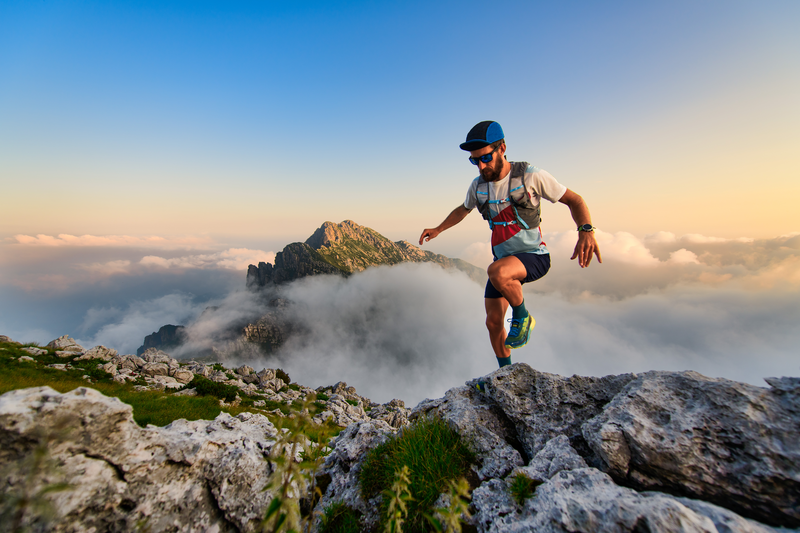
95% of researchers rate our articles as excellent or good
Learn more about the work of our research integrity team to safeguard the quality of each article we publish.
Find out more
ORIGINAL RESEARCH article
Front. Immunol. , 28 November 2024
Sec. Vaccines and Molecular Therapeutics
Volume 15 - 2024 | https://doi.org/10.3389/fimmu.2024.1506638
Introduction: We previously identified Bordetella pertussis-derived outer membrane vesicles (OMVs) as a promising immunogen for improving pertussis vaccines. In this study, we evaluated the efficacy of our vaccine prototype in immunization strategies aimed at reducing disease transmission by targeting colonization in the upper airways while maintaining protection against severe disease by reducing colonization in the lower respiratory tract.
Methods: We assessed different mucosal administration strategies in a murine model, including homologous mucosal 2-dose prime-boost schedules and heterologous prime-boost strategies combining intramuscular (IM) systemic immunization with mucosal routes (intranasal, IN; or sublingual, SL). We utilized alum and c-di-AMP as adjuvants for the systemic and mucosal formulations of the OMV vaccine prototype, respectively. A homologous prime/boost IM immunization schedule and commercial vaccines were used for comparisons.
Results: All tested heterologous schemes induced higher levels of specific IgG with significant avidity, as well as higher levels of IgG1 and IgG2c, compared to the corresponding homologous prime-boost 2-dose schemes via mucosal routes (OMVIN-IN or OMVSL-SL). High IgA levels were observed post-B. pertussis challenge following OMVIN-IN treatments and heterologous treatments where the second dose was administered via a mucosal route (prime-pull scheme). Furthermore, schemes involving the intranasal route, whether in a homologous or heterologous scheme, induced the highest levels of IL-17 and IFN-γ. Accordingly, these schemes showed superior efficacy against nasal colonization than the commercial vaccines. Homologous intranasal immunization exhibited the highest protective capacity against nasal colonization while maintaining an excellent level of protection in the lower respiratory tract. To further enhance protection against nasal colonization, we performed a comparative analysis of formulations containing either single or combined adjuvants, administered via homologous intranasal route. These assays revealed that the use of alum combined with c-di-AMP, did not enhance the immune protective capacity in comparison with that observed for the formulation containing c-di-AMP alone.
Conclusions: All the experiments presented here demonstrate that the use of OMVs, regardless of the scheme applied (except for OMVSL-SL), significantly outperformed acellular pertussis (aP) vaccines, achieving a higher reduction in bacterial colonization in the upper respiratory tract (p<0.01).
Whooping cough is a respiratory infectious disease that affects individuals of any age but is more severe in young children who are unvaccinated or have incomplete vaccination schedules. In unimmunized infants, the disease can be lethal. Characterized by bouts of violent coughing, vomiting after coughing, cyanosis, and apneas, the disease is caused by the Gram-negative bacterium B. pertussis (1). Studies in animal models and even in humans have shown that a robust humoral immune response and IFN-γ, produced by Th1 cells, play a critical role in protection against the symptoms caused by primary infection with B. pertussis and also in adaptive immunity against reinfection (2–5). Recent studies using the non-human primate model (baboons) have shown that Th17 cells and IgA also play a role in protective immunity against this bacterium (6, 7).
Vaccination is the preferred prevention strategy for this contagious disease. Currently, two types of pertussis vaccines exist, with the first one developed consisting of non-replicating cells from the causative agent (wP). This formulation that induces potent Th1 and Th17 cell responses, as well as the establishment of a tissue-resident memory population (TRM) (8, 9) is effective in preventing pertussis in children up to 7 years old. Adverse effects associated with wP vaccines led to their non-recommendation for the adolescent and adult population and prompted the development of a new generation of more tolerable vaccines based on purified B. pertussis immunogens (acellular vaccines, aP) (10–13). The aP vaccines were introduced in the late 90s into routine immunization programs in many developed countries. In recent years, the incidence of pertussis has increased in several countries, including those with high vaccination coverage (14–17). Various explanations have been proposed for the resurgence of pertussis, including improvements in integrated disease surveillance, the prevalence of circulating B. pertussis strains that are more resistant—likely due to bacterial genomic and phenotypic changes driven by selective vaccine pressure—to immunity conferred by vaccination (especially that induced by acellular vaccines), and the more rapid waning of vaccine-induced immunity in the case of aP vaccines (18–24). The resurgence of the disease has prompted a short to medium-term response, leading to the incorporation of additional vaccine boosters in the adolescent and adult population, particularly in pregnant women (25, 26). The data collected so far indicate that pregnant women vaccination strategy is proving successful (27, 28).
However, significant challenges persist in vaccination strategies that demand closer examination. A major concern is that current vaccines, particularly acellular ones, fail to effectively reduce bacterial colonization in the upper respiratory tract (6, 29, 30). As a result, even vaccinated individuals can continue to transmit the disease. This is especially concerning, as vaccinated individuals who become infected often remain asymptomatic, unknowingly facilitating the silent transmission of the pathogen (6, 31, 32). Recently, it has been demonstrated that replacing alum with adjuvants promoting Th1 cells and/or TRM, including Toll-like receptor (TLR) agonists, can enhance the protective efficacy of experimental aP vaccines in mice (33–35). In particular, the combination of LP1569 (a ligand for TLR2) and the agonist for the intracellular receptor stimulating interferon genes, c-di-GMP, in an aP formulation induced significant numbers of respiratory IL-17-secreting CD4 TRM cells and prevented nasal colonization for at least 10 months after immunization (35). In preclinical assays, we have previously demonstrated that outer membrane vesicles (OMVs) derived from B. pertussis show promise for evaluation as a candidate for a third generation of pertussis vaccines. They have proven to be safe, induce a Th1/Th17/Th2 profile, generate TRM cells, and exhibit significant capability to reduce bacterial colonization in the lower airways, which is associated with severe disease (36–43). We detected this protective capacity with the OMV administered both systemically and mucosally (38, 43). Raeven et al. (44) conducted comparative studies between vaccination schemes using OMVs derived from another strain of B. pertussis administered subcutaneously and intranasally. The results obtained showed that intranasal administration of OMVs is an appropriate strategy to reduce transmission (bacterial colonization in upper tract) and severe disease (bacterial colonization in lower respiratory tract) when animals were exposed to bacterial suspensions containing 105 CFU (44). In this study, aimed at further establishing OMVs as a viable immunization alternative. To this end, we assessed the impact of administering OMVs via different mucosal routes, sublingual and intranasal, in both homologous (same formulation and route for different doses) and heterologous (different formulations and routes for different doses) schemes on immunogenicity and protective capacity against bacterial colonization in the upper and lower respiratory tracts. Regarding the heterologous scheme, we incorporated a prime-pull strategy based on the rationale that intramuscular priming followed by an intranasal or sublingual booster would elicit robust systemic and mucosal immune responses. This approach may be particularly beneficial for booster vaccination in individuals who have already been immunized via the intramuscular route. We also assessed the protective efficacy of OMVs formulated with different adjuvants. For comparison purposes OMVs, aP and wP administered by intramuscular route were also evaluated.
C57bl/6 mice (4 weeks old), obtained from the Faculty of Veterinary Sciences, La Plata, Argentina, were kept in ventilated cages and housed under standardized conditions with regulated daylight, humidity, and temperature. The animals received food and water ad libitum. The animal experiments were authorized by the Ethical Committee for Animal Experiments of the Faculty of Science at La Plata National University (approval number 004-06-15, 003-06-15 extended its validity until August 10, 2027).
B. pertussis Tohama phase I strain CIP 8132 was used throughout this study as the strain for challenge in the murine model of protection. Bacteria were grown in Bordet–Gengou (BG) agar supplemented with 10% (v/v) defibrinated sheep blood (BG-blood agar) for 72 h at 36.5°C. Isolated colonies were replated in the same medium for 24 h and then resuspended in phosphate-buffered saline (PBS: 123 mM NaCl, 22.2 mM Na2HPO4, 5.6 mM KH2PO4 in MilliQ® nanopure water; pH 7.4). The optical density at 650 nm was measured and serial 10-fold dilutions plated onto BG-blood agar to determine the number of bacteria in the challenge inoculum.
OMVs were isolated and characterized as previously described (38, 45). Briefly, culture samples from the decelerating growth phase were centrifuged and the bacterial pellet obtained was resuspended in 20 mM Tris–HCl, 2 mM EDTA pH 8.5. The suspension was sonicated in cool water for 20 min. After two centrifugations at 10,000×g for 20 min at 4°C, the supernatant was pelleted at 100,000×g for 2 h at 4°C. This pellet was re-suspended in Tris buffer (20 mM pH 7.6). The samples obtained were negatively stained for electron microscope examination. Protein content was estimated by the Bradford method using bovine serum albumin as standard (46). The presence of the main immunogenic proteins in the OMVs was detected by immunoblot assays using specific antibodies as we previously described (not shown) (38, 40).
The characterized OMVs that range in size from approximately 50 to 200 nanometers in diameter were used to formulate the vaccine with tetanus (5 to 7 Lf/dose with a power greater than or equal to 2 UIA/ml serum) and diphtheria (1 to 3 Lf/dose with an output of 0.1 UIA/ml serum) toxoids as we previously described. OMVs formulated with alum (Alhydrogel 2%, CRODA), c-di-AMP (7.5 µg per dose) (Invivogen), or c-di-AMP (7.5 µg) combined with alum (Alhydrogel 2%) as adjuvants were evaluated throughout this study. To perform the experiments described below we verified that the OMV based vaccine prepared by us fulfilled the WHO criteria for safety in the weight-gain test (https://cdn.who.int/media/docs/default-source/biologicals/vaccine-standardization/pertussis/annex-6-whole-cell-pertussis.pdf?sfvrsn=f734b4_3&download=true). The safety of OMV-based vaccines was also confirmed by human whole-blood assays (47). This protocol evaluates the endotoxin activity of vaccines by measuring cytokine responses in a whole-blood setting. This approach allowed us to examine the safety and the tolerability of OMVs within a human-based ex vivo system, offering a relevant model to predict inflammatory potential and confirm the absence of excessive immune activation linked to OMV exposure.
Groups of 4-8 female C57BL/6 mice were immunized with OMV-based vaccine formulated as previously described with 3-6 µg total protein per dose formulated with alum, c-di-AMP or a combination of both as adjuvant (described in the legends to the figures), or 1:10 human dose of aP vaccine BOOSTRIX® [GlaxoSmithKline, with composition per human dose: pertussis toxoid (8 µg), pertactin (2.5 µg), filamentous hemagglutinin (8 µg), tetanus toxoid (20 IU), diphtheria toxoid (2 IU) and alum as adjuvant] or 1:10 human dose of whole cell vaccine (Serum Institute of India PVT LTD, composition per human dose is: diphtheria toxoid ≤ 25 Lf (≥ 30 IU), tetanus toxoid ≥ 5 Lf (≥ 40 IU), B. pertussis ≤ 16 OU (≥ 4 IU) adsorbed on aluminum phosphate ≤ 1.25 mg) or) using a 2-dose schedule. Two weeks after the last immunization blood samples were obtained. For protection assays mice were challenged with B. pertussis by intranasal inoculation (sublethal dose 1x107- 5x107 CFU 40μl-1) as is described below. Mice were sacrificed 1 week after challenge and bacterial counts were conducted following a previously established protocol (36, 37). Lungs and nose tissues were aseptically excised, homogenized in sterile PBS, serially diluted, and plated onto BG agar supplemented with defibrinated sheep blood to assess bacterial recovery at various time points post-infection. A minimum of three independent experiments were performed to ensure result robustness.
As we previously described (48), plates (Nunc A/S, Roskilde, Denmark) were coated with proteins from bacterial lysates at 3 µg/ml in 0.5 M carbonate buffer pH 9.5, by means of an overnight incubation at 4°C. Blocked plates with 3% milk in PBS (2 h 37°C) were incubated with serially diluted samples of mouse serum (1 h 37°C). Sera obtained after leaving the blood samples to clot for 1 h at 37°C followed by centrifuging for 10 min at 6,000xg. IgGs from individual serum or pooled sera bound to the plates were detected after a 2-h incubation with goat anti–mouse-IgG–linked horseradish peroxidase (1:8,000 Invitrogen, USA). For measuring IgG isotypes, detection of bound antibody was determined using HRP labeled subclass-specific anti-mouse IgG1 (1:3,000), IgG2c (1:2,000) (Invitrogen USA) or IgA (1/750) (Sigma Aldrich). As substrate 1.0 mg/ml o-phenylendiamine (OPD, Bio Basic Canada Inc) in 0.1 M citrate-phosphate buffer, pH 5.0 containing 0.1% hydrogen peroxide was used. Optical densities (ODs) were measured with Titertek Multiskan Model 340 microplate reader (ICN, USA) at 490 nm. From the experimental protocol performed in triplicate, one representative experiment is presented in the Results.
Avidity was measured by an ELISA elution assay as the overall strength of binding between antibody and antigen, using plates incubated for 10 min with increasing concentration of ammonium thiocyanate (NH4SCN) from 0 to 1 M. Antibody avidity was defined as the amount (percentage) of antibody retained for each increment of NH4SCN concentration.
After B. pertussis challenge, spleens from untreated and immunized mice were passed through a 40-mm cell strainer to obtain a single-cell suspension. Spleen cells (43) were seeded in 48 well culture plates in a final volume of 500 µl/well RPMI 1640 with 10% fetal bovine serum, containing 100 IU/ml penicillin and 100 µg/ml streptomycin. All cell samples were stimulated with OMV (2 µg/ml) or medium only. After 72 h of incubation (37°C and 5% CO2), IFN-γ, IL-5 and IL-17 concentrations were quantified in supernatants by ELISA (BD Biosciences, San Diego, USA), using conditions recommended by the manufacturer.
The data were evaluated statistically by two-way or one-way analysis of variance (ANOVA) followed by Bonferroni for multiple comparisons (via the GraphPad Prism® software). Differences were considered significant at a p <0.05.
To assess the immunogenicity and then the ability of OMVs to reduce B. pertussis colonization in the lower and upper respiratory tract, groups of mice were immunized with a 2-dose scheme administered with a formulation containing OMVs using mucosal routes (sublingual OMVSL or intranasal OMVIN) in homologous schemes (OMVSL-SL or OMVIN-IN) or heterologous schemes (OMVSL-IM, OMVIM-SL, OMVIN-IM, OMVIM-IN). A homologous 2-dose scheme using the intramuscular route (OMVIM-IM) was also incorporated for comparative analysis. For the intramuscular systemic route, we employed a previously tested and proven effective formulation comprising OMVs at a final protein concentration of 3 μg and alum as adjuvant (38, 43). In the case of mucosal routes, formulations containing OMVs at a final protein concentration of 6 μg, supplemented with the mucosal adjuvant c-di-AMP, were utilized. We selected this adjuvant due to its promising results in mucosal immunization and its effectiveness in eliciting IFN-γ and IL-17 responses (49) —both essential for protection against B. pertussis (8, 29). The experimental setup and timelines of the experiment are shown in Figure 1A. High levels of serum B. pertussis-specific IgG were obtained with OMVIM-IM and to a lesser extent in heterologous schemes, but not in homologous mucosal schemes or the non-immunized group (Figures 1B, C). Intranasal immunization elicited significantly higher B. pertussis-specific IgG antibody responses compared to sublingual immunization (p<0.001) (Figures 1D). Comparative avidity assays conducted with different concentrations of the chaotropic agent NH4SCN showed that among the homologous schemes, the OMVIM-IM scheme had the B. pertussis-specific IgG with the highest avidity (p<0.01 when 0.5 M of NH4SCN was used (Figure 1E, F). Additionally, higher antibody affinity was observed in sera obtained from the heterologous schemes, with the lowest affinity detected in sera from mice immunized with OMVSL-IM (Figure 1E).
Figure 1. Characterization of the humoral immune response induced by OMVs administered through heterologous and homologous immunization schemes. C57BL/6 mice (n=7/group) were immunized on days 0 and 14, with a 2-dose scheme administered with a formulation containing OMVs using mucosal routes (sublingual OMVSL or intranasal OMVIN) in homologous schemes (OMVSL-SL or OMVIN-IN) or heterologous schemes (OMVIM-SL OMVSL-IM, OMVIM-IN or OMVIN-IM),. A homologous 2-dose scheme using the intramuscular route (OMVIM-IM) was also incorporated for comparative analysis. Different bars colors are used to discriminate the route used for the first dose and the colors of circles denote the route used for the booster dose (A). Specific IgG levels induced by schedules that include SL administration (B), IN administration (C) or homologous mucosal administration (D) were determined in sera collected on day 14 after the last dose (absorbance values at 490 nm for 2 sera dilutions). The avidity of IgG antibodies was also measured 14 days post the second dose and the results are presented as percentages of B. pertussis-specific IgG antibodies retained after exposure with 0.5 M and 1M of ammonium thiocyanate (NH4SCN) (E, F). *p<0.05, ***p<0.001, ****p<0.0001 by two-way ANOVA using Bonferroni for multiple comparisons. The # symbol indicate significant differences between treatment and the other groups. #p<0.05, ##p<0.01, ###p<0.001, #### p <0.0001.
Regarding the levels of B. pertussis-specific IgG1 (Figure 2A, B), the highest levels were observed in the treatment group that received 2 doses of the OMVIM formulation (Figure 2A), and in the heterologous scheme group OMVIM-IN (p <0.0001). The levels detected for the prime-pull OMVIM-IN scheme were significantly higher than those detected for OMVIN-IM (p<0.0001, Figure 2B). Once again, the lowest levels of IgG1 were detected for the homologous schemes OMVSL-SL or OMVIN-IN (Figure 2A, B). No difference was detected in IgG1 levels between the mucosal homologous schemes.
Figure 2. Specific B. pertussis IgG isotypes and IgA levels induced by OMVs administered through different heterologous and homologous immunization schemes. Groups of C57BL6 mice were immunized with a 2-dose scheme as described in panel A of Figure 1. A homologous 2-dose scheme using the intramuscular route was incorporated for comparative analysis (OMVIM-IM) and a group of non-immunized mice was employed as a control. Specific IgG1 (A, B) and IgG2a (C, D) levels were determined in sera of immunized mice two weeks after last dose (absorbance values at 490 nm for 3 sera dilutions). (A, C): levels of IgG isotypes induced by schedules that include SL immunization. (B, D): levels of IgG isotypes induced by schedules that include IN administration. IgA antibody responses in serum were evaluated before and after intranasal B. pertussis challenge (absorbance values at 490 nm) in the groups of immunized mice with schedules that include SL (E) and IN (F) administration. *p<0.05, ****p<0.0001 by two-way ANOVA using Bonferroni for multiple comparisons. The # symbol indicate significant differences between OMVIM-IM and the other groups. # p<0.05, #### p <0.0001.
When assessing IgG2c, the highest levels were found in the OMVIN-IM and OMVIM-IM treatments (Figure 2C, D). However, it is important to note that the heterologous schemes involving OMVSL also induced significantly high levels, albeit lower than those observed in the former (Figure 2C). The IgG2c levels induced by OMVIM-IN were higher than those induced by OMVIN-IN (p<0.001). For mucosal homologous schemes and, as expected, the non-immunized control group, the levels of IgG2c isotypes were undetectable (Figure 2C, D). An interesting finding was that for all heterologous evaluated schemes except OMVIM-IN, the IgG2c/IgG1 ratios were higher than 1 (Supplementary Table S1), suggesting the induction of a Th1-directed immune response.
IgA antibody responses were only detected following intranasal B. pertussis challenge in mice treated with homologous intranasal immunization scheme and in those receiving the booster dose via mucosal route (prime-pull OMVIM-IN and OMVIM-SL heterologous treatments), whereas they were barely detectable in the other tested groups (Figure 2E, F).
IFN-γ (Th1 profile marker), IL-5 (Th2 profile marker) and IL-17 (Th17 profile marker) levels were also evaluated through splenocytes stimulation assays in mice challenged with a B. pertussis suspension (Figure 3A). The highest levels of OMV-specific IFN-γ and IL-17 were detected when OMVIN was included, either in a homologous or heterologous routes of immunization, with no significant differences observed between the heterologous treatments (Figure 3B, D). It was also found that IL-17 levels in the heterologous scheme OMVSL-IM were significantly higher than those induced by the other treatments containing any dose of OMVSL (p<0.01).
Figure 3. Characterization of cellular immune response induced by OMVs administered through heterologous and homologous immunization schemes. Mice were immunized on day 0 and 14, with a 2-dose scheme administered with a formulation containing OMVs using mucosal routes in homologous schemes or heterologous schemes, as described in Figure 1. Seven days after challenge with a sublethal dose (1x107-5x107 CFU/40 ul), mice were sacrificed, and their spleen cells were restimulated ex-vivo with 2μg/ml of OMV derived from B. pertussis or non-stimulated as controls (n/s). (A) Levels of secreted IFN-γ (B), IL-5 (C) and IL-7 (D) following splenocytes stimulation with medium or OMVs were determined by ELISA. Bars are means ± SEM of pg/ml. *p<0.05, **p<0.01, ***p<0.001, ****p<0.0001 by two-way ANOVA using Bonferroni for multiple comparisons. The # symbol indicate significant differences between the treatment and the other groups. ##p<0.01, ###p<0.001, #### p <0.0001.
The highest IL-5 levels were observed in heterologous schemes where the second dose was administered via IN route (Figure 3C).
These results demonstrate that the tested mucosal immunization schedules induced a robust specific humoral immune response when they were used in heterologous schemes showing higher levels of specific IgG in comparison with those detected for homologous mucosal schemes. It was also observed that the OMVs used in homologous or heterologous mucosal administration schemes induce a mixed Th1/Th17 profile, although more pronounced in schemes containing OMVIN and in the heterologous scheme OMVSL-IM. IL-5 was also detected in some of the heterologous treatments here tested. High levels of IgA were detected for OMVIN-IN and for prime-pull schemes OMVIM-SL and OMVIM-IN.
To assess the protective capacity exhibited by the different vaccination strategies tested here, groups of animals receiving 2-dose regimens were intranasally challenged with a suspension of B. pertussis at sublethal dose (1x107 – 5x107 CFU/40 μl) 14 days after receiving the last dose (Figure 3A). Seven days post-challenge mice, including those in the non-immunized group, were euthanized by cervical dislocation to evaluate bacterial colonization in the upper and lower respiratory tract. As expected, the non-immunized control group had a highest level of bacteria: 4.99x104 CFU/lungs and 4.42x104 CFU/nose (Figure 4A, B). Most immunized mice showed a significant reduction in the bacterial load in the lungs compared to non-immunized animals (p<0.0001), with the smallest reduction observed in the OMVSL-SL immunized mice (reduction of 1.67 logs). For the heterologous treatments OMVSL-IM and OMVIM-SL, significant reductions of 2.49 and 2.77 logs, respectively, were recorded. In the case of treatments that include an intranasal mucosal dose, reductions were 1.75 and 1.76 for mice immunized with OMVIM-IN and OMVIN-IM (Figure 4B). It is noteworthy that in the homologous schemes OMVIN-IN and OMVIM-IM, reductions were at least 3.22 logs, similar to what was observed for commercial aP (reduction of 3.08 logs, Figure 4C) and wP (reduction of 3.33 logs, Figure 4C) vaccines administered intramuscularly.
Figure 4. Effect of OMV mucosal homologous and heterologous immunization on protection against B. pertussis infection. C57BL/6 mice were immunized on days 0 and 14 with a 2-dose scheme OMV administered as described in panel A of Figure 1. Groups of animals immunized with commercial aP, or commercial wP vaccines or non-immunized were included as positive and negative protection control. Mice from all groups were challenged with a sublethal dose (1x107 – 5 x107/40 μl) B. pertussis Tohama phase I 14 days after the second dose followed by sacrifice 7 days after challenge. The number of bacteria recovered from mouse lungs (A-C) or nose (D-F) expressed as the log10 of CFUs per lungs or nose, is plotted on the ordinate, the different treatments here tested are indicated on the abscissa, with the data representing the means ± the SD. The dotted horizontal line indicates the lower limit of detection. The reduction detected in protection levels induced by different formulations in comparison with non-immunized animals is indicated at the top of the figures. *p<0.05, **p<0.01 by one way ANOVA using Bonferroni for multiple comparisons. The # symbol indicate significant differences between the treatment and the other tested groups. ## p<0.01, #### p <0.0001.
Similar results were observed regarding the ability to reduce bacterial colonization in the upper respiratory tract (nose) (Figure 4D, E). The heterologous treatments showed a reduction of at least 0.74 logs (Figure 4D, E), similar to that detected for mice that received 2 doses of wPIM (reduction of 0.78 logs) (Figure 4F). The heterologous schemes that induced the highest reduction were OMVIM-SL and OMV IN-IM with a reduction of at least 0.92 logs. The homologous schemes OMVIN-IN (reduction of 1.81 logs) and OMVIM-IM (reduction of 1.09 logs) but not OMVSL-SL, reduced bacterial colonization in the nose more effectively than the commercial aP vaccine applied in a 2-dose intramuscular scheme (no reduction) (p<0.001), (Figure 4F).
In summary, while homologous immunization with OMVIN-IN showed the highest protective capacity in both upper and lower airways, heterologous schemes, particularly OMVIM-SL, exhibited adequate levels of protection surpassing the protection against nasal carriage conferred by the commercial vaccines.
With the aim of evaluating whether the combination of c-di-AMP and alum adjuvants can improve the efficacy of the best scheme identified thus far in this study (OMVIN-IN), we conducted comparative assays using formulations containing the adjuvant combination versus those with a single adjuvant. For our assays, a group of animals immunized with OMV formulated without any adjuvant was used as a control to assess the protective capacity of OMV alone.
The idea of testing the adjuvant combination, particularly with alum—though controversial—arises from recent findings showing the antibody production-enhancing effects of alum in nasal administration, as a consequence of increased IL-33 (50). It has been suggested that alum induces necroptosis of alveolar epithelial cells, followed by the release of IL-33, which enhances antigen-specific IgA antibody production by activating ILC2s and increasing the MHC class II level for antigen uptake by APCs (50).
We again performed experiments involving 2-dose vaccination schemes. In these assays, we found that the addition of alum to formulations containing OMVs + c-di-AMP did not enhance IgG or IgG1 levels (Figure 5A, C). Furthermore, the highest antibody affinity was observed in the sera of mice that received two intranasal doses of OMVs formulated with c-di-AMP (Figure 5B). OMVs formulated without adjuvant induced higher IgG levels compared to those induced by the formulation with combined adjuvants. Additionally, OMVs without adjuvant or formulated with alum induced similar IgG1 levels, which were higher than those detected in the other treatments evaluated. Notably, the non-adjuvanted OMV group showed the highest increase in IgG2c levels (Figure 5D). In terms of IgA levels, both the group immunized with non-adjuvanted OMVs and the group treated with OMVs formulated with c-di-AMP exhibited the highest values (Figure 5E). No significant differences were observed in IFN-γ and IL-17 levels across all treatment groups (Figure 5F, H). A slight difference in IL-5 levels was detected, with the alum-adjuvanted OMV formulation inducing higher levels compared to both the c-di-AMP-adjuvanted and non-adjuvanted OMV formulations (Figure 5G).
Figure 5. Characterization of immunogenicity and the protection capacity induce by OMVs administered by intranasal route using different adjuvants. Groups of C57BL6 mice were immunized with a 2-dose scheme administered IN with a formulation containing OMVs formulated with c-di AMP, Alum, or c-di AMP plus Alum. As a control, a group of non-immunized were employed. Specific IgG levels induced by different schedules were determined in sera collected on day 14 after the last dose (absorbance values at 490 nm) (A). The avidity of IgG antibodies was also measured 14 days after the second dose and the results are presented as percentages of B. pertussis-specific IgG antibodies retained after exposure with 0.5 M and 1 M of ammonium thiocyanate (NH4SCN) (B). Specific IgG1 (C) and IgG2c levels (D) were determined in sera of immunized mice two weeks after last dose (absorbance values at 490 nm). Mice from all groups were challenged with a sublethal dose (1x107 – 5 x107/40 μl) B. pertussis Tohama phase I 14 days after the second dose followed by sacrifice 7 days after challenge. IgA antibody responses in serum were detected before and after intranasal B. pertussis challenge (absorbance values at 490 nm) (E). Levels of secreted IFN-γ (F), IL-5 (G) and IL-7 (H) following splenocytes stimulation with medium or OMVs were determined by ELISA. Bars are means ± SEM of pg/ml. *p<0.05, **p<0.01, ***p<0.001 by two-way ANOVA using Bonferroni for multiple comparisons. The # symbol indicate significant differences between the treatment and the other groups. # p<0.05, ### p <0.001.
Regarding the efficacy in reducing bacterial colonization in the upper and lower respiratory tracts (Figure 6), the formulation containing the two adjuvants did not improve the protective capacity induced by OMVs+c-di-AMP formulation. In fact, the group of animals immunized with the c-di-AMP formulation showed the most substantial reduction. In the lower respiratory tract, bacterial colonization was reduced by 3.30 logs compared to non-immunized animals, whereas in the upper respiratory tract the reduction was 1.81 logs. The non-adjuvanted OMV formulation also induced a significant reduction in both the lower and upper respiratory tracts, with reductions of 1.8 logs and 1.38 logs, respectively. The lowest reduction in the upper respiratory tract was observed in the control group treated with OMVs formulated with alum (0.76 logs, Figure 6).
Figure 6. Effect of OMV intranasal homologous immunization on protection against B. pertussis infection. The number of bacteria recovered from mouse lungs (left panel A) or nose (right panel B) expressed as the log10 of CFUs per lungs or nose, is plotted on the ordinate, the different treatments here tested are indicated on the abscissa, with the data representing the means ± SEM. The reduction detected in protection levels induced by different formulations in comparison with non-immunized animals is indicated at the top of the figures. The dotted horizontal line indicates the lower limit of detection. p<0.05, **p<0.01, ***p<0.001, by one way ANOVA using Bonforroni for multiple comparisons. The # symbol indicate significant differences between the treatment and the other tested groups. ## p<0.01.
We have previously demonstrated that B. pertussis OMVs, which contain a diverse array of immunogens and PAMPs, represent a safe and effective vaccine prototype for preventing bacterial colonization in the lower respiratory tract (associated with severe disease) when administered either parenterally or intranasally (38, 42). Recently, we also demonstrated the adjuvant properties of OMVs when delivered through both systemic and mucosal routes (51). In this study, we evaluated the efficacy of Bordetella pertussis-derived outer membrane vesicles (OMVs) administered through mucosal immunization, specifically via sublingual or intranasal routes, to confer protective immunity not only in the lower respiratory tract but also in the upper respiratory tract, given its key role in disease transmission. We employed two-dose regimens involving either homologous (same route) or heterologous (different route) administration, including the prime-pull strategy, where the first dose is delivered systemically, and the second dose is administered via the mucosal route. In all cases involving mucosal immunization, a double dose of antigen (OMVs) was utilized compared to systemic administration, due to the partial loss of antigen crossing the mucosal barrier (50).
While many questions remain about sublingual immunization, such as identifying appropriate adjuvants and optimizing formulations to improve efficacy, there is a solid body of preclinical evidence demonstrating its safety and effectiveness in protecting against various respiratory pathogens, including influenza, RSV, and SARS-CoV-2 (52, 53). Regarding intranasal vaccination, the presence of abundant lymphatic tissue in the nasal cavity, which triggers both humoral (particularly the production of immunoglobulin A, accounting for more than 15% of total immunoglobulins) and cellular immune responses, has prompted numerous studies confirming its safety and efficacy (54).
Our findings show that OMVs formulated with c-di-AMP and administered via the sublingual route in a heterologous schedule, or intranasally in both homologous and heterologous schedules, significantly reduce bacterial colonization in both the upper and lower respiratory tracts. In heterologous schedules involving sublingual administration, colony counts in the lower respiratory tract were reduced by at least 309-fold, and by at least 5-fold in the upper respiratory tract. Interestingly, the heterologous prime-pull OMVIM-SL schedule achieved the highest reductions, with a 588-fold decrease in the lungs and an 8-fold decrease in the nasal cavity compared to CFU counts in non-immunized mice (p< 0.01). Conversely, the least immunogenic and protective scheme was the homologous OMVSL-SL schedule. The highest reductions in colonization in both the lungs and nose were observed in schedules that included at least one intranasal dose of OMVs formulated with c-di-AMP, with the OMVIN-IN schedule yielding the highest reductions—1995-fold in the lungs and 64-fold in the nasal cavity—compared to non-immunized animals (p< 0.01). This result is consistent with the findings of Raeven et al. (44), who reported similar outcomes in a comparative study of OMV vaccination schemes. However, differences in colonization levels between their study and ours are likely attributable to variations in sampling methods (nasal lavages versus nasal tissue), the bacterial strains used to obtain OMVs, and the challenge doses administered.
Consistent with previously published data from baboon models, our study in mice further emphasizes the inherent limitations of acellular pertussis (aP) vaccines adjuvanted with alum in controlling bacterial colonization within the nasal cavity (6, 35). While intramuscular immunization with alum-adjuvanted aP vaccines effectively prevents lung infections, it does not significantly reduce nasal colonization by B. pertussis. Moreover, intranasal administration of the aP vaccine demonstrated poor protection against bacterial colonization in both the lungs and the nasal cavity, with colonization levels comparable to those of non-immunized animals (Supplementary Figure S1). As a result, the current formulation of this vaccine, regardless of whether it is delivered via the systemic or mucosal route, is unlikely to have a meaningful impact on horizontal transmission to susceptible hosts. In contrast, commercially available whole-cell pertussis (wP) vaccines, even when administered intramuscularly—as still practiced in several countries, including Argentina—demonstrated a substantial reduction in lung colonization (approximately 2000-fold) and nasal colonization (6-fold) compared to non-immunized controls. Likewise, OMV-based formulations, when administered systemically in a homologous two-dose scheme, significantly reduced bacterial colonization in both the lungs and nasal cavity, achieving over a 1600-fold reduction in the lungs and a 12-fold reduction in the nasal cavity relative to non-immunized animals.
Our findings underscore the superior efficacy of OMV-based formulations, particularly the OMVIN-IN schedule containing OMVs formulated with c-di-AMP, when compared to currently available vaccines. Even though the formulation of OMVs with combined adjuvants (c-di-AMP plus alum) did not enhance the overall efficacy compared to OMVs with c-di-AMP alone, it still demonstrated substantial reductions in colonization in both the lower and upper respiratory tracts. Importantly, this dual-adjuvant formulation showed a clear advantage over the acellular pertussis vaccine, especially in reducing colonization in the upper respiratory tract. Notably, even OMVs without any adjuvant significantly reduced nasal colonization, achieving a 24-fold decrease relative to non-immunized animals (p < 0.01).
Another important finding of our study is that prime-pull schemes, while less effective than the homologous OMVIN-IN schedule, demonstrated superior results in terms of the reduction of nasal colonization compared to the currently used commercial vaccines, specifically both wP and aP. In fact, these schemes, which delivered the second dose via the mucosal route, similarly to the homologous OMVIN-IN schedule, induced elevated IgA levels post-challenge. Furthermore, schedules incorporating an intranasal dose (either homologous or heterologous) elicited high levels of IL-17, a cytokine described as crucial for protection in the upper respiratory tract. Previous studies from our team showed the intrinsic capacity of intranasal vaccination to promote per se strong IL-17 responses (55). In this regard, Solans et al. (56) highlighted the importance of IL-17 and IgA in mediating protection against B. pertussis nasopharyngeal colonization, emphasizing the differential mechanisms of protection in the upper (IgA and IL-17) and lower respiratory tract, where complete bacterial clearance primarily depends on cellular immunity mediated by T-helper type 1 (Th1) and Th17 cells (4).
In conclusion, OMV-containing formulations represent a promising prototype that should be considered for further evaluation as a third-generation vaccine against pertussis. When formulated with c-di-AMP and administered via the intranasal route, whether in homologous or heterologous schedules, these formulations effectively induce humoral, IL-17, and IFN-γ responses, offering protection against B. pertussis colonization in the lungs, but also in the nasal cavity, at levels superior to those observed with currently available commercial vaccines.
Since the beginning of 2024, the government has decided to defund science, and as a result, the money from our grants has not been transferred to the funding units of our institutions so far.
The datasets presented in this study can be found in online repositories. The names of the repository/repositories and accession number(s) can be found in the article/Supplementary Material.
The animal experiments were authorized by the Ethical Committee for Animal Experiments of the Faculty of Science at La Plata National University (approval number 004-06-15, 003-06-15 extended its validity until August 10, 2027). The study was conducted in accordance with the local legislation and institutional requirements.
ER: Investigation, Methodology, Writing – review & editing. EG: Formal analysis, Investigation, Methodology, Writing – review & editing. DB: Formal analysis, Methodology, Writing – review & editing. TE: Formal analysis, Methodology, Writing – review & editing. CG: Formal analysis, Investigation, Writing – review & editing. DH: Conceptualization, Data curation, Formal analysis, Funding acquisition, Investigation, Methodology, Project administration, Supervision, Writing – original draft, Writing – review & editing.
The author(s) declare that financial support was received for the research, authorship, and/or publication of this article. This work was supported by ANCPyT (PICT 2020 3034) PIP 3022 grants to HD. HD, GE and BD are members of the Scientific Career of CONICET. RE is fellow from CONICET.
Luciana Cayuela and Alejandro Fernández provided excellent technical assistance.
The authors declare that the research was conducted in the absence of any commercial or financial relationships that could be construed as a potential conflict of interest.
The author(s) declared that they were an editorial board member of Frontiers, at the time of submission. This had no impact on the peer review process and the final decision.
All claims expressed in this article are solely those of the authors and do not necessarily represent those of their affiliated organizations, or those of the publisher, the editors and the reviewers. Any product that may be evaluated in this article, or claim that may be made by its manufacturer, is not guaranteed or endorsed by the publisher.
The Supplementary Material for this article can be found online at: https://www.frontiersin.org/articles/10.3389/fimmu.2024.1506638/full#supplementary-material
Supplementary Figure 1 | Effect of aP immunization on protection against B. pertussis infection. C57BL/6 mice were immunized on days 0 and 14, with a 2-dose scheme aP administered by intramuscular or intranasal route. Mice from all groups were challenged with a sublethal dose (1x107 – 5 x107/40 μl) B. pertussis Tohama phase I 14 days after the second dose followed by sacrifice 7 days after challenge. The number of bacteria recovered from mouse lungs (A) or nose (B) expressed as the log10 of CFUs per lungs or nose, is plotted on the ordinate, the different treatments here tested are indicated on the abscissa, with the data representing the means ± SEM. The dotted horizontal line indicates the lower limit of detection. The reduction detected in protection levels induced by different formulations in comparison with non-immunized animals is indicated at the top of the figures. ****p<0.0001 by one way ANOVA using Bonferroni for multiple comparisons.
1. Tozzi AE, Gesualdo F, Rizzo C, Carloni E, Russo L, Campagna I, et al. A data driven clinical algorithm for differential diagnosis of pertussis and other respiratory infections in infants. PloS One. (2020) 15:e0236041. doi: 10.1371/journal.pone.0236041
2. Barbic J, Leef MF, Burns DL, Shahin RD. Role of gamma interferon in natural clearance of Bordetella pertussis infection. Infect Immun. (1997) 65:4904–8. doi: 10.1128/IAI.65.12.4904-4908.1997
3. Mills KHG, Redhead K. Cellular immunity in pertussis. J Med Microbiol. (1993) 39:163–4. doi: 10.1099/00222615-39-3-163
4. Higgs R, Higgins SC, Ross PJ, Mills KHG. Immunity to the respiratory pathogen Bordetella pertussis. Mucosal Immunol. (2012) 5:485–500. doi: 10.1038/mi.2012.54
5. Mills KHG, Ryan M, McGuirk P, Griffin F, Murphy G, Mahon B. The immunology of Bordetella pertussis infection. Biologicals. (1999) 27:77–89. doi: 10.1006/biol.1999.0183
6. Warfel JM, Zimmerman LI, Merkel TJ. Acellular pertussis vaccines protect against disease but fail to prevent infection and transmission in a nonhuman primate model. Proc Natl Acad Sci U.S.A. (2014) 111:787–92. doi: 10.1073/pnas.1314688110
7. Locht C, Papin JF, Lecher S, Debrie AS, Thalen M, Solovay K, et al. Live attenuated pertussis vaccine BPZE1 protects baboons against bordetella pertussis disease and infection. J Infect Dis. (2017) 216:117–24. doi: 10.1093/INFDIS/JIX254
8. Brummelman J, Wilk MM, Han WGH, Van Els ACM, Mills KHG. Roads to the development of improved pertussis vaccines paved by immunology. (2015) 73:1–12. doi: 10.1093/femspd/ftv067
9. Wilk MM, Borkner L, Misiak A, Curham L, Allen AC, Mills KHG. Immunization with whole cell but not acellular pertussis vaccines primes CD4 TRM cells that sustain protective immunity against nasal colonization with Bordetella pertussis. Emerg Microbes Infect. (2019) 8:169–85. doi: 10.1080/22221751.2018.1564630
10. Sato Y, Kimura M, Fukumi H. Development of a pertussis component vaccine in Japan. Lancet. (1984) 323:122–6. doi: 10.1016/S0140-6736(84)90061-8
11. Pichichero ME, Rennels MB, Edwards KM, Blatter MM, Marshall GS, Bologa M, et al. Combined tetanus, diphtheria, and 5-component pertussis vaccine for use in adolescents and adults. J Am Med Assoc. (2005) 293:3003–11. doi: 10.1001/jama.293.24.3003
12. Halperin SA, Scheifele D, Mills E, Guasparini R, Humphreys G, Barreto L, et al. Nature, evolution, and appraisal of adverse events and antibody response associated with the fifth consecutive dose of a five-component acellular pertussis-based combination vaccine. Vaccine. (2003) 21:2298–306. doi: 10.1016/S0264-410X(03)00173-7
13. Gustafsson L, Hallander HO, Olin P, Reizenstein E, Storsaeter J. A controlled trial of a two-component acellular, a five-component acellular, and a whole-cell pertussis vaccine. N Engl J Med. (1996) 334:349–56. doi: 10.1056/NEJM199602083340602
14. Tan T, Dalby T, Forsyth K, Halperin SA, Heininger U, Hozbor D, et al. Pertussis across the globe: recent epidemiologic trends from 2000 to 2013. Pediatr Infect Dis J. (2015) 34:pe22–232. doi: 10.1097/INF.0000000000000795
15. Clark TA. Changing pertussis epidemiology: Everything old is new again. J Infect Dis. (2014) 209:978–81. doi: 10.1093/infdis/jiu001
16. Choi YH, Campbell H, Amirthalingam G, van Hoek AJ, Miller E. Investigating the pertussis resurgence in England and Wales, and options for future control. BMC Med. (2016) 14:121–31. doi: 10.1186/s12916-016-0665-8
17. Celentano LP, Massari M, Paramatti D, Salmaso S, Tozzi AE. Resurgence of pertussis in europe. Pediatr Infect Dis J. (2005) 24:761–5. doi: 10.1097/01.inf.0000177282.53500.77
18. Breakwell L, Kelso P, Finley C, Schoenfeld S, Goode B, Misegades LK, et al. Pertussis vaccine effectiveness in the setting of pertactin-deficient pertussis. Pediatrics. (2016) 137:e20153973. doi: 10.1542/peds.2015-3973
19. Ma L, Caulfield A, Dewan KK, Harvill ET. Pertactin-deficient bordetella pertussis, vaccine-driven evolution, and reemergence of pertussis. Emerg Infect Dis. (2021) 27:1561–6. doi: 10.3201/eid2706.203850
20. Mooi FR, van der Maas NAT, De Melker HE. Pertussis resurgence: Waning immunity and pathogen adaptation - Two sides of the same coin. Epidemiol Infect. (2014) 142:685–94. doi: 10.1017/S0950268813000071
21. Loconsole D, De Robertis AL, Morea A, Metallo A, Lopalco PL, Chironna M. Resurgence of pertussis and emergence of the ptx p3 toxin promoter allele in south Italy. Pediatr Infect Dis J. (2018) 37:E126–31. doi: 10.1097/INF.0000000000001804
22. Sealey KL, Belcher T, Preston A. Bordetella pertussis epidemiology and evolution in the light of pertussis resurgence. Infection Genet Evol. (2016) 40:136–43. doi: 10.1016/J.MEEGID.2016.02.032
23. Mooi FR, Van Loo IHM, King AJ. Adaptation of Bordetella pertussis to vaccination: a cause for its reemergence? Emerg Infect Dis. (2001) 7:526–8. doi: 10.3201/eid0707.017708
24. He Q, Mertsola J. Factors contributing to pertussis resurgence. Future Microbiol. (2008) 3:329–39. doi: 10.2217/17460913.3.3.329
25. Baxter R, Bartlett J, Fireman B, Lewis E, Klein NP. Effectiveness of vaccination during pregnancy to prevent infant pertussis. Pediatrics. (2017) 139:e20164091. doi: 10.1542/PEDS.2016-4091
26. Campbell H, Gupta S, Dolan GP, Kapadia SJ, Singh AK, Andrews N, et al. Review of vaccination in pregnancy to prevent pertussis in early infancy. J Med Microbiol. (2018) 67:1426–56. doi: 10.1099/jmm.0.000829
27. Vizzotti C, Neyro S, Katz N, Juárez MV, Perez Carrega ME, Aquino A, et al. Maternal immunization in Argentina: A storyline from the prospective of a middle income country. Vaccine. (2015) 33:6413–9. doi: 10.1016/j.vaccine.2015.07.109
28. Abu-Raya B, Forsyth K, Halperin SA, Maertens K, Jones CE, Heininger U, et al. Vaccination in pregnancy against pertussis: A consensus statement on behalf of the global pertussis initiative. Vaccines (Basel). (2022) 10:1990–2002. doi: 10.3390/VACCINES10121990
29. Schmitt P, Borkner L, Jazayeri SD, McCarthy KN, Mills KH. Nasal vaccines for pertussis. Curr Opin Immunol. (2023) 84:102355–64. doi: 10.1016/J.COI.2023.102355
30. Chasaide CN, Mills KHG. Next-generation pertussis vaccines based on the induction of protective t cells in the respiratory tract. Vaccines (Basel). (2020) 8:1–28. doi: 10.3390/vaccines8040621
31. Lapidot R, Gill CJ. The Pertussis resurgence: Putting together the pieces of the puzzle. Trop Dis Travel Med Vaccines. (2016) 2:26–34. doi: 10.1186/s40794-016-0043-8
32. Althouse BM, Scarpino SV. Asymptomatic transmission and the resurgence of Bordetella pertussis. BMC Med. (2015) 13:146. doi: 10.1186/s12916-015-0382-8
33. Dunne A, Mielke LA, Allen AC, Sutton CE, Higgs R, Cunningham CC, et al. A novel TLR2 agonist from Bordetella pertussis is a potent adjuvant that promotes protective immunity with an acellular pertussis vaccine. Mucosal Immunol. (2015) 8:607–17. doi: 10.1038/MI.2014.93
34. Asokanathan C, Corbel M, Xing D. A CpG-containing oligodeoxynucleotide adjuvant for acellular pertussis vaccine improves the protective response against Bordetella pertussis. Hum Vaccin Immunother. (2013) 9:325–31. doi: 10.4161/HV.22755
35. Allen AC, Wilk MM, Misiak A, Borkner L, Murphy D, Mills KHG. Sustained protective immunity against Bordetella pertussis nasal colonization by intranasal immunization with a vaccine-adjuvant combination that induces IL-17-secreting T RM cells. Mucosal Immunol. (2018) 11:1763–76. doi: 10.1038/s41385-018-0080-x
36. Hozbor DF. Outer membrane vesicles: an attractive candidate for pertussis vaccines. Expert Rev Vaccin. (2017) 16(3):193–6. doi: 10.1080/14760584.2017.1276832
37. Zurita ME, Wilk MM, Carriquiriborde F, Bartel E, Moreno G, Misiak A, et al. A Pertussis Outer Membrane Vesicle-Based Vaccine Induces Lung-Resident Memory CD4 T Cells and Protection against Bordetella pertussis, including Pertactin Deficient Strains. Front Cell Infect Microbiol. (2019) 9:125. doi: 10.3389/fcimb.2019.00125
38. Roberts R, Moreno G, Bottero D, Gaillard ME, Fingermann M, Graieb A, et al. Outer membrane vesicles as acellular vaccine against pertussis. Vaccine. (2008) 26. doi: 10.1016/j.vaccine.2008.07.004
39. Elizagaray ML, Gomes MTR, Guimaraes ES, Rumbo M, Hozbor DF, Oliveira SC, et al. Canonical and non-canonical inflammasome activation by outer membrane vesicles derived from bordetella pertussis. Front Immunol. (2020) 11:1879. doi: 10.3389/fimmu.2020.01879
40. Asensio CJA, Gaillard ME, Moreno G, Bottero D, Zurita E, Rumbo M, et al. Outer membrane vesicles obtained from Bordetella pertussis Tohama expressing the lipid a deacylase PagL as a novel acellular vaccine candidate. Vaccine. (2011) 29:1649–56. doi: 10.1016/j.vaccine.2010.12.068
41. Carriquiriborde F, Martin Aispuro P, Ambrosis N, Zurita E, Bottero D, Gaillard ME, et al. Pertussis vaccine candidate based on outer membrane vesicles derived from biofilm culture. Front Immunol. (2021) 12:730434. doi: 10.3389/fimmu.2021.730434
42. Bottero D, Gaillard MEE, Zurita E, Moreno G, Martinez DSS, Bartel E, et al. Characterization of the immune response induced by pertussis OMVs-based vaccine. Vaccine. (2016) 34:3303–9. doi: 10.1016/j.vaccine.2016.04.079
43. Gaillard ME, Bottero D, Errea A, Ormazábal M, Zurita ME, Moreno G, et al. Acellular pertussis vaccine based on outer membrane vesicles capable of conferring both long-lasting immunity and protection against different strain genotypes. Vaccine. (2014) 32:931–7. doi: 10.1016/j.vaccine.2013.12.048
44. Raeven RHM, Rockx-Brouwer D, Kanojia G, van der Maas L, Bindels THE, ten Have R, et al. Intranasal immunization with outer membrane vesicle pertussis vaccine confers broad protection through mucosal IgA and Th17 responses. Sci Rep. (2020) 10:7396–402. doi: 10.1038/S41598-020-63998-2
45. Hozbor D, Rodriguez MEE, Fernández J, Lagares A, Guiso N, Yantorno O. Release of outer membrane vesicles from Bordetella pertussis. Curr Microbiol. (1999) 38:273–8. doi: 10.1007/PL00006801
46. Kielkopf CL, Bauer W, Urbatsch IL. Bradford assay for determining protein concentration. Cold Spring Harb Protoc. (2020) 2020:136–8. doi: 10.1101/pdb.prot102269
47. Stoddard MB, Pinto V, Keiser PB, Zollinger W. Evaluation of a whole-blood cytokine release assay for use in measuring endotoxin activity of group B neisseria meningitidis vaccines made from lipid A acylation mutants. Clin Vaccine Immunol. (2010) 17:98. doi: 10.1128/CVI.00342-09
48. Martin Aispuro P, Ambrosis N, Zurita ME, Gaillard ME, Bottero D, Hozbor DF. Use of a neonatal-mouse model to characterize vaccines and strategies for overcoming the high susceptibility and severity of pertussis in early life. Front Microbiol. (2020) 11:723. doi: 10.3389/fmicb.2020.00723
49. Cheng X, Ning J, Xu X, Zhou X. The role of bacterial cyclic di-adenosine monophosphate in the host immune response. Front Microbiol. (2022) 13:958133. doi: 10.3389/FMICB.2022.958133
50. Sasaki E, Asanuma H, Momose H, Furuhata K, Mizukami T, Hamaguchi I. Nasal alum-adjuvanted vaccine promotes IL-33 release from alveolar epithelial cells that elicits IgA production via type 2 immune responses. PloS Pathog. (2021) 17:e1009890. doi: 10.1371/JOURNAL.PPAT.1009890
51. Pschunder B, Locati L, López O, Martin Aispuro P, Zurita E, Stuible M, et al. Outer membrane vesicles derived from Bordetella pertussis are potent adjuvant that drive Th1-biased response. Front Immunol. (2024) 15:1387534. doi: 10.3389/fimmu.2024.1387534
52. Song JH, Nguyen HH, Cuburu N, Horimoto T, Ko SY, Park SH, et al. Sublingual vaccination with influenza virus protects mice against lethal viral infection. Proc Natl Acad Sci U.S.A. (2008) 105:1644–9. doi: 10.1073/PNAS.0708684105
53. Kim S, Joo DH, Lee JB, Shim BS, Cheon IS, Jang JE, et al. Dual role of respiratory syncytial virus glycoprotein fragment as a mucosal immunogen and chemotactic adjuvant. PloS One. (2012) 7:e32226. doi: 10.1371/JOURNAL.PONE.0032226
54. Yusuf H, Kett V. Current prospects and future challenges for nasal vaccine delivery. Hum Vaccin Immunother. (2017) 13:34–45. doi: 10.1080/21645515.2016.1239668
55. Zygmunt BM, Rharbaoui F, Groebe L, Guzman CA. Intranasal immunization promotes th17 immune responses. J Immunol. (2009) 183:6933–8. doi: 10.4049/JIMMUNOL.0901144
Keywords: Bordetella pertussis, outer-membrane vesicles, mucosal, intranasal, IgA
Citation: Rudi E, Gaillard E, Bottero D, Ebensen T, Guzman CA and Hozbor D (2024) Mucosal vaccination with outer membrane vesicles derived from Bordetella pertussis reduces nasal bacterial colonization after experimental infection. Front. Immunol. 15:1506638. doi: 10.3389/fimmu.2024.1506638
Received: 05 October 2024; Accepted: 01 November 2024;
Published: 28 November 2024.
Edited by:
Paola Massari, Tufts University, United StatesReviewed by:
Peter Van Der Ley, Intravacc (Netherlands), NetherlandsCopyright © 2024 Rudi, Gaillard, Bottero, Ebensen, Guzman and Hozbor. This is an open-access article distributed under the terms of the Creative Commons Attribution License (CC BY). The use, distribution or reproduction in other forums is permitted, provided the original author(s) and the copyright owner(s) are credited and that the original publication in this journal is cited, in accordance with accepted academic practice. No use, distribution or reproduction is permitted which does not comply with these terms.
*Correspondence: Daniela Hozbor, aG96Ym9yLmRhbmllbGFAZ21haWwuY29t; aG96Ym9yQGJpb2wudW5scC5lZHUuYXI=
Disclaimer: All claims expressed in this article are solely those of the authors and do not necessarily represent those of their affiliated organizations, or those of the publisher, the editors and the reviewers. Any product that may be evaluated in this article or claim that may be made by its manufacturer is not guaranteed or endorsed by the publisher.
Research integrity at Frontiers
Learn more about the work of our research integrity team to safeguard the quality of each article we publish.