- 1College of Animal Science and Technology, Jiangxi Agricultural University, Nanchang, China
- 2Key Laboratory of Sustainable Development of Marine Fisheries of Ministry of Agriculture, Yellow Sea Fisheries Research Institute, Chinese Academy of Fishery Sciences, Qingdao, China
- 3Laboratory for Marine Fisheries Science and Food Production Processes, Qingdao National Laboratory for Marine Science and Technology, Qingdao, China
Th2 immunity is a primary host defense against extracellular pathogens, and different IL4/13 paralogues are involved in this immune response in fish. Here, we identified IL4/13A for further Th2 immune response providing information in turbot. The results showed that the full length of the IL4/13A gene is 1,333 bp, containing a 432-bp open reading frame (ORF) that encoded 144 amino acids. Phylogenetic analysis recently showed that turbot IL4/13A has a relationship with Dicentrarchus labrax. Moreover, syntenic analysis revealed similar neighboring genes associated with turbot IL4/13A, compared with other teleosts and mammals. In addition, IL4/13A was widely expressed in all examined tissues with the highest expression level in skin, followed by liver and gill. Finally, IL4/13A showed a general trend of upregulation in immune tissues following bacterial challenge. The significant quick induction of IL4/13A indicated its key roles to prevent pathogens. Characterizations of IL4/13A will probably contribute to understanding of Th2 immunity in fish.
1 Introduction
Interleukin-4 (IL-4) and interleukin-13 (IL-13) are Th2-type cytokines that drive canonical Th2 immunity in mammals (1). Although IL-4 and IL-13 have low amino acid sequence identity (<30%), they are really close. They are often activated together and participate in the same immune response (2–4). IL-4 and IL-13 are involved in adaptive immune responses by multiple Th2-mediated humoral immune responses and promoting CD4+ Th-cell differentiation, including Ig production, Ig class switching, B-cell proliferation, and B-cell activation (1, 5, 6). They act as anti-inflammatory agents by inhibiting the production of TNF-α, IL-1β, and IL-6. In addition, they can also induce programmed macrophages to differentiate into alternately activated macrophages (4, 7), thereby improving acute inflammatory response and promoting wound healing, tissue repair, and angiogenesis (8).
In fish, IL-4/13A and IL-4/13B function similarly to IL-4 and IL-13 in mammals (9–14). However, the low homology of IL4/13A makes it difficult to obtain in different fish especially those without a genome sequence. IL4/13A has been identified, and it provides a basal level of immunity. It has been reported that bony fish IL-4/13A can promote the proliferation and differentiation of B cells in vivo (15). After injecting recombinant IL-4/13A into zebrafish, T cells were also activated (16). In addition, IL-4Rα has been shown to bind to IL-4/13A in vitro, thereby interfering with IL-4/13A-mediated B-cell proliferation (12). In conclusion, the induction of Th2 by IL-4/13 homologues has been confirmed.
The origin and evolution of the Th2 immune response may be that a single IL-4/13 gene is replicated in different lineages by tandem duplication or whole genome duplication (WGD) events. In the second round (2R) WGD bony spotted fish (Lepisosteus oculatus) genome, a single IL-4/13 gene was found between KIF3A and RAD50 (4). Bony fish have two genes in the IL-4/13 family, IL-4/13A (adjacent to RAD50) and IL-4/13B (adjacent to KIF3A), which are most likely the result of round 3 (3R) WGD (17). Recently, four IL-4/13 loci were identified in the genomes of rainbow trout and Atlantic salmon, and three active genes IL-4/13A, IL-4/13B1, and IL-4/13B2 were cloned (14). With this likelihood, IL-4/13B1 and IL-4/13B2 have originated from the salmonid 4R WGD event (18).
In order to better understand the Th2 immune responses of turbot, we first reported the identification, molecular characterization, and IL-4/13A genomic loci in the turbot genome. Phylogenetic analysis showed that the IL4/13A gene had relatively low homology among different fish. The transcriptional expression before and after bacterial injection was compared and analyzed.
2 Materials and methods
2.1 Fish and sampling
Juvenile turbot weighing 150 ± 10 g were taken from the farm of Shandong Oriental Ocean Sci-Tech Co., Ltd. (Shandong, China). The fish adapted to the test environment for 7 days using a flow-through system. The fish were randomly assigned to six tanks, and among them, three were vaccinated with attenuated E. tarda. The immune method was exactly as described in our previous study (19). The experimental fish were injected with 100 µl attenuated Edwardsiella tarda (approximately 1 × 106 colony forming units), and the control fish received 100 µl of 0.9% sterile physiological saline. Three fish were randomly collected at 0 h, 6 h, 12 h, 24 h, and 48 h from each tank immediately after anesthetization with 0.05% tricaine methane sulfonate (MS-222, Sigma, St. Louis, MO, USA). Samples at 0 h were used as control. Samples were flash-frozen in liquid nitrogen and stored at −80°C for standby.
2.2 Total RNA extraction
RNA was extracted using TRIzol reagents (Invitrogen, Carlsbad, CA, USA) according to the manufacturer’s instructions. RNA degradation was detected by 1% agarose gel electrophoresis. The quality of RNA was measured using the NanoDrop 2000 spectrophotometer (Thermo, New York, MA, USA). The RNA was then reverse-transcribed into cDNA for real-time fluorescence quantitative PCR assay.
2.3 Cloning and analysis of turbot IL-4/13A gene
RACE-PCR was performed using the 5′ and 3′-Full RACE Kit (TaKaRa, China). Primers F1/F2 and R3/R4 (Table 1) for the RACE-PCR were designed according to turbot IL-4/13A gene sequences predicted from our transcriptome database (SRA accession no. SRP129900). PCR products were cloned into the pMD18-T simple vector (TaKaRa, China), and primers RV-M/M13-47 were used for sequencing. Primers F5/R5 were used to verify the integrity of the IL4/13A gene sequence.
The sequence similarity analysis was carried out by the NCBI BLAST program. The Molecular Evolutionary Genetic Analysis (MEGA) software was used to construct the phylogenetic tree. Multiple-sequence alignment was performed by the Clustal X2 program. Signal peptide and glycosylation sites were predicted using Signal P4.0 software and the NetNGlyc1.0 server, respectively. The genomic structure of the IL-4/13A gene was analyzed by GeneWise software. The genes related to IL-4/13A in other vertebrate genomes were identified by Ensembl and GenBank databases, and the phylogenetic relationship between species was obtained.
2.4 Expression analysis of the turbot IL-4/13A gene
Reverse transcription was performed using the TaKaRa PrimeScript™ RT Reagent Kit with gDNA Eraser (Perfect Real Time). The gene-specific primer sets of F6/R6 (Table 1) were used for quantitative real-time PCR (qPCR). The β-actin gene was used as an internal parameter for quality control. β-Actin F and β-actin R as reference gene primers are provided in Table 1. The 25-μl reaction system was as follows: SYBR® Premix Ex Taq п (Tli RNase H Plus (2×) 12.5 μl, upstream primer 1.0 μl (10 μM), downstream primer 1.0 μl (10 μM), cDNA template 2.0 μl (100 ng), and RNase Free dH2O 8.5 μl. Cycling conditions were as follows: 95°C for 1 min, followed by 40 cycles of 95°C 15 s, 60°C 15 s, 72°C 30 s. The fluorescence output of each cycle was measured and recorded for subsequent analysis. Each reaction had three parallels. Real-time quantitative PCR data analysis method: the 2−△△Ct method was used in this experiment, and the calculation formula were as follows: (1) relative expression level (fold change) = 2−△△Ct; (2) △△Ct = (Ct target gene − Ct internal reference gene) treated group − (Ct target gene − Ct internal reference gene) untreated group.
2.5 Statistical analysis
The data were expressed as mean ± SD (standard deviation of the mean) and were analyzed in SPSS 19.0 software. Statistical significance was considered if p < 0.05.
2.6 Animal ethics
The experiment was performed in strict accordance with the guidelines and ethical principles of the Experiment Animal Welfare Ethics Committee of China. The experimental design was approved by the Committee on Research Ethics of the Department of Laboratory Animal Science, Jingxi Agricultural University. All efforts were made to minimize fish suffering. Approval Code: JAU-2020-0021.
3 Results and discussion
3.1 Identification and characterization of turbot IL-4/13A
The results have been submitted to NCBI (BankIt2748472 Seq OR621078). The complete cDNA sequence of IL-4/13 was 1,333 bp, containing a 432-bp open reading frame (ORF) that encoded 144 amino acids (Figure 1), with predicted signal peptides of 21 amino acids and 4 potential N-glycosylation sites (Table 2). The theoretical molecular weight of the turbot IL-4/13 mature peptide was 16.2 kDa. The turbot IL-4/13 has a basic pI 9.28 (Table 2). The instability index of turbot IL-4/13 is 21.06 belonging to stable protein. For all of fish IL-4/13A, the protein of turbot IL-4/13 is hydrophilic and the grand average of hydropathicity of turbot IL-4/13 is −0.130. As can be seen from Table 2, the characterizations of turbot IL-4/13 were similar with other fish IL-4/13A.
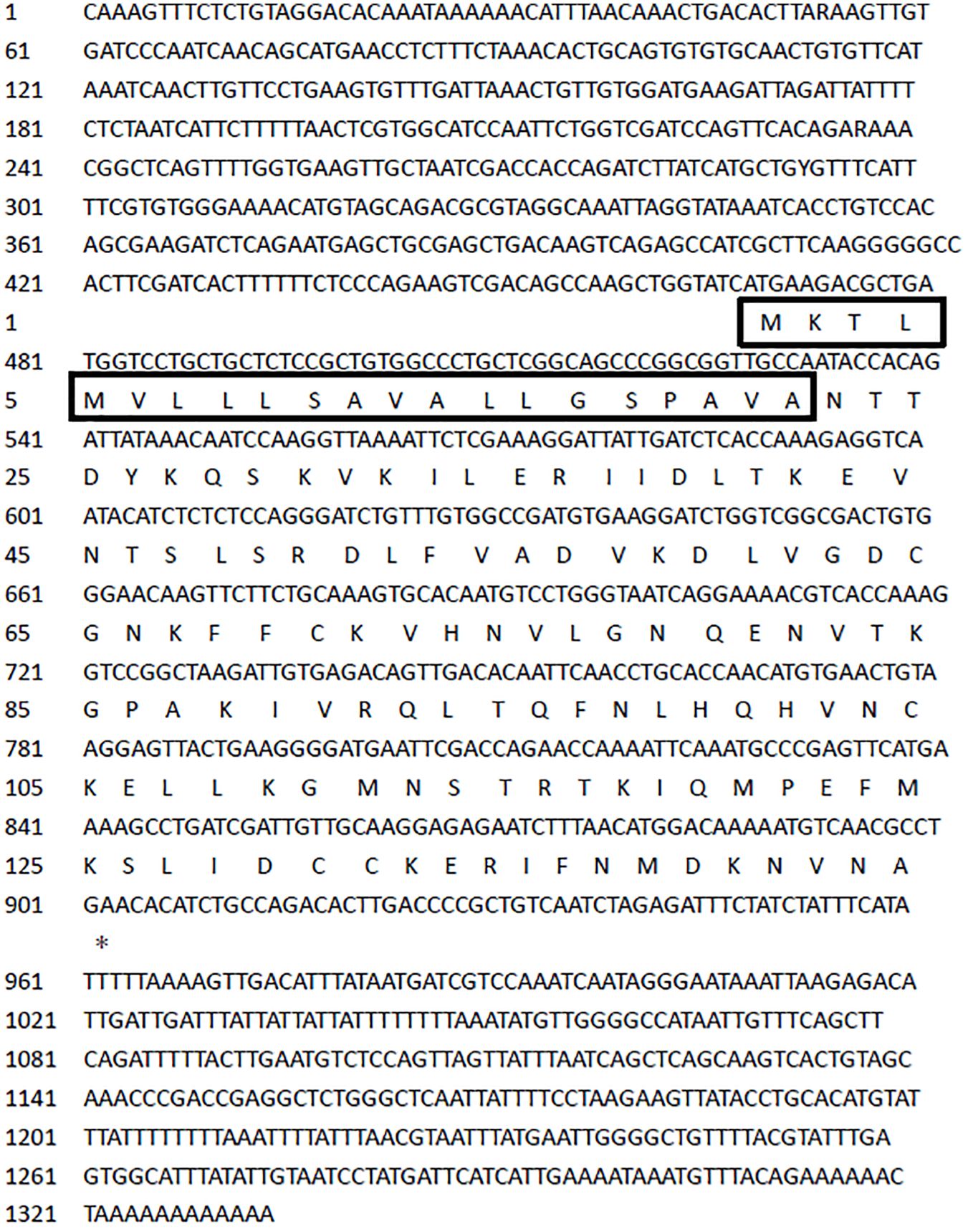
Figure 1. The determined cDNA sequence of the IL-4/13 gene is 1,333 bp, encoding 144 amino acids. The black box denotes predicted signal peptides of 21 amino acids. * is termination codon.
A BLAST search was performed to determine the homologous amino acids to other species. The turbot IL-4/13 shares low identity (32%–35%) to IL-4/13A from Sparus aurata, Tetraodon nigroviridis, and Dicentrarchus labrax. The IL-4/13A gene which has low homology was also found in salmonid, sea bass, yellow croaker, tetraodon, and so on (14, 20–22). The homology between tetrapods and tetraodon is <15%. Low homology demonstrated that IL-4/13 genes were fast evolving.
In the IL-4/13A locus, we further identified a highly conserved homologous gene segment in bony fish (Figure 2). The turbot IL-4/13 gene was adjacent to the RAD50 gene, which was exactly the same as previously reported for other teleost IL-4/13A (23). There are at least six conserved genes (containing, LARS, RBM27, POU4F3, IL-4/13A, RAD50, and DRD1) that are identical with 3R teleost Danio rerio and Tetraodon nigroviridis (Figure 2). This suggested the turbot IL-4/13 is IL-4/13A.
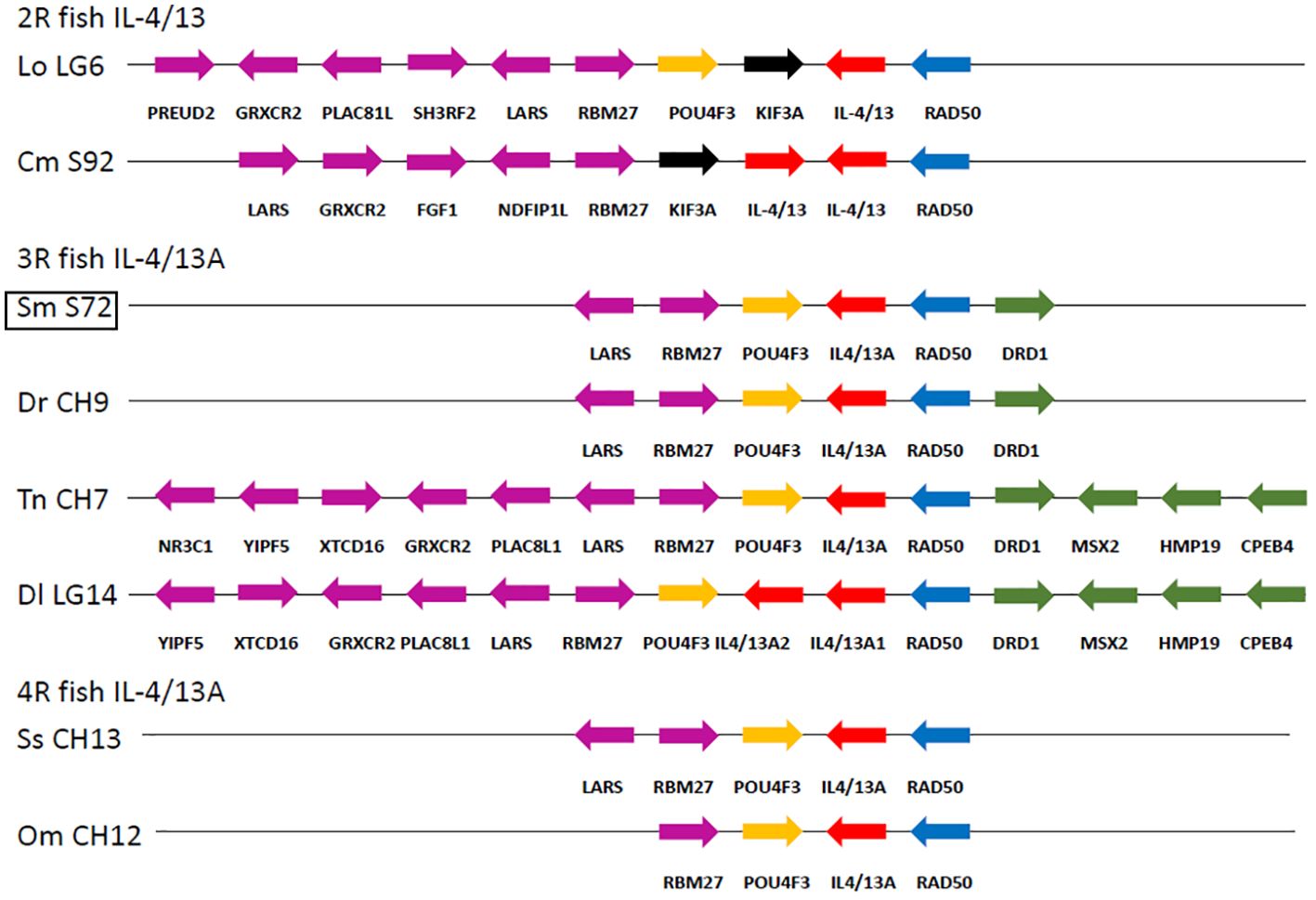
Figure 2. Gene synteny of IL-4/13A loci across bony fish. Evolutionarily relevant genes located at the 5′ and at the 3′ of the IL-4/13 loci are evidenced, and their strand orientations are indicated with arrows. The immediately flanking (POU4F3/RAD50) and neighboring genes at each side of the 2R/3R/4 fish IL-4/13 loci are differently colored using the European sea bass genomic organization as a reference. Lo, Lepisosteus oculatus; Cm, Callorhinchus milii; Sm, Scophthalmus maximus; Dr, Danio rerio; Tn, Tetraodon nigroviridis; Dl, Dicentrarchus labrax; Ss, Salmo salar; Om, Oncorhynchus mykiss.
3.2 Phylogenetic analyses
To gain a deeper understanding of the evolution of IL-4/13A, a phylogenetic tree was constructed based on multiple comparisons of IL-4/13A, IL-4/13B in fish, and IL-4 and IL-13 in mammals (Figure 3). The IL-4/13 of 2R fish Callorhinchus milii and IL-4 and IL-13 of 2R mammals formed a cluster with 64% bootstrap support. The turbot IL-4/13A, along with the IL-4/13A from some 3R fish (Dicentrarchus labrax, Tetraodon nigroviridis, Oryzias latipes, and Takifugu rubripes), formed a cluster with 51% bootstrap support. Then, the two formed a large cluster with 28% bootstrap support. Although the bootstrap support is low, they are more closely related compared with the IL-4/13A and IL-4/13B molecules from some other 3R fish (Danio rerio, Carassius auratus, Ctenopharyngodon idella, Cyprinus carpio). The IL-4/13A molecules form 4R fish (Oncorhynchus mykiss and Salmo salar) and the IL-4/13B molecules from 3R fish (Takifugu rubripes and Dicentrarchus labrax) are furthest with turbot IL-4/13A. These suggested that the differentiation of these IL-4/13 genes in fish occurs after the third round (3R) of whole genome duplication (WCG). This result agrees with Ohtani (13). Meanwhile, the IL-4/13 gene is still differentiated in 4R fish.
3.3 Structural features of turbot IL-4/13A
In teleost IL-4/13A, four to six cysteine residues were found and the residues are in different positions. The nine cysteine residue positions conserved in different lineages (14) are highlighted below the sequences (Figure 4). The only conserved cysteine residue in all sequences was C4. Turbot IL-4/13A contains four conserved cysteine residues Cys64, Cys70, Cys104, and Cys130 (conserved positions corresponding to C3, C4, C8, and C9, respectively) and one additional cysteine residue Cys131; as predicted for 3R of fish IL-4/13A, they should form two disulfide bridges (C3 and C4, C8, and C9) (24). The predicted alpha helix of turbot IL-4/13A is 62.50%, the random coil is 36.11%, and the extended strand is 1.39%.
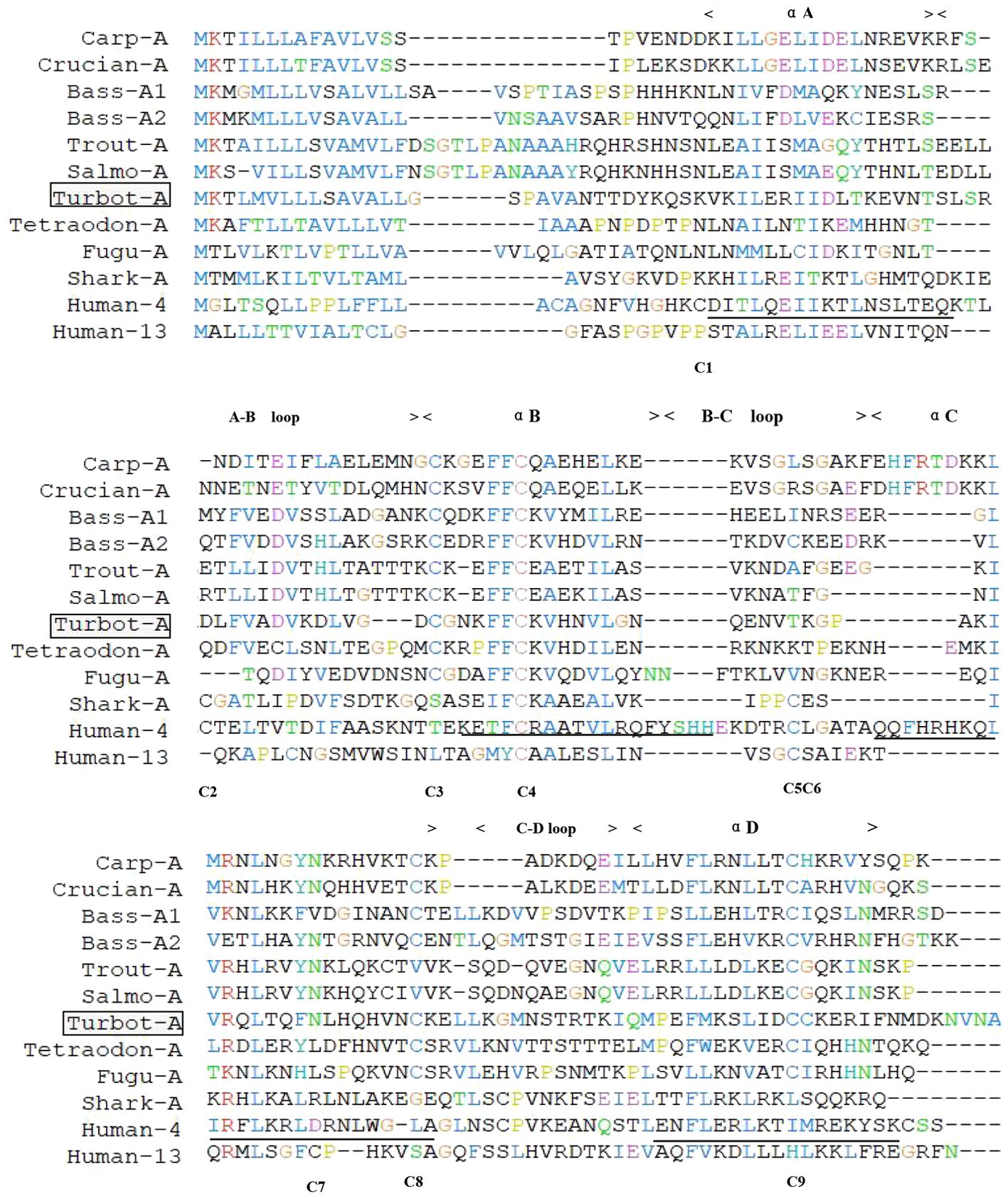
Figure 4. Amino acid sequence alignment of the predicted turbot IL4-13A with selected IL4-13 molecules. The position of the nine cysteine residues found in the different sequences is highlighted below the alignment and shown belong the sequences. Human-4 C5 and Huan-13 C6 are on the same vertical line. The four α-helices and loop regions known for human IL-4 are shown above the alignment and the amino acids involved in the α-helices underlined in the human IL-4 sequence.
3.4 Expression analyses of turbot IL-4/13A
The expression of turbot IL-4/13A could be detected in the kidney, spleen, intestine, gill, liver, and skin by real-time qPCR (Figure 5). In general, the expression level was highest in skin, followed by liver, gill, kidney, and spleen, and lowest in intestine (Figure 3). The expression level of IL-4/13A was similar to large yellow croaker (Larimichthys crocea) and Atlantic salmon (Salmo salar) (21, 25). Interesting to note is that the lowest expression of IL-4/13A1 was found in gill in sea bass (Dicentrarchus labrax) (17). The expression of IL-4/13A2 was abundant in gill in sea bass. The IL-4/13A gene was further differentiated into two genes in sea bass. The regulation of type 2 immune response in fish is complicated due to the existence of multiple IL-4/13 subtypes.
To further understand the role of IL-4/13A in the immune response of turbot, we examined its expression after inoculation with attenuated Edwardsiella tarda vaccine. As we focused on immunity, the spleen and kidney were selected. Following attenuated Edwardsiella tarda challenge, IL-4/13A expression increased rapidly in both spleen and kidney. From 6 h to 24 h postinfection, IL-4/13A expression was downregulated in spleen (Figure 6A). IL-4/13A expression touched the peak at 48 h postinfection by 3.2-fold relative to the control fish in spleen. In the kidney, IL-4/13A expression was upregulated gradually and touched the peak at 24 h by 2.2-fold, and then quickly plummeted to a minimum at 48 h (Figure 6B). Similar results were found in salmon (25) and large yellow croaker (21) after vaccine infection.
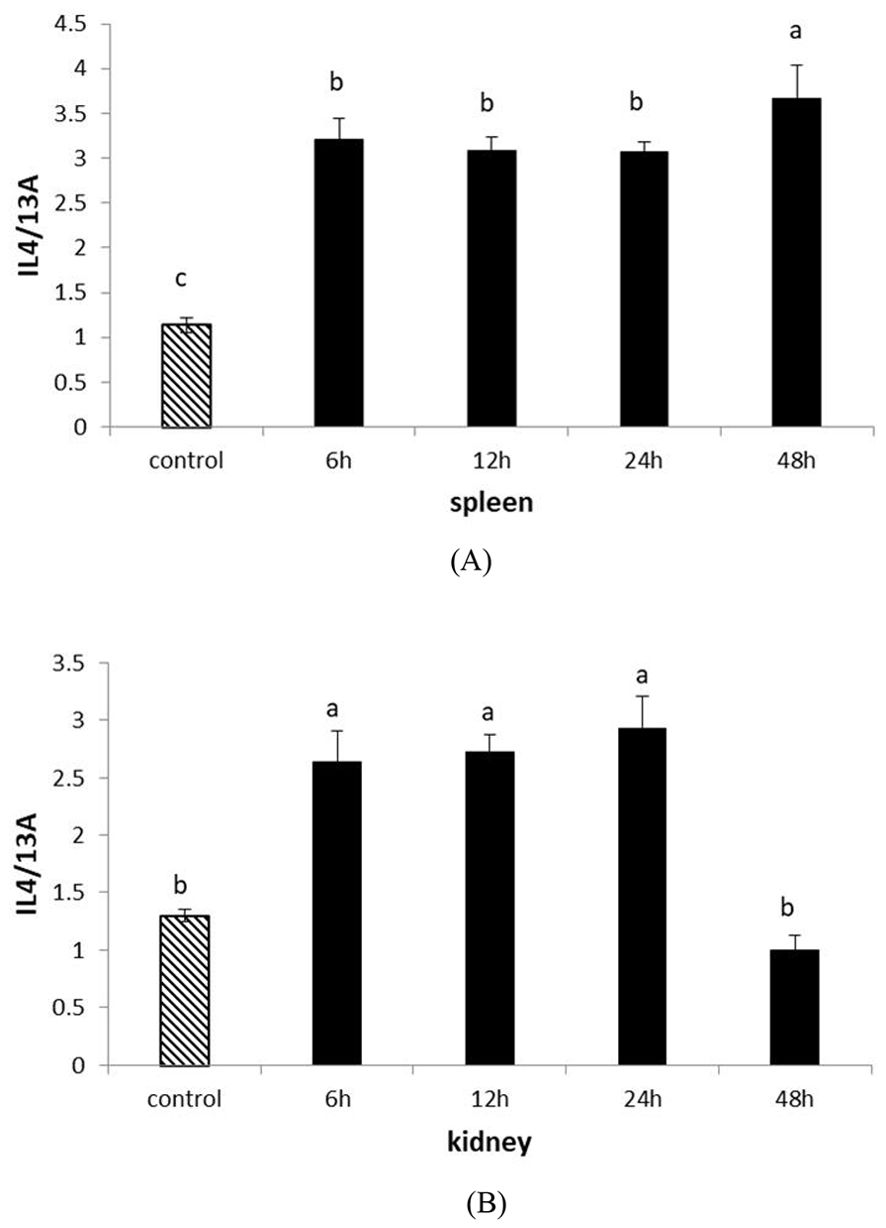
Figure 6. The expression of turbot IL-4/13A after stimulation with attenuated Edwardsiella tarda in kidney (A) and spleen (B). The data were expressed as mean ± SD. The relative significance of an LSD post-hoc test after a significant one way-ANOVA between different time points. Different lowercase letters represent significant differences (p < 0.05).
In conclusion, we identified the IL-4/13A gene from turbot and analyzed its characters and expression pattern. Turbot IL-4/13A is broadly and highly expressed and has potent bioactivities at low concentrations, which may provide a basal level of type 2 immunity. Further studies are needed to identify and analyze the homologous genes, gaining a deeper understanding of the evolution of type 2 immunity in turbot.
Data availability statement
The experiment was performed in strict accordance with the guidelines and ethical principles of the Experiment Animal Welfare Ethics Committee of China. The experimental design was approved by the Committee on Research Ethics of the Department of Laboratory Animal Science, Jingxi Agricultural University. All efforts were made to minimize fish suffering. Approval Code: JAU-2020-0021.
Ethics statement
The studies involving humans were approved by The Committee on Research Ethics of the Department of Laboratory Animal Science, Jingxi Agricultural University. The studies were conducted in accordance with the local legislation and institutional requirements. Written informed consent for participation was not required from the participants or the participants’ legal guardians/next of kin in accordance with the national legislation and institutional requirements. The animal study was approved by The Committee on Research Ethics of the Department of Laboratory Animal Science, Jingxi Agricultural University. The study was conducted in accordance with the local legislation and institutional requirements.
Author contributions
HH: Writing – original draft, Writing – review & editing. BL: Conceptualization, Methodology, Writing – review & editing. ZW: Formal analysis, Validation, Writing – review & editing. QZ: Funding acquisition, Supervision, Writing – review & editing.
Funding
The author(s) declare financial support was received for the research, authorship, and/or publication of this article. The research was supported by the Youth Scientific Research Funds of Science and Technology Department of Jiangxi Province (20202BABL215026) and the China Agriculture Research System (CARS-46).
Conflict of interest
The authors declare that the research was conducted in the absence of any commercial or financial relationships that could be construed as a potential conflict of interest.
Publisher’s note
All claims expressed in this article are solely those of the authors and do not necessarily represent those of their affiliated organizations, or those of the publisher, the editors and the reviewers. Any product that may be evaluated in this article, or claim that may be made by its manufacturer, is not guaranteed or endorsed by the publisher.
References
1. Bao K, Reinhardt RL. The differential expression of IL-4 and IL-13 and its impact on type-2 immunity. Cytokine. (2015) 75:25–37. doi: 10.1016/j.cyto.2015.05.008
2. McCormick S, Heller NM. Commentary: IL-4 and IL-13 receptors and signaling. Cytokine. (2015) 75:38–50. doi: 10.1016/j.cyto.2015.05.023
3. Redpath SA, Heieis G, Perona-Wright G. Spatial regulation of IL-4 signaling in vivo. Cytokine. (2015) 75:51–6. doi: 10.1016/j.cyto.2015.02.026
4. Wang T, Secombes CJ. The evolution of IL-4 and IL-13 and their receptor subunits. Cytokine. (2015) 75:8–13. doi: 10.1016/j.cyto.2015.04.012
5. Zhu J. T helper 2 (Th2) cell differentiation, type 2 innate lymphoid cell (ILC2) development and regulation of interleukin-4 (IL-4) and IL-13 production. Cytokine. (2015) 75:14–24. doi: 10.1016/j.cyto.2015.05.010
6. Gour N, Wills-Karp M. IL-4 and IL-13 signaling in allergic airway disease. Cytokine. (2015) 75:68–78. doi: 10.1016/j.cyto.2015.05.014
7. Liu G, Yang H. Modulation of macrophage activation and programming in immunity. J Cell Physiol. (2013) 228:502–12. doi: 10.1002/jcp.v228.3
8. Martinez FO. Regulators of macrophage activation. Eur J Immunol. (2011) 41:1531–4. doi: 10.1002/eji.201141670
9. Yamaguchi T, Miyata S, Katakura F, Nagasawa T, Shibasaki Y, Yabu T, et al. Recombinant carp IL-4/13B stimulates in vitro proliferation of carp IgM+ B cells. Fish Shellfish Immunol. (2016) 49:225–9. doi: 10.1016/j.fsi.2015.12.043
10. Braden LM, Koop BF, Jones S. Signatures of resistance to Lepeophtheirus salmonis include a TH2-type response at the louse-salmon interface. Dev Comp Immunol. (2015) 48:178–91. doi: 10.1016/j.dci.2014.09.015
11. Biswas G, Nagamine R, Hikima J, Sakai M, Kono T. Inductive immune responses in the Japanese pufferfish (Takifugu rubripes) treated with recombinant IFN-γ, IFN-γrel, IL-4/13A and IL-4/13B. Int Immunopharmacol. (2016) 31:50–6. doi: 10.1016/j.intimp.2015.12.005
12. Zhu LY, Pan PP, Fang W, Shao J, Xiang L. Essential role of IL-4 and IL-4Rα interaction in adaptive immunity of zebrafish: insight into the origin of Th2-like regulatory mechanism in ancient vertebrates. J Immunol. (2012) 188:5571–84. doi: 10.4049/jimmunol.1102259
13. Hodgkinson JW, Fibke C, Belosevic M. Recombinant IL-4/13A and IL-4/13B induce arginase activity and down-regulate nitric oxide response of primary goldfish (Carassius auratus L.) macrophages. Dev Comp Immunol. (2016) 67:377–84. doi: 10.1016/j.dci.2016.08.014
14. Wang T, Petronella T, Beatriz A, Amy H, Carolina T, Jiang Y, et al. First in-depth analysis of the novel Th2-type cytokines in salmonid fish reveals distinct patterns of expression and modulation but overlapping bioactivities. Oncotarget. (2016) 7:10917–46. doi: 10.18632/oncotarget.v7i10
15. Hu YL, Xiang LX, Shao JZ. Identification and characterization of a novel immunoglobulin Z isotype in zebrafish: implications for a distinct B cell receptor in lower vertebrates. Mol Immunol. (2010) 47:738–46. doi: 10.1016/j.molimm.2009.10.010
16. Lin AF, Xiang L, Wang QL, Dong WR, Gong YF, Shao JZ. The DC-SIGN of zebrafish: insights into the existence of a CD209 homologue in a lower vertebrate and its involvement in adaptive Immunity. J Immunol. (2009) 183:7398–410. doi: 10.4049/jimmunol.0803955
17. Ohtani M, Hayashi N, Hashimoto K, Nakanishi T, Dijkstra JM. Comprehensive clarification of two paralogous interleukin 4/13 loci in teleost fish. Immunogenetics. (2008) 60:383–97. doi: 10.1007/s00251-008-0299-x
18. Macqueen DJ, Johnston IA. A well-constrained estimate for the timing of the salmonid whole genome duplication reveals major decoupling from species diversification. Proc R Soc B: Biol Sci. (2014) 281:20132881. doi: 10.1098/rspb.2013.2881
19. Huo HH, Yin ST, Jia R, Huang B, Lei JL, Liu BL. Effect of crowding stress on the immune response in turbot (Scophthalmus maximus) vaccinated with attenuated edwardsiella tarda. Fish Shellfish Immunol. (2017) 67:353–8. doi: 10.1016/j.fsi.2017.05.067
20. Stocchi V, Wang T, Randelli E, Mazzini M, Gerdol M, Pallavicini A, et al. Evolution of Th2 responses: characterization of IL-4/13 in sea bass (Dicentrarchus labrax L.) and studies of expression and biological activity. Sci Rep. (2017) 7:2240. doi: 10.1038/s41598-017-02472-y
21. Mao KQ, Chen W, Mu YN, Ao JQ, Chen XH. Identification of two IL-4/13 homologues in large yellow croaker (Larimichthys crocea) revealed their similar roles in inducing alternative activation of monocytes/macrophages. Fish Shellfish Immunol. (2018) 80:180–90. doi: 10.1016/j.fsi.2018.06.002
22. Li JH, Shao JZ, Xiang LX, Wen Y. Cloning, characterization and expression analysis of pufferfish interleukin-4 cDNA: the first evidence of Th2-type cytokine in fish. Mol Immunol. (2007) 44:2078–86. doi: 10.1016/j.molimm.2006.09.010
23. Takuya Y, Fumio T, Uwe F, Johannes D. Along the axis between type 1 and type 2 immunity; principles conserved in evolution from fish to mammals. Biology. (2015) 4:814–59. doi: 10.3390/biology4040814
24. Alessio C, Andrea P, Alessandro V, Paolo F. DISULFIND: a disulfide bonding state and cysteine connectivity prediction server. Nucleic Acids Res. (2006) 34:W177–81. doi: 10.1093/nar/gkl266
25. Takizawa F, Koppang EO, Ohtani M, Nakanishi T, Hashimoto K, Fischer U, et al. Constitutive high expression of interleukin-4/13A and GATA-3 in gill and skin of salmonid fishes suggests that these tissues form Th2-skewed immune environments. Mol Immunol. (2011) 48:1360–8. doi: 10.1016/j.molimm.2011.02.014
Keywords: Scophthalmus maximus, IL4/13A, Th2 immunity, immune response, gene identification
Citation: Huo H, Liu B, Wang Z and Zhou Q (2024) Identification and expression analysis of Th2 immune-related gene IL4/13A in turbot (Scophthalmus maximus). Front. Immunol. 15:1500840. doi: 10.3389/fimmu.2024.1500840
Received: 25 September 2024; Accepted: 11 November 2024;
Published: 03 December 2024.
Edited by:
Jiong Chen, Ningbo University, ChinaReviewed by:
Avinash Premraj, Management of Scientific Centers and Presidential Camels, United Arab EmiratesZhujin Ding, Jiangsu Ocean University, China
Copyright © 2024 Huo, Liu, Wang and Zhou. This is an open-access article distributed under the terms of the Creative Commons Attribution License (CC BY). The use, distribution or reproduction in other forums is permitted, provided the original author(s) and the copyright owner(s) are credited and that the original publication in this journal is cited, in accordance with accepted academic practice. No use, distribution or reproduction is permitted which does not comply with these terms.
*Correspondence: Qiubai Zhou, emhvdXFpdWJhaUAxNjMuY29t