris MAJ, Wortel IMN, Creemers JHA, Verrijp K, Monkhorst K, et al. Tumor microenvironment shows an immunological abscopal effect in patients with NSCLC treated with pembrolizumab-radiotherapy combination. J Immunother Cancer. (2022) 10:e005248. doi: 10.1136/jitc-2022-005248
20. Hotta T, Okuno T, Nakao M, Amano Y, Isobe T, Tsubata Y. Reproducible abscopal effect in a patient with lung cancer who underwent whole-brain irradiation and atezolizumab administration. Thorac Cancer. (2021) 12:985–8. doi: 10.1111/1759-7714.13875
21. Erdogdu IH. MHC class 1 and PDL-1 status of primary tumor and lymph node metastatic tumor tissue in gastric cancers. Gastroenterol Res Pract. (2019) 2019:4785098. doi: 10.1155/2019/4785098
22. DhatChinamoorthy K, Colbert JD, Rock KL. Cancer immune evasion through loss of MHC class I antigen presentation. Front Immunol. (2021) 12:636568. doi: 10.3389/fimmu.2021.636568
23. Gao J, Shi LZ, Zhao H, Chen J, Xiong L, He Q, et al. Loss of IFN-γ Pathway genes in tumor cells as a mechanism of resistance to anti-CTLA-4 therapy. Cell. (2016) 167:397–404.e9. doi: 10.1016/j.cell.2016.08.069
24. Chen X, Lu Q, Zhou H, Liu J, Nadorp B, Lasry A, et al. A membrane-associated MHC-I inhibitory axis for cancer immune evasion. Cell. (2023) 186:3903–3920.e21. doi: 10.1016/j.cell.2023.07.016
25. Dersh D, Hollý J, Yewdell JW. A few good peptides: MHC class I-based cancer immunosurveillance and immunoevasion. Nat Rev Immunol. (2021) 21:116–28. doi: 10.1038/s41577-020-0390-6
26. Gu SS, Zhang W, Wang X, Jiang P, Traugh N, Li Z, et al. Therapeutically increasing MHC-I expression potentiates immune checkpoint blockade. Cancer Discovery. (2021) 11:1524–41. doi: 10.1158/2159-8290.CD-20-0812
27. Zhang Q, Wu S, Zhang J. New insight on tumor microenvironment remodelling and augmented therapeutic efficacy of immunotherapy by radiotherapy. Cancer Res Prev Treat. (2021) 48:1–6. doi: 10.3971/j.issn.1000-8578.2021.20.0836
28. Vanpouille-Box C, Formenti SC, Demaria S. Toward precision radiotherapy for use with immune checkpoint blockers. Clin Cancer Res. (2018) 24:259–65. doi: 10.1158/1078-0432.CCR-16-0037
29. McLaughlin M, Patin EC, Pedersen M, Wilkins A, Dillon MT, Melcher AA, et al. Inflammatory microenvironment remodelling by tumour cells after radiotherapy. Nat Rev Cancer. (2020) 20:203–17. doi: 10.1038/s41568-020-0246-1
30. Li W, Wu H, Xu J. Construction of a genomic instability-derived predictive prognostic signature for non-small cell lung cancer patients. Cancer Genet. (2023) 278-279:24–37. doi: 10.1016/j.cancergen.2023.07.008
31. Chen W, Wang Y, Zheng J, Chen Y, Zhang C, Yang W, et al. Characterization of cellular senescence in radiation ulcers and therapeutic effects of mesenchymal stem cell-derived conditioned medium. Burns Trauma. (2023) 11:tkad001. doi: 10.1093/burnst/tkad001
32. Jarosz-Biej M, Smolarczyk R, Cichoń T, Kułach N. Tumor microenvironment as A “Game changer” in cancer radiotherapy. Int J Mol Sci. (2019) 20:3212. doi: 10.3390/ijms20133212
33. Zhai D, An D, Wan C, Yang K. Radiotherapy: Brightness and darkness in the era of immunotherapy. Transl Oncol. (2022) 19:101366. doi: 10.1016/j.tranon.2022.101366
34. Wang Z, Zhao F, Xu C, Zhang Q, Ren H, Huang X, et al. Metabolic reprogramming in skin wound healing. Burns Trauma. (2024) 12:tkad047. doi: 10.1093/burnst/tkad047
35. Liu S, Wang W, Hu S, Jia B, Tuo B, Sun H, et al. Radiotherapy remodels the tumor microenvironment for enhancing immunotherapeutic sensitivity. Cell Death Dis. (2023) 14:679. doi: 10.1038/s41419-023-06211-2
36. Jin Z, Liu YH. Metabolic-related gene signatures for survival prediction and immune cell subtypes associated with prognosis in intrahepatic cholangiocarcinoma. Cancer Genet. (2023) 274-275:84–93. doi: 10.1016/j.cancergen.2023.04.001
37. Chen S, Xu Y, Zhuo W, Zhang L. The emerging role of lactate in tumor microenvironment and its clinical relevance. Cancer Lett. (2024) 590:216837. doi: 10.1016/j.canlet.2024.216837
38. Chaudagar K, Hieromnimon HM, Khurana R, Labadie B, Hirz T, Mei S, et al. Reversal of lactate and PD-1-mediated macrophage immunosuppression controls growth of PTEN/p53-deficient prostate cancer. Clin Cancer Res. (2023) 29:1952–68. doi: 10.1158/1078-0432.CCR-22-3350
39. Chen X, Kang R, Kroemer G, Tang D. Broadening horizons: the role of ferroptosis in cancer. Nat Rev Clin Oncol. (2021) 18:280–96. doi: 10.1038/s41571-020-00462-0
40. Zhao L, Zhou X, Xie F, Zhang L, Yan H, Huang J, et al. Ferroptosis in cancer and cancer immunotherapy. Cancer Commun (Lond). (2022) 42:88–116. doi: 10.1002/cac2.12250
41. Chen D, Menon H, Verma V, Guo C, Ramapriyan R, Barsoumian H, et al. Response and outcomes after anti-CTLA4 versus anti-PD1 combined with stereotactic body radiation therapy for metastatic non-small cell lung cancer: retrospective analysis of two single-institution prospective trials. J Immunother Cancer. (2020) 8:e000492. doi: 10.1136/jitc-2019-000492
42. Marconcini R, Spagnolo F, Stucci LS, Ribero S, Marra E, Rosa F, et al. Current status and perspectives in immunotherapy for metastatic melanoma. Oncotarget. (2018) 9:12452–70. doi: 10.18632/oncotarget.23746
43. Demaria S, Guha C, Schoenfeld J, Morris Z, Monjazeb A, Sikora A, et al. Radiation dose and fraction in immunotherapy: one-size regimen does not fit all settings, so how does one choose? J Immunother Cancer. (2021) 9:e002038. doi: 10.1136/jitc-2020-002038
44. Chen Y, Gao M, Huang Z, Yu J, Meng X. SBRT combined with PD-1/PD-L1 inhibitors in NSCLC treatment: a focus on the mechanisms, advances, and future challenges. J Hematol Oncol. (2020) 13:105. doi: 10.1186/s13045-020-00940-z
45. de Goeje PL, Smit EF, Waasdorp C, Schram MTB, Kaijen-Lambers MEH, Bezemer K, et al. Stereotactic ablative radiotherapy induces peripheral T-cell activation in patients with early-stage lung cancer. Am J Respir Crit Care Med. (2017) 196:1224–7. doi: 10.1164/rccm.201610-2178LE
46. Morisada M, Clavijo PE, Moore E, Sun L, Chamberlin M, Van Waes C, et al. PD-1 blockade reverses adaptive immune resistance induced by high-dose hypofractionated but not low-dose daily fractionated radiation. Oncoimmunology. (2017) 7:e1395996. doi: 10.1080/2162402X.2017.1395996
47. Welsh J, Menon H, Chen D, Verma V, Tang C, Altan M, et al. Pembrolizumab with or without radiation therapy for metastatic non-small cell lung cancer: a randomized phase I/II trial. J Immunother Cancer. (2020) 8:e001001. doi: 10.1136/jitc-2020-001001
48. Xia WY, Feng W, Zhang CC, Shen YJ, Zhang Q, Yu W, et al. Radiotherapy for non-small cell lung cancer in the immunotherapy era: the opportunity and challenge-a narrative review. Transl Lung Cancer Res. (2020) 9:2120–36. doi: 10.21037/tlcr-20-827
49. Bentzen SM, Harari PM, Bernier J. Exploitable mechanisms for combining drugs with radiation: concepts, achievements and future directions. Nat Clin Pract Oncol. (2007) 4:172–80. doi: 10.1038/ncponc0744
50. Antonia SJ, Villegas A, Daniel D, Vicente D, Murakami S, Hui R, et al. Durvalumab after chemoradiotherapy in stage III non-small-cell lung cancer. N Engl J Med. (2017) 377:1919–29. doi: 10.1056/NEJMoa1709937
51. Jung HA, Noh JM, Sun JM, Lee SH, Ahn JS, Ahn MJ, et al. Real world data of durvalumab consolidation after chemoradiotherapy in stage III non-small-cell lung cancer. Lung Cancer. (2020) 146:23–9. doi: 10.1016/j.lungcan.2020.05.035
52. Garon EB, Hellmann MD, Rizvi NA, Carcereny E, Leighl NB, Ahn MJ, et al. Five-year overall survival for patients with advanced non-Small-cell lung cancer treated with pembrolizumab: results from the phase I KEYNOTE-001 study. J Clin Oncol. (2019) 37:2518–27. doi: 10.1200/JCO.19.00934
53. McBride S, Sherman E, Tsai CJ, Baxi S, Aghalar J, Eng J, et al. Randomized phase II trial of nivolumab with stereotactic body radiotherapy versus nivolumab alone in metastatic head and neck squamous cell carcinoma. J Clin Oncol. (2021) 39:30–7. doi: 10.1200/JCO.20.00290
54. Yi M, Zheng X, Niu M, Zhu S, Ge H, Wu K. Combination strategies with PD-1/PD-L1 blockade: current advances and future directions. Mol Cancer. (2022) 21:28. doi: 10.1186/s12943-021-01489-2
55. Wei SC, Duffy CR, Allison JP. Fundamental mechanisms of immune checkpoint blockade therapy. Cancer Discovery. (2018) 8:1069–86. doi: 10.1158/2159-8290.CD-18-0367
56. Ribas A. Releasing the brakes on cancer immunotherapy. N Engl J Med. (2015) 373:1490–2. doi: 10.1056/NEJMp1510079
57. Yan M, Durm GA, Mamdani H, Ernani V, Jabbour SK, Naidoo J, et al. Consolidation nivolumab/ipilimumab versus nivolumab following concurrent chemoradiation in patients with unresectable stage III NSCLC: A planned interim safety analysis from the BTCRC LUN 16-081 trial. J Clin Oncol. (2020) 38:9010–0. doi: 10.1200/JCO.2020.38.15suppl.9010
58. Zhang Z, Zhou J, Verma V, Liu X, Wu M, Yu J, et al. Crossed pathways for radiation-induced and immunotherapy-related lung injury. Front Immunol. (2021) 12:774807. doi: 10.3389/fimmu.2021.774807
59. Wang Y, Han G. Advances in research on treatment-associated pneumonitis in lung cancer patients treated with radiation combined with immunotherapy. Radiat Med Protection. (2024) 44:711–7. doi: 10.3760/cma.j.cn112271-20240127-00034
60. Lawrence R, Watters M, Davies CR, Pantel K, Lu YJ. Circulating tumour cells for early detection of clinically relevant cancer. Nat Rev Clin Oncol. (2023) 20:487–500. doi: 10.1038/s41571-023-00781-y
61. Reardon DA, Brandes AA, Omuro A, Mulholland P, Lim M, Wick A, et al. Effect of nivolumab vs bevacizumab in patients with recurrent glioblastoma: the checkMate 143 phase 3 randomized clinical trial. JAMA Oncol. (2020) 6:1003–10. doi: 10.1001/jamaoncol.2020.1024
62. Yuan Z, Fernandez D, Dhillon J, Abraham-Miranda J, Awasthi S, Kim Y, et al. Proof-of-principle Phase I results of combining nivolumab with brachytherapy and external beam radiation therapy for Grade Group 5 prostate cancer: safety, feasibility, and exploratory analysis. Prostate Cancer Prostatic Dis. (2021) 24:140–9. doi: 10.1038/s41391-020-0254-y
63. Schneider BJ, Naidoo J, Santomasso BD, Lacchetti C, Adkins S, Anadkat M, et al. Management of immune-related adverse events in patients treated with immune checkpoint inhibitor therapy: ASCO guideline update. J Clin Oncol. (2021) 39:4073–126. doi: 10.1200/JCO.21.01440
64. Welsh JW, Heymach JV, Guo C, Menon H, Klein K, Cushman TR, et al. Phase 1/2 trial of pembrolizumab and concurrent chemoradiation therapy for limited-stage SCLC. J Thorac Oncol. (2020) 15:1919–27. doi: 10.1016/j.jtho.2020.08.022
65. Chang JY, Lin SH, Dong W, Liao Z, Gandhi SJ, Gay CM, et al. Stereotactic ablative radiotherapy with or without immunotherapy for early-stage or isolated lung parenchymal recurrent node-negative non-small-cell lung cancer: an open-label, randomised, phase 2 trial. Lancet. (2023) 402:871–81. doi: 10.1016/S0140-6736(23)01384-3
66. Bestvina CM, Pointer KB, Karrison T, Al-Hallaq H, Hoffman PC, Jelinek MJ, et al. A phase 1 trial of concurrent or sequential ipilimumab, nivolumab, and stereotactic body radiotherapy in patients with stage IV NSCLC study. J Thorac Oncol. (2022) 17:130–40. doi: 10.1016/j.jtho.2021.08.019
67. Hallqvist A, Koyi H, de Petris L, Lindberg K, Farooqi S, Helland Å, et al. 63MO Safety analysis of durvalumab following stereotactic body radiotherapy (SBRT) in early-stage non-small cell lung cancer (NSCLC) patients: A first report of a randomized phase II trial (ASTEROID). J Thorac Oncol. (2021) 16:S729–30. doi: 10.1016/S1556-0864(21)01905-5
68. Durm GA, Jabbour SK, Althouse SK, Liu Z, Sadiq AA, Zon RT, et al. A phase 2 trial of consolidation pembrolizumab following concurrent chemoradiation for patients with unresectable stage III non-small cell lung cancer: Hoosier Cancer Research Network LUN 14-179. Cancer. (2020) 126:4353–61. doi: 10.1002/cncr.33083
69. Lin SH, Lin Y, Yao L, Kalhor N, Carter BW, Altan M, et al. Phase II trial of concurrent atezolizumab with chemoradiation for unresectable NSCLC. J Thorac Oncol. (2020) 15:248–57. doi: 10.1016/j.jtho.2019.10.024
70. Tachihara M, Tsujino K, Ishihara T, Hayashi H, Sato Y, Kurata T, et al. Durvalumab plus concurrent radiotherapy for treatment of locally advanced non-small cell lung cancer: the DOLPHIN phase 2 nonrandomized controlled trial. JAMA Oncol. (2023) 9:1505–13. doi: 10.1001/jamaoncol.2023.3309
71. Garassino MC, Mazieres J, Reck M, Chouaid C, Bischoff H, Reinmuth N, et al. Durvalumab after sequential chemoradiotherapy in stage III, unresectable NSCLC: the phase 2 PACIFIC-6 trial. J Thorac Oncol. (2022) 17:1415–27. doi: 10.1016/j.jtho.2022.07.1148
72. Sakaguchi T, Ito K, Furuhashi K, Nakamura Y, Suzuki Y, Nishii Y, et al. Patients with unresectable stage III non-small cell lung cancer eligible to receive consolidation therapy with durvalumab in clinical practice based on PACIFIC study criteria. Respir Investig. (2019) 57:466–71. doi: 10.1016/j.resinv.2019.03.011
73. Faivre-Finn C, Vicente D, Kurata T, Planchard D, Paz-Ares L, Vansteenkiste JF, et al. Four-year survival with durvalumab after chemoradiotherapy in stage III NSCLC-an update from the PACIFIC trial. J Thorac Oncol. (2021) 16:860–7. doi: 10.1016/j.jtho.2020.12.015
74. Kievit H, Muntinghe-Wagenaar MB, Hijmering-Kappelle LBM, Hiddinga BI, Ubbels JF, Wijsman R, et al. Safety and tolerability of stereotactic radiotherapy combined with durvalumab with or without tremelimumab in advanced non-small cell lung cancer, the phase I SICI trial. Lung Cancer. (2023) 178:96–102. doi: 10.1016/j.lungcan.2023.02.004
75. Theelen WSME, Chen D, Verma V, Hobbs BP, Peulen HMU, Aerts JGJV, et al. Pembrolizumab with or without radiotherapy for metastatic non-small-cell lung cancer: a pooled analysis of two randomised trials. Lancet Respir Med. (2021) 9:467–75. doi: 10.1016/S2213-2600(20)30391-X
Keywords: NSCLC, immunotherapy, radiotherapy, combination therapy, clinical perspective
Citation: Xue H, Chen Y and Zhou Y (2024) Radioimmunotherapy: a game-changer for advanced non-small cell lung cancer. Front. Immunol. 15:1522508. doi: 10.3389/fimmu.2024.1522508
Received: 04 November 2024; Accepted: 18 November 2024;
Published: 06 December 2024.
Edited by:
Dechao Feng, University College London, United KingdomCopyright © 2024 Xue, Chen and Zhou. This is an open-access article distributed under the terms of the Creative Commons Attribution License (CC BY). The use, distribution or reproduction in other forums is permitted, provided the original author(s) and the copyright owner(s) are credited and that the original publication in this journal is cited, in accordance with accepted academic practice. No use, distribution or reproduction is permitted which does not comply with these terms.
*Correspondence: Yunshang Chen, Y3lzOTYwOTI4QDE2My5jb20=; Yun Zhou, emhvdXl1bjAyMTNAMTI2LmNvbQ==