- 1Inflammation and Chronic Disease Program, The Ottawa Hospital Research Institute, Ottawa, ON, Canada
- 2Department of Medicine, Centre Hospitalier de l’Université de Montréal, Montreal, QC, Canada
- 3Department of Medicine, McGill University Health Centre, Montreal, QC, Canada
- 4IWK Health, Department of Pediatrics, Dalhousie University, Halifax, NS, Canada
- 5Centre Hospitalier de l’Université (CHU) Ste Justine, Centre de Recherche, Montreal, QC, Canada
- 6Department of Pediatrics, Children’s Hospital of Eastern Ontario, Ottawa, ON, Canada
- 7The Hospital for Sick Children, Department of Pediatrics, University of Toronto, Toronto, ON, Canada
- 8Clinical Immunology and Allergy, Unity Health Toronto, Toronto, ON, Canada
- 9Department of Pediatrics and Child Health, University of Manitoba, Winnipeg, MB, Canada
- 10Department of Pediatrics, University of Alberta, Edmonton, AB, Canada
- 11Alberta Children’s Hospital, Calgary, AB, Canada
- 12Department of Medicine, Dalhousie University, Halifax, NS, Canada
- 13Department of Biochemistry, Microbiology, and Immunology, University of Ottawa, Ottawa, ON, Canada
- 14Department of Pediatrics, University of British Columbia, Vancouver, BC, Canada
Purpose: Many individuals with inborn errors of immunity (IEIs) have poor humoral immune (HI) vaccine responses. Only a few studies have examined specific cell-mediated immune (CMI) responses to coronavirus disease 2019 (COVID-19) vaccines in this population. Therefore, the purpose of this study was to examine HI and CMI responses up to 6 months post-COVID-19 vaccine dose 3 in adults with IEIs.
Methods: A multi-center prospective observational study was conducted across Canada to collect severe acute respiratory syndrome coronavirus-2 (SARS-CoV-2)-specific HI and CMI data at 4- and 24-week intervals after vaccine doses 2 and 3 (D2 + 4wk/D2 + 24wk/D3 + 4wk/D3 + 24wk).
Results: A total of 149 adults with IEIs and 423 healthy controls were recruited from July 2021 to October 2023. Geometric mean anti-spike IgG (binding antibody units/mL) and spike-specific T-cell responses [IFN-γ+ T cells/106 peripheral blood mononuclear cells (PBMCs)] were significantly lower in IEIs compared to controls at D2 + 4wk, D3 + 4wk, and D3 + 24wk. However, at 6 months after completing the primary series (three doses for IEIs and two doses for healthy), both HI and CMI responses of both IEI participants and healthy controls persisted and were comparable. There was a strong correlation between neutralizing antibody titer (ID50) and anti-spike IgG but not between ID50 and CMI. There was only one reported case of hospitalized COVID-19 disease before and none after completing the primary series among IEI participants.
Conclusion: Adults with IEIs mounted both HI and CMI responses following COVID-19 vaccines, which were lower than those of healthy individuals but were present at least up to 6 months after dose 3. These data support the initial recommendation for a three-dose primary series among IEIs.
Introduction
Individuals with inborn errors of immunity (IEIs) have germline variants in single genes that lead to defects in the innate or adaptive immune system. IEIs are classified by the branch of the immune system affected: immunodeficiencies affecting cellular and humoral immunity, predominantly antibody deficiencies, congenital defects of phagocytes, and complement deficiencies, as well as other defects in intrinsic and innate immunity. The spectrum of diseases included in IEIs is an ever-growing area of clinical research and also includes diseases of immune dysregulation, autoinflammatory disorders, and bone marrow failure. Many individuals with IEIs are at increased risk of certain types of infections but may also present with features of autoimmunity and malignancy and other features of immune dysregulation. Individuals with IEIs have been found to be at increased risk of severe coronavirus disease 2019 (COVID-19), with higher rates of hospitalization, intensive care unit (ICU) admission, and death compared to the general population (1–4). Further, several case reports and case series have described an increased frequency of protracted COVID-19, prolonged viral replication, and variant evolution in patients with immunodeficiencies (5–14).
COVID-19 vaccines are one of the most effective public health strategies to reduce morbidity and mortality from COVID-19 (15–18). Studies have demonstrated that individuals with IEIs often have suboptimal immune responses to vaccines, and therefore, additional vaccine doses may be warranted (19). Most immunization advisory groups initially recommended a three-dose COVID-19 primary series for individuals with immunodeficiencies as compared to two doses for healthy individuals (20–22). However, few studies have compared the responses, especially cell-mediated immune (CMI) responses, between individuals with IEIs and healthy individuals after dose 3 (23).
The primary objective of this study was to longitudinally assess both humoral immune (HI) and CMI responses to COVID-19 vaccines available in Canada [BNT162b2 (Pfizer-BioNTech), mRNA-1273 (Moderna), or ChAdOx1 nCoV-19 (AstraZeneca)] in patients with IEIs versus healthy individuals up to 6 months following their primary vaccination series. The secondary objective was to measure the frequency and severity of COVID-19 infections post-vaccination and vaccine safety among these patients.
Materials and methods
Study design and participants
A multi-center prospective observational cohort study was conducted between July 2021 and March 2024 at seven adult and seven pediatric centers to assess COVID-19 vaccination immunogenicity and safety in IEIs. The study was conducted in accordance with the Declaration of Helsinki. The Ottawa Hospital Research Institute was the lead site and obtained overarching ethics approval (CTO#1978) for Ontario sites. Each study site also obtained approval from their local ethics board prior to study commencement. All participants provided written informed consent or assent before enrollment. There was no restriction on the number of COVID-19 vaccine doses received prior to study enrolment if they were less than 24 weeks after dose 4 and received any of the following vaccines available in Canada: BNT162b2, mRNA-1273, or ChAdOx1 nCoV-19.
The current article reports data on adult participants aged 18 years or older and up to 24 weeks after dose 3. Eligible participants with IEIs were recruited into three study subgroups based on the type of IEIs (24): A) predominant antibody deficiency (PAD), B) immunodeficiencies affecting cellular and humoral immunity [combined B-cell and T-cell immunodeficiency (CID)], and C) defects of intrinsic and innate immunity (III) or other IEIs not meeting criterion A) or B). Healthy individuals who did not report any known immunocompromising conditions at the time of initial study screening were recruited as controls. Additional healthy controls meeting our eligibility criteria were recruited from the Stop the Spread Ottawa study, a prospective cohort study investigating longitudinal antibody titers since October 2020 (25, 26).
Participants were excluded if they had been diagnosed with an IgG subclass deficiency without evidence of functional antibody deficiency and isolated IgA deficiency, had evidence of human immunodeficiency virus infection, had a positive COVID-19 infection by PCR or rapid antigen test (RAT) <12 weeks prior to vaccination, or were otherwise contraindicated from receiving COVID-19 vaccines.
Vaccination
All participants received vaccines in accordance with their local provincial vaccination guidance, which had varied recommended intervals between vaccine doses. In general, dose 2 was administered 4–12 weeks after dose 1, and dose 3 was administered 6–24 weeks after dose 2.
Sample collection
The study protocol was initially developed in early 2021 when two COVID-19 vaccine doses were recommended as a primary series. Thus, we collected blood at 0–28 days prior to administration of dose 1 (D1) as well as 0–14 days before dose 2 (D2), 4 ± 1 weeks after (D2 + 4wk), and 24 ± 4 weeks after (D2 + 24wk) dose 2. A three-dose primary series was recommended for IEIs as of September 2021 in Canada (27). The study protocol was therefore amended to include data collection before and after dose 3 for IEIs and controls. To minimize the frequency of blood draws and maintain participant retention, we omitted the study visit at D2 + 4wk but added 0–14 days before dose 3 (D3), as well as 4 ± 1 (D3 + 4wk) and 24 ± 4 (D3 + 24wk) weeks after dose 3. Participants who did not want to proceed with dose 3 despite recommendations were followed up until 48 ± 4 weeks after dose 2 (D2 + 48wk) for blood collection.
Plasma and peripheral blood mononuclear cell (PBMC) samples were isolated from participants’ blood and stored at each participating center prior to shipment to the University of Ottawa Serology and Diagnostics High Throughput Facility (plasma) for antibody assays and Dalhousie University (PBMC) for T-cell IFN-γ enzyme-linked immunosorbent spot (ELISpot) assay.
Immunogenicity
Plasma samples were assessed for levels of IgG, IgA, and IgM antibodies against severe acute respiratory syndrome coronavirus-2 (SARS-CoV-2) trimeric spike (S), receptor binding domain of spike (RBD), and nucleoprotein (N) by chemiluminescent ELISA (28). Antibody neutralization was assessed using surrogate neutralization ELISA on the full trimeric spike (wild-type and B.1.1.529-Omicron BA.1 subvariant, NRC Metrology and laboratory of Dr. Yves Durocher) (26). IgG serological titers were reported in WHO international units as binding antibody units (BAU)/mL. IgA and IgM titers were reported as concentrations compared to laboratory-calibrated standard curves (ng/μL). Neutralization data were reported as the inhibitory dilution at 50% inhibition (ID50) calculated from a four-parameter logarithmic regression applied to a 5-point serial dilution of samples.
Human IFN-γ ELISPOT set (BD Biosciences, Mississauga, ON, Canada) was used as per manufacturer instructions to determine the proportion of SARS-CoV-2-specific T cells. PBMCs were assayed in duplicate at 2 × 105/well after 18-hour stimulation with 2.5 µg/mL phytohemagglutinin-L (PHA; Life Technologies, Burlington, ON, Canada) as the positive control, an equivalent concentration of dimethyl sulfoxide (DMSO) (Sigma-Aldrich, St. Louis, MO, USA) as the negative control (0.32%), or 1 µg/mL peptide pools, consisting mainly of 15 mer sequences with 11 amino acids overlapping, derived from the complete protein-coding sequences of S-protein from wild-type, B.1.617.2-Delta (S-Delta) and B.1.1.529-Omicron (S-Omicron) variants, wild-type N, or control peptide pool. Spots were enumerated using a CTL-ImmunoSpot® S6 Micro Analyzer (CTL, Cleveland, OH, USA), and CMI response was reported as the proportion of SARS-CoV-2 specific T cells (IFN-γ+ T cells/106 PBMCs).
Vaccine safety and breakthrough COVID-19 infection
Vaccine safety questionnaires were completed by the participants 7 and 28 days after each dose and 24 weeks after their last dose. Details on medically attended adverse events (MAAEs) including onset, symptoms, type of medical attention sought, treatments, severity (29), and evaluation of vaccine relatedness were collected. Additionally, information on historical COVID-19 infections was collected at the baseline visit. Breakthrough COVID-19 infections confirmed by PCR or RAT were noted at each follow-up.
Analysis
Baseline demographics were summarized using descriptive analyses. Age, body mass index (BMI), and baseline laboratory parameters were summarized using means and standard deviations. Intervals in days between vaccine doses were reported as median with interquartile ranges (IQRs). The remainder of the demographic data were expressed as percentages. Primary analyses were conducted by comparing HI and CMI responses of IEIs with those of controls at each timepoint and 4 weeks after completing the primary series. Secondary analyses included the comparison of CMI and HI responses based on the type of IEIs. HI responses were stratified and analyzed by use of immunoglobulin replacement therapy (IGRT) and previous COVID-19 infection confirmed by PCR or RAT. Hybrid immunity was defined as having positive anti-N IgG and/or previous SARS-CoV-2 infection. Confidence intervals of geometric mean values of serological titers and IFN-γ+ T cells/106 PBMCs were calculated. The independent t-test was used to compare geometric means at each timepoint between IEIs and controls. The proportion (Fisher’s exact test) of COVID-19 infections post-vaccination was compared between participant groups and IEI subgroups. Geometric means of HI and CMI among IEI subgroups and controls were compared using one-way ANOVA. The Mann–Whitney U-test was used to compare medians between IEIs and controls. The Kruskal–Wallis test was used to compare medians between IEI subgroups and controls. All tests were two-sided with statistical significance set at α = 0.05. Analyses were performed using SAS version 9.4 (SAS Institute Inc., Cary, NC, USA), and graphs were generated using Prism version 10.2 (GraphPad Software, LLC, Boston, MA, USA).
Results
A total of 149 adult participants with IEIs (99 in the PAD subgroup, 35 in the CID subgroup, and 15 in the III subgroup), 37 controls, and 386 controls from the Stop the Spread Ottawa (SSO) study were recruited (Supplementary Material). Participant demographic information is summarized in Table 1. The average (SD) age of adult participants with IEIs, our controls, and SSO controls were 47.7 (15.1), 52.5 (13.2), and 47.3 (13.9) years, respectively; 68.1% and 56.5% of our and the SSO controls, respectively, were female, and 57.7% of participants with IEIs were female. The most common IEI was common variable immunodeficiency (CVID) (n = 77). Among the remainder of the participants with PAD, five had agammaglobulinemia, four had hyper-IgM syndrome, five had specific antibody deficiency, one had hypogammaglobulinemia, and seven had other types of PAD. Among CID participants, one had severe combined immunodeficiency (SCID) status post-hematopoietic stem cell transplant, three had CID with syndromic features, two had purine nucleoside phosphorylase (PNP) deficiency, three had 22q11 deletion syndrome, and 25 had other types of CID. Finally, among participants in the III subgroup, two had a diagnosis of chronic granulomatous disease or phagocyte defect, six had complement deficiency, three had autoinflammatory disorders, and four had diseases of immune dysregulation (Table 1). A total of 107 of the participants with IEIs were treated with IGRT.
At the time of study enrolment, 20 IEI participants and eight controls were unvaccinated. Of the 129 vaccinated IEI participants, 60 had received three doses, 52 had received two doses, and 16 had received one dose. Out of the 29 vaccinated controls, 12 had received three doses, 15 had received two doses, and two had received one dose (Table 1).
Serological response
At D2 + 4wk, anti-RBD and anti-S IgG titers were significantly lower in participants with IEIs compared to controls {geometric mean (95% CI), 265.5 [95.31–739.7] vs. 4,221.4 [3,436.4–5,185.7] BAU/mL, p < 0.0001; 198.7 [66.43–594.3] vs. 3,830.1 [3,010.6–4,872.7] BAU/mL, p < 0.001, respectively}. However, at 6 months after dose 2 (D2 + 24wk), there was no longer a statistically significant difference in anti-RBD and anti-S IgG levels between IEIs and controls. At D3 + 4wk, anti-RBD and anti-S IgG titers were significantly lower in IEIs compared to controls (834.2 [499.9–1,392.1 vs. 6,961.8 [4,936.2–9,818.7] BAU/mL, p < 0.001; 669.8 [393.9–1,138.8] vs. 5,301.7 [3,402.6–8,260.7] BAU/mL, p < 0.0001, respectively). Anti-RBD and anti-S IgG titers at D3 + 24wk were also significantly lower in IEIs than in controls (852 [428–1,696.1] vs. 3,410.7 [2,664.9–4,365.2], p = 0.0004; 763.6 [402.7–1,447.6] vs. 3,273.7 [2,584.9–4,146], p < 0.0001, respectively).
Comparison of serological responses after completion of the primary series showed lower anti-RBD and anti-S IgG titers in IEIs than controls at 4 weeks but not at 24 weeks (Figures 1A, B, Supplementary Material). Anti-RBD and anti-S IgA titers at D2, D2 + 4wk, D2 + 24wk, D3 + 4wk, and D3 + 24wk were significantly lower in IEIs compared to controls (Figures 1C, D). Anti-RBD and anti-S IgM titers at D2, D2 + 4wk, D3 + 4wk, and D3 + 24wk were also lower in IEIs than in controls (Figures 1E, F).
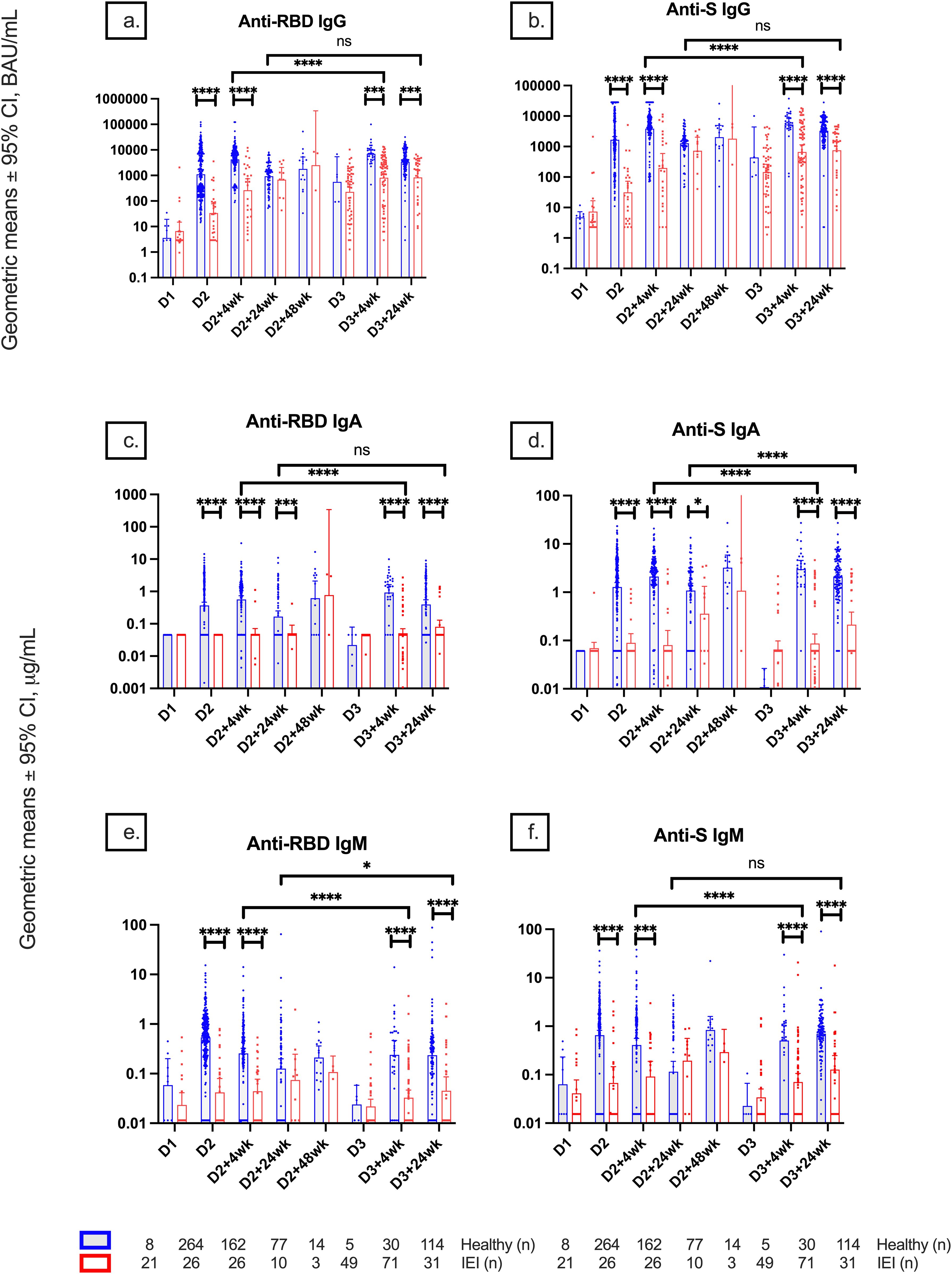
Figure 1. Geometric mean IgG, IgA, and IgM serologies among healthy participants and participants with inborn errors of immunity (IEIs). Geometric mean ± 95% confidence interval of (A) anti-RBD IgG, (B) anti-S IgG, (C) anti-RBD IgA, (D) anti-S IgA, (E) anti-RBD IgM, and (F) anti-S IgM titers among healthy participants and participants with IEIs at different timepoints pre- and post-COVID-19 vaccination up to 24 weeks after dose 3. Number (n) of healthy and primary immunodeficient participants with data included per timepoint are indicated. * indicates p < 0.05, *** indicates p < 0.001, **** indicates p < 0.0001, and ns indicates not significant. Error bars indicate the 95% CI. D1, before dose 1; D2, before dose 2; D2 + 4wk, 4 weeks after dose 2; D2 + 24wk, 24 weeks after dose 2; D2 + 48wk, 48 weeks after dose 2; D3, before dose 3; D3 + 4wk, 4 weeks after dose 3; D3 + 24wk, 24 weeks after dose 3; CI, confidence interval; anti-RBD, anti-receptor binding domain; anti-S, anti-spike protein; anti-N, anti-nucleocapsid; ns, not significant.
Evidence of natural infection and hybrid immunity
SARS-CoV-2 infections before vaccine doses 2 and 3 and within 6 months of receiving dose 3 were reported in 15/37 (40.5%), 5/37 (13.5%), and 11/37 (29.7%) of controls and 1/149 (0.7%), 9/149 (6.0%), and 32/149 (21.5%) of IEIs. Anti-RBD and anti-S IgG titers were only higher in controls with hybrid immunity compared to controls without hybrid immunity at D2, D2 + 24wk, and D3 + 24wk, while no difference was found in IEIs with or without hybrid immunity at any timepoint (Figures 2A, B). Anti-N IgG titers of controls increased at an earlier timepoint, whereas the titers increased at a later timepoint in IEI participants, consistent with the report of SARS-CoV-2 infection (Figure 2C). Further, anti-N IgG titers were higher in both healthy and IEI participants with hybrid immunity than those without hybrid immunity (Figure 2C). Interestingly, the higher anti-N IgG titers at D3 + 24wk in IEIs were driven by IEI participants on IGRT (12.9 [9.1–18.28] vs. 3.76 [1.79–7.89], p = 0.0008) (Figure 3).
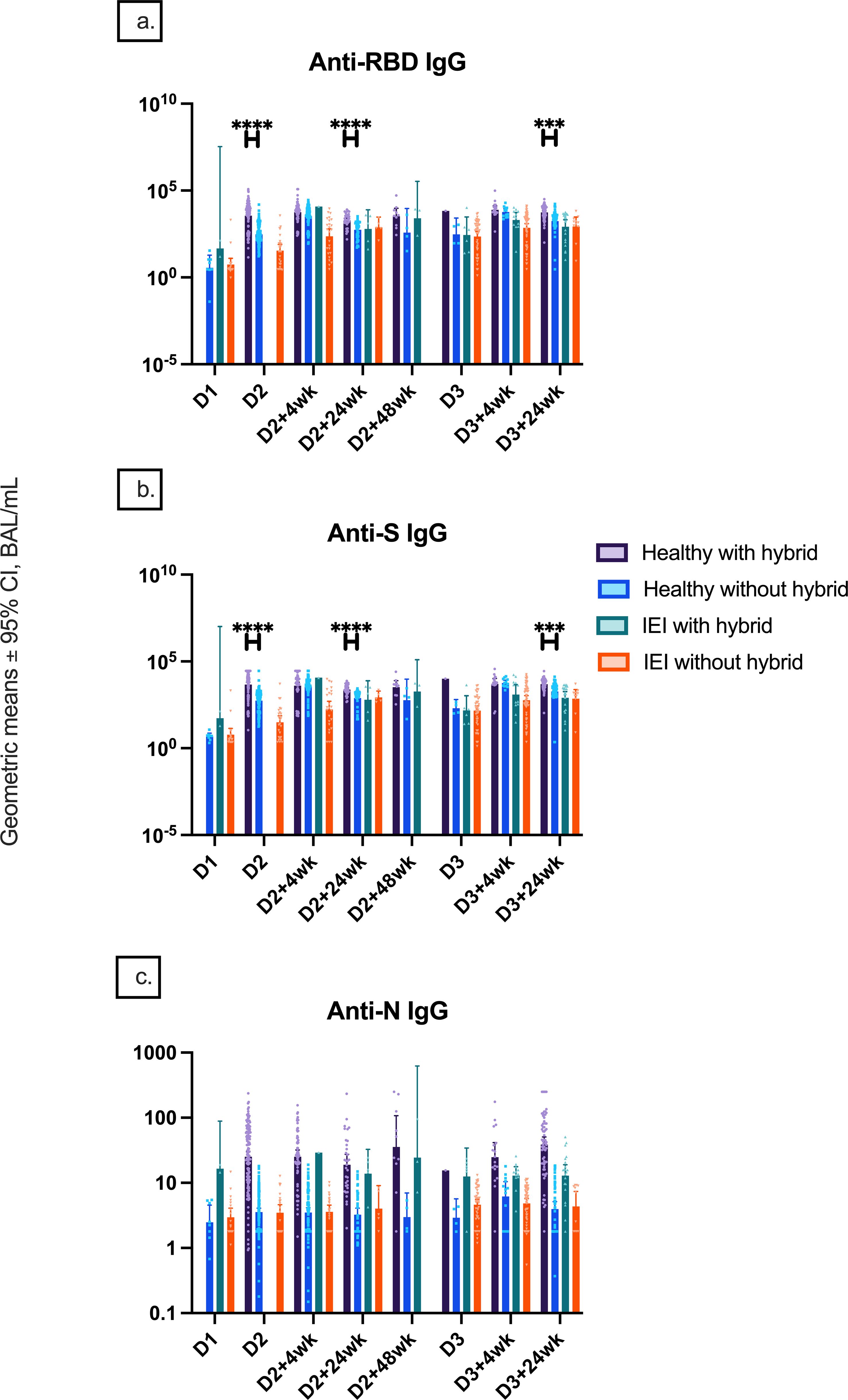
Figure 2. Natural and hybrid immunity. Geometric mean ± 95% confidence interval of (A) anti-RBD IgG responses by hybrid immunity status and group at different timepoints pre- and post-COVID-19 vaccination up to 24 weeks after dose 3, (B) anti-S IgG responses by hybrid immunity status and group at these different timepoints, and (C) anti-N IgG serology by hybrid immunity status and group at these different timepoints. *** indicates p < 0.001, **** indicates p < 0.0001, and D1, before dose 1; D2, before dose 2; D2 + 4wk, 4 weeks after dose 2; D2 + 24wk, 24 weeks after dose 2; D2 + 48wk, 48 weeks after dose 2; D3, before dose 3; D3 + 4wk, 4 weeks after dose 3; D3 + 24wk, 24 weeks after dose 3; anti-RBD, anti-receptor binding domain; anti-S, anti-spike protein; anti-N, anti-nucleocapsid; IEIs, inborn errors of immunity; CI, confidence interval.
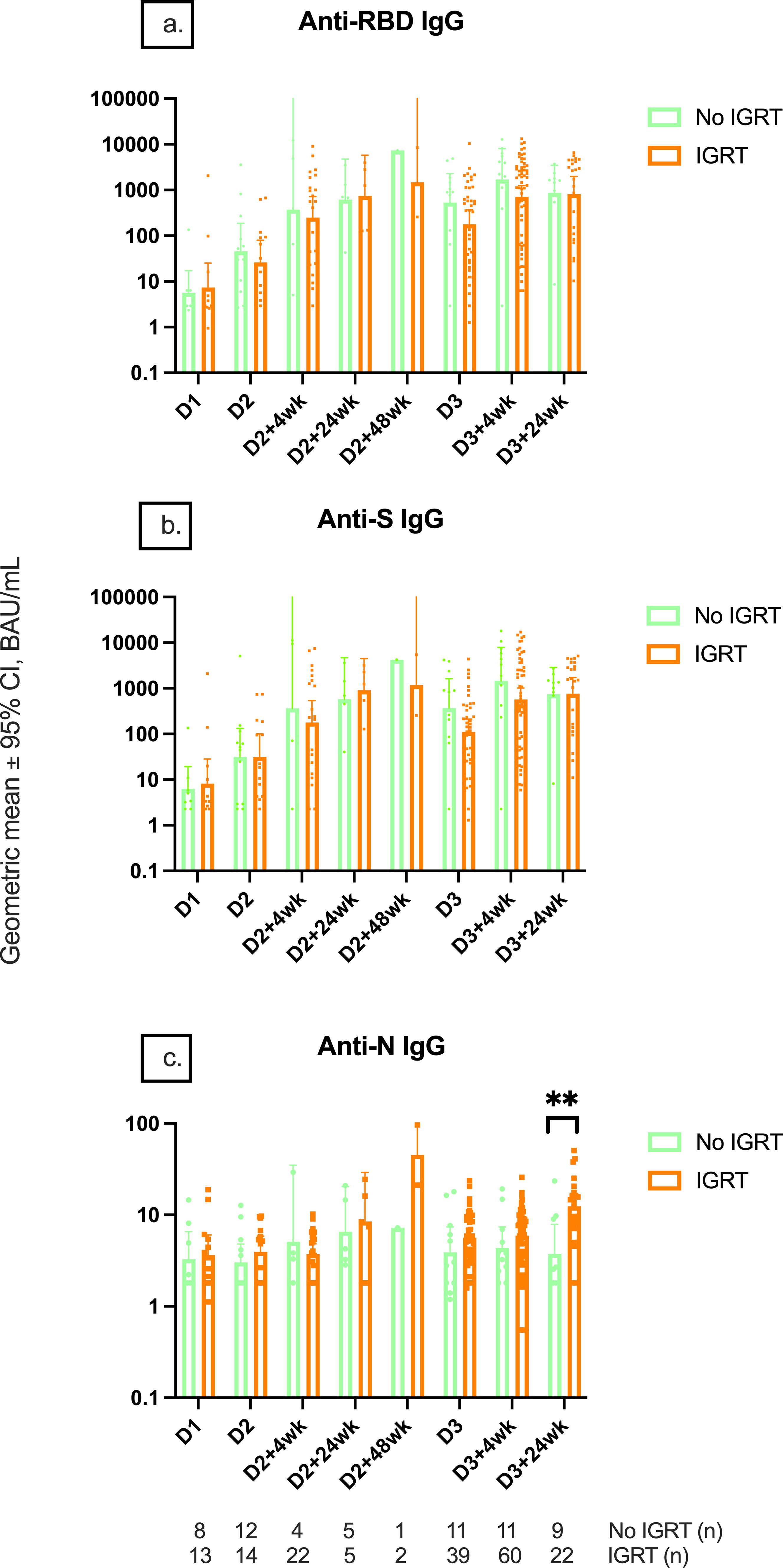
Figure 3. Serological responses among IEI participants receiving IGRT or not receiving IGRT. Geometric mean IgG serology of (A) anti-RBD, (B) anti-S, and (C) anti-N IgG titers between IEI participants receiving and not receiving immunoglobulin replacement therapy at different timepoints pre- and post-COVID-19 vaccination up to 24 weeks after dose 3. Number (n) immunodeficient participants with data included per timepoint are indicated. ** indicates p < 0.01. Error bars indicate the 95% CI. D1, before dose 1; D2, before dose 2; D2 + 4wk, 4 weeks after dose 2; D2 + 24wk, 24 weeks after dose 2; D2 + 48wk, 48 weeks after dose 2; D3, before dose 3; D3 + 4wk, 4 weeks after dose 3; D3 + 24wk, 24 weeks after dose 3; IGRT, immunoglobulin replacement therapy; CI, confidence interval; anti-RBD, anti-receptor binding domain; anti-S, anti-spike protein; anti-N, anti-nucleocapsid; IEI, inborn error of immunity.
Impact of IGRT on serological responses
To evaluate if measured serological responses in IEIs were impacted by IGRT, we analyzed serology data based on IGRT (Figure 3). IGRT did not impact anti-RBD and anti-S IgG titers at any timepoint. This was confirmed by the analysis of anti-S IgG levels in IEI participants in the PAD subgroup (i.e., with predominant B-cell deficiency), as they were the most likely to have the poorest HI response to the vaccine (Supplementary Material). In fact, PAD participants who were on IGRT tended to have lower anti-S IgG (not statistically significant) and anti-S IgA than those not on IGRT.
Neutralization antibody response
At D2 + 4wk, D3 + 4wk, and D3 + 24wk, the median (IQR) ID50 against the ancestral strain was significantly lower in IEIs compared to controls (6.55 [1.00, 22.9] vs. 73.4 [48.6, 155.8], p < 0.0001; 20.34 [3.9, 71] vs. 99.4 [57.5, 150.6], p = 0.0032; 14.7 [1, 68.9] vs. 104.9 [48.6, 426.6], p = 0.0005, respectively) (Figure 4). Similar results were seen against Omicron BA.1 variant, where IEIs had a significantly lower ID50 compared to controls at D2 + 4wk, D3 + 4wk, and D3 + 24wk (1 [0.5, 1.8] vs. 13.6 [6.6, 27.7], p < 0.0001; 2.1 [1, 10.3] vs. 24.3 [12.3, 27.6], p = 0.0021; 1 [1, 6.6] vs. 21.5 [4, 62.7], p = 0.0013, respectively). There were reduced ID50 titers against the Omicron BA.1 variant in both groups as compared to those against the ancestral strain.
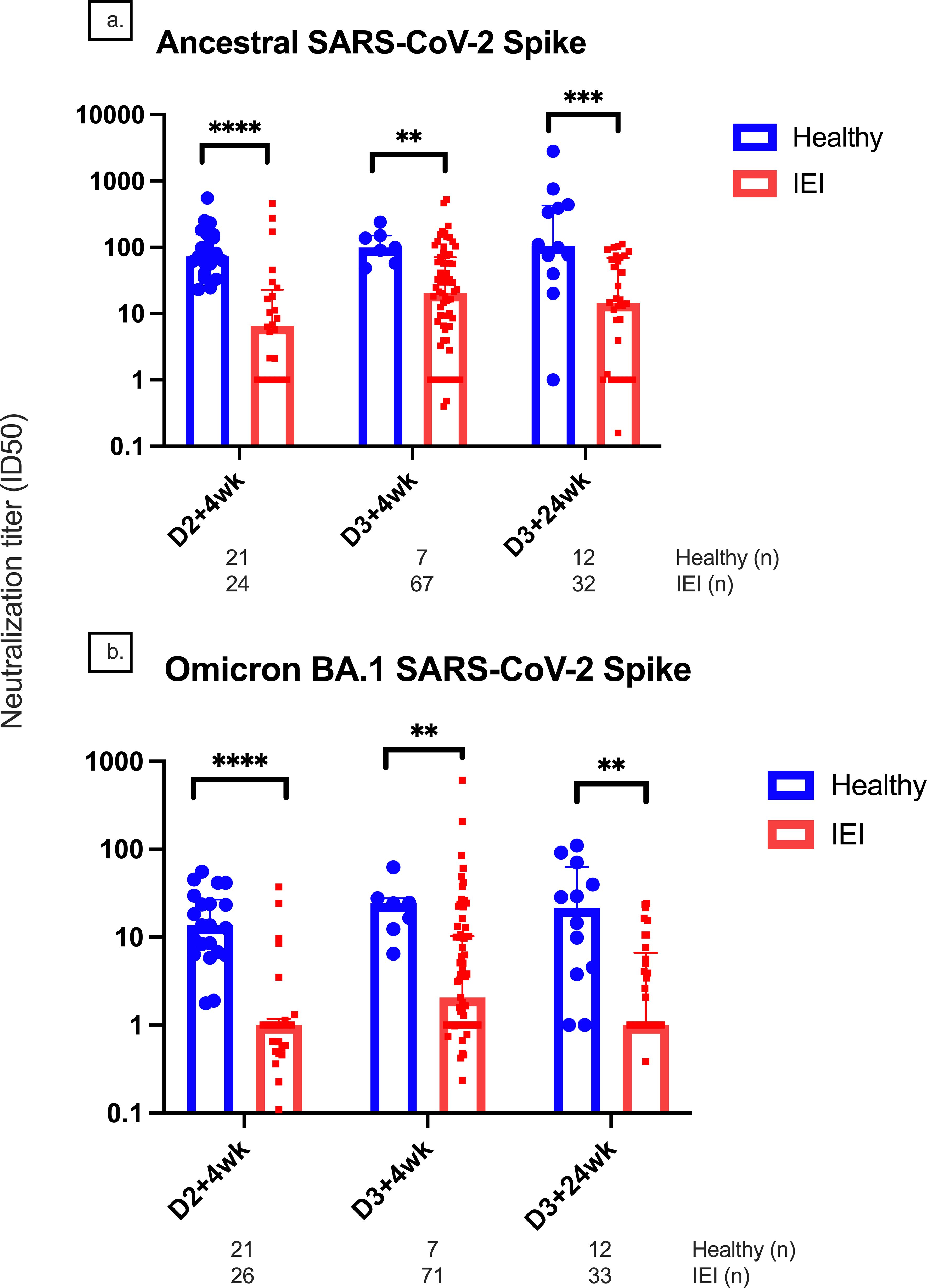
Figure 4. Neutralization titers for the ancestral and Omicron BA.1 variants. Comparison of neutralization titers at 4 weeks after doses 2 and 3 between healthy and immunodeficient participants for the (A) ancestral and (B) Omicron BA.1 variants. Number (n) of healthy and IEI participant data included per timepoint are indicated. ** indicates p < 0.01, *** indicates p < 0.001, **** indicates p < 0.0001. The error bars indicate the standard deviation. D1, before dose 1; D2, before dose 2; D2 + 4wk, 4 weeks after dose 2; D2 + 24wk, 24 weeks after dose 2; D2 + 48wk, 48 weeks after dose 2; D3, before dose 3; D3 + 4wk, 4 weeks after dose 3; D3 + 24wk, 24 weeks after dose 3; IGRT, immunoglobulin replacement therapy; CI, confidence interval; IQR, interquartile range; anti-RBD, anti-receptor binding domain; anti-S, anti-spike protein; anti-N, anti-nucleocapsid.
Cell-mediated vaccine response
The T-cell responses measured by S-specific IFN-γ+ T cells/106 PBMCs at D2 + 4wk, D3 + 4wk, and D3 + 24wk were significantly lower in IEIs compared to controls (23.6 [95% CI, 8.8–63.1] vs. 163.0 [77.9–340.9], p = 0.002; 21.65 [13.50–34.70] vs. 109.00 [29.85–398.02], p = 0.03; 13.7 [6.8–27.4] vs. 55.9 [25.0–124.6], p = 0.02) (Figure 5A).
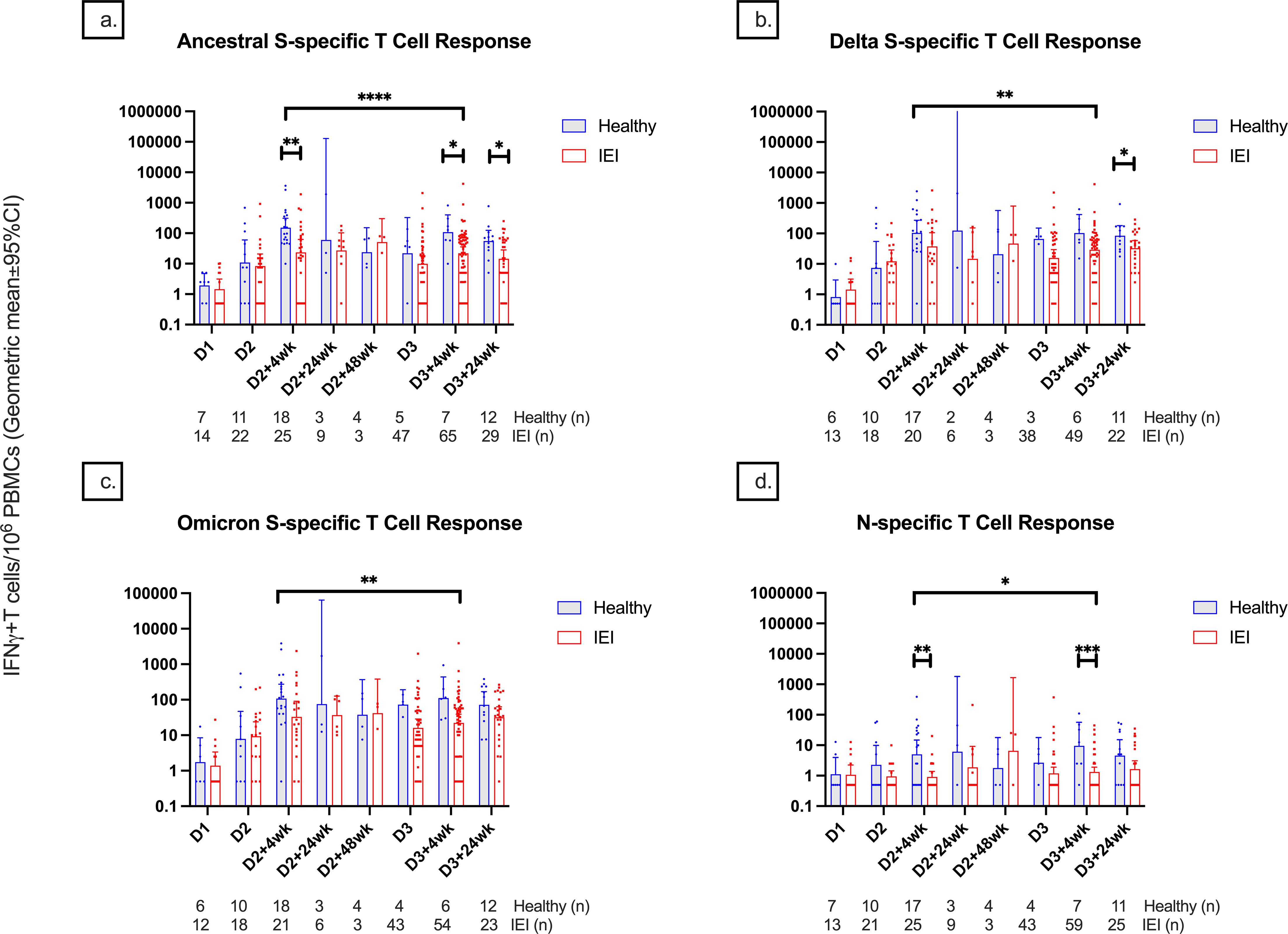
Figure 5. T-cell responses among healthy controls and participants with inborn errors of immunity at different timepoints pre- and post-COVID-19 vaccination up until 24 weeks after dose 3. T-cell responses against the (A) spike protein of the ancestral variant, (B) spike protein of the delta variant, (C) spike protein of the B.1.1.529 Omicron variant, and (D) nucleocapsid protein are depicted and measured as IFN-γ positive T cells per 1 million PBMCs. Number (n) of healthy and immunodeficient participant data included per timepoint are indicated. * indicates p < 0.05, ** indicates p < 0.01, *** indicates p < 0.001, **** indicates p < 0.0001. The error bars indicate the 95% confidence interval. S, spike; N, nucleocapsid; IFN-γ, interferon gamma cytokine; PBMCs, peripheral blood mononuclear cells; D1, before dose 1; D2, before dose 2; D2 + 4wk, 4 weeks after dose 2; D2 + 24wk, 24 weeks after dose 2; D2 + 48wk, 48 weeks after dose 2; D3, before dose 3; D3 + 4wk, 4 weeks after dose 3; D3 + 24wk, 24 weeks after dose 3.
There was no significant difference in S-specific T-cell responses against S-Delta and B.1.1.529-S-Omicron responses between IEIs and controls at any timepoint except at D3 + 24wk, where there was a significantly lower response in IEIs compared to controls (31.5 [16.8–57.5] vs. 84.3 [39.3–180.8], p = 0.048) (Figures 5B, C).
IEIs had lower T-cell response than controls at 4 weeks but not at 6 months after completing their respective primary series (p < 0.0001 for S-ancestral, p = 0.007 for S-Delta, and p = 0.03 for B.1.1.529-S-Omicron). Similar to the serological response, T-cell response to natural infection was more evident in controls than in IEI at D2 + 4wk and D3 + 4wk (Figure 5D), corresponding with their history of prior SARS-CoV-2 infections.
Responses by IEI subgroups
Participants in the PAD subgroup mounted weaker anti-RBD, anti-S, and anti-N responses at D2, D2 + 4wk, D3 + 4wk, and D3 + 24wk compared to controls (Figure 6). Similar differences were also seen in the CID subgroup (Figure 6). Although there was no statistically significant difference in anti-S IgG between the PAD and CID subgroups, the PAD subgroup had lower median anti-S IgG than the CID and III subgroups at D3–4 (533.1 [295.5, 2,512.0] vs. 3,105.7 [52.0, 7,338.8] vs. 5,866.3 [4,377.1, 10,777.2], respectively) (Figures 6, 7). Conversely, S-specific T-cell response was higher although not statistically significant in the PAD subgroup than in the CID subgroup at D3 + 4wk (46.3 [12.5,85] vs. 5 [2.5, 50], p = 0.07). HI comparisons were performed; however, CMI comparisons with the III subgroup were not performed due to a small sample size (Figure 7).
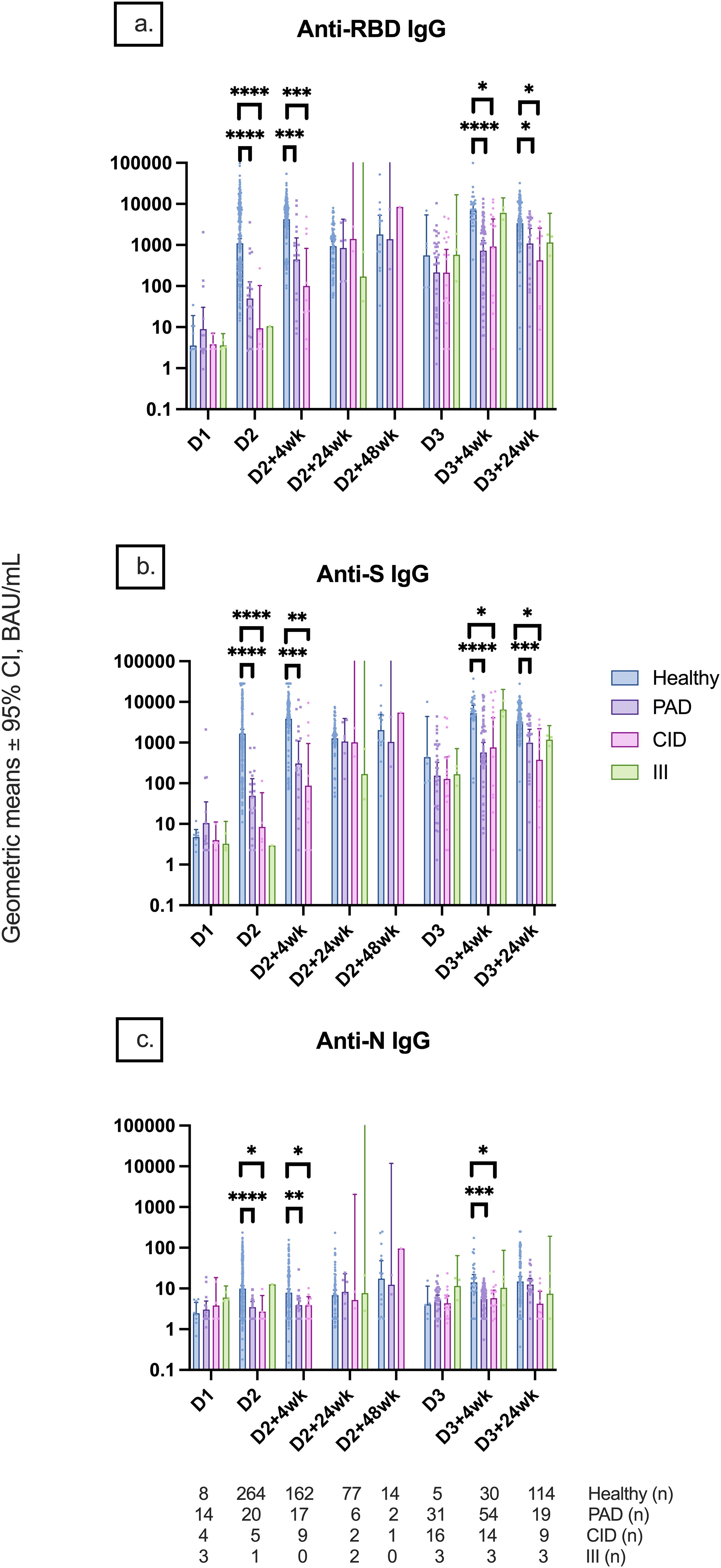
Figure 6. Geometric mean IgG serology levels by participant subgroups. Comparison of (A) anti-RBD IgG, (B) anti-S IgG, and (C) anti-N IgG titers among participant subgroups (healthy, PAD subgroup, CID subgroup, and III subgroup) at different timepoints pre- and post-COVID-19 vaccination up to 24 weeks after dose 3. Statistical analysis was not performed for the III subgroup due to the small sample size. Number (n) of healthy and immunodeficient subgroup participants with data included per timepoint are indicated. * indicates p < 0.05, ** indicates p < 0.01, *** indicates p < 0.001, **** indicates p < 0.0001. Error bars indicate the 95% CI. D1, before dose 1; D2, before dose 2; D2 + 4wk, 4 weeks after dose 2; D2 + 24wk, 24 weeks after dose 2; D2 + 48wk, 48 weeks after dose 2; D3, before dose 3; D3 + 4wk, 4 weeks after dose 3; D3 + 24wk, 24 weeks after dose 3; CI, confidence interval; PAD, primary antibody deficiency; CID, combined immunodeficiency; III, intrinsic innate immune defect.
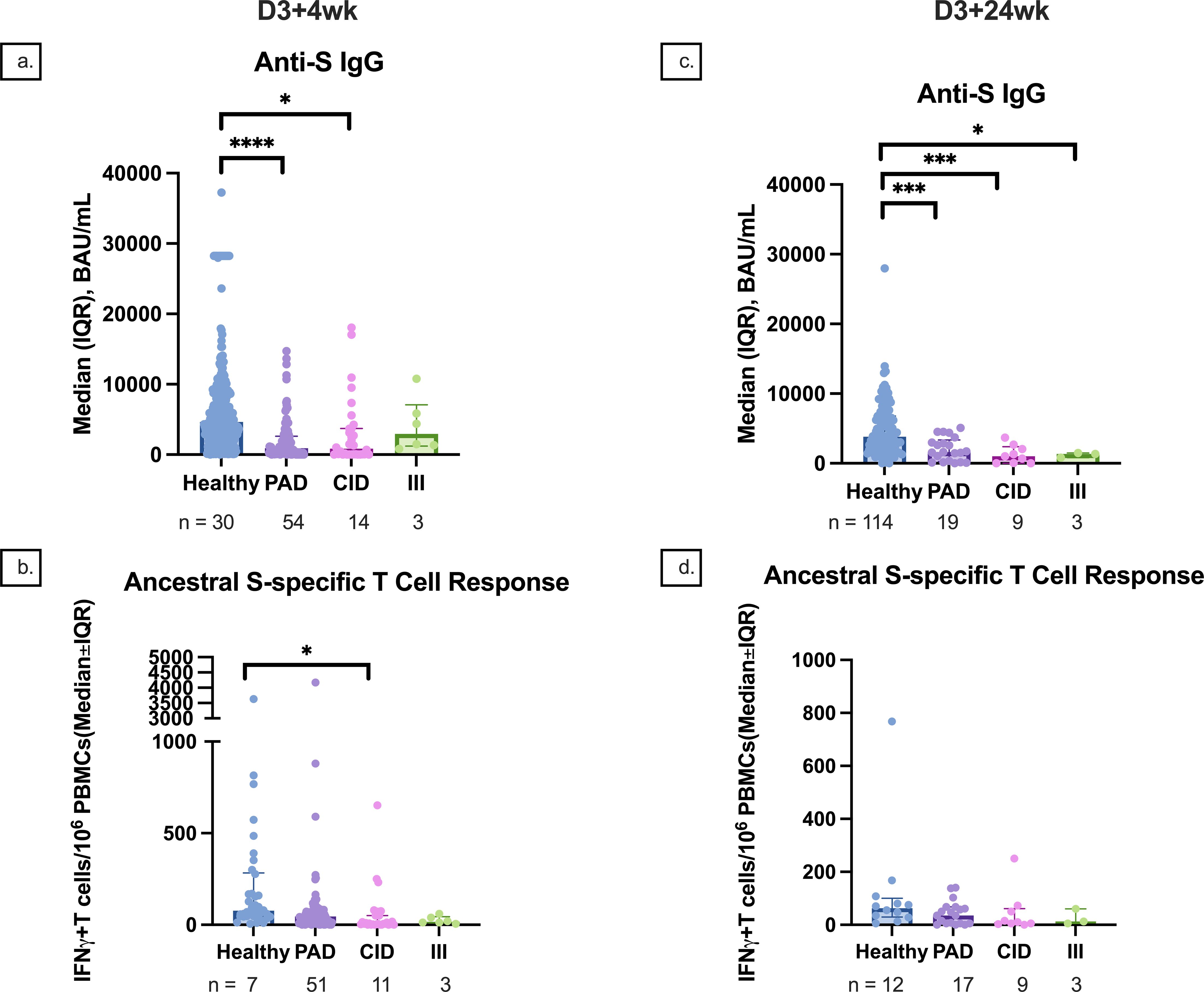
Figure 7. Median anti-S IgG and T-cell responses by participant subgroup at 4 and 24 weeks after dose 3. (A) Median anti-S IgG responses at 4 weeks after dose 3 compared between healthy, PAD, CID, and III subgroup participants. (B) Median S-specific T-cell responses against the ancestral strain at 4 weeks after dose 3 compared between healthy, PAD, CID, and III subgroup participants. (C) Median anti-S IgG responses at 24 weeks after dose 3 compared between healthy, PAD, CID, and III subgroup participants. (D) Median S-specific T-cell responses against the ancestral strain at 24 weeks after dose 3 compared between healthy, PAD, CID, and III subgroup participants. * indicates p < 0.05, *** indicates p < 0.001, **** indicates p < 0.0001. Error bars indicate the 95% CI. D3 + 4wk, 4 weeks after dose 3; D3 + 24wk, 24 weeks after dose 3; IQR, interquartile range; S, spike protein; PAD, primary antibody deficiency; CID, combined immunodeficiency; III, intrinsic innate immune defect.
Correlation between HI and CMI
The degree of correlation of markers of HI and CMI against S-protein differed between healthy and IEI participants (Supplementary Material, Figure 8). In general, there was a strong correlation between anti-S IgG and ID50 (r = 0.83, p < 0.0001 for controls and 0.86, p < 0.0001 for IEI participants), a moderate correlation between serologies and cellular responses (r = 0.564, p < 0.0001 for healthy and 0.373, p < 0.0001 for IEI), but no correlation between ID50 and cellular responses (r = −0.013, p = 0.94 for controls and 0.154, p = 0.11 for IEI participants). At specific timepoints (Figure 8), there was also a strong correlation between anti-S IgG and ID50 at D3 + 4wk (r = 0.750, p = 0.067 for controls and 0.88, p < 0.0001 for IEI participants) and D3 + 24wk (r = 0.867, p = 0.0005 for controls and 0.729, p < 0.0001 for IEI participants). There was weak to no correlation between serologies and cellular responses at D3 + 4wk (r = 0.143, p = 0.78 in controls and 0.149, p = 0.24 in IEI participants) and at D3 + 24wk (r = 0.112, p = 0.73 in controls and −0.023, p = 0.91 in IEI participants). Similarly, there was weak to no correlation between ID50 and cellular responses at D3 + 4wk (r = −0.013, p = 0.94 in controls and 0.153, p = 0.11 in IEI participants) and at D3 + 24wk (r = 0.136, p = 0.69 in controls and 0.007, p = 0.97 in IEI participants).
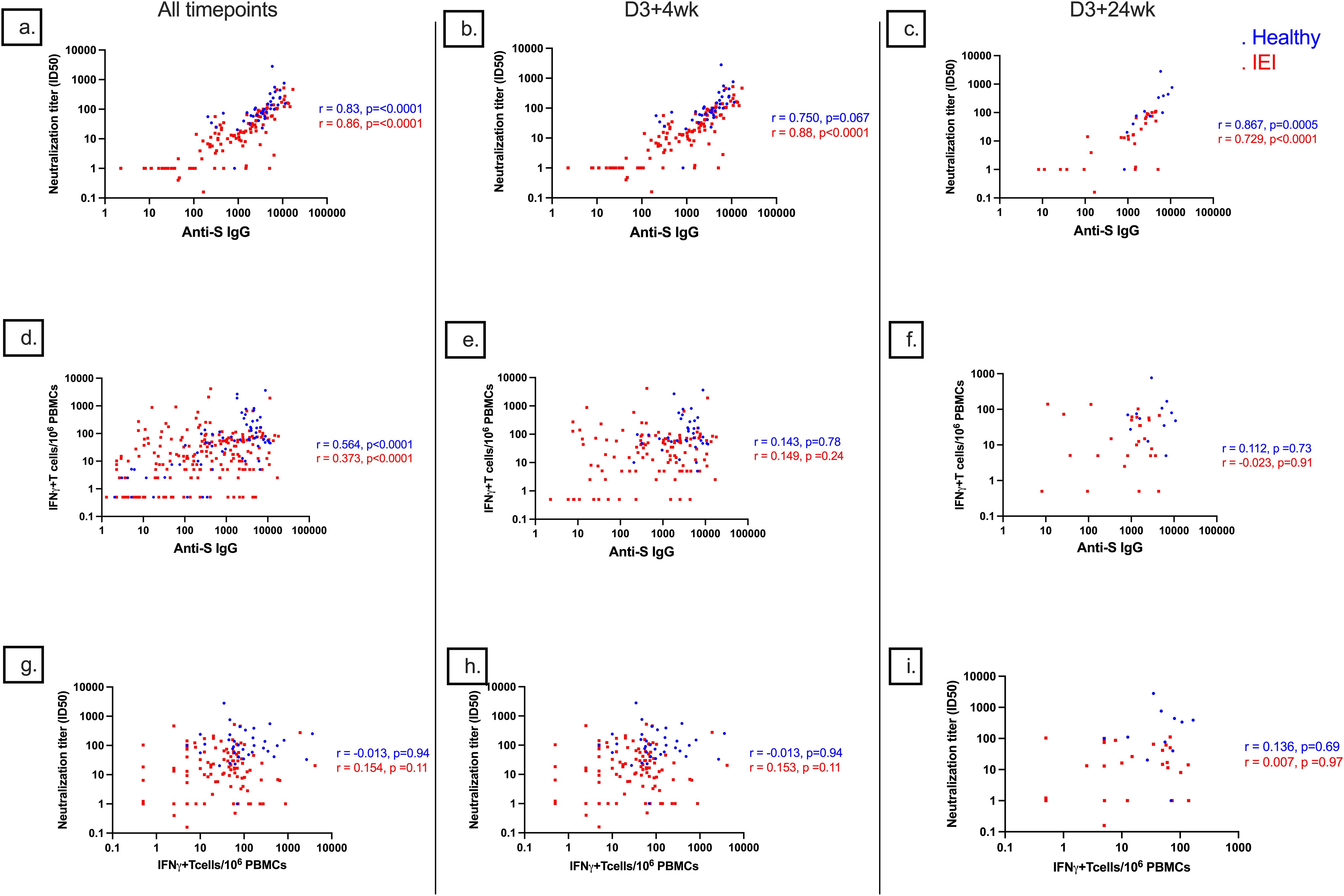
Figure 8. Correlations of anti-S IgG, ID50, and S-specific T-cell responses. Correlations of (A) anti-S IgG and ID50 with combined data from all timepoints, (B) anti-S IgG and ID50 at D3 + 4wk, (C) anti-S IgG and ID50 at D3 + 24wk, (D) anti-S IgG and S-specific T-cell responses with combined data from all timepoints, (E) anti-S IgG and S-specific T-cell responses at D3 + 4wk, (F) anti-S IgG and S-specific T-cell responses at D3 + 24wk, (G) S-specific T-cell responses and ID50 with combined data from all timepoints, (H) S-specific T-cell responses and ID50 at D3 + 4wk, and (I) S-specific T-cell responses and ID50 at D3 + 24wk. D3 + 4wk, 4 weeks after dose 3; D3 + 24wk, 24 weeks after dose 3; S, spike; IQR, interquartile range; S, spike; IFN-γ, interferon gamma; PBMC, peripheral blood mononuclear cell.
Vaccine safety
Among participants enrolled between D1 and D3 + 24wk, there were 21 MAAEs in 17 (11.4%) IEI participants and only one MAAE in controls (Table 2). For IEI participants, over 90% of these events were considered moderate severity. Of all MAAEs, 80.95% were determined to be unrelated to vaccination, while the remaining events were identified as probably (4.8%) or possibly (9.5%) related to vaccination. The average onset of MAAEs in IEI participants was 88.3 days from a vaccination, all of which occurred between D3 and D3 + 24wk. The MAAE in the controls was a spontaneous abortion reported 2 weeks after the first vaccination at approximately 7 weeks of gestation and determined to be possibly related to vaccination.
SARS-CoV-2 infection
Overall, including infection history from before and after study enrolment, we found that 24 (64.9%) of controls reported at least one SARS-CoV-2 infection with a total of 31 infections, and 15/31 occurred when the participant was vaccine-naive. However, 40 (26.9%) of IEI participants had at least one SARS-CoV-2 infection. The total number of reported SARS-CoV-2 infections was 42, and only one occurred before any vaccination (Supplementary Material). Nine (21.43%) infections were reported between doses 2 and 3 for IEIs, while 5 (16.13%) were reported for healthy controls. Most infections reported by IEI participants occurred after dose 3 (32, 76.19%) and after January 2022 (35, 82.33%).
During the study, 8.7% of IEI participants and 2.7% of controls had medically attended COVID-19 infections (p = 0.31) (Table 2). No severe infections were reported during the study, but there was one severe infection requiring 8 days of hospitalization reported from one IEI patient prior to study enrolment.
Participants with breakthrough infections had lower median anti-S IgG titers, ID50 titers, and S-specific T-cell responses prior to infection than participants who did not have breakthrough infections [301.7 (25.5, 1,445.0) vs. 2,367 (656.7, 6,507.0) BAU/mL, p < 0.0001; 14.0 (1, 56.6) vs. 34.7 (8.5, 98.3), p = 0.0.4; 16.25 (2.5, 56.6) vs. 35 (5.0, 75.0), p = 0.03, respectively] (Figure 9).
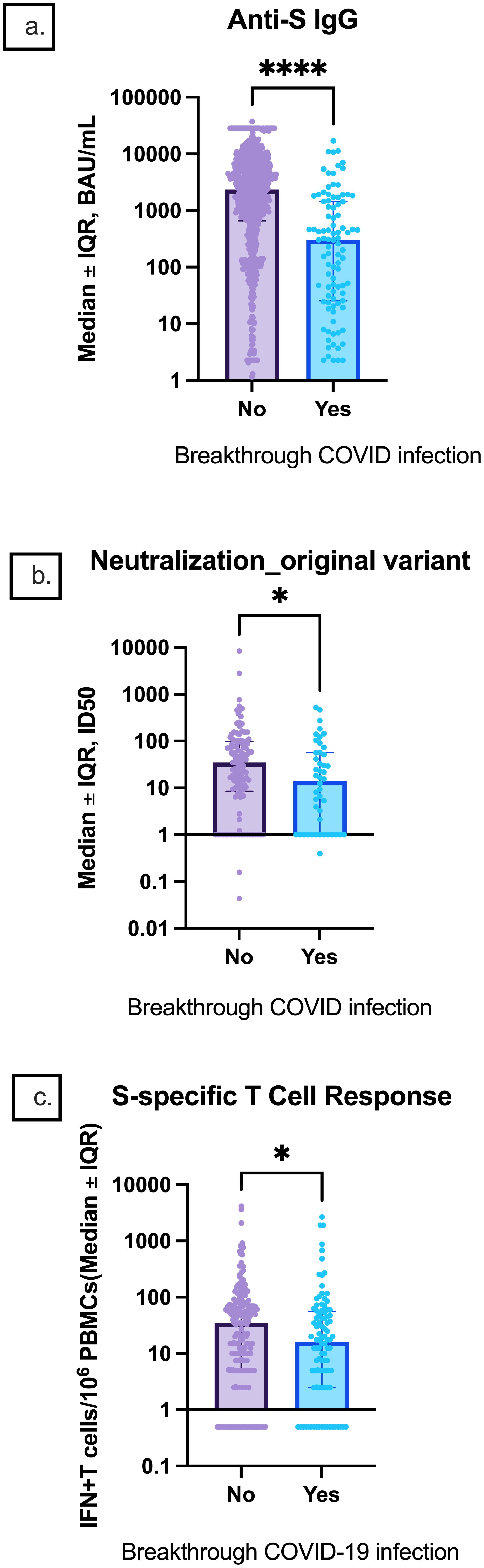
Figure 9. Comparison of pre-infection median anti-S IgG, neutralization titers, and S-specific T-cell responses between participants with and without breakthrough infection. Median (A) anti-S IgG, (B) original strain ID50, and (C) S-specific T-cell responses are depicted. Error bars indicate the IQR. IQR, interquartile range; S, spike; IFN-γ, interferon gamma; PBMC, peripheral blood mononuclear cell. * indicates p < 0.05, **** p < 0.0001.
Discussion
In this study, we compared HI and CMI responses to COVID-19 vaccines in participants with IEIs and controls. Consistent with previous studies, HI responses at D2 + 4wk and D3 + 4wk were significantly lower in participants with IEIs compared to controls (30, 31). Nevertheless, antibodies produced by IEIs were functional. CMI responses in IEI patients remain relatively understudied compared to HI. We observed that IEI patients could mount T-cell response to spike, although lower than that of controls after doses 2 and 3 (32). Reassuringly, both antibody and T-cell responses were durable and remained at comparable levels at 6 months after dose 3 to those at 4 weeks after dose 3 in IEI patients, whereas these responses waned moderately over time in controls (Supplementary Material). Similarly, anti-S IgG responses were examined in a study by Zendt et al. and were also found to persist from 4 weeks to 6 months after dose 3 (30). In our study, given that the response waned over time in controls but persisted in IEIs, this resulted in no significant difference in serological responses between the two groups at 24 weeks after their respective primary series.
Due to rapid changes in circulating viral strains, we also examined the neutralizing antibody titer against the BA.1 Omicron variant and T-cell response against B.1.1.529-Omicron-spike. We found that antibodies generated by ancestral COVID-19 vaccines had reduced neutralizing capacity to the Omicron variant compared to the ancestral strain, which is consistent with other studies (30, 33). For instance, Zendt et al. found that their participants with immunodeficiency had significantly lower anti-S IgG and as well as similar but lower ACE2 inhibition against the Omicron BA.1 variant compared to other variants (30). However, we did observe a relatively stable T-cell response to B.1.1.529-S-Omicron. In addition to IgG response, IgA and IgM responses to vaccines were also lower in IEI participants than in controls. Anti-S IgG correlated very well with neutralizing antibody titer, particularly in IEI patients, and there was a moderate correlation between anti-S IgG and T-cell response in both groups. However, T-cell response did not correlate well with neutralizing antibody titers.
Despite severe COVID-19 infections being a concern in the IEI community, our study reported only one severe COVID-19 infection that occurred before the completion of the primary series. Durable T-cell response may have protected IEI patients from severe outcomes (34–37). As expected, we found that low anti-S IgG titers, ID50, and S-specific T cells were associated with breakthrough infections. Most SARS-CoV-2 infections occurred after December 2021 when the Omicron variant dominated. This temporal increase in post-vaccination infection was also noted in the Chen et al. real-world assessment of immunogenicity in immunocompromised individuals following SARS-CoV-2 vaccination, where they also attributed the increase in cases at this time to the emergence of the Omicron variant (33).
Many studies have reported that participants with hybrid immunity (i.e., a history of SARS-CoV-2 vaccination and prior SARS-CoV-2 infection) mount stronger HI responses compared to vaccine-induced immunity (without prior history of infection) or natural immunity alone (38–41). These findings are consistent with our study of controls; however, we did not observe this pattern in IEI participants. There was no difference in HI responses in IEI participants with hybrid immunity compared to IEI participants without hybrid immunity at any timepoint. This evidence suggests that the presence of impaired immune response in IEIs was not only to vaccination but also to infection. We also speculated that this lack of difference in HI responses between IEIs with and without hybrid immunity could be due to IGRT. However, we found that IG products started to contain anti-S IgG only in late 2021 or early 2022 based on our X-linked agammaglobulinemia (XLA) patients’ data who should not have any antibody response to vaccines (42–44) yet had very high anti-S IgG in late 2021 and early 2022 (Supplementary Material). It is known that manufacturing IG products from plasma donation can take up to 1.5 years (45, 46). As such, it is very likely that IG products administered to patients in our study did not contain anti-S IgG until 1–2 years after the start of COVID-19 vaccine campaigns. In addition, we did not observe a difference between anti-S IgG in IEI participants on IGRT compared to IEI participants not on IGRT. This is also consistent with other studies where there was no significant difference in anti-S IgG concentrations between patients on IGRT and those not on IGRT at all timepoints (30). Thus, IGRT did not explain the lack of difference in HI responses between IEIs with and without hybrid immunity.
Participants with combined B- and T-cell deficiency had lower CMI response compared with participants with B-cell deficiencies, and there was no significant difference between CMI response in healthy and B cell-deficient participants. Importantly, we observed two patients with predominant B-cell deficiency who had S-specific T-cell responses that were higher than those in controls (Figure 5). This suggests that B cells are not crucial in mounting a functional T-cell response to COVID-19 vaccine. Indeed, this was further supported in a study conducted by Guiterrez-Bautista et al., where they found that all 26 CVID patients and three of four XLA patients mounted a positive cellular response to doses 2 and 3 (31).
The anti-N antibody response at D3 + 24wk increased in IEIs when compared to D3 + 4wk, while the response decreased in controls. This is consistent with the timing of the reported SARS-CoV-2 infections in the study. Additionally, it is established that participants with IEIs can have a higher frequency of viral persistence and prolonged COVID-19 compared to controls and may in part explain the durability of anti-S IgG, IgA, and IgM at D3 + 24wk (47, 48).
The large sample size of 149 IEI cases is a major strength of this study, as this is the first collaboration of its size across Canada to assess post-vaccination responses in individuals with IEIs. Additionally, this is one of the few studies to examine CMI responses to COVID-19 vaccination in IEIs, particularly IEIs other than PAD. Nonetheless, this study has several limitations. First, due to limited control recruitment, additional serological data were collected from the SSO study, as described. Therefore, variables such as recruitment strategy and technical and methodological differences may vary between our cohort and SSO controls. An assessment of vaccine safety and effectiveness was also limited with a small number of controls. Further, this study only examined HI and CMI responses up to D3 + 24wk. In Canada, individuals with IEIs are recommended to receive COVID-19 vaccination every 6 months with the updated vaccines. Therefore, more research on the immune responses to additional doses is being conducted by our group. Finally, subgroup analysis of vaccine response particularly in IEI participants with innate immune defects was limited by the small sample size.
To conclude, IEI patients can elicit both HI and CMI responses that are durable at least 6 months after their primary series, but the degrees of responses vary depending on underlying immune defects and generally are lower than those of healthy individuals. Although the correlation between S-specific antibodies and viral neutralizing titers is strong, the correlation between HI and CMI is not similarly seen. We did not observe a concerning vaccine safety signal. This study supports the Canadian vaccine recommendations at the time that the study was conducted, which recommended a three-dose primary series in people with IEIs.
Data availability statement
The original contributions presented in the study are included in the article/Supplementary Material. Further inquiries can be directed to the corresponding author.
Ethics statement
The Ottawa Hospital Research Institute was the lead site and obtained overarching ethics approval (CTO#1978) for Ontario sites. Each study site also obtained approval from their local Ethics Board prior to study commencement. The studies were conducted in accordance with the local legislation and institutional requirements. The participants provided their written informed consent to participate in this study.
Author contributions
DU: Conceptualization, Data curation, Formal analysis, Visualization, Writing – original draft, Writing – review & editing. EF: Data curation, Methodology, Resources, Writing – review & editing. HC: Data curation, Methodology, Resources, Writing – review & editing. DV: Data curation, Methodology, Writing – review & editing, Resources. KT: Data curation, Methodology, Writing – review & editing, Resources. BD: Data curation, Methodology, Writing – review & editing, Resources. TI: Data curation, Methodology, Writing – review & editing, Resources. HD: Data curation, Methodology, Writing – review & editing, Resources. AP-H: Data curation, Methodology, Writing – review & editing, Resources. JU: Data curation, Methodology, Resources, Writing – review & editing. SB: Data curation, Methodology, Writing – review & editing, Resources. TRu: Data curation, Methodology, Resources, Writing – original draft. SS: Data curation, Methodology, Writing – review & editing, Resources. NW: Writing – review & editing, Data curation, Methodology, Resources. LM-F: Data curation, Writing – review & editing, Methodology, Resources. TK: Data curation, Writing – review & editing, Methodology, Resources. LB: Data curation, Formal analysis, Writing – review & editing. SO: Data curation, Formal analysis, Writing – review & editing. M-AL: Formal analysis, Writing – review & editing, Data curation. CA: Formal analysis, Writing – review & editing, Data curation. MS: Formal analysis, Writing – review & editing, Methodology. TZ: Formal analysis, Writing – review & editing. TRa: Formal analysis, Methodology, Writing – review & editing. DY: Data curation, Formal analysis, Writing – review & editing. JC: Conceptualization, Formal analysis, Funding acquisition, Investigation, Methodology, Project administration, Supervision, Writing – original draft, Writing – review & editing.
Funding
The author(s) declare financial support was received for the research, authorship, and/or publication of this article. This study was funded by the Canada COVID-19 Immunity Task Force, Public Health Agency of Canada.
Acknowledgments
We would like to thank the VISID study investigators (Drs. Alejandro Palma, Dalhousie University; Bruce Ritchie, Alberta Health Sciences; C. Arianne Buchan, The Ottawa Hospital Research Institute; Chrystyna Kalincinsky, University of Manitoba; Elie Haddad, University of Montreal; Fabien Touzot, University of Montreal; Gina Lacuesta, Dalhousie University; Kyla Hildebrand, University of British Columbia; and Vy Kim, University of Toronto), the Special Immunization Clinic Network, the Clinical Immunology Network-Canada, and Immunity Canada for their partnership and support. We thank Dr. L. Porto, Ms. S. Oluwafemi, and Mr. M. Smolinski for their help with data cleaning. We thank Dr. C. Cooper at the Ottawa Hospital Research Institute for sharing the serology data of controls from the SSO cohort. We thank all participants and their caregivers for their involvement in the study. We would like to acknowledge the assistance of the CoVaRR-Net Biobank in storing and shipping biological specimens. MS is supported via salary awards from the BC Children’s Hospital Foundation and Michael Smith Health Research BC.
Conflict of interest
HC has received consultation, speaker fees, and educational funding not related to this study from Takeda, CSL Behring, Pharming, Sanofi, Novartis, Sobi, Pharvaris, and KalVista. KT receives grants from the Coalition for Epidemic Preparedness Innovations outside the submitted work. BD received consultation and speaker fees from Takeda and Pharming, not related to this study. MS has been an investigator on projects funded by GlaxoSmithKline, Merck, Moderna, Pfizer, and Sanofi-Pasteur. All funds have been paid to his institute, and he has not received any personal payments. JC has received consultation, speaker fees, and educational funding not related to this study from Takeda, CSL Behring, Grifols, Octapharma, GSK, Pfizer, AstraZeneca, EMD Serono, and Avir Pharma. LM-F is a member of the Data Safety Monitoring Committee for Encoded Therapeutics and has participated as an Advisory Board Member for Takeda, both unrelated to the submitted work.
The remaining authors declare that the research was conducted in the absence of any commercial or financial relationships that could be construed as a potential conflict of interest.
The author(s) declared that they were an editorial board member of Frontiers, at the time of submission. This had no impact on the peer review process and the final decision.
Generative AI statement
The author(s) declare that no Generative AI was used in the creation of this manuscript.
Publisher’s note
All claims expressed in this article are solely those of the authors and do not necessarily represent those of their affiliated organizations, or those of the publisher, the editors and the reviewers. Any product that may be evaluated in this article, or claim that may be made by its manufacturer, is not guaranteed or endorsed by the publisher.
Supplementary material
The Supplementary Material for this article can be found online at: https://www.frontiersin.org/articles/10.3389/fimmu.2024.1501908/full#supplementary-material
References
1. Fung M, Babik JM. COVID-19 in immunocompromised hosts: what we know so far. Clin Infect Dis. (2021) 72:340–50. doi: 10.1093/cid/ciaa863
2. Shields AM, Burns SO, Savic S, Richter A, UK PIN COVID-19 Consortium. COVID-19 in patients with primary and secondary immunodeficiency: The United Kingdom experience. J Allergy Clin Immunol. (2021) 147:870. doi: 10.1016/j.jaci.2020.12.620
3. Meyts I, Bucciol G, Quinti I, Neven B, Fischer A, Seoane E, et al. Coronavirus disease 2019 in patients with inborn errors of immunity: An international study. J Allergy Clin Immunol. (2021) 147:520–31. doi: 10.1016/j.jaci.2020.09.010
4. Tangye SG, COVID Human Genetic Effort consortium. Impact of SARS-CoV-2 infection and COVID-19 on patients with inborn errors of immunity. J Allergy Clin Immunol. (2022) 151:818–31. doi: 10.1016/j.jaci.2022.11.010
5. Avanzato VA, Matson MJ, Seifert S, Pryce R, Williamson B, Anzick SL, et al. Case study: prolonged infectious SARS-coV-2 shedding from an asymptomatic immunocompromised individual with cancer. Cell. (2020) 183:1901–1912.e9. doi: 10.1016/j.cell.2020.10.049
6. Baang JH, Smith C, Mirabelli C., Valesano AL, Manthei DM, Bachman MA, et al. Prolonged severe acute respiratory syndrome coronavirus 2 replication in an immunocompromised patient. J Infect Dis. (2021) 223:23–7. doi: 10.1093/infdis/jiaa666
7. Mileto D, Foschi A, Mancon A, Merli S, Staurenghi F, Pezzati L, et al. A case of extremely prolonged viral shedding: Could cell cultures be a diagnostic tool to drive COVID-19 patient discharge? Int J Infect Dis. (2021) 104:631. doi: 10.1016/j.ijid.2020.11.161
8. Tarhini H, Recoing A, Bridier-nahmias A, Rahi M, Lambert C, Martres P, et al. Long term SARS-CoV-2 infectiousness among three immunocompromised patients: from prolonged viral shedding to SARS-CoV-2 superinfection. J Infect Dis. (2021) 223:1522–7. doi: 10.1093/infdis/jiab075
9. Aydillo T, Gonzalez-Reiche A, Aslam S, de Guchte A, Khan Z, Obla A, et al. Shedding of viable SARS-coV-2 after immunosuppressive therapy for cancer. N Engl J Med. (2020) 383:2586–8. doi: 10.1056/NEJMc2031670
10. Hensley MK, Bain WG, Jacobs J, Nambulli S, Parikh U, Cillo A, et al. Intractable coronavirus disease 2019 (COVID-19) and prolonged severe acute respiratory syndrome coronavirus 2 (SARS-coV-2) replication in a chimeric antigen receptor-modified T-cell therapy recipient: A case study. Clin Infect Dis. (2021) 73:E815–21. doi: 10.1093/cid/ciab072
11. Choi B, Choudhary MC, Regan J, Sparks JA, Padera RF, Qiu X, et al. Persistence and evolution of SARS-coV-2 in an immunocompromised host. N Engl J Med. (2020) 383:2291–3. doi: 10.1056/NEJMc2031364
12. Kemp SA, Collier DA, Datir RP, Ferreira I, Gayed S, Jahun A, et al. SARS-CoV-2 evolution during treatment of chronic infection. Nature. (2021) 592:277–82. doi: 10.1038/s41586-021-03291-y
13. Guetl K, Moazedi-Fuerst F, Rosskopf K, Brodmann M, Krause R, Eller P, et al. SARS-CoV-2 positive virus culture 7 weeks after onset of COVID-19 in an immunocompromised patient suffering from X chromosome-linked agammaglobulinemia. J Infect. (2021) 82:414. doi: 10.1016/j.jinf.2020.10.025
14. Decker A, Welzel M, Laubner K, Grundmann S, Kochs G, Panning M, et al. Prolonged SARS-CoV-2 shedding and mild course of COVID-19 in a patient after recent heart transplantation. Am J Transplant. (2020) 20:3239. doi: 10.1111/ajt.16133
15. Feikin DR, Higdon MM, Abdu-Raddad LJ, Andrews N, Araos R, Goldberg Y, et al. Duration of effectiveness of vaccines against SARS-CoV-2 infection and COVID-19 disease: results of a systematic review and meta-regression. Lancet. (2022) 399:924–44. doi: 10.1016/S0140-6736(22)00152-0
16. Polack FP, Thomas SJ, Kitchin N, Absalon J, Gurtman A, Lockhart S, et al. Safety and efficacy of the BNT162b2 mRNA covid-19 vaccine. New Engl J Med. (2020) 383:2603–15. doi: 10.1056/NEJMoa2034577
17. Baden LR, El Sahly HM, Essink B, Kotloff K, Frey S, Novak R, et al. Efficacy and safety of the mRNA-1273 SARS-coV-2 vaccine. New Engl J Med. (2021) 384:403–16. doi: 10.1056/NEJMoa2035389
18. Voysey M, Clemens SA, Madhi SA, Weckx LY, Folegatti PM, Aley PK, et al. Safety and efficacy of the ChAdOx1 nCoV-19 vaccine (AZD1222) against SARS-CoV-2: an interim analysis of four randomised controlled trials in Brazil, South Africa, and the UK. Lancet. (2021) 397:99–111. doi: 10.1016/S0140-6736(20)32661-1
19. Bonilla FA. Update: Vaccines in primary immunodeficiency. J Allergy Clin Immunol. (2017) 141:474–81. doi: 10.1016/j.jaci.2017.12.980
20. Ismail SJ, Langley JM, Harris TM, Warshawsky BF, Desai S, FarhangMehr M, et al. Canada’s National Advisory Committee on Immunization (NACI): Evidence-based decision-making on vaccines and immunization. Vaccine. (2010) 28(Suppl1):A58. doi: 10.1016/j.vaccine.2010.02.035
21. Panagiotakopoulos L, Godfrey M, Moulia D, Link-Gelles R, Taylor CA, Chatham-Stephens K, et al. Use of an additional updated 2023–2024 COVID-19 vaccine dose for adults aged ≥65 years: recommendations of the advisory committee on immunization practices — United states, 2024. MMWR Morbidity Mortality Weekly Rep. (2024) 73:377–81. doi: 10.15585/mmwr.mm7316a4
22. COVID-19: the green book, chapter 14a - GOV.UK . Available online at: https://www.gov.uk/government/publications/covid-19-the-green-book-chapter-14a (Accessed June 25, 2024).
23. van Leeuwen LPM, Grobben M, GeurtsvanKessel C, Ellerbroek P, de Bree G, Potjewijd J, et al. Immune responses 6 months after mRNA-1273 COVID-19 vaccination and the effect of a third vaccination in patients with inborn errors of immunity. J Clin Immunol. (2023) 43:1104–17. doi: 10.1007/s10875-023-01514-7
24. Tangye S, Al-Hertz W, Bousfiha A, Cunningham-Rundles C, Franco JL, Holland SM, et al. Human inborn errors of immunity: 2022 update on the classifications from the international union of immunological societies expert committee. J Clin Immunol. (2022) 42:1473–507. doi: 10.1007/s10875-022-01289-3
25. Collins E, Galipeau Y, Arnold C, Bosveld C, Heishkanen A, Keeshan A, et al. Cohort profile: Stop the Spread Ottawa (SSO)—a community-based prospective cohort study on antibody responses, antibody neutralisation efficiency and cellular immunity to SARS-CoV-2 infection and vaccination. BMJ Open. (2022) 12:e062187. doi: 10.1136/bmjopen-2022-062187
26. Keeshan A, Galipeau Y, Heiskanen A, Collins E, McCluskie PS, Arnold C, et al. Results of the Stop the Spread Ottawa (SSO) cohort study: a Canadian urban-based prospective evaluation of antibody responses and neutralisation efficiency to SARS-CoV-2 infection and vaccination. BMJ Open. (2023) 13:e077714. doi: 10.1136/bmjopen-2023-077714
27. Immunization of immunocompromised persons: Canadian Immunization Guide - Canada.ca . Available online at: https://www.Canada.ca/en/public-health/services/publications/healthy-living/canadian-immunization-guide-part-3-vaccination-specific-populations/page-8-immunization-immunocompromised-persons.html (Accessed September 19, 2024).
28. Colwill K, Galipeau Y, Stuible M, Gervais C, Arnold C, Rathood B, et al. A scalable serology solution for profiling humoral immune responses to SARS-CoV-2 infection and vaccination. Clin Transl Immunol. (2022) 11:e1380. doi: 10.1002/cti2.v11.3
29. Cancer Institute, N. Common Terminology Criteria for Adverse Events (CTCAE) Common Terminology Criteria for Adverse Events (CTCAE) v5.0 (2017). Available online at: https://www.meddra.org/ (Accessed June 25, 2024).
30. Zendt M, Carrillo FAB, Kelly S, Saturday T, DeGrange M, Ginigeme A, et al. Characterization of the antispike IgG immune response to COVID-19 vaccines in people with a wide variety of immunodeficiencies. Sci Adv. (2023) 13:41. doi: 10.1126/sciadv.adh3150
31. Gutiérrez-Bautista J, Diaz-Alberola I, Tarrino M, Aguilera M, Cobo F, Reguera JA, et al. Follow-up of immune response in patients with common variable immunodeficiency following SARS-CoV-2 vaccination. Clin Exp Immunol. (2024) 217:253–62. doi: 10.1093/cei/uxae039
32. Delmonte OM, Oguz C, Dobbs K, Snyder TM, Lack J, Notarangelo L, et al. Perturbations of the T-cell receptor repertoire in response to SARS-CoV-2 in immunocompetent and immunocompromised individuals. J Allergy Clin Immunol. (2023) 153:1655–67. doi: 10.1016/j.jaci.2023.12.011
33. Chen P, Bergman P, Blennow O, Hansson L, Mielke S, Nowak P, et al. Real-world assessment of immunogenicity in immunocompromised individuals following SARS-CoV-2 mRNA vaccination: a one-year follow-up of the prospective clinical trial COVAXID. EBioMedicine. (2023) 94:104700. doi: 10.1016/j.ebiom.2023.104700
34. Loyal L, Braun J, Henze L, Kruse B, Dingeldey M, Reimer U, et al. Cross-reactive CD4+ T cells enhance SARS-CoV-2 immune responses upon infection and vaccination. Science. (2021) 374:eabh1823. doi: 10.1126/science.abh1823
35. Mateus J, Grifoni A, Tarke A, Sidney J, Ramirez SI, Dan JM, et al. Selective and cross-reactive SARS-CoV-2 T cell epitopes in unexposed humans. Science. (2020) 370:89–94. doi: 10.1126/science.abd3871
36. Almendro-Vázquez P, Laguna-Goya R, Paz-Artal E. Defending against SARS-CoV-2: The T cell perspective. Front Immunol. (2023) 14. doi: 10.3389/fimmu.2023.1107803
37. Moss P. The T cell immune response against SARS-CoV-2. Nat Immunol. (2022) 23:186–93. doi: 10.1038/s41590-021-01122-w
38. de Gier B, Huiberts AJ, Hoeve CE, den Hartog G, van Wekhoven H, van Binnendijk R. Effects of COVID-19 vaccination and previous infection on Omicron SARS-CoV-2 infection and relation with serology. Nat Commun. (2023) 14:1–7. doi: 10.1038/s41467-023-40195-z
39. McKeigue PM, McAllister D, Robertson C, Stockton D, Colhoun H. Reinfection with SARS-CoV-2: outcome, risk factors and vaccine efficacy in a Scottish cohort. medRxiv (2021), 2021.11.23.21266574. doi: 10.1101/2021.11.23.21266574
40. Bobrovitz N, Ware H, Ma X, Li Z., Hosseini R, Cao C, et al. Protective effectiveness of previous SARS-CoV-2 infection and hybrid immunity against the omicron variant and severe disease: a systematic review and meta-regression. Lancet Infect Dis. (2023) 23:556–67. doi: 10.1016/S1473-3099(22)00801-5
41. Altarawneh HN, Chemaitelly H, Ayoub H, Tang P, Hasan M, Yassine H, et al. Effects of previous infection, vaccination, and hybrid immunity against symptomatic Alpha, Beta, and Delta SARS-CoV-2 infections: an observational study. EBioMedicine. (2023) 95:104734. doi: 10.1016/j.ebiom.2023.104734
42. Salinas AF, Mortari EP, Terreri S, Quintarelli C, Pulvirenti F, Di Cecca S, et al. SARS-coV-2 vaccine induced atypical immune responses in antibody defects: everybody does their best. J Clin Immunol. (2021) 41:1709–22. doi: 10.1007/s10875-021-01133-0
43. van Leeuwen LPM, GeurtsvanKessel C, Ellerbroek P, de Bree G, Potjewijd J, Rutgers A, et al. Immunogenicity of the mRNA-1273 COVID-19 vaccine in adult patients with inborn errors of immunity. J Allergy Clin Immunol. (2022) 149:1949–57. doi: 10.1016/j.jaci.2022.04.002
44. Hagin D, Freund T, Navon M, Haperin T, Adir D, Marom R, et al. Immunogenicity of Pfizer-BioNTech COVID-19 vaccine in patients with inborn errors of immunity. J Allergy Clin Immunol. (2021) 148:739–49. doi: 10.1016/j.jaci.2021.05.029
45. Barahona Afonso AF, Joao CM. The production processes and biological effects of intravenous immunoglobulin. Biomolecules. (2016) 6:15. doi: 10.3390/biom6010015
46. Grifols. Plasma journey . Available online at: https://www.grifols.com/en/plasma-journey (Accessed January 11, 2024).
47. Ruffner MA, Sullivan KE, Henrickson SE. Recurrent and sustained viral infections in primary immunodeficiencies. Front Immunol. (2017) 8. doi: 10.3389/fimmu.2017.00665
Keywords: COVID-19, vaccine response, inborn error of immunity, humoral immunity, cellular mediated immunity, immunogenicity
Citation: Unninayar D, Falcone EL, Chapdelaine H, Vinh DC, Top KA, Derfalvi B, Issekutz TB, Decaluwe H, Pham-Huy A, Upton J, Betschel SD, Rubin T, Suresh S, Wright NAM, Murguía-Favela L, Kalashnikova T, Barrett L, Oldford S, Langlois M-A, Arnold C, Sadarangani M, Zhang T, Ramsay T, Yazji D and Cowan J (2025) Humoral and cell-mediated immune responses to COVID-19 vaccines up to 6 months post three-dose primary series in adults with inborn errors of immunity and their breakthrough infections. Front. Immunol. 15:1501908. doi: 10.3389/fimmu.2024.1501908
Received: 25 September 2024; Accepted: 16 December 2024;
Published: 21 January 2025.
Edited by:
Jens Van Praet, AZ Sint-Jan Brugge-Oostende AV, BelgiumReviewed by:
Davide Firinu, University of Cagliari, ItalyEmily S. J. Edwards, Monash University, Australia
Copyright © 2025 Unninayar, Falcone, Chapdelaine, Vinh, Top, Derfalvi, Issekutz, Decaluwe, Pham-Huy, Upton, Betschel, Rubin, Suresh, Wright, Murguía-Favela, Kalashnikova, Barrett, Oldford, Langlois, Arnold, Sadarangani, Zhang, Ramsay, Yazji and Cowan. This is an open-access article distributed under the terms of the Creative Commons Attribution License (CC BY). The use, distribution or reproduction in other forums is permitted, provided the original author(s) and the copyright owner(s) are credited and that the original publication in this journal is cited, in accordance with accepted academic practice. No use, distribution or reproduction is permitted which does not comply with these terms.
*Correspondence: Juthaporn Cowan, amNvd2FuQHRvaC5jYQ==
†See Acknowledgments for contributors