- 1Reproductive Immunology Research Center, Shahid Sadoughi University of Medical Sciences, Yazd, Iran
- 2Research and Clinical Center for Infertility, Yazd Reproductive Sciences Institute, Shahid Sadoughi University of Medical Sciences, Yazd, Iran
- 3Department of Immunology, Faculty of Medicine, Shahid Sadoughi University of Medical Sciences and Health Services, Yazd, Iran
Background: To address knowledge gaps, this study aimed to investigate the involvement of inflammasomes in the etiology of azoospermia. This study focused on the gene expression of key inflammasome components, including NLR family pyrin domain containing 3 (NLRP-3), CASPASE-1, Interleukin-1β (IL-1β), Interleukin-18 (IL-18), NLR family CARD domain-containing protein 4/ice protease-activating factor (NLRC-4/IPAF), and Absent in melanoma 2 (AIM-2).
Methods: We analyzed gene expression in blood and testicular tissue from patients with obstructive azoospermia (OA) and non-obstructive azoospermia (NOA). Additionally, we compared IL-1β and IL-18 expression levels in seminal plasma samples using the Enzyme-Linked Immunosorbent Assay (ELISA) method. For comparison, blood samples from normospermic (NS) individuals were also genetically evaluated.
Results: Our results indicated significantly higher gene expression of inflammasome components in NOA patients than those in OA patients either in blood or in testicular tissue. Both azoospermic groups exhibited higher mRNA levels of inflammasome genes comparing with those from blood samples of NS men. Seminal plasma samples showed significantly increased levels of IL-1β and IL-18 in NOA patients compared to men with OA. The ROC curve analysis indicated strong and significant predictive power of IL-18, AIM-2 and NLRC-4/IPAF gene expression profiles between NOA vs. NS patients and NOA vs. OA.
Conclusions: Our findings highlight the role of hidden chronic inflammation in azoospermia, particularly within the NOA group. This study provides a foundation for further detailed research, which could aid in the development of diagnostic panels to differentiate between various azoospermic groups.
1 Introduction
Azoospermia, characterized by the absence of spermatozoa in the seminal fluid, affects 5 to 20 percent of infertile men, compared to approximately 2 percent of the general population of reproductive age (1, 2). It arises when males are unable to produce spermatozoa due to various factors, or when there is an obstruction in the passage of spermatozoa from the testes to the ejaculatory ducts (3). The causes of azoospermia can be classified into three categories: pre-testicular causes (secondary testicular failure), testicular causes (primary testicular failure), and post-testicular causes (obstruction) (4). However, in clinical practice and treatment decision-making, azoospermia is often categorized into two main types: obstructive azoospermia (OA) and non-obstructive azoospermia (NOA) (5). OA can result from various factors, including genetic diseases, vasectomy, congenital absence of the vas deferens, and idiopathic or acquired epididymal obstruction, such as sexually transmitted infections (6). Similarly, NOA can be attributed to a wide range of causes, including genetic/chromosomal abnormalities, iatrogenic/surgical interventions, developmental/structural etiologies, and hypogonadism. Despite advances in understanding the underlying conditions that lead to azoospermia, a significant proportion of cases remain idiopathic (7).
Emerging evidence suggests that chronic inflammation may contribute to idiopathic azoospermia, both OA and NOA. Immunological factors are known to play a significant role in inflammatory processes and contribute to idiopathic azoospermia (8). The inflammasome, a crucial structure involved in inflammation, has been implicated in certain cases of azoospermia, such as varicocele (9, 10). The importance of the inflammasome in male infertility is increasingly recognized, with NLRP-3 and IL-1 being identified as regulators of spermatogenesis. Their effects extend to enhancing various conditions associated with male infertility, highlighting the role of inflammasomes in the immune response within the testes, blood, seminal plasma, etc. (11, 12).
The inflammasome, a component of innate immunity, is triggered by exposure to viruses, bacteria, fungi, and non-organic stimuli. Key components of the inflammasome include NLRP-3, CASPASE-1, IL-1β, IL-18, AIM-2, and NLRC4/IPAF. When NLRP-3, AIM-2, and NLRC-4/IPAF are activated, they trigger the conversion of pro-CASPASE-1, pro-IL-1β, and pro-IL-18 into their active forms, CASPASE-1, IL-1β, and IL-18, respectively (8, 13, 14). NLRP-3 have been implicated as a central player in the immune response of the male reproductive system. When testicular inflammation occurs, cells can release pro-inflammatory cytokines like IL-1β and CCL2 via a mechanism requiring the NLRP-3 inflammasome. Previous studies have reported elevated concentrations of crucial inflammasome components in the semen of men with reproductive diseases, suggesting a potential link between inflammasome activation and disease pathogenesis (15, 16).
Strong inflammasome activation preferentially leads to caspase 1 recruitment and activation, IL-1β and IL-18 activation, and inflammation. Weak inflammasome activation promotes ASC-mediated caspase 8 recruitment and activation of the apoptotic pathway (17). Caspase 8 may play a role in the infertility caused by long-term alcohol consumption (18). Mature IL-1β and IL-18 are produced by macrophages and dendritic cells in response to different types of cellular stress (19, 20). The Caspases have been implicated in the pathogenesis of multiple andrological pathologies, such as impaired spermatogenesis, decreased sperm motility and increased levels of sperm DNA fragmentation, testicular torsion, varicocele, and immunological infertility (21).
Despite the growing interest in inflammasomes, the literature currently lacks compelling evidence regarding their specific role in azoospermia. This research gap motivated the primary goal of this study investigating the involvement of inflammasomes in the etiology of azoospermia. The study design is shown schematically in Figure 1.
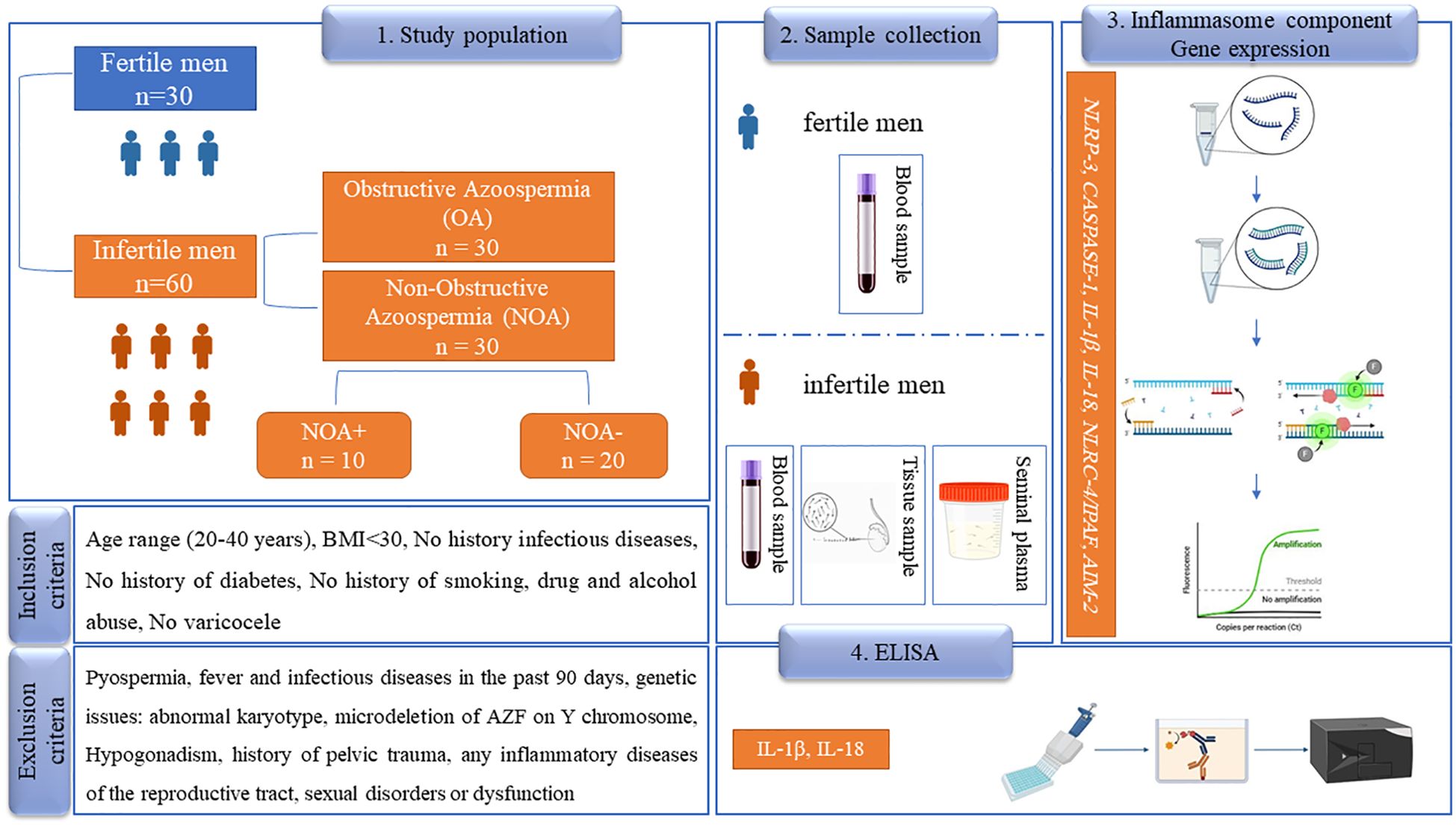
Figure 1. The study design of the paper. In this prospective study, 60 patients with azoospermia were examined, while 30 healthy, fertile men served as a control group (normospermia). OA, obstructive azoospermia; NOA, non-obstructive azoospermia; NOA+, successful sperm retrieval; NOA-, unsuccessful sperm retrieval; BMI, body mass index; AZF, azoospermia factor; NLRP-3, NLR family pyrin domain containing 3; NLRC4/IPAF, NLR family CARD domain-containing protein 4/ ice protease-activating factor, AIM-2, absent in melanoma; 2 IL-1β, interleukin-1β; IL-18, interleukin-18; ELISA, Enzyme-Linked Immunosorbent Assay. The pictures are taken from the Biorender website.
2 Materials and methods
2.1 Study population
Participants were divided into two main groups: patients with OA and infertile men with NOA. Each group consisted of 30 patients. The NOA group was further subdivided into subgroups based on sperm retrieval outcomes: NOA+ (successful sperm retrieval) and NOA- (unsuccessful sperm retrieval). Additionally, 30 healthy fertile men served as a control group, referred to as normospermia (NS), for comparison of blood outcomes. Inclusion criteria for the NS group included normospermic sperm cells and at least one child within the past 2 years. Demographic variables, including age, duration of infertility, and occupation, were collected through patient questionnaires and face-to-face interviews with all participants. For the NOA and OA groups, men were selected based on the following criteria: primary infertility, age between 20-40 years, body mass index (BMI) below 30, no history of smoking or drug abuse, no diabetes, no alcohol consumption, and no varicocele. Patients with pyospermia (presence of more than one million white blood cells per milliliter of semen), fever or infectious diseases within the past 90 days, abnormal karyotype, microdeletion of the AZF region on the Y chromosome, hypogonadism, abnormal levels of sexual hormones (prolactin/FSH/testosterone), any infections and/or inflammatory diseases related to the reproductive tract, and sexual disorders or dysfunction in ejaculation were excluded from the study population.
2.2 Measurement of IL-1β and IL-18 in seminal plasma
Quantitative assessments of IL-1β and IL-18 concentrations in seminal plasma were performed using ELISA Kits (R&D, USA) according to the manufacturer’s instructions. Semen samples were collected from both NOA and OA groups after at least 72 hours of the last ejaculation, and seminal plasma was subsequently isolated. The sensitivities of the IL-1β and IL-18 assays were 1.0 pg/ml and 5.15 pg/ml, respectively.
2.3 Gene expression assessments
2.3.1 Sample collection
Blood samples and testicular biopsy samples were collected for gene expression analysis. Testicular biopsy samples were obtained from NOA patients using micro-TESE (Testicular Sperm Extraction) and TESA (Testicular Sperm Aspiration), while OA patients underwent TESE/PESA (Percutaneous Epididymal Sperm Aspiration). Following testicular biopsy, sperm presence and analysis were conducted in the andrology laboratory for each patient. Due to ethical considerations, only blood samples were collected from fertile participants. Blood samples (from the antecubital vein) were collected from all study groups between 8:00 AM and 11:00 AM.
2.3.2 RNA extraction and cDNA synthesis
Total RNA was extracted from various tissue and blood samples using a total RNA extraction kit from SinaclonBioscience, Iran, following the manufacturer’s instructions. The purity and concentration of each extracted RNA sample were measured using a spectrophotometer at optical densities of 260/280 and 260 nm, respectively. RNA samples with values ranging from 1.8 to 2.0 were normalized prior to cDNA synthesis (22). Subsequently, cDNA synthesis for each RNA sample was performed using the Revert Aid First Strand cDNA Synthesis Kit, following the protocol provided by Parstous Biotechnology, Iran. The synthesized cDNA was stored at -20°C until use.
2.3.3 Quantitative real-time PCR
Real-time quantitative reverse transcriptase-polymerase chain reaction (qRT-PCR) was conducted using specific primers for inflammasome components (NLRP-3, CASPASE-1, IL-1β, IL-18, NLRC-4/IPAF, AIM-2) and GAPDH as a housekeeping gene (Table 1) along with SYBR green master mix (Parstous, Iran). All specific primers used in this study have been selected after deep search in data resources and papers published in global and valid journals. Specificity and accuracy of each primer has been verified using Primer-BLAST tool. Prior to start the main qPCR run, each primer has been checked and set up for optimal Tm and concentrations, and efficiently. All primers with efficiently more than 95% considered for perform qPCR using the 2-△△CT method. During each run, melt curve stage has been considered along with run method due to verify the specificity of own primer. Each qRT-PCR reaction consisted of 1 µL cDNA, 0.5 µL forward primer, 0.5 µL reverse primer, 5 µL master mix, and 3 µL nuclease-free water. The reactions were performed in duplicate using the StepOne system from Applied Biosystems, CA, USA. The qRT-PCR protocol involved an initial denaturation step (15 minutes at 95°C) followed by 40 cycles of amplification at 95°C for 15 seconds, 58°C, 60°C, or 64°C (depending on the specific primer) for 30 seconds, and 72°C for 30 seconds. A final extension step was conducted at 72°C for 5 minutes, followed by a melting curve analysis. Data analysis for relative gene expression was performed using the 2-△△CT method.
2.4 Statistical analysis
The sample size for this study was determined using statistical formulas and the Morgan table (α=0.05, β=0.2). Statistical analyses were conducted using GraphPad Prism software and SPSS 24 for Windows. Data were presented as means ± Standard Error of the Mean (SEM). The paired t-test or Mann–Whitney test was chosen based on data normality, which was assessed using the Kolmogorov-Smirnov test. One-way ANOVA (and nonparametric or mixed models) were used for specific group comparisons. Dunn’s Test was performed as a post-hoc analysis following a one-way ANOVA when significant differences were detected among multiple groups. Additionally, the Pearson correlation test was employed to evaluate the correlation between variables. A P-value less than 0.05 was considered statistically significant.
3 Results
Table 2 shows that there were no significant differences between the NS, NOA, and OA groups in terms of mean age and BMI. Additionally, no significant differences were observed in any of the sexual hormone levels among the study groups. Within the NOA group, 20 patients (66.6%) had unsuccessful sperm retrieval, while 10 patients (33.3%) had successful sperm retrieval.
3.1 Gene expression profile in blood samples
Across all studied genes, the highest expression levels were observed in the NOA group compared to other groups. Conversely, the lowest mRNA levels for all genes were found in the NS group. Our data revealed that CASPASE-1, IL-18, AIM-2, and IPAF/NLRC-4 genes were significantly overexpressed in the NOA group, as well as the NOA+ subgroup, compared to the NS group. IL-1β, IL-18, AIM-2, and NLRC-4/IPAF mRNA levels were significantly higher in the NOA group compared to the OA group. No significant differences were observed between the NS group and the OA group for any of the target genes. Additionally, no significant differences in NLRP-3 gene expression were found between any of the groups. While the NOA+ subgroup exhibited higher expression levels for all genes, no significant changes were detected between the two NOA subgroups. There was a significant upregulation in IL-1β, IL-18, AIM-2, and NLRC4/IPAF genes in the NOA+ subgroup compared to OA cases (Figure 2).
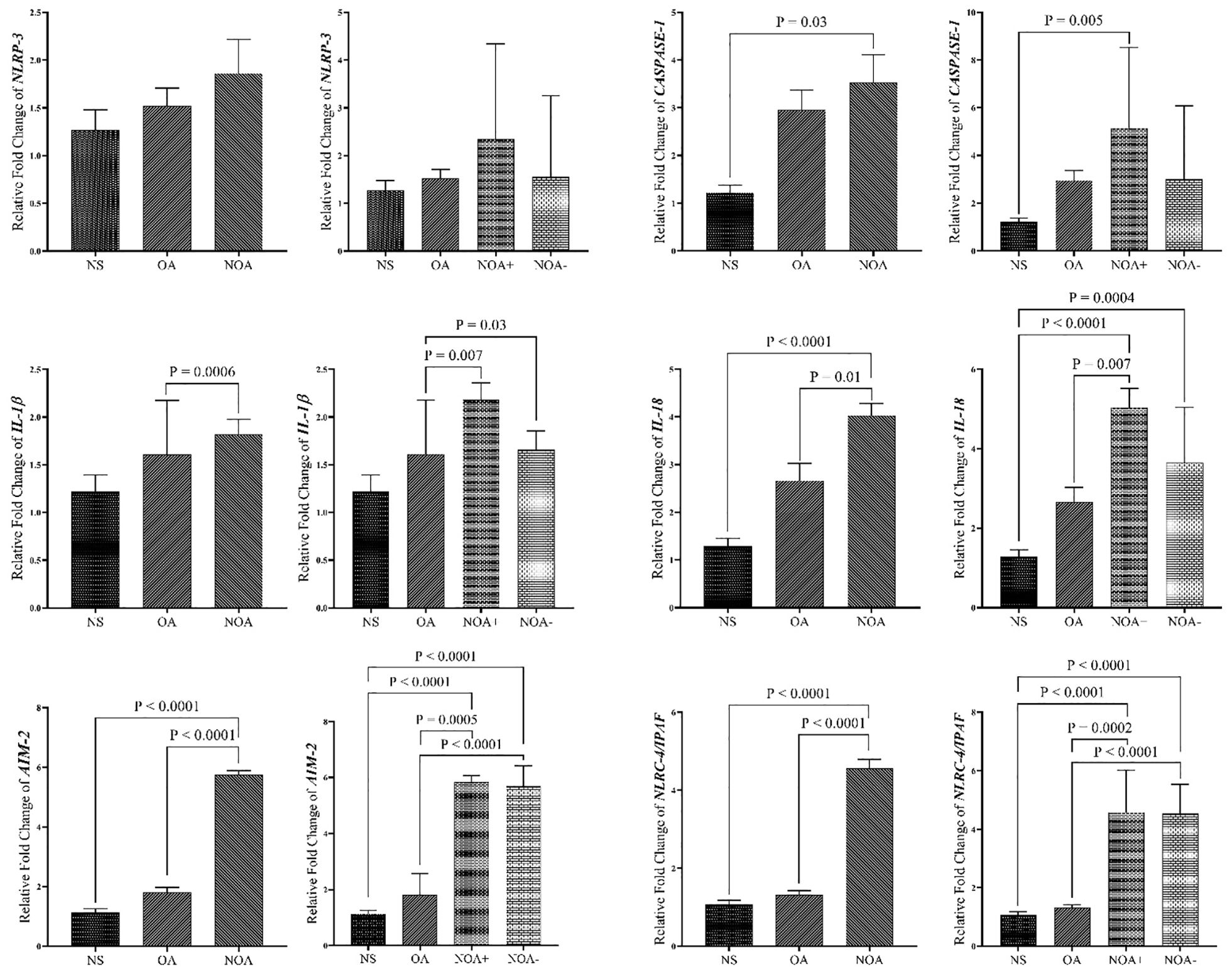
Figure 2. Comparison of gene expression in blood samples of study groups. The data are presented as mean ± SEM, performed by One-way ANOVA test. P < 0.05 represents significant values. NS, normospermia; OA, obstructive azoospermia; NOA+, positive sperm retrieval non-obstructive azoospermia; NOA-, negative sperm retrieval nonobstructive azoospermia; NLRP-3, NLR family pyrin domain containing 3; NLRC4/IPAF, NLR family CARD domain-containing protein 4/ ice protease-activating factor; AIM-2, absent in melanoma 2; IL-1β, interleukin-1β; IL-18, interleukin-18.
ROC curve analysis was performed for all target genes. The ROC curve analysis indicated strong and significant predictive power of IL-18, AIM-2, and NLRC-4/IPAF gene expressions in differentiating between NOA vs. NS patients and NOA vs. OA infertile men (Figure 3). IL-18 gene expression exhibited a sensitivity of 93.10%, specificity of 95%, and area under the curve (AUC) of 62.07%, with cut-off values (COV) of 2.498 and 2.678 for NOA vs. NS and NOA vs. OA, respectively. AIM-2 mRNA levels demonstrated a sensitivity and specificity of 100%, with a COV of 3.201 and 3.758 for NOA vs. NS and NOA vs. OA, respectively. When comparing NOA vs. NS and NOA vs. OA, NLRC-4/IPAF mRNA levels displayed a sensitivity of 100% and specificity of 95% and 95.83%, respectively, with a COV of 1.888 and 2.324.
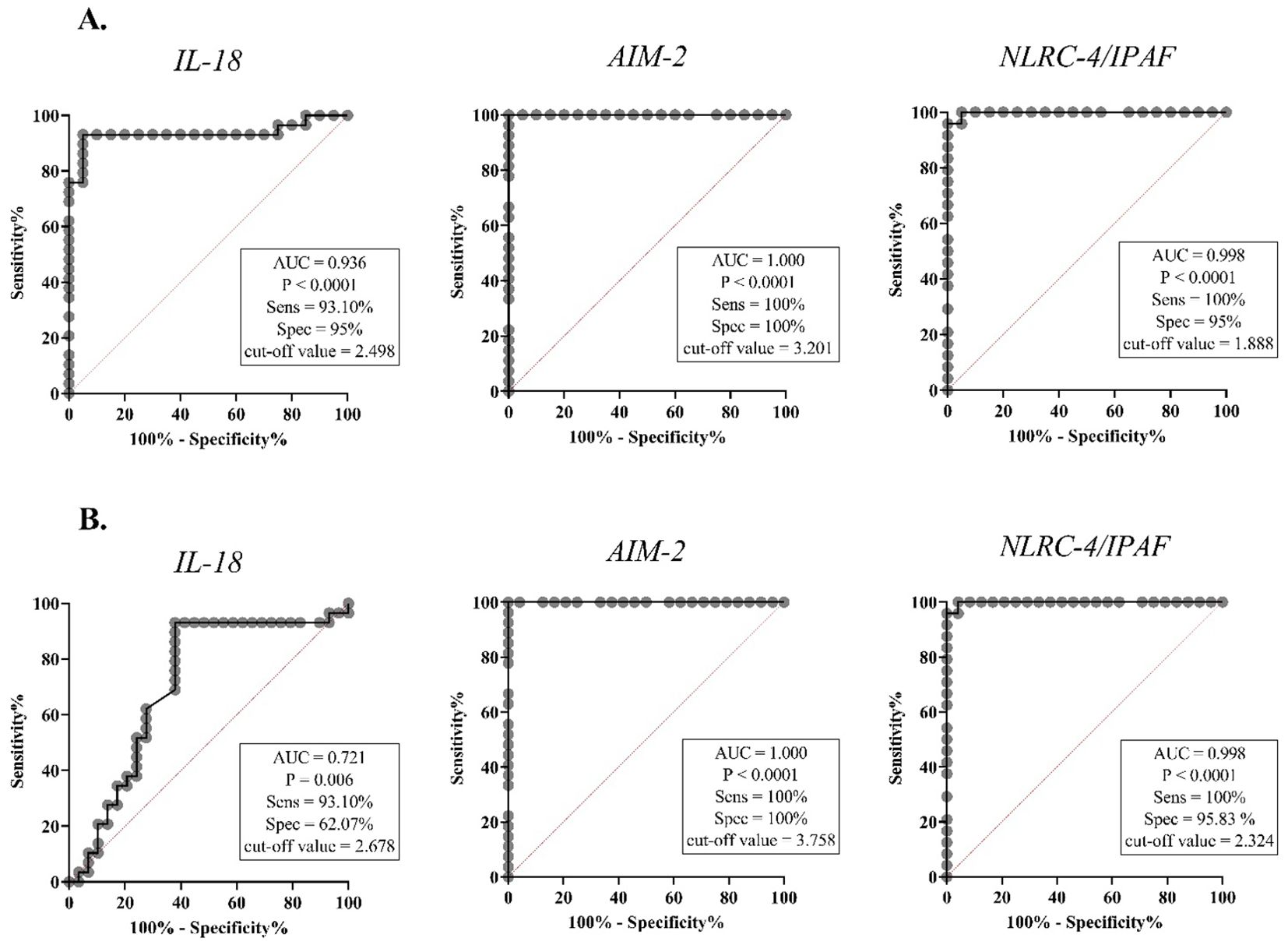
Figure 3. The receiver operating characteristic (ROC) analysis of IL-18, AIM-2, and NLRC-4/IPAF mRNA levels in blood samples. The right-hand panel of each graph displays the area under the ROC curve (AUC), the optimal cut-off value determined by the Youden index, and the corresponding sensitivity and specificity values for each prediction. IL-18, interleukin-18; AIM-2, absent in melanoma 2; NLRC4/IPAF, NLR family CARD domain-containing protein 4/ ice protease-activating factor. (A) ROC curve comparing NOA and NS in blood samples, (B) ROC curve comparing NOA and OA in blood samples. P < 0.05 is regarded as a significant value. AUC, area under curve; Sens, sensitivity; Spec, specificity.
3.2 Gene expression profile in tissue samples
Figure 4 demonstrates that overexpression of all genes was more pronounced in the NOA group compared to the OA group. When comparing the two NOA subgroups, overexpression was observed to be higher in the NOA+ group compared to the NOA- group. NLRP-3, IL-18, and AIM-2 gene upregulation were significantly higher in the NOA group compared to the OA group. AIM-2 and IL-18 expression levels were significantly higher in both the NOA+ and NOA- groups compared to the OA group. No significant differences were observed between the NOA+ and NOA- groups. In both study groups, ROC curve analysis of IL-18 and AIM-2 demonstrated a strong and significant predictive capacity in all azoospermic men (sensitivity of 85.19% vs. 83.87%, specificity of 100% vs. 83.87%, COV of 2.329 vs. 1.772, respectively). However, no strong ROC values were observed for NLRC-4/IPAF against blood data (Supplementary Figure 1).
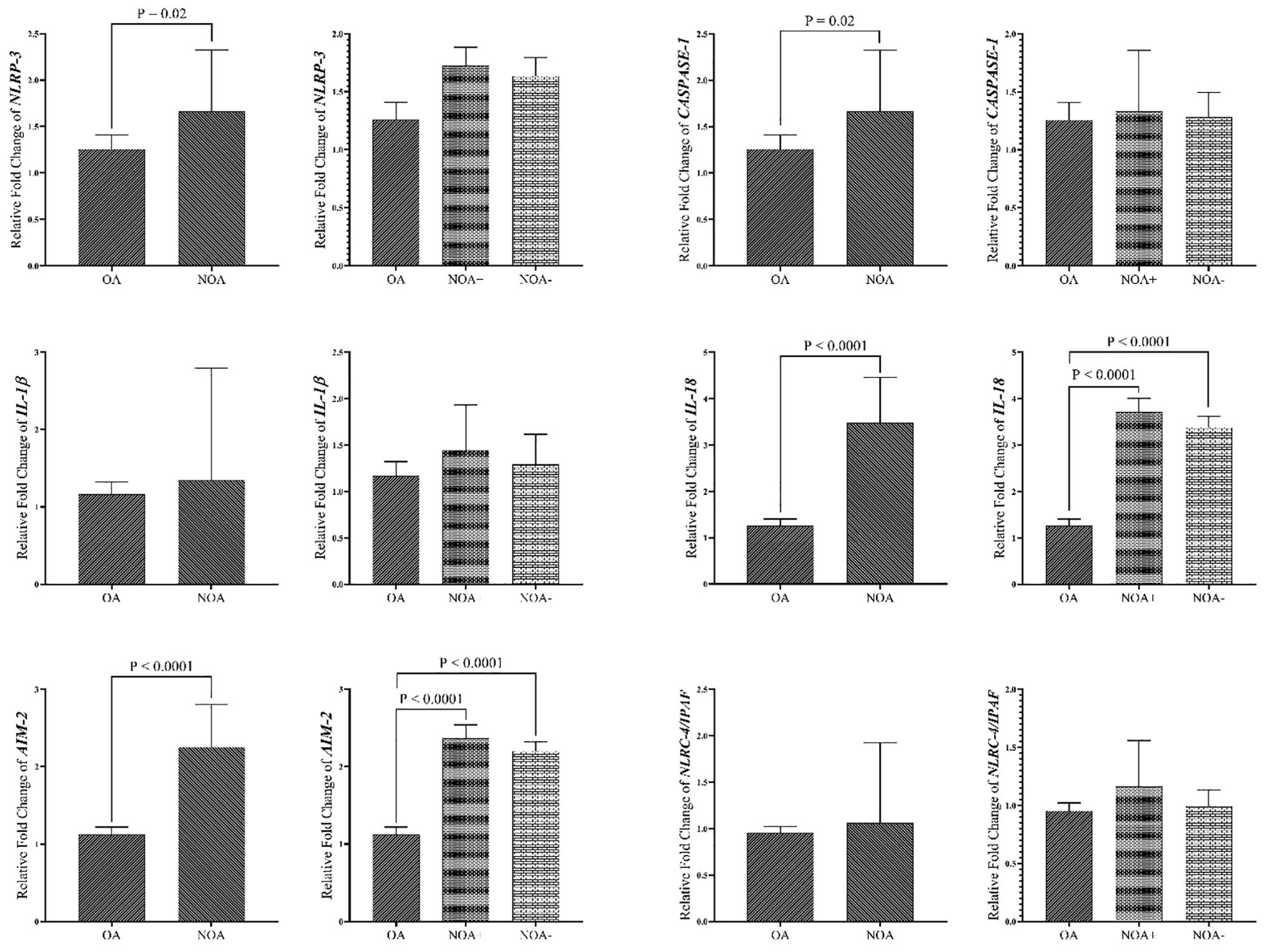
Figure 4. Comparison of Gene expression in tissue samples of study groups. The data are presented as mean ± SEM , performed by paired t-test or Mann–Whitney test was chosen based on data normality and One-way ANOVA test. P < 0.05 represents significant values. OA, obstructive azoospermia; NOA, non-obstructive azoospermia; NOA, non-obstructive azoospermia; NOA+, positive sperm retrieval nonobstructive azoospermia; NOA-, negative sperm retrieval non-obstructive azoospermia; NLRP-3, NLR family pyrin domain containing 3; NLRC4/IPAF, NLR family CARD domain-containing protein 4/ ice protease-activating factor; AIM-2, absent in melanoma 2; IL-1β, interleukin-1β; IL-18, interleukin-18.
3.3 Concentration of IL-1β and IL-18 in seminal plasma samples
Protein levels of IL-1β and IL-18 were significantly higher in the NOA group compared to the OA group (p=0.0036) (Table 3). Table 4 reveals a positive and significant correlation between these two cytokines in both groups of azoospermia.
Supplementary Figure 2 indicates that no significant correlation was observed between IL-1β and IL-18 in the gene expression of total samples and the levels of seminal plasma cytokines in both groups.
3.4 Correlation
Figure 5 shows a direct and significant correlation between blood and tissue samples for NLRP-3, CASPASE-1, and AIM-2 in the NOA group, but no correlation was observed in the OA group. Additionally, no significant correlations were found for the other three genes in either group. Referring to Figures 3, 4 in the supplementary data for more information, no specific or significant correlations were observed among all genes in each group.
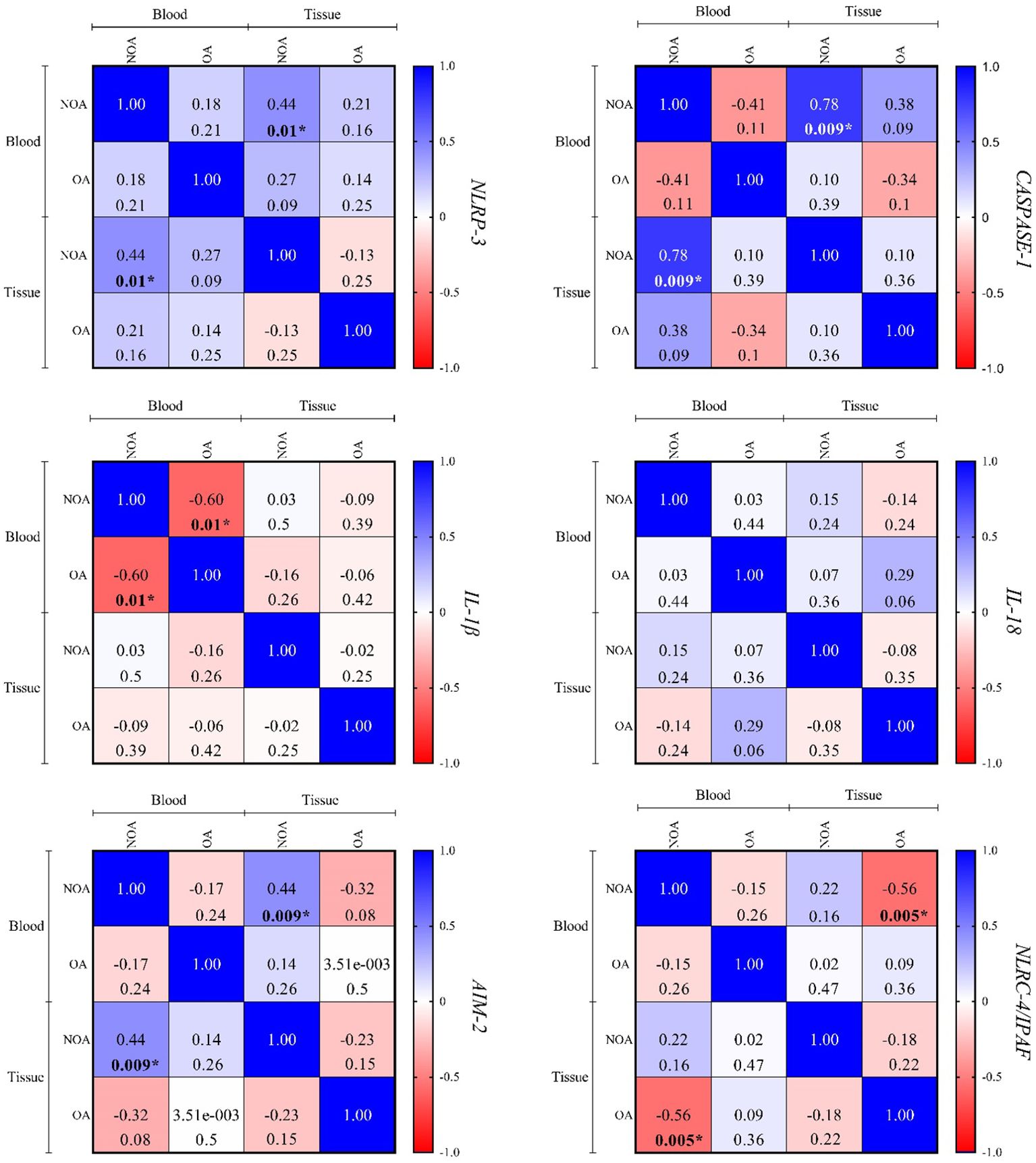
Figure 5. The correlation between the blood and tissue samples was performed using Spearman's rank correlation. In each cell, the top value is correlation coefficient (r), and the bottom is the P-value. P < 0.05 is regarded as a significant value. Where P-values are significant, numbers are written in bold and marked by an asterisk. OA, obstructive azoospermia; NOA, non-obstructive azoospermia; NLRP-3, NLR family pyrin domain containing 3; IL-1β, interleukin-1β; IL-18, interleukin-18; AIM-2, absent in melanoma 2; NLRC4/IPAF, NLR family CARD domain-containing protein 4/ ice protease-activating factor.
4 Discussion
Inflammasomes are cytoplasmic multiprotein complexes composed of inflammatory caspases, a sensor protein, and, depending on the specific context, an adapter protein that links the two. These complexes can be activated by a diverse range of endogenous and exogenous stimuli, leading to the enzymatic activation of canonical CASPASE-1. This activation results in the secretion of IL-1β and IL-18, as well as apoptotic and pyroptotic cell death (23).
A deeper understanding of the molecular mechanisms underlying NLRP-3 inflammasome activation will provide opportunities to develop strategies for preventing and treating diseases, including male infertility associated with the NLRP-3 inflammasome (24). The first evidence of inflammasome complex involvement in an infertility-related disease was reported in a prospective study in 2013 by Zheng et al. This study found that inflammasome complex proteins were activated in men with spinal cord injury, and their results indicated increased seminal plasma concentrations of IL-1β and IL-18 compared to a control group (25).
Our data demonstrated that the expression of all inflammasome-associated genes was higher in the group with NOA followed by the group with OA, compared to NS. Furthermore, within the NOA subtype, NOA+ exhibited higher expression levels than NOA-. A cross-sectional cohort study conducted by Ordek et al. in 2023 yielded similar results to our findings. NLRP-3 and IL-1β levels were measured in serum and semen. Their study found that serum and seminal plasma NLRP-3 and IL-1β levels in patients with varicocele or azoospermia were significantly higher than in those without either condition (26). In a 2022 study by Kati et al., examining the function of NLRP-3 and IL-1β markers in patients with TESE+ and TESE-, they observed increased expression of these two genes in the TESE+ group compared to TESE-, which was inconsistent with our findings (27). Malcher et al. (2013) reported significantly downregulated IL1-RA gene expression in the infertile group compared to controls, while expression levels of IL-1a and IL-1b did not show significant differences between groups. They also observed significantly higher expression levels of the CASPASE-1 gene in the Sertoli-cell-only syndrome group (6). In a 2013 study by Shen et al., increased overexpression of IL-1β and IL-18 was found to be associated with an increased number of abnormal sperm cells, reduced sperm motility, and ultimately, infertility (28). Ata-abadi et al. examined varicocele by analyzing semen samples six months before and six months after varicocelectomy. The levels of IL-1β, IL-18, and CASPASE-1 cytokines were examined, revealing that following treatment, sperm morphology improved and inflammation in the seminal plasma decreased (29). Inflammasome activation and subsequent overexpression of IL-1β and IL-18 negatively impact spermatogenesis by causing DNA damage, mitochondrial dysfunction, and reduced sperm motility. These effects are likely mediated by the disruption of the seminiferous tubule epithelium and increased germ cell apoptosis (15, 16, 30).
Differences in gene expression affect NOA by described mechanisms. The NLRP-3 inflammasome is a multiprotein complex composed of the sensor NLRP-3, the adaptor ASC (apoptosis-associated speck-like protein containing a CARD), and the effector CASPASE-1. This complex facilitates the processing of pro-inflammatory cytokines IL-1β and IL-18 and induces pyroptosis through the cleavage of gasdermin D (15).
Overexpression of the canonical inflammasome gene leads to an increase in ASC. ASC affects multiple sperm structures, including the acrosome, equatorial segment, and midpiece, potentially altering their functions (25).
Elevated IL-18 levels in semen have been positively correlated with a higher probability of pregnancy failure following IVF and ICSI, suggesting an association with impaired sperm function (31, 32). Current literature provides limited information regarding the role of AIM-2 and NLRC-4/IPAF genes in inflammatory conditions. Onodi et al., conducted a groundbreaking study in 2021, demonstrating how AIM-2 and NLRC-4/IPAF inflammasome activation contributes to persistent inflammation in heart failure (33). Another recent study suggested that overexpression of the NLRC-4/IPAF inflammasome could serve as a potential therapeutic target and diagnostic marker for gliomas, potentially associated with poor prognosis (34). Our study’s ROC curve analysis identified three genes, AIM-2, NLRC-4/IPAF, and IL-18, with high sensitivity and specificity in the blood of NOA patients compared to the NS group. In particular view, ROC curve analysis showed that both AIM-2 and IL-18 have significant predictive ability. AIM-2 showed 100% sensitivity and specificity in distinguishing between NOA and NS groups, as well as between NOA and obstructive azoospermia. Similarly, IL-18 showed a sensitivity of 93.10% and a specificity of 95%, confirming its utility as a reliable biomarker. The insights gained from the study of inflammasome biomarkers, particularly AIM-2 and IL-18, can significantly inform future research and clinical practices. Firstly, the high sensitivity and specificity of these biomarkers suggest their potential inclusion in diagnostic panels for azoospermia, allowing clinicians to more accurately differentiate between obstructive and non-obstructive forms. Moreover, future research could explore the integration of additional biomarkers identified in recent studies, such as those related to macrophage infiltration in NOA or seminal fluid profiles from patients with oligozoospermia and azoospermia (35, 36). These findings suggest that diverse factors contribute to these reactions, potentially attributed to differences in gene expression, genetic variations, epigenetic modifications, signaling pathways, and stimulatory and inhibitory factors of molecules (37–41). Furthermore, ethical constraints and legal regulations prevented us from performing testicular biopsies on fertile men. Consequently, in similar comparative studies involving two groups of azoospermia, obstructive azoospermia patients have been utilized as the control group in comparison to the NOA group (42, 43).
To our knowledge, this study is the first leading study with specific focus on exploring the role of inflammasome in infertile men with different history of azoospermia as the most severe complications relate to male infertility. Despite a little literature on evaluating the potative function of the some involved inflammasome components (not all members) in patients with varicocele (8, 11, 26, 44, 45), our study provides a novel and detailed exploration of inflammasome members and their mechanisms in azoospermia. Additionally, our results highlight the significant role of chronic inflammation in azoospermia, particularly within the NOA group. Although it is too early to provide a definitive opinion, with only a single-center study and a limited sample size, our study has clearly shown the role of the immunological system and the effect of inflammation in the pathogenesis of azoospermia with unknown causes. Additionally, the future perspective of such a this research could lead to introduce and design an accurate and highly specific hybrid-diagnostic panel aiding experts in the treatment process of azoospermic men to estimate of the success rate of sperm recovery surgeries by using molecular and non-invasive tools. Taken together, our findings in line with newly reports (46) emphasizes lack of current data and need for future studies to refine diagnostic approaches.
5 Conclusion
Our findings demonstrated a significant increase in both gene and protein expression of inflammasome components in azoospermic patients, particularly in men with NOA. The alignment of gene expression results from tissue and blood samples, coupled with ROC curve analysis, suggests that investigating AIM-2, NLRC-4/IPAF, and IL-18 could serve as three potential biomarkers for predicting and differentiating the causes of azoospermia.
5.1 Limitations
Due to ethical concerns and the specific conditions of individuals with normospermia, invasive sampling from their testes could not be possible. Therefore, we could compared only samples from blood of azoospermic patient with fertile ones not at the levels of testicular tissue. One of the other limitations was the relatively small number of samples. Although the multiple sample collection in clinical studies similar to ours meet all criteria is usually difficult and time-consuming, we tried to touch the standard sample size similar to other studies (47, 48). It seems that future studies should conduct with larger populations and gather all data reported from multicenter with meta-analysis.
Data availability statement
The original contributions presented in the study are included in the article/Supplementary Material. Further inquiries can be directed to the corresponding author.
Ethics statement
This prospective study was conducted with the approval of the Reproductive Immunology Research Center of Shahid Sadoughi University of Medical Sciences and received ethical clearance from the ethics committee (IR.SSU.SPH.REC.1402.006).
Author contributions
HH: Conceptualization, Supervision, Writing – review & editing. SM: Investigation, Project administration, Writing – original draft. FF: Methodology, Project administration, Writing – review & editing. FZ: Conceptualization, Methodology, Project administration, Writing – review & editing. MI: Methodology, Project administration, Writing – review & editing. SV: Data curation, Methodology, Supervision, Validation, Writing – review & editing. HA: Conceptualization, Formal analysis, Writing – review & editing. AZ: Conceptualization, Data curation, Project administration, Writing – review & editing.
Funding
The author(s) declare that no financial support was received for the research, authorship, and/or publication of this article.
Acknowledgments
This study was conducted at Yazd Shahid Sadoughi University of Medical Sciences. The authors express sincere gratitude to the Reproductive Immunology Research Center for providing research facilities and to the Research and Clinical Center for Infertility, Yazd Reproductive Sciences Institute, for their assistance with patient sampling and interviews.
Conflict of interest
The authors declare that the research was conducted in the absence of any commercial or financial relationships that could be construed as a potential conflict of interest.
Generative AI statement
The authors declare that no Generative AI was used in the creation of this manuscript.
Publisher’s note
All claims expressed in this article are solely those of the authors and do not necessarily represent those of their affiliated organizations, or those of the publisher, the editors and the reviewers. Any product that may be evaluated in this article, or claim that may be made by its manufacturer, is not guaranteed or endorsed by the publisher.
Supplementary material
The Supplementary Material for this article can be found online at: https://www.frontiersin.org/articles/10.3389/fimmu.2024.1507885/full#supplementary-material
References
1. Wosnitzer M, Goldstein M, Hardy MP. Review of azoospermia. Spermatogenesis. (2014) 4:e28218. doi: 10.4161/spmg.28218
2. Wyrwoll MJ, Köckerling N, Vockel M, Dicke A-K, Rotte N, Pohl E, et al. Genetic architecture of azoospermia—time to advance the standard of care. Eur Urol. (2023) 83:452–62. doi: 10.1016/j.eururo.2022.05.011
3. Zhou L, Lv M-Q, Ge P, Yang Y-Q, He D-L, Wang H-X, et al. The expression of Beclin-1 in testicular tissues of non-obstructive azoospermia patients and its predictive value in sperm retrieval rate. Trans Androl Urol. (2021) 10:3267. doi: 10.21037/tau-21-320
4. Cocuzza M, Alvarenga C, Pagani R. The epidemiology and etiology of azoospermia. Clinics. (2013) 68:15–26. doi: 10.6061/clinics/2013(Sup01)03
5. Takeshima T, Karibe J, Saito T, Kuroda S, Komeya M, Uemura H, et al. Clinical management of nonobstructive azoospermia: An update. Int J Urol. (2024) 31:17–24. doi: 10.1111/iju.15301
6. Malcher A, Rozwadowska N, Stokowy T, Jedrzejczak P, Zietkowiak W, Kurpisz M. The gene expression analysis of paracrine/autocrine factors in patients with spermatogenetic failure compared with normal spermatogenesis. Am J Reprod Immunol. (2013) 70:522–8. doi: 10.1111/aji.2013.70.issue-6
7. Kang C, Punjani N, Schlegel PN. Reproductive chances of men with azoospermia due to spermatogenic dysfunction. J Clin Med. (2021) 10:1400. doi: 10.3390/jcm10071400
8. Tavalaee M, Rahmani M, Drevet JR, Nasr-Esfahani MH. The NLRP3 inflammasome: molecular activation and regulation in spermatogenesis and male infertility; a systematic review. Basic Clin Androl. (2022) 32:8. doi: 10.1186/s12610-022-00157-9
9. Fang Y, Su Y, Xu J, Hu Z, Zhao K, Liu C, et al. Varicocele-mediated male infertility: from the perspective of testicular immunity and inflammation. Front Immunol. (2021) 12. doi: 10.3389/fimmu.2021.729539
10. Xu F, Chen Y, Chen H, Xu ZP, Han YF, Yu W, et al. Impact of varicocele and varicocelectomy on the apoptosis of spermatogenic cells and the levels of nitrogen monoxidum and interleukin 1 in the rat testis. Zhonghua Nan Ke Xue. (2016) 22:200–4.
11. Minas A, Costa LVS, Miyazaki MA, Antoniassi MP. Insight toward inflammasome complex contribution to male infertility. Am J Reprod Immunol. (2023) 90:e13734. doi: 10.1111/aji.13734
12. Walenta L, Schmid N, Schwarzer JU, Köhn FM, Urbanski HF, Behr R, et al. NLRP3 in somatic non-immune cells of rodent and primate testes. Reproduction. (2018) 156:231–8. doi: 10.1530/REP-18-0111
13. Hornung V, Ablasser A, Charrel-Dennis M, Bauernfeind F, Horvath G, Caffrey DR, et al. AIM2 recognizes cytosolic dsDNA and forms a caspase-1-activating inflammasome with ASC. Nature. (2009) 458:514–8. doi: 10.1038/nature07725
14. Liu L, Chan C. IPAF inflammasome is involved in interleukin-1β production from astrocytes, induced by palmitate; implications for Alzheimer’s disease. Neurobiol Aging. (2014) 35:309–21. doi: 10.1016/j.neurobiolaging.2013.08.016
15. Chen Y, Miao C, Zhao Y, Yang L, Wang R, Shen D, et al. Inflammasomes in human reproductive diseases. Mol Hum Reprod. (2023) 29:gaad035. doi: 10.1093/molehr/gaad035
16. Zhang X, Dong X, Kang Y, Liu X, Zhang C, Zhang J, et al. Research progress on NLRP3 inflammasomes in male reproductive immunity. Explor Immunol. (2024) 4:490-501. doi: 10.37349/ei
17. Grabinger T, Peterburs P, Brunner T. dsDNA ASCs for caspase 8-mediated apoptosis. Cell Death Differ. (2013) 20:1128–30. doi: 10.1038/cdd.2013.80
18. Said TM, Paasch U, Glander HJ, Agarwal A. Role of caspases in male infertility. Hum Reprod Update. (2004) 10:39–51. doi: 10.1093/humupd/dmh003
19. Chan AH, Schroder K. Inflammasome signaling and regulation of interleukin-1 family cytokines. J Exp Med. (2019) 217:e20190314. doi: 10.1084/jem.20190314
20. Qian L, Zhou Y, Du C, Wen J, Teng S, Teng Z. IL-18 levels in the semen of male infertility: semen analysis. Int J Biol Macromol. (2014) 64:190–2. doi: 10.1016/j.ijbiomac.2013.12.005
21. Asadi A, Ghahremani R, Abdolmaleki A, Rajaei F. Role of sperm apoptosis and oxidative stress in male infertility: A narrative review. Int J Reprod Biomed. (2021) 19:493. doi: 10.18502/ijrm.v19i6.9371
22. Afsari M, Fesahat F, Talebi AR, Agarwal A, Henkel R, Zare F, et al. ANXA2, SP17, SERPINA5, PRDX2 genes, and sperm DNA fragmentation differentially represented in male partners of infertile couples with normal and abnormal sperm parameters. Andrologia. (2022) 54:e14556. doi: 10.1111/and.14556
23. Zheng D, Liwinski T, Elinav E. Inflammasome activation and regulation: toward a better understanding of complex mechanisms. Cell Discov. (2020) 6:36. doi: 10.1038/s41421-020-0167-x
24. Paik S, Kim JK, Silwal P, Sasakawa C, Jo E-K. An update on the regulatory mechanisms of NLRP3 inflammasome activation. Cell Mol Immunol. (2021) 18:1141–60. doi: 10.1038/s41423-021-00670-3
25. Zhang X, Ibrahim E, de Rivero Vaccari JP, Lotocki G, Aballa TC, Dietrich WD, et al. Involvement of the inflammasome in abnormal semen quality of men with spinal cord injury. Fertil Steril. (2013) 99:118–24. e2. doi: 10.1016/j.fertnstert.2012.09.004
26. Ördek E, Kati B, Koyuncu I, Demir M, Yağmur I, Pelit ES, et al. What is the impact of inflammasome mechanisms on male infertility? Turkish J Med Sci. (2023) 53:685–91. doi: 10.55730/1300-0144.5631
27. Kati B, Gonel A, Ordek E, Pelit ES, Ciftci H. Do inflammatory markers such as NLRP3 and IL1-β have an effect on the prediction of testicular sperm extraction success in infertile men? Andrologia. (2022) 54:e14572. doi: 10.1111/and.v54.11
28. Shen H, Xu W, Zhang J, Chen M, Martin FL, Xia Y, et al. Urinary metabolic biomarkers link oxidative stress indicators associated with general arsenic exposure to male infertility in a Han Chinese population. Environ Sci Technol. (2013) 47:8843–51. doi: 10.1021/es402025n
29. Ata-Abadi NS, Mowla SJ, Aboutalebi F, Dormiani K, Kiani-Esfahani A, Tavalaee M, et al. Hypoxia-related long noncoding RNAs are associated with varicocele-related male infertility. PloS One. (2020) 15:e0232357. doi: 10.1371/journal.pone.0232357
30. Ramezanikhah H, Farrokhi A, Nejatbakhsh R, Shokri S, Zendedel A, Abdanipour A. Involvement of Asc and Nlrp3 inflammasomes in the testes following spinal cord injury. Endocrine Regulat. (2020) 54:96–108. doi: 10.2478/enr-2020-0012
31. Nikolaeva M, Arefieva A, Babayan A, Chagovets V, Kitsilovskaya N, Starodubtseva N, et al. Immunoendocrine markers of stress in seminal plasma at IVF/ICSI failure: a preliminary study. Reprod Sci. (2021) 28:144–58. doi: 10.1007/s43032-020-00253-z
32. Nikolaeva MA, Babayan AA, Stepanova EO, Smolnikova VY, Kalinina EA, Fernández N, et al. The relationship of seminal transforming growth factor-β1 and interleukin-18 with reproductive success in women exposed to seminal plasma during IVF/ICSI treatment. J Reprod Immunol. (2016) 117:45–51. doi: 10.1016/j.jri.2016.03.006
33. Onódi Z, Ruppert M, Kucsera D, Sayour AA, Tóth VE, Koncsos G, et al. AIM2-driven inflammasome activation in heart failure. Cardiovasc Res. (2021) 117:2639–51. doi: 10.1093/cvr/cvab202
34. Lim J, Kim MJ, Park Y, Ahn JW, Hwang SJ, Moon J-S, et al. Upregulation of the NLRC4 inflammasome contributes to poor prognosis in glioma patients. Sci Rep. (2019) 9:7895. doi: 10.1038/s41598-019-44261-9
35. Luo X, Zheng H, Nai Z, Li M, Li Y, Lin N, et al. Identification of biomarkers associated with macrophage infiltration in non-obstructive azoospermia using single-cell transcriptomic and microarray data. Ann Trans Med. (2023) 11:55. doi: 10.21037/atm-22-5601
36. Nowicka-Bauer K, Kamieniczna M, Olszewska M, Kurpisz MK. Proteomic approach towards identification of seminal fluid biomarkers from individuals with severe oligozoospermia, cryptozoospermia and non-obstructive azoospermia: a pilot study. Trans Androl Urol. (2023) 12:1497. doi: 10.21037/tau-23-130
37. Cioppi F, Rosta V, Krausz C. Genetics of azoospermia. Int J Mol Sci. (2021) 22:3264. doi: 10.3390/ijms22063264
38. Kelley N, Jeltema D, Duan Y, He Y. The NLRP3 inflammasome: an overview of mechanisms of activation and regulation. Int J Mol Sci. (2019) 20:3328. doi: 10.3390/ijms20133328
39. Ramachandran R, Manan A, Kim J, Choi S. NLRP3 inflammasome: a key player in the pathogenesis of life-style disorders. Exp Mol Med. (2024) 56:1488-1500. doi: 10.1038/s12276-024-01261-8
40. kodi T, Sankhe R, Gopinathan A, Nandakumar K, Kishore A. New insights on NLRP3 inflammasome: mechanisms of activation, inhibition, and epigenetic regulation. J Neuroimmune Pharmacol. (2024) 19:7. doi: 10.1007/s11481-024-10101-5
41. Sharma M, de Alba E. Structure, activation and regulation of NLRP3 and AIM2 inflammasomes. Int J Mol Sci. (2021) 22:872. doi: 10.3390/ijms22020872
42. Romano M, Cirillo F, Ravaioli N, Morenghi E, Negri L, Ozgur B, et al. Reproductive and obstetric outcomes in TESE–ICSI cycles: A comparison between obstructive and non-obstructive azoospermia. Andrology. (2023) 18. doi: 10.1111/andr.13568
43. Talreja D, Gupta C, Pai H, Palshetkar N, Shah R. Comparative analysis of surgically retrieved sperms in cases of obstructive and nonobstructive azoospermia treated for infertility. J Hum Reprod Sci. (2020) 13:201–8. doi: 10.4103/jhrs.JHRS_41_19
44. Chen J, Chen J, Fang Y, Shen Q, Zhao K, Liu C, et al. Microbiology and immune mechanisms associated with male infertility. Front Immunol. (2023) 14:1139450. doi: 10.3389/fimmu.2023.1139450
45. Wang LH, Zheng L, Jiang H, Jiang T. Research advances in inflammation and oxidative stress in varicocele-induced male infertility: a narrative review. Asian J Androl. (2024) 11. doi: 10.4103/aja202488
46. Kaltsas A, Markou E, Zachariou A, Dimitriadis F, Symeonidis EN, Zikopoulos A, et al. Evaluating the predictive value of diagnostic testicular biopsy for sperm retrieval outcomes in men with non-obstructive azoospermia. J Pers Med. (2023) 13:1362. doi: 10.3390/jpm13091362
47. Zhang F, Li L, Wang L, Yang L, Liang Z, Li J, et al. Clinical characteristics and treatment of azoospermia and severe oligospermia patients with Y-chromosome microdeletions. Mol Reprod Dev. (2013) 80:908–15. doi: 10.1002/mrd.v80.11
Keywords: inflammasome, azoospermia, gene expression, interleukin, seminal plasma
Citation: Mirghanizadeh Bafghi SA, Fesahat F, Zare F, Imani M, Vahidi S, Ansariniya H, ZareHoroki A and Hadinedoushan H (2024) The role of inflammasome dysregulation in obstructive and non-obstructive azoospermia: a comparative molecular analysis of blood, tissue, and seminal plasma. Front. Immunol. 15:1507885. doi: 10.3389/fimmu.2024.1507885
Received: 08 October 2024; Accepted: 13 November 2024;
Published: 06 December 2024.
Edited by:
Vinay Kumar, The Pennsylvania State University, United StatesReviewed by:
Hina Sultana, University of North Carolina System, United StatesSimna Saraswathi Prasannakumari, University of North Carolina at Chapel Hill, United States
Copyright © 2024 Mirghanizadeh Bafghi, Fesahat, Zare, Imani, Vahidi, Ansariniya, ZareHoroki and Hadinedoushan. This is an open-access article distributed under the terms of the Creative Commons Attribution License (CC BY). The use, distribution or reproduction in other forums is permitted, provided the original author(s) and the copyright owner(s) are credited and that the original publication in this journal is cited, in accordance with accepted academic practice. No use, distribution or reproduction is permitted which does not comply with these terms.
*Correspondence: Hossein Hadinedoushan, aGhhZGluMjAwMEBnbWFpbC5jb20=
†ORCID: Hossein Hadinedoushan, orcid.org/0000-0002-7102-3045