- 1Department of Medical Laboratory Science, College of Medicine and Health Sciences, Debre Markos University, Debre Markos, Ethiopia
- 2Department of Medicine, Centre for Inflammatory Diseases, Monash University, Clayton, VIC, Australia
- 3Department of Pharmacy, College of Medicine and Health Sciences, Debre Markos University, Debre Markos, Ethiopia
- 4Department of Biomedical Sciences, School of Medicine, Debre Markos University, Debre Markos, Ethiopia
Extracellular vesicles (EV) can be produced as part of pathology and physiology with increased amounts in pathological conditions. EVs can carry and transfer cargo such as proteins, nucleic acids, and lipids to target cells and mediate intercellular communication resulting in modulation of gene expression, signaling pathways, and phenotype of recipient cells. EVs greatly influence the extracellular environment and the immune response. Their immunomodulatory properties are crucial in rheumatoid arthritis (RA), a condition marked by dysregulated immune response. EVs can modulate the functions of innate and adaptive immune cells in RA pathogenesis. Differentially expressed EV-associated molecules in RA, such as microRNAs (miRNAs), long-noncoding RNAs (lncRNAs), messenger RNAs (mRNAs) and proteins are promising markers to diagnose the disease. miRNA, lncRNA, and circular RNA (circRNA) cargos in EV regulate inflammation and the pathogenic functions of RA fibroblast-like synoviocytes (RA-FLS). Downregulated molecules in RA tissue and drugs can be encapsulated in EVs for RA therapy. This review provides an updated overview of EVs’ immunomodulatory, diagnostic, and therapeutic roles, particularly emphasizing mesenchymal stem cell-derived EVs (MSC-EVs).
1 Introduction
Extracellular vesicles (EVs) are membrane-enclosed particles released by eukaryotic and prokaryotic cells as part of physiological and pathological processes with increased release under pathological conditions (1–3). EVs can carry various biomolecules, including proteins, nucleic acids, and lipids to the extracellular environment facilitating the transfer of their cargo to the recipient cells (4, 5). Recent evidence suggests that EVs may also contain mitochondria which control the epigenetics of target cells and organs (6).
The lipid bilayer of EVs encloses and protects their contents from the external environment (7). EVs vary in size from nanoscale exosomes to larger microvesicles and can be secreted by virtually all cell types (8). They are present in various body fluids including cerebrospinal fluid (CSF) (9), breast milk (10), synovial fluid (SF) (11), saliva (12), urine (13) and blood (14). Initially, EVs were considered mere cellular waste, leading to their limited investigation until recent years (15).
Traditionally, EVs were classified based on particle size and biogenesis into exosomes, microvesicles, and apoptotic bodies (4, 16). Exosomes, the smallest EVs, are formed through the inward budding of the plasma membrane during endosome generation, which matures into multivesicular bodies (MVBs) or late endosomes (7, 17). These MVBs either fuse with cell membrane to release exosomes or merge with lysosomes for degradation (1, 18). Microvesicles, (also called ectosomes) are generated through the outward budding and fission of the plasma membrane (19, 20), while apoptotic bodies, the largest EVs, are produced during programmed cell death and contain both cytoplasmic and nuclear materials (21, 22). However, no definitive molecular markers exist to distinguish these categories (8).
Given the limitations of biogenesis-based classification, the International Society for EVs recommended avoiding this terminology unless universal molecular markers and effective separation techniques are available. In its 2023 position paper, “Minimal Information for Studies of EVs (MISEV 2023),” the Society advocated using the general term “extracellular vesicles” and proposed size-based nomenclature, such as “small EVs (sEV)” for particles smaller than 200 nm and “large EVs (LEV)” for those larger than 200 nm (8).
EVs significantly impact the extracellular environment and immune responses (23, 24). They facilitate intercellular communication by transferring functional components or inducing receptor-mediated signaling (25). The surface proteins of EVs and their cargo can modulate gene expression, signaling pathways, and the phenotypes of target cells (15). EVs, produced endogenously, have advantages over synthetic nanoparticles and viral vectors, including higher biocompatibility, lower immunogenicity, and better evasion of phagocytosis (26). Moreover, their ability to cross biological barriers, such as the placental, blood-brain, blood-tumor, and blood-testis barriers, makes EVs promising tools for drug delivery (15, 27, 28). EVs have shown significant potential in the detection and treatment of autoimmune diseases including rheumatoid arthritis (RA) (29), multiple sclerosis (30), and type 1 diabetes (31).
RA is among the most prevalent chronic inflammatory disorders, affecting approximately 0.5% of the population globally (32, 33). This long-lasting autoimmune disease leads to the progressive destruction of joints. Despite advancements with disease-modifying antirheumatic drugs (DMARDs), treatment remains inconsistent, with 30-40% of patients discontinuing DMARDs due to ineffectiveness or side effects (34–36). Blocking tumor necrosis factor-α (TNF-α) helps reduce joint inflammation, prevent structural damage, and enhance the quality of life in 60-70% of RA patients. However, since some individuals don not respond to this therapy, alternative treatment options are necessary (37). Given the limitations of current treatments, researchers are increasingly exploring biotherapies, including EVs (38). In this review, we discuss what is presently known about the roles of EVs in RA’s immunomodulation, diagnosis, and therapeutic potential, with a special emphasis on mesenchymal stem cell (MSC)-derived EVs.
2 EV-induced immunomodulation in RA
Various immune cells, including T cells (39), B cells (40), macrophages (41), and mast cells (42) play a role in the progression of RA, with macrophages and T cell subsets playing particularly significant roles (43–45). T helper 1 (Th1) cells stimulate the production of interferon-γ (IFN-γ), TNF-α, and interleukin 2 (IL-2), contributing to cartilage damage and bone erosion, while, Th17 cells release IL-22, promoting the growth of synovial fibroblasts (46). B cells generate autoantibodies and drive autoimmune responses through the production of rheumatoid factor (40). Macrophages provide proinflammatory cytokines such as TNF- α and IL-1β (47).
New RA therapies have been proposed that focus on regulating the local immune response and promoting antigen-specific immune tolerance (48). EVs derived from MSC, neutrophils, granulocytic myeloid-derived suppressor cells (G-MDSCs), Dendritic cells (DC), and macrophages modulate the immune response within the inflammatory microenvironment of injured cartilage (49).
The type and condition of the source cell determine the influence of EVs on the immune response (50). MSC-derived EVs possess strong immunomodulatory properties, and their effectiveness is linked to their uptake by immune cells (51, 52). These EVs can regulate both innate and adaptive immune functions, reducing abnormal inflammation while ensuring safety in the surrounding microenvironment (53–55). This makes them a promising option for treating inflammatory diseases (56).
2.1 EV-induced innate immune modulation in RA
EVs influence the functions of innate immune cells, impacting processes like differentiation, activation, migration, and cytokine production, as well as their abilities in cytolysis, phagocytosis, and antigen transfer (57). Macrophages are crucial innate immune cells involved in the pathogenesis of RA (44, 58). In RA patients, there is an increase in pro-inflammatory M1 macrophages and a reduction in anti-inflammatory M2 macrophages (44). M1 macrophages secrete pro-inflammatory substances whereas M2 macrophages release anti-inflammatory agents (59). EVs can influence macrophage function by transferring regulatory miRNAs and proteins modulating inflammatory responses by affecting toll-like receptor 4 (TLR4) signaling and cytokine production (60, 61). M2 macrophage-derived EVs transfer proteins which can polarize macrophage to M2 phenotypes (62). Additionally, neutrophil-derived microvesicles can boost anti-inflammatory factors like transforming growth factor-β (TGF-β) and prevent inflammatory activation of synoviocytes in arthritis models (63).
MSC-derived EVs have been shown to promote M2 macrophages while reducing pro-inflammatory M1 macrophages in the synovial tissue of mice with collagen-induced arthritis (CIA) (64). Additionally, bone marrow MSC-derived EVs (BMSC-EVs) were found to inhibit the secretion of inflammatory cytokines including IL-1β, TNF-α, and IL-18 in macrophages from mice with RA (65). In vitro studies indicated that MSC-EVs can prevent DC maturation by downregulating the expression of CD80, CD83, and CD38, decreasing IL-6 and IL-12p70 secretion, and increasing TGF-β production. These findings suggest that MSC-EVs could be a promising therapeutic approach in mitigating autoimmune diseases such as RA by modulating dendritic cell function (66).
2.2 EV-induced adaptive immune modulation in RA
In RA, dysregulated immune responses activate auto-reactive T and B cells, leading to their proliferation and differentiation into pathogenic cells that produce autoantibodies, thereby driving joint inflammation and degradation (67).
EVs isolated from plasma of RA patients suppress early B cell activation in RA by downregulating the expression of activation markers like CD69+ and CD86+, and by inhibiting intracellular signaling pathways that are essential for B cell proliferation, function, and survival results (68). BMSCs and G-MDSCs release EVs that regulate B cell differentiation by promoting CD19+IL-10+ regulatory B (B reg) cells and reducing plasmablast phenotypes in the lymph node of mice with CIA (69, 70) (Figure 1).
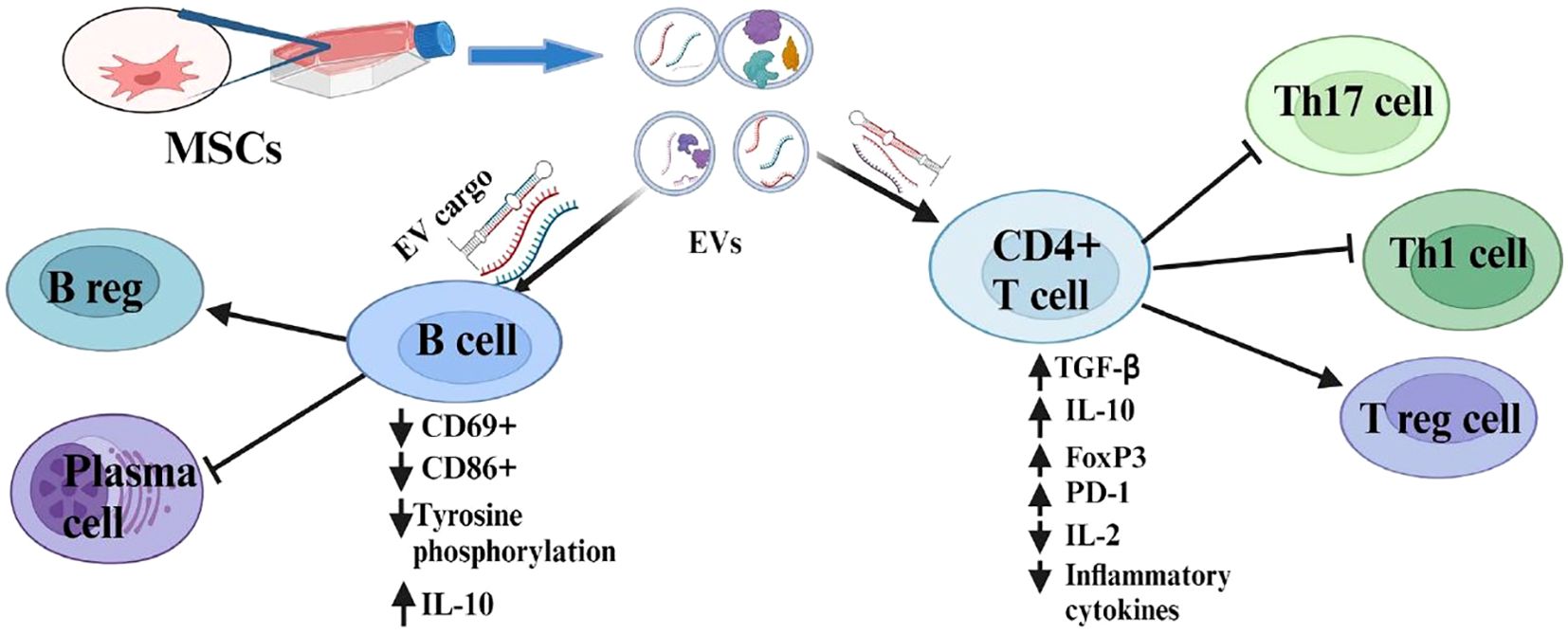
Figure 1. Immunosuppressive role of MSC-EVs and their modulatory effects on the adaptive immune cells.
Human gingival mesenchymal stem cell-derived EVs (GMSC-EVs) regulate CD4+ T cell subpopulations by increasing regulatory T (T reg) cells and decreasing Th1 and Th17 cells in the CIA model. Experiments conducted both in vivo and in vitro demonstrated that GMSC-EVs induce upregulation of anti-inflammatory cytokine (IL-10) and downregulation of proinflammatory cytokines including IFN-γ, IL-17A, TNF-α, and IL-6 (38). These EVs carry miR-148a-3p, which is responsible for immunomodulatory effects by directly targeting IKKB (inhibitor of nuclear factor kappa B kinase) in T cells (71). G-MDSC-derived EVs demonstrated a similar immunosuppressive effect on CD4 T cells in CIA mice. G-MDSC-EV cargos (miR-29a-3p and miR-93-5p) suppress the differentiation of Th1 and Th2 cells by targeting T-bet and signal transducer and activator of transcription 3 (STAT3), correspondingly (72).
EVs derived from human umbilical cord stem cells (hUCMSC-EVs) suppress T lymphocyte proliferation and induce apoptosis along with upregulation of forkhead box p3 (FoxP3) and downregulation of retinoic-related orphan receptor (RORγt) in the spleen of CIA mice (73, 74). These types of EVs demonstrated a Th1/Th17 and T reg cell balance accompanied by reduced levels of IL-17 and enhanced TGF-β and IL-10 in CIA mice (73–75). MSC-EVs derived from adipose tissue also modulate activated T cells by down-regulating miR23a-3p, which post-transcriptionally regulates TGF-β receptor 2 (TGFBR2) and increases the expression of FoxP3 (76). Furthermore, MSC-EV regulates the proliferation of activated T cells by inducing cell cycle arrest via upregulation of P27kip1 expression and downregulation of cdk2 expression (77). On the other hand, both CD4 and CD8 T cell proliferation were not affected in the presence of EVs derived from bone marrow MSC. However, an indirect inhibitory effect was observed through T reg cell induction resulting in a reduction of CD4 and CD8 T cells (69).
Research suggested that manipulating MSC-EVs could enhance a balance among Th cells and reduce the production of proinflammatory cytokines. EVs isolated from miR-146a transduced MSC resulted in upregulating FoxP3, TGFβ, and IL-10 and downregulating IFN-γ in CIA models (78, 79). More importantly, pro-inflammatory cytokine-priming of MSC-EVs does not affect its immunosuppressive potential (69, 80, 81). Moreover, EVs derived from interferon-β (IFN-β)-primed MSCs down-regulated the expression of RA-associated cytokines (IL-4, GM-CSF, IFN-γ, IL-2, TNF-α) and diminished CD4+ T-cell polyfunctionality in RA CD4+ T cells (82). Immortalized adipose tissue-derived MSCs primed with serum from RA disease conditions generate EVs that boost TGF-β1 production, promote Th2 induction, and facilitate M2 polarization, reducing inflammatory cytokines in CIA mice (83).
Under normoxic conditions (21% O2, 5% CO2), MSC-derived EVs promoted T reg cell phenotypes and reduced CD4+ T cell polarization toward Th17 phenotypes, demonstrating their immunomodulatory effects in an antigen-induced arthritis model (81). Under hypoxic conditions, EVs derived from polymorphonuclear myeloid-derived suppressor cells (PMN-MDSCs) suppress the proliferation of CD4+ T cells in the CIA mouse model (84). In contrast, synovial fibroblast-derived EVs in a hypoxic environment, reduce T reg cells and promote polarization of Th17 cells. Elevated levels of miR-424 under this condition downregulate FoxP3, thereby worsening RA (85).
EVs generated from TGF-β primed T reg cells effectively mitigated the Th17 and Treg cells imbalance in arthritic mice and regulated the inflammatory responses of recipient T cells via miR-449a-5p-dependent mechanism (86). Microvesicle mimetics (MVM) isolated from endotoxin-tolerant DCs possessed a bioactive miR155-3p and exhibited remarkable immunosuppression by inducing T reg and anti-inflammatory macrophages in RA models (87). In addition, in the RA microenvironment, EVs containing programmed death receptor 1 (PD-1) facilitate T cell exhaustion in the joints (88). Moreover, in RA patients, SF EVs expressing gangliosides (GD3), were associated with immunosuppression by inhibiting T cell activation after stimulation via TCR. This suggests that immunosuppressive EVs in the synovial fluid serve as a novel immune checkpoint for T cells (89).
3 EVs as a diagnostic marker of RA
The potential role of EVs in discovering specific biomarkers to diagnose various autoimmune diseases has been highlighted (2). The quantity of EVs is notably higher in the plasma and synovial fluid of individuals with RA than in healthy controls (90). EVs are recognized for containing distinct proteins that reflect the characteristics of their originating cells (91). Differentially expressed miRNA and lncRNAs containing EVs are also associated with RA’s immune response and metabolic process (92). By comprehending the variety of their contents and associated targets, it could be feasible to diagnose RA and other autoimmune diseases (93).
3.1 EV microRNAs (EV-miRNA) as a diagnostic marker of RA
miRNAs are short non-coding RNAs that play a role in cell signaling, intracellular communication, regulation of gene expression, and chronic inflammation and immune responses (94, 95). They are key regulators of skeletal remodeling and play a role in the development of RA (96). SF from joints exhibiting high-grade inflammation had 3.5 times more miRNA-positive EVs per ml than normal levels. Analysis of the most prevalent miRNAs indicated that they negatively regulate several inflammation-related genes, including STAT3, which play a pro-inflammatory role in RA (97).
While various EV-miRNAs have been investigated for HBV-related tumor detection (98), several studies have shown promising results in using EV-associated miRNAs for RA diagnosis (Table 1). Dysregulated RNAs in sEVs derived from FLS associated with arthritis in mice models were highlighted as a potential biomarker for RA (99). The miRNA content of EVs, such as miR-212-3p, miR-338-5p, miR-410-3p, and miR-537, showed elevated levels in early RA during methotrexate (MTX) treatment, suggesting their potential as diagnostic and prognostic biomarkers (100). miRNA cargos such as (hsa-miR-335-5p and hsa-miR-486-5p) were higher in the peripheral blood of RA patients than in healthy controls and associated with disease activity (101). Furthermore, miRNA-1915-3p containing EVs were elevated in the clinical remission group of Korean RA and negatively correlated with serum C-reactive proteins (CRP) levels and may be useful to indicate RA disease activity (102).
Differentially expressed miRNAs linked to RA pathogenesis, such as miR-155-5p, miR-146a-5p, miR-323a-5p, and miR-1307-3p, were found in EVs derived from RA synovial fibroblast cell lines after TNF-α stimulation (103). Based on the serum EV expression profiles, patients with RA exhibited elevated levels of variably expressed miR-125a-5p, miR-130b-3p, miR-151a-5p, miR-301a-3p, and miR-324-5p (104). A combination of sEV miRNAs and soluble tumor necrosis factor-like weak inducer of apoptosis (sTWEAK) diagnosed early RA with a sensitivity of 85.7% and a specificity of 100% (105) (Table 1).
3.2 EV-Long noncoding RNAs (EV-lncRNA) as a diagnostic marker of RA
Long non-coding RNAs (lncRNAs) represent a new category of non-coding RNAs that do not produce proteins (106). The expression profiling of lncRNAs in EVs obtained from the synovial fluid of RA demonstrated significant differences when compared to osteoarthritis (OA) and gout (107). The serum sEV lncRNA profiles in patients with RA were also distinct from those of healthy controls and patients with OA (92).
The expression of circular RNAs (circRNAs), such as circFTO, is elevated in EVs derived directly from FLS of RA patients. These EVs promote RA progression by suppressing chondrocyte growth and migration while enhancing apoptosis and catabolism (108). Variably expressed lncRNAs in serum EVs from RA patients showed both upregulation and downregulation (109). lncRNAs found in plasma EVs from individuals with RA exhibit distinct expression profiles, including several lncRNAs that may serve as diagnostic biomarkers. The receiver operating characteristics curve (ROC), which is used to evaluate the diagnostic accuracy of biomarkers, revealed that lncRNAs including SNHG6, RPS18P9, and CXXC4-AS1 demonstrated an area under the curve (AUC) ranges of 0.847-0.994 in diagnosing RA (110).
3.3 EV-associated protein and mRNAs as diagnostic markers of RA
Analysis of differentially expressed proteins in EVs from SF revealed that stromelysin-1 and pregnancy zone protein (PZP) were among the highly expressed proteins in RA as compared to OA (111). Proteomic analysis found that EVs from RA-FLS had higher pentraxin (PTX3) and lower proteasome 20S subunit beta 5 (PSMB5) levels than OA patients, promoting macrophage migration and RA progression (112). Lipid binding protein (LBP) and monocyte differentiation antigen (CD14) were also upregulated in EVs. Notably, the interaction of these proteins may play a role in nuclear factor kappa B (NF-κB) signaling, promoting the expression of IL-8 and TNF-α, which could contribute to the development of RA and serve as potential biomarkers for its diagnosis (113).
Differentially expressed proteins were identified as both upregulated and downregulated in the CD4+ T cell-derived EVs of RA patients, suggesting that these proteins could act as potential biomarkers for RA (114). The levels of CD3+ CD4+ protein containing EVs in the serum of RA patients are elevated, whereas the levels of CD3+CD8+ EVs are reduced, reflecting that total CD4+ T cells are dominant over CD8+ T cells (91).
In a subset of seropositive RA patients, rheumatoid factor immunoglobulin M (IgM-RF) was found on plasma EVs and associated with increased disease activity. This discovery suggests a potential biological factor that could explain the discrepancy between global disease activity assessments and the counts of tender and swollen joints (115). Elevated levels of circulating EVs testing positive for immunoglobulin G (IgG), IgM, CD41a, and citrulline were also observed in seropositive RA patients (116). Profiling of plasma EVs identifies proteins significantly linked to the patient’s global disease activity (PGA) in RA. Notably, actin-cytoskeleton linker proteins, including ezrin and moesin, correlate positively with PGA (117).
Circulating EVs express elevated levels of posttranslational modified proteins such as citrullinated proteins and contribute to the pathogenesis of RA by triggering autoimmunity (118). EVs containing major histocompatibility complex class II (MHC II) molecules can be loaded with citrullinated peptide antigens and presented to T cells (119). These peptide antigens can be recognized by autoreactive T cells and trigger the production of anticitrullinated protein antibodies, a key hallmark for RA (120, 121). Autophagy appears to contribute to the generation of citrullinated peptide and EVs in RA (122, 123). It also promotes the citrullinated peptide-MHC II interaction in RA synovial fibroblasts (124). The autophagic system releases cellular content through EVs (122, 125), which can propagate autoantigens and potentially contribute to joint inflammation in RA patients (126) (Figure 2).
The serum sEV mRNA profiles in RA patients differed from those of healthy controls and individuals with OA. A combination of differentially expressed mRNAs achieved an AUC of 0.845 in distinguishing RA from OA (92) (Table 1).
4 EV as a promising therapeutic agent for RA
EVs have gained interest as a potential cell-free therapy due to their low immunogenicity, tumorigenicity, and ease of management (38, 130). They are considered a promising approach for treating RA and may be used as drug delivery vehicles, including as nanocarriers to enhance the therapeutic effect of glucocorticoids in RA treatment (93, 131–133).
Research indicates that EVs from bone marrow macrophages lacking miR-100-5p exacerbate RA progression. In contrast, EVs overexpressing miR-100-5p help reduce inflammation and inhibit the proliferation of RA-FLS in RA (134). EVs from M2 macrophages, loaded with plasmid DNA for IL-10 and betamethasone sodium phosphate, reduced RA inflammation by promoting M1-to-M2 polarization and enhancing anti-inflammatory cytokine secretion (135). IL-4 delivered via small EVs (sEVs) showed a stronger anti-inflammatory effect in mice with CIA than soluble IL-4, indicating greater immunomodulatory potential (136). Additionally, macrophage-derived EVs loaded with IL-10 could be targeted to inflamed areas using noninvasive ultrasound, offering a promising strategy for macrophage polarization to M2 phenotypes in RA treatment (137).
A hybrid nanovesicle (HNV) combining an M1 macrophage membrane with exosome-mimic nanovesicles from M2 macrophages, loaded with black phosphorus sheets, can eliminate inflammatory cells in RA through near-infrared irradiation (138). Apoptotic EVs from macrophages and osteoclasts show synergistic effects in RA joints by reducing synovial inflammation, restoring cartilage, reversing bone erosion, and preserving joint structure (139). Additionally, EVs from immunosuppressive DCs can inhibit the onset and reduce the severity of CIA in mouse models (140). EVs from Indoleamine 2,3-dioxygenase-expressing DCs also demonstrated anti-inflammatory effects in murine models with CIA (141).
EVs engineered to carry super repressor IkB (srIkB), an NF-κB inhibitor, significantly reduced inflammatory cytokine production in PBMCs and synovial fibroblast mononuclear cells (SFMCs) collected from RA patients. Moreover, srIkB EVs treatment showed notable decreases in inflammation, cartilage degradation, and bone erosion in the joint tissues of CIA mice (85).
MSCs are a promising alternative for treating RA due to their immunomodulatory capabilities (142, 143) (Table 2). More importantly, the potential of EVs derived from MSCs in immunomodulation and tissue regeneration presents a novel concept for treating rheumatism (5, 144, 145). MSC EVs transfer non-coding RNAs that modulate crucial signaling pathways in the development of RA (34). Different miRNA and lncRNA cargos delivered by MSC-EVs influence RA disorders through the NF-κB and MAPK pathways (51). Moreover, EVs released from MSCs have been identified as important signaling molecules that play a role in the healing process by modulating the local microenvironment with anti-inflammatory properties (146, 147).
EVs derived from human embryonic stem cells MSCs reduce inflammation, cartilage degradation, and bone loss, primarily through the modulation of M2 macrophages in arthritis mouse models (64). Additionally, miR-378a-5p from BMSC-derived EVs enhances the proliferation, migration, and angiogenesis of human synovial microvascular endothelial cells by suppressing the IRF1/STAT1 pathway, contributing to the prevention of RA (148).
FLS are crucial in the progression of RA, making them a target for potential treatments (149, 150). EVs from human GMSC have been shown to reduce arthritis progression by decreasing the invasiveness of synovial fibroblasts and protecting cartilage, suggesting therapeutic benefits for RA (71, 151). Additionally, EVs from human umbilical cord MSCs containing miR-451a inhibit the proliferation, migration, and invasion of RA synovial fibroblasts, improving arthritis in rat models (152). BMSC-derived EVs elevated miR-34a levels, reducing RA inflammation and inhibiting RA-FLS proliferation by targeting the cyclin I/p53/ataxia-telangiectasia mutated signaling pathway (153).
MSC-derived EVs infused with curcumin effectively regulate the proliferation and inflammatory response of RA-FLS, significantly reducing anti-apoptotic proteins and inflammatory mediators (154). EVs from IFN-β-primed MSCs also inhibit RA-FLS migration and surface marker expression, showing therapeutic potential for RA (82). Additionally, transfected MSC EVs carrying the lncRNA HAND2-AS1 downregulate the pathogenic miR-143-3p, inhibiting RA-FLS proliferation and motility while inducing apoptosis in in vitro experiments (155).
A drug delivery system using adipose tissue-derived MSC EVs successfully delivered icariin to joints, reducing arthritis in rats with CIA by shifting macrophage polarization from pro-inflammatory M1 to anti-inflammatory M2 (156). These EVs enhanced therapeutic effectiveness by modulating macrophage diversity, especially when the MSCs were metabolically engineered to modify EV surface properties (157). Engineering modifications also improved the bone-targeting ability of MSC-EVs, reducing systemic side effects and increasing their clinical application potential (158).
5 Conclusion and future perspectives
EVs can modulate innate and adaptive immune responses in experimental RA models. They can transfer different noncoding RNA molecules that regulate gene expression of recipient cells. Molecular EV-cargos, including miRNAs, lncRNAs, mRNAs, and differentially expressed proteins, hold great potential as biomarkers for diagnosing RA. Additionally, MSC-EVs containing various types of miRNAs, lncRNAs, and circRNAs suppressed inflammation and the pathogenic activities of FLS in RA. EVs can also serve as carriers for existing medications. In summary, EVs can inhibit RA immunopathogenesis, reduce the disease’s progression, and serve as promising biomarkers for its diagnosis. Nonetheless, additional research including gene enrichment and pathway analysis is required to detect changes in key signaling pathways and immunoregulatory networks in immune cells exposed to EVs. This will help to completely unravel the molecular mechanisms underlying the immunomodulatory effects of EV cargos in RA.
Author contributions
DA: Conceptualization, Supervision, Writing – original draft, Writing – review & editing. YA: Conceptualization, Supervision, Writing – original draft, Writing – review & editing. AAd: Conceptualization, Writing – original draft, Writing – review & editing. ZT: Writing – review & editing. BT: Writing – review & editing. AF: Writing – review & editing. BS: Writing – review & editing. GA: Writing – review & editing. MG: Writing – review & editing. MJ: Writing – review & editing. TB: Writing – review & editing. AAt: Conceptualization, Writing – original draft, Writing – review & editing.
Funding
The author(s) declare that no financial support was received for the research, authorship, and/or publication of this article.
Conflict of interest
The authors declare that the research was conducted in the absence of any commercial or financial relationships that could be construed as a potential conflict of interest.
Publisher’s note
All claims expressed in this article are solely those of the authors and do not necessarily represent those of their affiliated organizations, or those of the publisher, the editors and the reviewers. Any product that may be evaluated in this article, or claim that may be made by its manufacturer, is not guaranteed or endorsed by the publisher.
References
1. Kalluri R, LeBleu VS. The biology, function, and biomedical applications of exosomes. Science. (2020) 367(6478):. doi: 10.1126/science.aau6977
2. Tian J, Casella G, Zhang Y, Rostami A, Li X. Potential roles of extracellular vesicles in the pathophysiology, diagnosis, and treatment of autoimmune diseases. Int J Biol Sci. (2020) 16:620–32. doi: 10.7150/ijbs.39629
3. Barile L, Vassalli G. Exosomes: Therapy delivery tools and biomarkers of diseases. Pharmacol Ther. (2017) 174:63–78. doi: 10.1016/j.pharmthera.2017.02.020
4. Wu Y, Fu H, Hao J, Yang Z, Qiao X, Li Y, et al. Tumor-derived exosomal PD-L1: a new perspective in PD-1/PD-L1 therapy for lung cancer. Front Immunol. (2024) 15:1342728. doi: 10.3389/fimmu.2024.1342728
5. Bakinowska E, Kiełbowski K. The role of extracellular vesicles in the pathogenesis and treatment of rheumatoid arthritis and osteoarthritis. Cells. (2023) 12(23):. doi: 10.3390/cells12232716
6. Morrison TJ, Jackson MV, Cunningham EK, Kissenpfennig A, McAuley DF, O'Kane CM, et al. Mesenchymal stromal cells modulate macrophages in clinically relevant lung injury models by extracellular vesicle mitochondrial transfer. Am J Respir Crit Care Med. (2017) 196(10):1275–86. doi: 10.1164/rccm.201701-0170OC
7. Skotland T, Sagini K, Sandvig K, Llorente A. An emerging focus on lipids in extracellular vesicles. Adv Drug Delivery Rev. (2020) 159:308–21. doi: 10.1016/j.addr.2020.03.002
8. Welsh JA, Goberdhan DCI. Minimal information for studies of extracellular vesicles (MISEV2023): From basic to advanced approaches. J Extracell Vesicles. (2024) 13(2):. doi: 10.1002/jev2.12416
9. Vella LJ, Scicluna BJ. A rigorous method to enrich for exosomes from brain tissue. J Extracell Vesicles. (2017) 6(1):1348885. doi: 10.1080/20013078.2017.1348885
10. Admyre C, Johansson SM, Qazi KR, Filén JJ, Lahesmaa R, Norman M, et al. Exosomes with immune modulatory features are present in human breast milk. J Immunol. (2007) 179:1969–78. doi: 10.4049/jimmunol.179.3.1969
11. Esa A, Connolly KD, Williams R, Archer CW. Extracellular vesicles in the synovial joint: is there a role in the pathophysiology of osteoarthritis? Malays Orthop J. (2019) 13:1–7. doi: 10.5704/MOJ.1903.012
12. Han P, Bartold PM. Salivary outer membrane vesicles and DNA methylation of small extracellular vesicles as biomarkers for periodontal status: A pilot study. Int J Mol Sci. (2021) 22(5). doi: 10.3390/ijms22052423
13. Wu A, Wolley MJ, Fenton RA, Stowasser M. Using human urinary extracellular vesicles to study physiological and pathophysiological states and regulation of the sodium chloride cotransporter. Front Endocrinol (Lausanne). (2022) 13:981317. doi: 10.3389/fendo.2022.981317
14. Alberro A, Iparraguirre L. Extracellular vesicles in blood: sources, effects, and applications. Int J Mol Sci. (2021) 22(15). doi: 10.3390/ijms22158163
15. Aloi N, Drago G. Extracellular vesicles and immunity: at the crossroads of cell communication. Int J Mol Sci. (2024) 25(2). doi: 10.3390/ijms25021205
16. Bandini S, Ulivi P, Rossi T. Extracellular vesicles, circulating tumor cells, and immune checkpoint inhibitors: hints and promises. Cells. (2024) 13(4). doi: 10.3390/cells13040337
17. Xie S, Zhang Q, Jiang L. Current knowledge on exosome biogenesis, cargo-sorting mechanism and therapeutic implications. Membranes (Basel). (2022) 12(5). doi: 10.3390/membranes12050498
18. Han QF, Li WJ, Hu KS, Gao J, Zhai WL, Yang JH, et al. Exosome biogenesis: machinery, regulation, and therapeutic implications in cancer. Mol Cancer. (2022) 21:207. doi: 10.1186/s12943-022-01671-0
19. Li S. The basic characteristics of extracellular vesicles and their potential application in bone sarcomas. J Nanobiotechnology. (2021) 19:277. doi: 10.1186/s12951-021-01028-7
20. Lim HJ, Yoon H, Kim H, Kang YW, Kim JE, Kim OY, et al. Extracellular vesicle proteomes shed light on the evolutionary, interactive, and functional divergence of their biogenesis mechanisms. Front Cell Dev Biol. (2021) 9:734950. doi: 10.3389/fcell.2021.734950
21. Battistelli M, Falcieri E. Apoptotic bodies: particular extracellular vesicles involved in intercellular communication. Biol (Basel). (2020) 9. doi: 10.3390/biology9010021
22. Dieudé M, Bell C, Turgeon J, Beillevaire D, Pomerleau L, Yang B, et al. The 20S proteasome core, active within apoptotic exosome-like vesicles, induces autoantibody production and accelerates rejection. Sci Transl Med. (2015) 7:318ra200. doi: 10.1126/scitranslmed.aac9816
23. Joseph J, Rahmani B, Cole Y, Puttagunta N, Lin E, Khan ZK, et al. Can soluble immune checkpoint molecules on exosomes mediate inflammation? J Neuroimmune Pharmacol. (2022) 17(3-4):381–97. doi: 10.1007/s11481-021-10018-3
24. Yin Z, Yu M, Ma T, Zhang C, Huang S, Karimzadeh MR, et al. Mechanisms underlying low-clinical responses to PD-1/PD-L1 blocking antibodies in immunotherapy of cancer: a key role of exosomal PD-L1. J Immunother Cancer. (2021) 9(1). doi: 10.1136/jitc-2020-001698
25. Wang M, Zhang B. The immunomodulation potential of exosomes in tumor microenvironment. J Immunol Res. (2021) 2021:3710372. doi: 10.1155/2021/3710372
26. Jung I, Shin S, Baek MC. Modification of immune cell-derived exosomes for enhanced cancer immunotherapy: current advances and therapeutic applications. Exp Mol Med. (2024) 56(1):19–31. doi: 10.1038/s12276-023-01132-8
27. Lyu C, Sun H, Sun Z. Roles of exosomes in immunotherapy for solid cancers. Cell Death Dis. (2024) 15(2):106. doi: 10.1038/s41419-024-06494-z
28. Ma Y, Zhou Y, Zou SS, Sun Y. Exosomes released from Sertoli cells contribute to the survival of Leydig cells through CCL20 in rats. Mol Hum Reprod. (2022) 28(2). doi: 10.1093/molehr/gaac002
29. Zhang B, Zhao M, Lu Q. Extracellular vesicles in rheumatoid arthritis and systemic lupus erythematosus: functions and applications. Front Immunol. (2020) 11:575712. doi: 10.3389/fimmu.2020.575712
30. Ovchinnikova LA, Zalevsky AO. Extracellular vesicles in chronic demyelinating diseases: prospects in treatment and diagnosis of autoimmune neurological disorders. Life (Basel). (2022) 12(11). doi: 10.3390/life12111943
31. Suire CN, Hade MD. Extracellular vesicles in type 1 diabetes: A versatile tool. Bioengineering (Basel). (2022) 9(3). doi: 10.3390/bioengineering9030105
32. Smolen JS, Aletaha D, McInnes IB. Rheumatoid arthritis. Lancet. (2016) 388:2023–38. doi: 10.1016/S0140-6736(16)30173-8
33. Aletaha D, Smolen JS. Diagnosis and management of rheumatoid arthritis: A review. Jama. (2018) 320:1360–72. doi: 10.1001/jama.2018.13103
34. Alcaraz MJ, Guillén MI. Cellular and molecular targets of extracellular vesicles from mesenchymal stem/stromal cells in rheumatoid arthritis. Stem Cells Transl Med. (2022) 11:1177–85. doi: 10.1093/stcltm/szac075
35. Liu H, Li R, Liu T, Yang L, Yin G, Xie Q. Immunomodulatory effects of mesenchymal stem cells and mesenchymal stem cell-derived extracellular vesicles in rheumatoid arthritis. Front Immunol. (2020) 11:1912. doi: 10.3389/fimmu.2020.01912
36. Favalli EG, Raimondo MG, Becciolini A, Crotti C, Biggioggero M, Caporali R. The management of first-line biologic therapy failures in rheumatoid arthritis: Current practice and future perspectives. Autoimmun Rev. (2017) 16:1185–95. doi: 10.1016/j.autrev.2017.10.002
37. Feldmann M, Maini RN. Discovery of TNF-alpha as a therapeutic target in rheumatoid arthritis: preclinical and clinical studies. Joint Bone Spine. (2002) 69:12–8. doi: 10.1016/S1297-319X(01)00335-9
38. Tian X, Wei W, Cao Y, Ao T, Huang F, Javed R, et al. Gingival mesenchymal stem cell-derived exosomes are immunosuppressive in preventing collagen-induced arthritis. J Cell Mol Med. (2022) 26(3):693–708. doi: 10.1111/jcmm.17086
39. Masoumi M, Alesaeidi S, Khorramdelazad H, Behzadi M, Baharlou R, Alizadeh-Fanalou S, et al. Role of T cells in the pathogenesis of rheumatoid arthritis: focus on immunometabolism dysfunctions. Inflammation. (2023) 46:88–102. doi: 10.1007/s10753-022-01751-9
40. Singh A, Behl T, Sehgal A, Singh S, Sharma N, Naved T, et al. Mechanistic insights into the role of B cells in rheumatoid arthritis. Int Immunopharmacol. (2021) 99:108078. doi: 10.1016/j.intimp.2021.108078
41. Kuo D, Ding J. HBEGF(+) macrophages in rheumatoid arthritis induce fibroblast invasiveness. Sci Transl Med. (2019) 11(491). doi: 10.1126/scitranslmed.aau8587
42. Min HK, Kim KW, Lee SH, Kim HR. Roles of mast cells in rheumatoid arthritis. Korean J Intern Med. (2020) 35:12–24. doi: 10.3904/kjim.2019.271
43. Yang P, Qian FY, Zhang MF, Xu AL, Wang X, Jiang BP, et al. Th17 cell pathogenicity and plasticity in rheumatoid arthritis. J Leukoc Biol. (2019) 106:1233–40. doi: 10.1002/JLB.4RU0619-197R
44. Ross EA, Devitt A, Johnson JR. Macrophages: the good, the bad, and the gluttony. Front Immunol. (2021) 12:708186. doi: 10.3389/fimmu.2021.708186
45. Chemin K, Gerstner C, Malmström V. Effector functions of CD4+ T cells at the site of local autoimmune inflammation-lessons from rheumatoid arthritis. Front Immunol. (2019) 10:353. doi: 10.3389/fimmu.2019.00353
46. Luo P, Wang P. Immunomodulatory role of T helper cells in rheumatoid arthritis: a comprehensive research review. Bone Joint Res. (2022) 11(7):426–38. doi: 10.1302/2046-3758.117.BJR-2021-0594.R1
47. Siouti E, Andreakos E. The many facets of macrophages in rheumatoid arthritis. Biochem Pharmacol. (2019) 165:152–69. doi: 10.1016/j.bcp.2019.03.029
48. Li C, Han Y, Luo X, Qian C, Li Y, Su H, et al. Immunomodulatory nano-preparations for rheumatoid arthritis. Drug Delivery. (2023) 30:9–19. doi: 10.1080/10717544.2022.2152136
49. Jouybari MT, Mojtahedi F, Babaahmadi M, Faeed M, Eslaminejad MB, Taghiyar L. Advancements in extracellular vesicle targeted therapies for rheumatoid arthritis: insights into cellular origins, current perspectives, and emerging challenges. Stem Cell Res Ther. (2024) 15(1):276. doi: 10.1186/s13287-024-03887-x
50. Lindenbergh MFS, Stoorvogel W. Antigen presentation by extracellular vesicles from professional antigen-presenting cells. Annu Rev Immunol. (2018) 36:435–59. doi: 10.1146/annurev-immunol-041015-055700
51. Ahmed SF, Jasim SA, Pallathadka H, Kaur H, Renuka Jyothi S, Bansal P, et al. New Therapeutic Strategies for the Inflammatory Rheumatoid Arthritis Disease: Emphasizing Mesenchymal Stem Cells and Associated exo-miRNA or exo-lncRNA. Cell Biochem Biophys. (2024) 82:1599–611. doi: 10.1007/s12013-024-01316-7
52. Di Trapani M, Bassi G, Midolo M, Gatti A, Kamga PT, Cassaro A, et al. Differential and transferable modulatory effects of mesenchymal stromal cell-derived extracellular vesicles on T, B and NK cell functions. Sci Rep. (2016) 6:24120. doi: 10.1038/srep24120
53. Shen Z, Huang W, Liu J, Tian J, Wang S, Rui K. Effects of mesenchymal stem cell-derived exosomes on autoimmune diseases. Front Immunol. (2021) 12:749192. doi: 10.3389/fimmu.2021.749192
54. Liu X, Wei Q, Lu L, Cui S, Ma K, Zhang W, et al. Immunomodulatory potential of mesenchymal stem cell-derived extracellular vesicles: Targeting immune cells. Front Immunol. (2023) 14:1094685. doi: 10.3389/fimmu.2023.1094685
55. Liao HJ, Hsu PN. Immunomodulatory effects of extracellular vesicles from mesenchymal stromal cells: Implication for therapeutic approach in autoimmune diseases. Kaohsiung J Med Sci. (2024) 40(6):520–9. doi: 10.1002/kjm2.12841
56. Wang S, Lei B, Zhang E, Gong P, Gu J, He L, et al. Targeted therapy for inflammatory diseases with mesenchymal stem cells and their derived exosomes: from basic to clinics. Int J Nanomedicine. (2022) 17:1757–81. doi: 10.2147/IJN.S355366
57. Chen Z, Larregina AT, Morelli AE. Impact of extracellular vesicles on innate immunity. Curr Opin Organ Transplant. (2019) 24:670–8. doi: 10.1097/MOT.0000000000000701
58. Yang S, Zhao M, Jia S. Macrophage: Key player in the pathogenesis of autoimmune diseases. Front Immunol. (2023) 14:1080310. doi: 10.3389/fimmu.2023.1080310
59. Arabpour M, Saghazadeh A, Rezaei N. Anti-inflammatory and M2 macrophage polarization-promoting effect of mesenchymal stem cell-derived exosomes. Int Immunopharmacol. (2021) 97:107823. doi: 10.1016/j.intimp.2021.107823
60. Harrell CR, Jovicic N. Mesenchymal stem cell-derived exosomes and other extracellular vesicles as new remedies in the therapy of inflammatory diseases. Cells. (2019) 8(12). doi: 10.3390/cells8121605
61. Xu D, et al. Exosome-encapsulated miR-6089 regulates inflammatory response via targeting TLR4. J Cell Physiol. (2019) 234(2):1502–11. doi: 10.1002/jcp.v234.2
62. Kim H, Back JH, Han G, Lee SJ, Park YE, Gu MB, et al. Extracellular vesicle-guided in situ reprogramming of synovial macrophages for the treatment of rheumatoid arthritis. Biomaterials. (2022) 286:121578. doi: 10.1016/j.biomaterials.2022.121578
63. Rhys HI, Dell'Accio F, Pitzalis C, Moore A, Norling LV, Perretti M. Neutrophil microvesicles from healthy control and rheumatoid arthritis patients prevent the inflammatory activation of macrophages. EBioMedicine. (2018) 29:60–9. doi: 10.1016/j.ebiom.2018.02.003
64. Zhang B, Lai RC. Therapeutic efficacy of mesenchymal stem/stromal cell small extracellular vesicles in alleviating arthritic progression by restoring macrophage balance. Int J Mol Sci. (2023) 13. doi: 10.3390/biom13101501
65. Huang Y, Lu D, Ma W, Liu J, Ning Q, Tang F, et al. miR-223 in exosomes from bone marrow mesenchymal stem cells ameliorates rheumatoid arthritis via downregulation of NLRP3 expression in macrophages. Mol Immunol. (2022) 143:68–76. doi: 10.1016/j.molimm.2022.01.002
66. Reis M, Mavin E, Nicholson L, Green K, Dickinson AM, Wang XN. Mesenchymal stromal cell-derived extracellular vesicles attenuate dendritic cell maturation and function. Front Immunol. (2018) 9:2538. doi: 10.3389/fimmu.2018.02538
67. Pozsgay J, Szekanecz Z, Sármay G. Antigen-specific immunotherapies in rheumatic diseases. Nat Rev Rheumatol. (2017) 13:525–37. doi: 10.1038/nrrheum.2017.107
68. Rincón-Arévalo H, Burbano C, Atehortúa L, Rojas M, Vanegas-García A, Vásquez G, et al. Modulation of B cell activation by extracellular vesicles and potential alteration of this pathway in patients with rheumatoid arthritis. Arthritis Res Ther. (2022) 24(1):169. doi: 10.1186/s13075-022-02837-3
69. Cosenza S, Toupet K, Maumus M, Luz-Crawford P, Blanc-Brude O, Jorgensen C, et al. Mesenchymal stem cells-derived exosomes are more immunosuppressive than microparticles in inflammatory arthritis. Theranostics. (2018) 8:1399–410. doi: 10.7150/thno.21072
70. Wu X, Zhu D, Tian J, Tang X, Guo H, Ma J, et al. Granulocytic myeloid-derived suppressor cell exosomal prostaglandin E2 ameliorates collagen-induced arthritis by enhancing IL-10(+) B cells. Front Immunol. (2020) 11:588500. doi: 10.3389/fimmu.2020.588500
71. Chen J, Shi X, Deng Y, Dang J, Liu Y, Zhao J, et al. miRNA-148a-containing GMSC-derived EVs modulate Treg/Th17 balance via IKKB/NF-κB pathway and treat a rheumatoid arthritis model. JCI Insight. (2024) 9. doi: 10.1172/jci.insight.177841
72. Zhu D, Tian J, Wu X, Li M, Tang X, Rui K, et al. G-MDSC-derived exosomes attenuate collagen-induced arthritis by impairing Th1 and Th17 cell responses. Biochim Biophys Acta Mol Basis Dis. (2019) 1865:165540. doi: 10.1016/j.bbadis.2019.165540
73. Ma D, Xu K, Zhang G, Liu Y, Gao J, Tian M, et al. Immunomodulatory effect of human umbilical cord mesenchymal stem cells on T lymphocytes in rheumatoid arthritis. Int Immunopharmacol. (2019) 74:105687. doi: 10.1016/j.intimp.2019.105687
74. Xu K, Ma D, Zhang G, Gao J, Su Y, Liu S, et al. Human umbilical cord mesenchymal stem cell-derived small extracellular vesicles ameliorate collagen-induced arthritis via immunomodulatory T lymphocytes. Mol Immunol. (2021) 135:36–44. doi: 10.1016/j.molimm.2021.04.001
75. Fu Y, Li J, Zhang Z, Ren F, Wang Y, Jia H, et al. Umbilical cord mesenchymal stem cell-derived exosomes alleviate collagen-induced arthritis by balancing the population of Th17 and regulatory T cells. FEBS Lett. (2022) 596(20):2668–77. doi: 10.1002/1873-3468.14460
76. Franco da Cunha F, Andrade-Oliveira V, Candido de Almeida D, Borges da Silva T, Naffah de Souza Breda C, Costa Cruz M, et al. Extracellular vesicles isolated from mesenchymal stromal cells modulate CD4(+) T lymphocytes toward a regulatory profile. Cells. (2020) 9(4). doi: 10.3390/cells9041059
77. Lee S, Kim S, Chung H, Moon JH, Kang SJ, Park CG. Mesenchymal stem cell-derived exosomes suppress proliferation of T cells by inducing cell cycle arrest through p27kip1/Cdk2 signaling. Immunol Lett. (2020) 225:16–22. doi: 10.1016/j.imlet.2020.06.006
78. Ren Z, Liu X, Abdollahi E, Tavasolian F. Genetically engineered exosomes as a potential regulator of th1 cells response in rheumatoid arthritis. Biopreserv Biobank. (2023) 21(4):355–66. doi: 10.1089/bio.2022.0003
79. Tavasolian F, Hosseini AZ, Soudi S, Naderi M. miRNA-146a improves immunomodulatory effects of MSC-derived exosomes in rheumatoid arthritis. Curr Gene Ther. (2020) 20:297–312. doi: 10.2174/1566523220666200916120708
80. Hyland M, Mennan C, Davies R. Extracellular vesicles derived from umbilical cord mesenchymal stromal cells show enhanced anti-inflammatory properties via upregulation of miRNAs after pro-inflammatory priming. Stem Cell Rev Rep. (2023) 19(7):2391–406. doi: 10.1007/s12015-023-10586-2
81. Kay AG, Treadwell K, Roach P, Morgan R, Lodge R, Hyland M. Therapeutic effects of hypoxic and pro-inflammatory priming of mesenchymal stem cell-derived extracellular vesicles in inflammatory arthritis. Int J Mol Sci. (2021) 23(1). doi: 10.3390/ijms23010126
82. Tsiapalis D, Floudas A. Therapeutic effects of mesenchymal/stromal stem cells and their derived extracellular vesicles in rheumatoid arthritis. Sci Adv. (2023) 12:849–62. doi: 10.1093/stcltm/szad065
83. Choi EW, Lim IR, Park JH, Song J, Choi B, Kim S. Exosomes derived from mesenchymal stem cells primed with disease-condition-serum improved therapeutic efficacy in a mouse rheumatoid arthritis model via enhanced TGF-β1 production. Stem Cell Res Ther. (2023) 14:283. doi: 10.1186/s13287-023-03523-0
84. Chu X, Chen L, Zhang Y. Exosomes derived from PMN-MDSCs preconditioned by hypoxia attenuate arthropathy of collagen-induced arthritis mice. Xi Bao Yu Fen Zi Mian Yi Xue Za Zhi. (2021) 37:728–35.
85. Lee HI, Ahn MJ, Yoo JK, Ahn SH, Park SY, Seo H, et al. Exosome-mediated delivery of super-repressor IκBα alleviates inflammation and joint damages in rheumatoid arthritis. Arthritis Res Ther. (2024) 26(1):2. doi: 10.1186/s13075-023-03225-1
86. Chen J, Huang F, Hou Y, Lin X, Liang R, Hu X, et al. TGF-β-induced CD4+ FoxP3+ regulatory T cell-derived extracellular vesicles modulate Notch1 signaling through miR-449a and prevent collagen-induced arthritis in a murine model. Cell Mol Immunol. (2021) 18:2516–29. doi: 10.1038/s41423-021-00764-y
87. Lin M, Lei S, Chai Y, Xu J, Wang Y, Wu C, et al. Immunosuppressive microvesicles-mimetic derived from tolerant dendritic cells to target T-lymphocytes for inflammation diseases therapy. J Nanobiotechnology. (2024) 22:201. doi: 10.1186/s12951-024-02470-z
88. Greisen SR, Yan Y, Hansen AS, Venø MT, Nyengaard JR, Moestrup SK, et al. Extracellular vesicles transfer the receptor programmed death-1 in rheumatoid arthritis. Front Immunol. (2017) 8:851. doi: 10.3389/fimmu.2017.00851
89. Shenoy GN, Bhatta M. Exosomes represent an immune suppressive T cell checkpoint in human chronic inflammatory microenvironments. Immunol Invest. (2020) 49(7):726–43. doi: 10.1080/08820139.2020.1748047
90. Schioppo T, Ubiali T. The role of extracellular vesicles in rheumatoid arthritis: a systematic review. Clin Rheumatol. (2021) 40(9):3481–97. doi: 10.1007/s10067-021-05614-w
91. Oba R, Isomura M, Igarashi A, Nagata K. Circulating CD3(+)HLA-DR(+) extracellular vesicles as a marker for th1/tc1-type immune responses. J Immunol Res. (2019) 2019:6720819. doi: 10.1155/2019/6720819
92. Xue L, Wang B, Li X, Zhu J, Wang W, Huang F, et al. Comprehensive analysis of serum exosome-derived lncRNAs and mRNAs from patients with rheumatoid arthritis. Arthritis Res Ther. (2023) 25:201. doi: 10.1186/s13075-023-03174-9
93. Heydari R, Koohi F, Rasouli M. Exosomes as rheumatoid arthritis diagnostic biomarkers and therapeutic agents. Vaccines (Basel). (2023) 11(3). doi: 10.3390/vaccines11030687
94. Liu H, Chen Y, Yin G, Xie Q. Therapeutic prospects of MicroRNAs carried by mesenchymal stem cells-derived extracellular vesicles in autoimmune diseases. Life Sci. (2021) 277:119458. doi: 10.1016/j.lfs.2021.119458
95. Letonja J, Petrovič D. A review of microRNAs and lncRNAs in atherosclerosis as well as some major inflammatory conditions affecting atherosclerosis. Biomedicines. (2024) 12(6). doi: 10.3390/biomedicines12061322
96. Maeda Y, Farina NH, Matzelle MM, Fanning PJ, Lian JB, Gravallese EM. Synovium-derived microRNAs regulate bone pathways in rheumatoid arthritis. J Bone Miner Res. (2017) 32:461–72. doi: 10.1002/jbmr.3005
97. Foers AD. Extracellular vesicles in synovial fluid from rheumatoid arthritis patients contain miRNAs with capacity to modulate inflammation. Cell Mol Life Sci. (2021) 22. doi: 10.3390/ijms22094910
98. Adugna A, Muche Y, Melkamu A, Jemal M, Belew H, Amare GA. Current updates on the molecular and genetic signals as diagnostic and therapeutic targets for hepatitis B virus-associated hepatic Malignancy. Heliyon. (2024) 10:e34288. doi: 10.1016/j.heliyon.2024.e34288
99. Li N, Chen Z, Feng W, Gong Z, Lin C, Chen J, et al. Triptolide improves chondrocyte proliferation and secretion via down-regulation of miR-221 in synovial cell exosomes. Phytomedicine. (2022) 107:154479. doi: 10.1016/j.phymed.2022.154479
100. Maunder D, Brown PM, Barron-Millar B, Lendrem DW, Naamane N, Macdonald J, et al. Micro-RNA content of circulating extracellular vesicles in early rheumatoid arthritis as biomarkers and mediators of methotrexate efficacy. Rheumatology (Oxford). (2023) 63:2259–67. doi: 10.1093/rheumatology/kead104.155
101. Yu Y, Park S, Lee H, Kwon EJ, Park HR, Kim YH, et al. Exosomal hsa-miR-335-5p and hsa-miR-483-5p are novel biomarkers for rheumatoid arthritis: A development and validation study. Int Immunopharmacol. (2023) 120:110286. doi: 10.1016/j.intimp.2023.110286
102. Lim MK, Yoo J, Sheen DH, Ihm C, Lee SK, Kim SA. Serum exosomal miRNA-1915-3p is correlated with disease activity of korean rheumatoid arthritis. In Vivo. (2020) 34:2941–5. doi: 10.21873/invivo.12124
103. Takamura Y, Aoki W, Satomura A, Shibasaki S, Ueda M. Small RNAs detected in exosomes derived from the MH7A synovial fibroblast cell line with TNF-α stimulation. PLoS One. (2018) 13(8). doi: 10.1371/journal.pone.0201851
104. Wielińska J, Crossland RE. Exploring the extracellular vesicle microRNA expression repertoire in patients with rheumatoid arthritis and ankylosing spondylitis treated with TNF inhibitors. Dis Markers. (2021) 2021:2924935. doi: 10.1155/2021/2924935
105. Rodríguez-Muguruza S, Altuna-Coy A, Castro-Oreiro S, Poveda-Elices MJ, Fontova-Garrofé R, Chacón MR. A Serum Biomarker Panel of exomiR-451a, exomiR-25-3p and Soluble TWEAK for Early Diagnosis of Rheumatoid Arthritis. Front Immunol. (2021) 12:790880. doi: 10.3389/fimmu.2021.790880
106. Karimi B, Dehghani Firoozabadi A, Peymani M, Ghaedi K. Circulating long noncoding RNAs as novel bio-tools: Focus on autoimmune diseases. Hum Immunol. (2022) 83:618–27. doi: 10.1016/j.humimm.2022.06.001
107. Sun S, Liang L, Tian R, Huang Q, Ji Z, Li X, et al. LncRNA expression profiling in exosomes derived from synovial fluid of patients with rheumatoid arthritis. Int Immunopharmacol. (2024) 130:111735. doi: 10.1016/j.intimp.2024.111735
108. Li G, Fang Y, Xu N, Ding Y, Liu D. Fibroblast-like synoviocytes-derived exosomal circFTO deteriorates rheumatoid arthritis by enhancing N6-methyladenosine modification of SOX9 in chondrocytes. Arthritis Res Ther. (2024) 26:56. doi: 10.1186/s13075-024-03290-0
109. Wu H, Chen Q, Wang S, Yang C, Xu L, Xiao H, et al. Serum exosomes lncRNAs: TCONS_I2_00013502 and ENST00000363624 are new diagnostic markers for rheumatoid arthritis. Front Immunol. (2024) 15:1419683. doi: 10.3389/fimmu.2024.1419683
110. Shuai ZQ, Wang ZX, Ren JL, Yang XK, Xu B. Differential expressions and potential clinical values of lncRNAs in the plasma exosomes of rheumatoid arthritis. Int Immunopharmacol. (2024) 128:111511. doi: 10.1016/j.intimp.2024.111511
111. Huang Y, Liu Y, Huang Q, Sun S, Ji Z, Huang L, et al. TMT-based quantitative proteomics analysis of synovial fluid-derived exosomes in inflammatory arthritis. Front Immunol. (2022) 13:800902. doi: 10.3389/fimmu.2022.800902
112. Nakamachi Y, Uto K, Hayashi S, Okano T, Morinobu A, Kuroda R, et al. Exosomes derived from synovial fibroblasts from patients with rheumatoid arthritis promote macrophage migration that can be suppressed by miR-124-3p. Heliyon. (2023) 9:e14986. doi: 10.1016/j.heliyon.2023.e14986
113. Qin Q, Song R. Systemic proteomic analysis reveals distinct exosomal protein profiles in rheumatoid arthritis. J Immunol Res. (2021) 2021:9421720. doi: 10.1155/2021/9421720
114. Huang L, Liang L, Ji Z, Chen S, Liu M, Huang Q, et al. Proteomics profiling of CD4 + T-cell-derived exosomes from patients with rheumatoid arthritis. Int Immunopharmacol. (2023) 122:110560. doi: 10.1016/j.intimp.2023.110560
115. Arntz OJ, Pieters BCH, Thurlings RM, Wenink MH, van Lent P, Koenders MI, et al. Rheumatoid arthritis patients with circulating extracellular vesicles positive for igM rheumatoid factor have higher disease activity. Front Immunol. (2018) 9:2388. doi: 10.3389/fimmu.2018.02388
116. Burbano C, Rojas M, Muñoz-Vahos C, Vanegas-García A. Extracellular vesicles are associated with the systemic inflammation of patients with seropositive rheumatoid arthritis. Sci Rep. (2018) 8. doi: 10.1038/s41598-018-36335-x
117. Arntz OJ, Thurlings RM, Blaney Davidson EN, Jansen P, Vermeulen M, Koenders MI, et al. Profiling of plasma extracellular vesicles identifies proteins that strongly associate with patient's global assessment of disease activity in rheumatoid arthritis. Front Med (Lausanne). (2023) 10:1247778. doi: 10.3389/fmed.2023.1247778
118. Ucci FM, Recalchi S, Barbati C, Manganelli V, Capozzi A, Riitano G, et al. Citrullinated and carbamylated proteins in extracellular microvesicles from plasma of patients with rheumatoid arthritis. Rheumatol (Oxford). (2023) 62:2312–9. doi: 10.1093/rheumatology/keac598
119. Buzas EI. The roles of extracellular vesicles in the immune system. Nat Rev Immunol. (2023) 23:236–50. doi: 10.1038/s41577-022-00763-8
120. Curran AM, Girgis AA. Citrullination modulates antigen processing and presentation by revealing cryptic epitopes in rheumatoid arthritis. Nat Commun. (2023) 14(1):1061. doi: 10.1038/s41467-023-36620-y
121. van Delft MAM, Huizinga TWJ. An overview of autoantibodies in rheumatoid arthritis. J Autoimmun. (2020) 110:102392. doi: 10.1016/j.jaut.2019.102392
122. Riitano G, Recalchi S, Capozzi A, Manganelli V, Misasi R, Garofalo T. The role of autophagy as a trigger of post-translational modifications of proteins and extracellular vesicles in the pathogenesis of rheumatoid arthritis. Int J Mol Sci. (2023) 24(16). doi: 10.3390/ijms241612764
123. Vomero M, Barbati C, Colasanti T, Perricone C, Novelli L, Ceccarelli F, et al. Autophagy and rheumatoid arthritis: current knowledges and future perspectives. Front Immunol. (2018) 9:1577. doi: 10.3389/fimmu.2018.01577
124. Sugawara E, Kato M, Kudo Y, Lee W. Autophagy promotes citrullination of VIM (vimentin) and its interaction with major histocompatibility complex class II in synovial fibroblasts. Autophagy. (2020) 16(5):946–55. doi: 10.1080/15548627.2019.1664144
125. Gudbergsson JM, Johnsen KB. Exosomes and autophagy: rekindling the vesicular waste hypothesis. J Cell Commun Signal. (2019) 13:443–50. doi: 10.1007/s12079-019-00524-8
126. Alghamdi M, Alamry SA, Bahlas SM, Uversky VN, Redwan EM. Circulating extracellular vesicles and rheumatoid arthritis: a proteomic analysis. Cell Mol Life Sci. (2021) 79(1):25. doi: 10.1007/s00018-021-04020-4
127. Wu LF, Zhang Q, Mo XB, Lin J, Wu YL, Lu X, et al. Identification of novel rheumatoid arthritis-associated MiRNA-204-5p from plasma exosomes. Exp Mol Med. (2022) 54:334–45. doi: 10.1038/s12276-022-00751-x
128. Lu J, Wu J, Zhang X, Zhong R, Wang B, Yang H, et al. Characterization of the MicroRNA profile in rheumatoid arthritis plasma exosomes and their roles in B-cell responses. Clinics (Sao Paulo). (2024) 79:100441. doi: 10.1016/j.clinsp.2024.100441
129. Gong J, Zhang X, Khan A, Liang J, Xiong T, Yang P, et al. Identification of serum exosomal miRNA biomarkers for diagnosis of Rheumatoid arthritis. Int Immunopharmacol. (2024) 129:111604. doi: 10.1016/j.intimp.2024.111604
130. Huldani H, Abdalkareem Jasim S, Olegovich Bokov D, Abdelbasset WK, Nader Shalaby M, Thangavelu L, et al. Application of extracellular vesicles derived from mesenchymal stem cells as potential therapeutic tools in autoimmune and rheumatic diseases. Int Immunopharmacol. (2022) 106:108634. doi: 10.1016/j.intimp.2022.108634
131. Fu H, Hu D, Zhang L, Tang P. Role of extracellular vesicles in rheumatoid arthritis. Clin Rheumatol. (2018) 93:125–32. doi: 10.1016/j.molimm.2017.11.016
132. Yan F, Zhong Z, Wang Y, Feng Y, Mei Z, Li H, et al. Exosome-based biomimetic nanoparticles targeted to inflamed joints for enhanced treatment of rheumatoid arthritis. J Nanobiotechnology. (2020) 18:115. doi: 10.1186/s12951-020-00675-6
133. Yang X, Xia H, Liu C, Wu Y, Liu X, Cheng Y, et al. The novel delivery-exosome application for diagnosis and treatment of rheumatoid arthritis. Pathol Res Pract. (2023) 242:154332. doi: 10.1016/j.prp.2023.154332
134. Liu H, Chen Y, Huang Y, Wei L, Ran J, Li Q, et al. Macrophage-derived mir-100-5p orchestrates synovial proliferation and inflammation in rheumatoid arthritis through mTOR signaling. J Nanobiotechnology. (2024) 22:197. doi: 10.1186/s12951-024-02444-1
135. Li H, Feng Y, Zheng X, Jia M, Mei Z, Wang Y, et al. M2-type exosomes nanoparticles for rheumatoid arthritis therapy via macrophage re-polarization. J Control Release. (2022) 341:16–30. doi: 10.1016/j.jconrel.2021.11.019
136. Takenaka M, Yabuta A, Takahashi Y, Takakura Y. Interleukin-4-carrying small extracellular vesicles with a high potential as anti-inflammatory therapeutics based on modulation of macrophage function. Biomaterials. (2021) 278:121160. doi: 10.1016/j.biomaterials.2021.121160
137. Tang Y, Wu Z, Guo R, Huang J, Rong X, Zhu B, et al. Ultrasound-augmented anti-inflammatory exosomes for targeted therapy in rheumatoid arthritis. J Mater Chem B. (2022) 10(38):7862–74. doi: 10.1039/D2TB01219G
138. Zhao C, Song W, Ma J, Wang N. Macrophage-derived hybrid exosome-mimic nanovesicles loaded with black phosphorus for multimodal rheumatoid arthritis therapy. Biomater Sci. (2022) 10:6731–9. doi: 10.1039/D2BM01274J
139. Li X, Li S, Fu X, Wang Y. Apoptotic extracellular vesicles restore homeostasis of the articular microenvironment for the treatment of rheumatoid arthritis. Cells. (2024) 35:564–76. doi: 10.1016/j.bioactmat.2023.11.019
140. Kim SH, Lechman ER, Bianco N, Menon R, Keravala A, Nash J, et al. Exosomes derived from IL-10-treated dendritic cells can suppress inflammation and collagen-induced arthritis. J Immunol. (2005) 174:6440–8. doi: 10.4049/jimmunol.174.10.6440
141. Bianco NR, Kim SH, Ruffner MA, Robbins PD. Therapeutic effect of exosomes from indoleamine 2,3-dioxygenase-positive dendritic cells in collagen-induced arthritis and delayed-type hypersensitivity disease models. Arthritis Rheum. (2009) 60:380–9. doi: 10.1002/art.24229
142. Shin TH, Kim HS, Kang TW, Lee BC, Lee HY, Kim YJ, et al. Human umbilical cord blood-stem cells direct macrophage polarization and block inflammasome activation to alleviate rheumatoid arthritis. Cell Death Dis. (2016) 7:e2524. doi: 10.1038/cddis.2016.442
143. Pistoia V, Raffaghello L. Mesenchymal stromal cells and autoimmunity. Int Immunol. (2017) 29:49–58. doi: 10.1093/intimm/dxx008
144. Yang JH, Liu FX, Wang JH, Cheng M, Wang SF, Xu DH. Mesenchymal stem cells and mesenchymal stem cell-derived extracellular vesicles: Potential roles in rheumatic diseases. World J Stem Cells. (2020) 12:688–705. doi: 10.4252/wjsc.v12.i7.688
145. Martinez-Arroyo O, Ortega A. Mesenchymal stem cell-derived extracellular vesicles as non-coding RNA therapeutic vehicles in autoimmune diseases. Pharmaceutics. (2022) 14(4). doi: 10.3390/pharmaceutics14040733
146. Kim IK, Kim SH, Choi SM, Youn BS, Kim HS. Extracellular vesicles as drug delivery vehicles for rheumatoid arthritis. Stem Cells Transl Med. (2016) 11:329–42. doi: 10.2174/1574888X11666151203223251
147. Liu S, Xu X, Liang S, Chen Z, Zhang Y, Qian A, et al. The application of MSCs-derived extracellular vesicles in bone disorders: novel cell-free therapeutic strategy. Front Cell Dev Biol. (2020) 8:619. doi: 10.3389/fcell.2020.00619
148. Zhang Y, Jiao Z, Wang S. Bone marrow mesenchymal stem cells release miR-378a-5p-carried extracellular vesicles to alleviate rheumatoid arthritis. J Innate Immun. (2023) 15:893–910. doi: 10.1159/000534830
149. You S, Koh JH, Leng L, Kim WU, Bucala R. The tumor-like phenotype of rheumatoid synovium: molecular profiling and prospects for precision medicine. Arthritis Rheumatol. (2018) 70:637–52. doi: 10.1002/art.40406
150. Pratt AG, Siebert S, Cole M, Stocken DD, Yap C, Kelly S, et al. Targeting synovial fibroblast proliferation in rheumatoid arthritis (TRAFIC): an open-label, dose-finding, phase 1b trial. Lancet Rheumatol. (2021) 3:e337–46. doi: 10.1016/S2665-9913(21)00061-8
151. Bruckner S, Capria VM, Zeno B, Leblebicioglu B, Goyal K, Vasileff WK, et al. The therapeutic effects of gingival mesenchymal stem cells and their exosomes in a chimeric model of rheumatoid arthritis. Arthritis Res Ther. (2023) 25:211. doi: 10.1186/s13075-023-03185-6
152. Mi L, Gao J, Li N, Liu Y, Zhang N, Gao Y, et al. Human umbilical cord mesenchymal stem cell-derived exosomes loaded miR-451a targets ATF2 to improve rheumatoid arthritis. Int Immunopharmacol. (2024) 127:111365. doi: 10.1016/j.intimp.2023.111365
153. Wu H, Zhou X, Wang X. miR-34a in extracellular vesicles from bone marrow mesenchymal stem cells reduces rheumatoid arthritis inflammation via the cyclin I/ATM/ATR/p53 axis. J Cell Mol Med. (2021) 25(4):1896–910. doi: 10.1111/jcmm.15857
154. He X, Zhang C, Amirsaadat S, Jalil AT, Kadhim MM, Abasi M, et al. Curcumin-loaded mesenchymal stem cell-derived exosomes efficiently attenuate proliferation and inflammatory response in rheumatoid arthritis fibroblast-like synoviocytes. Appl Biochem Biotechnol. (2023) 195:51–67. doi: 10.1007/s12010-022-04090-5
155. Su Y, Liu Y, Ma C, Guan C, Ma X, Meng S. Mesenchymal stem cell-originated exosomal lncRNA HAND2-AS1 impairs rheumatoid arthritis fibroblast-like synoviocyte activation through miR-143-3p/TNFAIP3/NF-κB pathway. J Orthop Surg Res. (2021) 16:116. doi: 10.1186/s13018-021-02248-1
156. Yan Q, Liu H, Sun S, Yang Y, Fan D, Yang Y, et al. Adipose-derived stem cell exosomes loaded with icariin alleviates rheumatoid arthritis by modulating macrophage polarization in rats. J Nanobiotechnology. (2024) 22:423. doi: 10.1186/s12951-024-02711-1
157. You DG. Metabolically engineered stem cell-derived exosomes to regulate macrophage heterogeneity in rheumatoid arthritis. Int J Mol Sci. (2021) 7. doi: 10.1126/sciadv.abe0083
158. Tang J, Wang X, Lin X, Wu C. Mesenchymal stem cell-derived extracellular vesicles: a regulator and carrier for targeting bone-related diseases. Cell Death Discov. (2024) 10(1):212. doi: 10.1038/s41420-024-01973-w
159. Chen Z, Wang H, Xia Y. Therapeutic potential of mesenchymal cell-derived miRNA-150-5p-expressing exosomes in rheumatoid arthritis mediated by the modulation of MMP14 and VEGF. J Immunol. (2018) 201(8):2472–82. doi: 10.4049/jimmunol.1800304
160. Li GQ, Fang YX, Liu Y, Meng FR, Wu X, Zhang CW, et al. MicroRNA-21 from bone marrow mesenchymal stem cell-derived extracellular vesicles targets TET1 to suppress KLF4 and alleviate rheumatoid arthritis. Ther Adv Chronic Dis. (2021) 12:20406223211007369. doi: 10.1177/20406223211007369
161. Chang L, Kan L. Mesenchymal Stem Cell-Originated Exosomal Circular RNA circFBXW7 Attenuates Cell Proliferation, Migration and Inflammation of Fibroblast-Like Synoviocytes by Targeting miR-216a-3p/HDAC4 in Rheumatoid Arthritis. J Inflammation Res. (2021) 14:6157–71. doi: 10.2147/JIR.S336099
162. Ma W, Tang F, Xiao L, Han S, Yao X, Zhang Q, et al. miR-205-5p in exosomes divided from chondrogenic mesenchymal stem cells alleviated rheumatoid arthritis via regulating MDM2 in fibroblast-like synoviocytes. J Musculoskelet Neuronal Interact. (2022) 22:132–41.
163. Meng Q, Qiu B. Exosomal microRNA-320a derived from mesenchymal stem cells regulates rheumatoid arthritis fibroblast-like synoviocyte activation by suppressing CXCL9 expression. Front Physiol. (2020) 11:441. doi: 10.3389/fphys.2020.00441
164. Zhang J, Zhang Y, Ma Y, Luo L, Chu M, Zhang Z. Therapeutic Potential of Exosomal circRNA Derived from Synovial Mesenchymal Cells via Targeting circEDIL3/miR-485-3p/PIAS3/STAT3/VEGF Functional Module in Rheumatoid Arthritis. Int J Nanomedicine. (2021) 16:7977–94. doi: 10.2147/IJN.S333465
165. Liu D, Fang Y, Rao Y, Tan W, Zhou W, Wu X, et al. Synovial fibroblast-derived exosomal microRNA-106b suppresses chondrocyte proliferation and migration in rheumatoid arthritis via down-regulation of PDK4. J Mol Med (Berl). (2020) 98(3):409–23. doi: 10.1007/s00109-020-01882-2
166. Lin Z, Li W, Wang Y, Lang X, Sun W, Zhu X, et al. SMSCs-derived sEV overexpressing miR-433-3p inhibits angiogenesis induced by sEV released from synoviocytes under triggering of ferroptosis. Int Immunopharmacol. (2023) 116:109875. doi: 10.1016/j.intimp.2023.109875
167. Ren J, Zhang F, Zhu S, Zhang W, Hou J, He R, et al. Exosomal long non-coding RNA TRAFD1-4:1 derived from fibroblast-like synoviocytes suppresses chondrocyte proliferation and migration by degrading cartilage extracellular matrix in rheumatoid arthritis. Exp Cell Res. (2023) 422:113441. doi: 10.1016/j.yexcr.2022.113441
168. Xu W, Liu X, Qu W, Wang X, Su H, Li W, et al. Exosomes derived from fibrinogen-like protein 1-overexpressing bone marrow-derived mesenchymal stem cells ameliorates rheumatoid arthritis. Bioengineered. (2022) 13(6):14545–61. doi: 10.1080/21655979.2022.2090379
169. Chen J, Liu M, Luo X, Peng L, Zhao Z, He C, et al. Exosomal miRNA-486-5p derived from rheumatoid arthritis fibroblast-like synoviocytes induces osteoblast differentiation through the Tob1/BMP/Smad pathway. Biomater Sci. (2020) 8:3430–42. doi: 10.1039/C9BM01761E
170. Huang Y, Chen L, Chen D, Fan P, Yu H. Exosomal microRNA-140-3p from human umbilical cord mesenchymal stem cells attenuates joint injury of rats with rheumatoid arthritis by silencing SGK1. Mol Med. (2022) 28(1):36. doi: 10.1186/s10020-022-00451-2
Keywords: extracellular vesicles, immunomodulation, rheumatoid arthritis, therapeutic roles, diagnostic markers
Citation: Abebaw D, Akelew Y, Adugna A, Teffera ZH, Tegegne BA, Fenta A, Selabat B, Amare GA, Getinet M, Jemal M, Baylie T and Atnaf A (2024) Extracellular vesicles: immunomodulation, diagnosis, and promising therapeutic roles for rheumatoid arthritis. Front. Immunol. 15:1499929. doi: 10.3389/fimmu.2024.1499929
Received: 22 September 2024; Accepted: 30 October 2024;
Published: 18 November 2024.
Edited by:
Giacomo Cafaro, University of Perugia, ItalyReviewed by:
Gloria Riitano, Sapienza University of Rome, ItalyOnno Arntz, Radboud University Medical Centre, Netherlands
Copyright © 2024 Abebaw, Akelew, Adugna, Teffera, Tegegne, Fenta, Selabat, Amare, Getinet, Jemal, Baylie and Atnaf. This is an open-access article distributed under the terms of the Creative Commons Attribution License (CC BY). The use, distribution or reproduction in other forums is permitted, provided the original author(s) and the copyright owner(s) are credited and that the original publication in this journal is cited, in accordance with accepted academic practice. No use, distribution or reproduction is permitted which does not comply with these terms.
*Correspondence: Desalegn Abebaw, RGVzYWxlZ25hYmViYXcyMEBnbWFpbC5jb20=