- 1Spanish Pneumococcal Reference Laboratory, National Center for Microbiology, Instituto de Salud Carlos III, Madrid, Spain
- 2CIBER de Enfermedades Respiratorias (CIBERES), Instituto de Salud Carlos III, Madrid, Spain
- 3Department of Genetics, Physiology and Microbiology, Faculty of Biology, Complutense University of Madrid, Madrid, Spain
In respiratory pathogens such as Streptococcus pneumoniae, biofilm formation is associated with the colonization of the nasopharynx and chronic respiratory infection. Previous data have shown that pneumococcal conjugate vaccines (PCVs) had an impact on S. pneumoniae colonization and a potential replacement by other respiratory pathogens such as Staphylococcus aureus. The objective of this work was to evaluate the evasion of the immune system by monospecific biofilms and by S. aureus-S. pneumoniae mixed biofilms. We performed opsonophagocytosis assays (OPA) using human HL-60 against previously disaggregated monospecific biofilms of MSSA, MRSA and S. aureus-S. pneumoniae mixed biofilms. We used pre-immune and post-immune serum from immunocompetent adult patients vaccinated with PCV13. Immune sera had a clear effect in reducing pneumococcal biofilms of serotypes 3, 14, 18C, 19F and 19A, whereas had no effect in non-PCV13 serotypes such as 8, 11A and 24F. Our study confirmed that serum from vaccinated patients with PCV13 did not have any effect in reducing S. aureus population in monospecific biofilms, regardless the methicillin resistance phenotype. Moreover, immunized sera from vaccinated patients with PCV13 did not have any effect in S. aureus population in the mixed biofilm, whereas significantly reduced the population of pneumococcal serotype 19A strain in the mixed biofilm which is of great interest because this serotype is included in PCV13, and it is associated with vaccine failures.
1 Introduction
Chronic obstructive pulmonary disease (COPD) and cystic fibrosis (CF) are progressive inflammatory pathologies affecting the lungs characterized by impaired mucociliary clearance, mucus hypersecretion, and altered mucosal immunity. These factors contribute to the colonization of the lower respiratory tract by different bacterial species. The presence of bacterial biofilms is very common in these two chronic pulmonary diseases as well as in acute otitis media or even during the carriage state (1, 2). Streptococcus pneumoniae, or pneumococcus, is a common pathogen causing these pathologies, being responsible for many of the acute exacerbations and chronic bronchiectasis in these patients (2). Polymicrobial biofilm development is a usual occurrence in persistent respiratory infections, allowing bacterial communities to evade host immune responses and enhance antimicrobial resistance. The pneumococcal biofilm demonstrates an enhanced ability to evade the host immune response, specifically by reducing complement system activation and avoiding phagocytosis (3). Compared to planktonic bacteria, biofilm formation is associated with a decreased deposition of complement components such as C3 and C1q, and increased recruitment of the regulatory protein factor H on the bacterial surface. Confocal microscopy analysis revealed that C3 molecules could permeate all layers of the intact biofilm and exposure to serum components do not affect its architecture. The observed differences in complement deposition are attributed to phenotypic alterations in pneumococcal cells within the biofilm, persisting even after biofilm disaggregation (3).
The use of pneumococcal conjugate vaccines (PCVs) has shown to be effective in reducing the burden of disease and the carrier state by vaccine serotypes, but long-term use may lead to serotype replacement by non-vaccine serotypes (4, 5). Additionally, some PCV13 vaccine serotypes have still a high burden of disease despite the introduction of the vaccine, being serotype 3 the most frequent followed by serotypes 19A, 14 and 19F (5–9). This is attributed to a moderate impact of PCVs against pediatric colonization (10). Since the introduction of PCV7, epidemiological studies have shown contradictory reports on the interaction between conjugate vaccines, pneumococcus, and Staphylococcus aureus. Some reports show a detrimental effect describing increase colonization and disease rates by S. aureus in vaccinated population (11–14) whereas other reports show no effect (15, 16) or even a beneficial contribution indicating that vaccination with PCV13 in children diminished S. aureus colonization in both the nasopharyngeal tract and the middle ear. (17, 18) The purpose of this report was to demonstrate for the first time that vaccination with PCV13 has effect against pneumococcal serotypes forming biofilms and the potential impact on polymicrobial biofilms including methicillin resistant S. aureus and S. pneumoniae.
2 Materials and methods
2.1 Strains and human sera
Pneumococcal strains used belonged to PCV13 serotypes (S3, S14, S18C, S19A, S19F) and non-PCV13 serotypes (S8, S11A, S24F) (Table 1). For S. aureus monospecific biofilms, we used the Methicillin-Sensitive S. aureus (MSSA) and Methicillin-Resistance S. aureus (MRSA) strains whereas for mixed biofilms we used the MRSA strain and a pneumococcal serotype 19A strain (Table 1). Human sera (pre-immune or post-PCV13 1 month after vaccination) were obtained from seven healthy immunocompetent adults with an age range of 25-50 years old and a gender distribution of 57% females and 43% males. All participants provided written informed consent (authorization approval of Ethics Committee: HULP: PI-1832). The project was approved by ISCIII Ethics Committee (Ref: CEI PI 45_2021-v2).
2.2 Biofilm formation
Pneumococcal monospecific biofilms of S. pneumoniae, S. aureus (MSSA and MRSA) or mixed biofilms of both bacterial species were developed following recent protocols described in our laboratory (19). Briefly, cells were grown in a C+Y medium to an A550 of ≈ 0.5-0.6 and diluted 100-fold. S. pneumoniae and S. aureus suspensions were used individually or 1:11 inoculum proportion mixed biofilms (MRSA: Sp, 1:11, to obtain 1:1 viability in the mature mixed biofilm), and aliquots of 200 µL were added into 96-well polystyrene treated plates. The biofilms were incubated from 5 h at 34°C. After incubation, the planktonic culture was separated, and biofilms were gently disaggregated using a pipette to serve as inoculum of opsonophagocytosis assays (OPA).
Dilutions were culture on blood agar plates and CFU/ml were determined the next day after incubation. In mixed infections, we used blood agar plates with 5 µg/ml of gentamicin and plates containing Salt Mannitol Agar to select S. pneumoniae and S. aureus respectively.
2.3 Opsonophagocytosis assays
OPA were performed using human HL-60 cells (CCL-240;ATCC) differentiated to granulocytes as previously described (20, 21). In brief, we used 105 HL-60 cells differentiated to granulocytes and 2.5 × 102 CFU of S. pneumoniae, S. aureus or both (mixed biofilms) [multiplicity of infection (MOI) 400:1] that were previously opsonized with HBSS (control) or 1/8 of heat-inactivated pre-immune or immune sera. If there were no differences between the OPA result from pre-immune sera compared to control, we consider that there are not natural anti-Streptococcus or anti-Staphylococcus functional antibodies in the immunocompetent subjects tested. The limit of ≥50% killing was considered as a positive response eliciting functional antibodies (20, 21). In addition, pre-immune or immune sera were tested against disaggregated biofilms and against planktonic cultures of the different strains to confirm the functional activity of immune sera.
2.4 Confocal laser scanning microscopy of biofilms
We used CLSM to visualize the cells as previously described (19). Briefly, biofilms were grown as explained above on glass-bottomed dishes (WillCo-dish; WillCo Wells B.V., The Netherlands) for 5 h at 34°C (19). Non-adherent bacteria were removed from biofilms by washing with sterile water, and bacterial viability within the biofilm was assessed using the LIVE/DEAD BacLight kit (Invitrogen). CLSM observations were made with a Leica spectral SP5 confocal microscope and analyzed with the LAS AF software. Images represent the x–y from XYZ-stacks at 0.5-µm intervals.
2.5 Statistical analysis
Data were obtained from different independent experiments, containing at least three replicates in each experiment. To obtain OPA (%), viability data (CFU/ml) was normalized to control (OPA with HBSS and the source of complement). A two-tailed Student’s t test was used for two groups’ comparison. Statistical analysis were performed using GraphPad 8.0 software. Each serotype was tested against at least three different patient’s sera.
3 Results
We first evaluated the morphology of S. pneumoniae when forming biofilms. Bacteria in a planktonic state and in a disaggregated biofilm state prior to serum addition exhibited a similar distribution or aggregation (Figure 1). Phenotypic biofilm resistance to the immune system was previously demonstrated to be equal in intact and disaggregated biofilms (3). Exposure of pneumococcal monospecific biofilms to human pre-immune sera did not show any killing effect compared to control (Figures 2A, B). However, using sera from PCV13 vaccinated individuals (immune sera), we observed a significant biofilm reduction for all the vaccine serotypes analyzed. These results confirm that PCV13 vaccination elicits functional antibodies that induce opsonophagocytosis of pneumococcal biofilms by vaccine serotypes (Figure 2B). In the case of biofilms by non-vaccine serotypes, immune sera did not reduce the pneumococcal biofilm demonstrating that the vaccine is effective only against the biofilms of vaccine-covered serotypes as expected (Figure 2C).
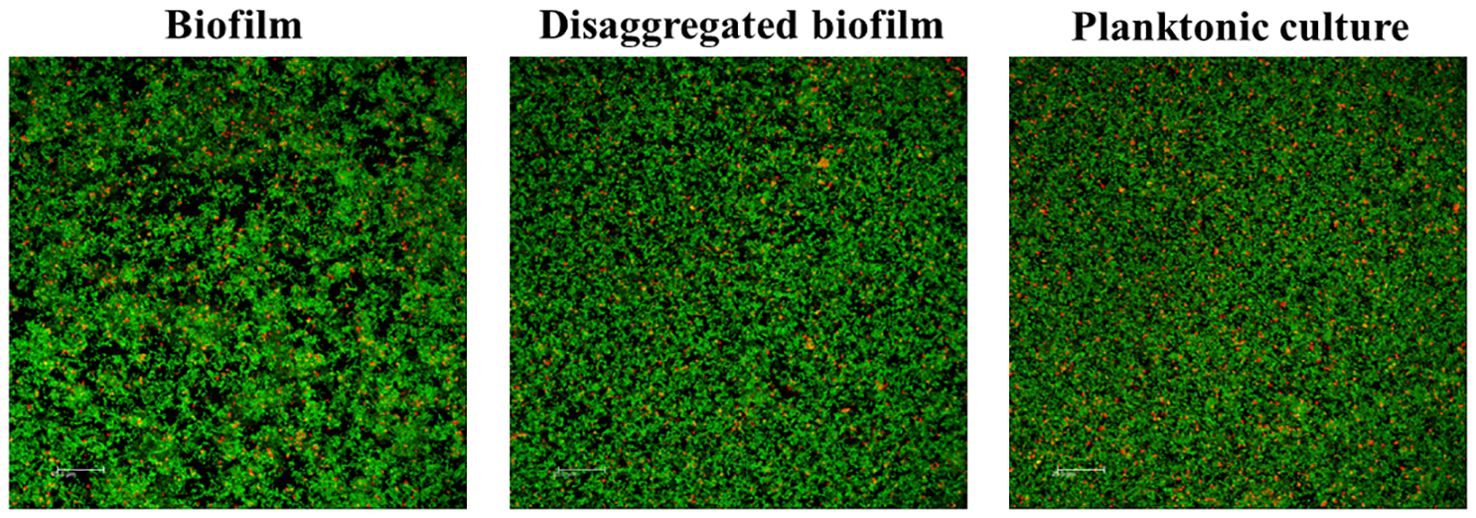
Figure 1. Architecture of a biofilm, disaggregated biofilm and planktonic culture of S. pneumoniae before adding vaccine serum. Biofilms and planktonic culture were stained with the BacLight kit (Invitrogen) to reveal viable (green fluorescence) and non-viable (red fluorescence) bacteria. Scale bars, 25 μm.
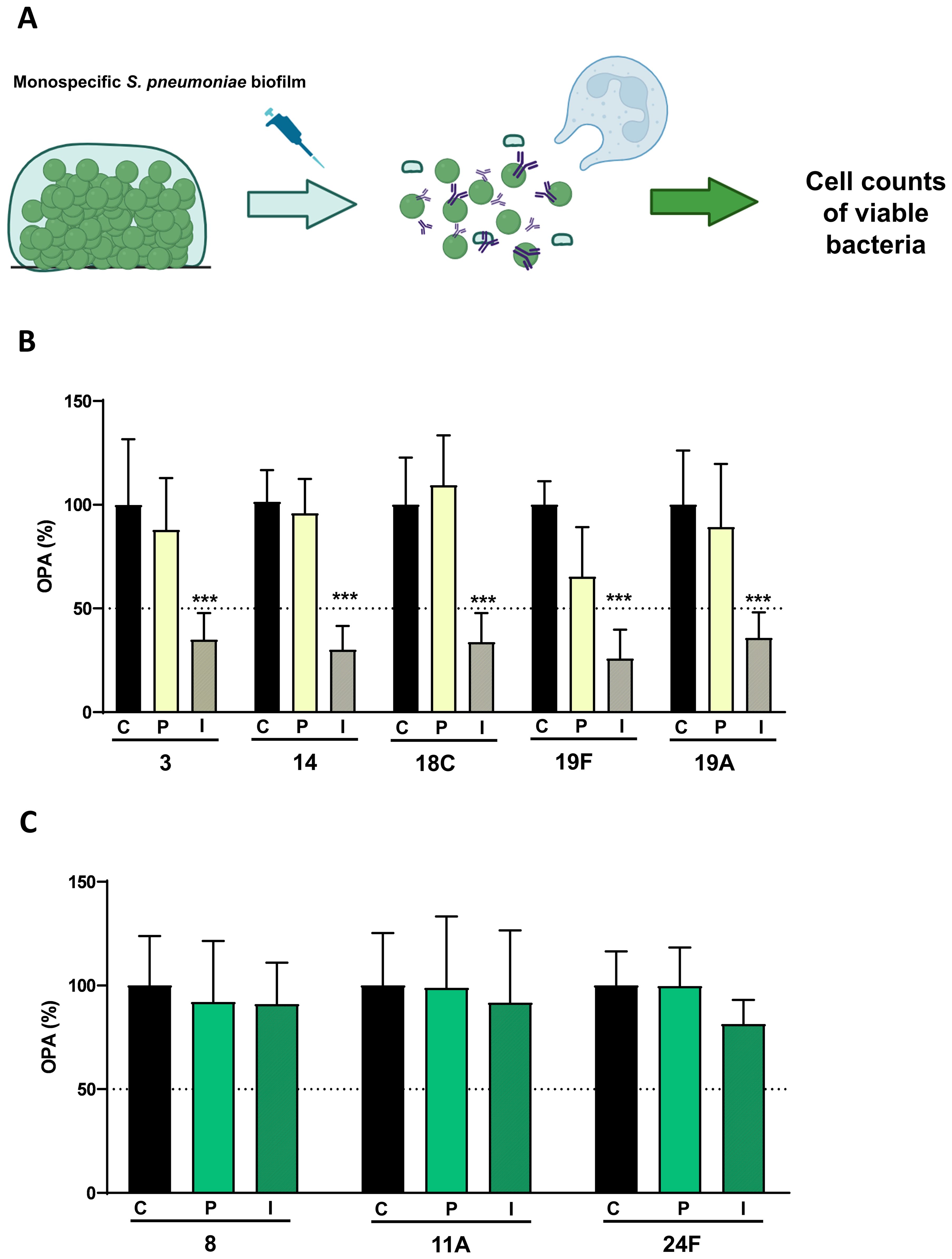
Figure 2. Opsonophagocytosis killing assays (OPA) of pneumococcal biofilms of different serotypes mediated by PCV13. (A) Scheme for the OPA assay of pneumococcal cells forming the biofilm. Briefly, after incubation, the biofilm was gently disaggregated with a pipette. Later, disaggregated cells were exposed to complement and HBSS (control), pre-vaccine (pre-immune) or immune serum (1/8 dilution), and to HL-60 neutrophils in a 400:1 MOI (HL-60: bacteria). (B) OPA (%) of pneumococcal serotypes included in PCV13 vaccine. (C) OPA (%) of pneumococcal serotypes not included in PCV13 vaccine. Black bars show biofilm control of different serotypes in the absence of human serum, with HBSS and the source of complement (C: Control). To obtain OPA (%), viability data (CFU/ml) were normalized to control (OPA with HBSS and the source of complement).Open bars show biofilms of different serotypes incubated with human non-vaccine serum (P: pre-vaccine serum). Hatched bars show biofilms of different serotypes incubated with serum from vaccinated individuals (I: Immune vaccine serum). Diagrams were made with BioRender. Columns represent means and standard deviation bars are shown, and asterisks mark statistically significant results (two-tailed Student’s t test: ***P < 0.001) when comparing the biofilm exposed to immune sera versus the control.
To evaluate the possible heterologous effect of PCV13 against other respiratory pathogens that colonize the upper respiratory tract such as S. aureus, we tested pre-immune sera or immune sera from patients vaccinated with PCV13 against the monospecific biofilm of MSSA and MRSA strains (Figures 3A–C). The level of the biofilm remained unchanged regardless of the sera used indicating that antibodies elicited against PCV13 did not have any impact on the S. aureus biofilm (Figure 3B, C).
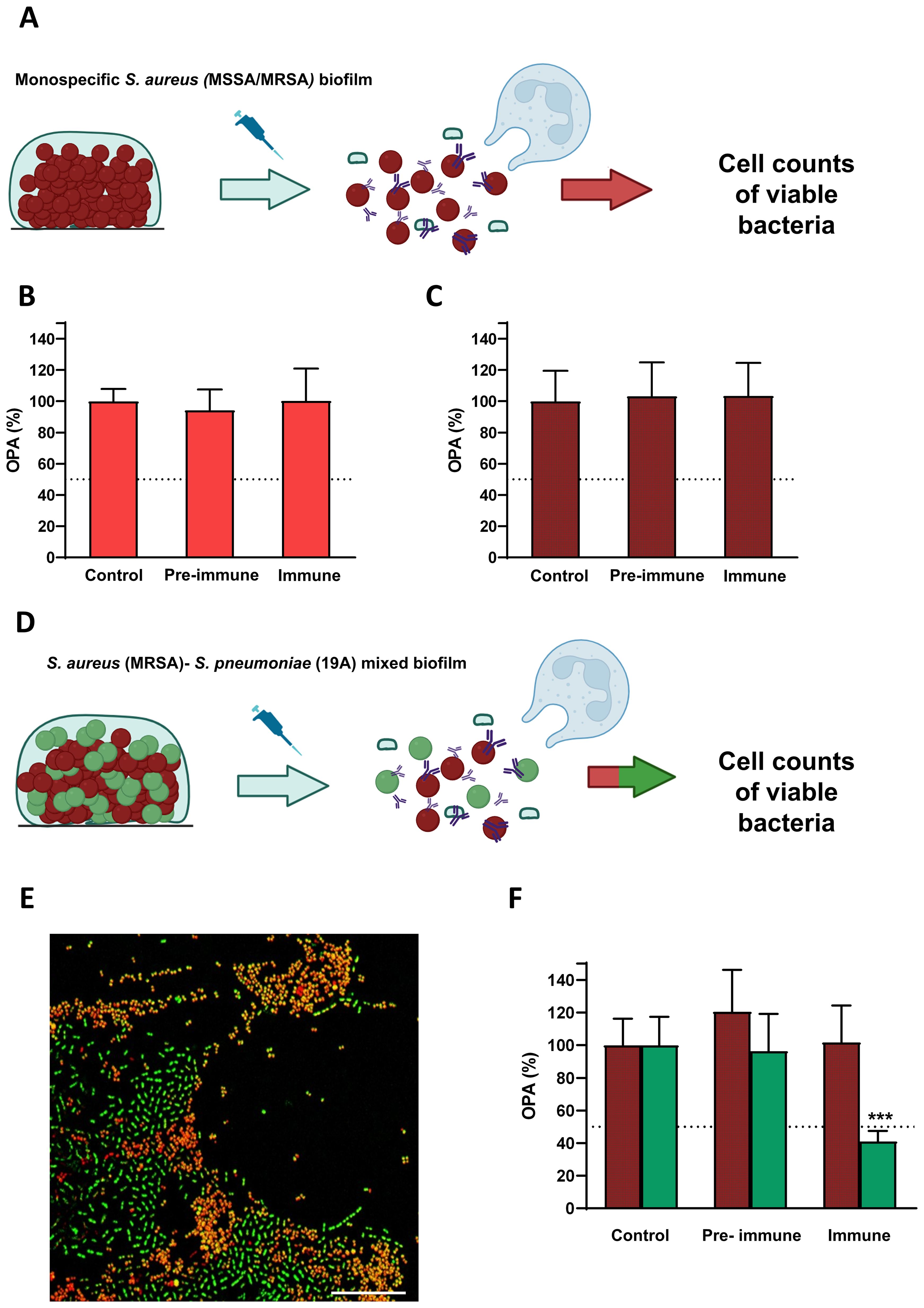
Figure 3. Opsonophagocytosis killing assays (OPA) of S. aureus monospecific biofilms or mixed biofilms containing S. pneumoniae mediated by PCV13. (A) Scheme for the OPA assay of S. aureus cells forming the monospecific biofilm. Briefly, after incubation, the biofilm was gently disaggregated with a pipette. Later, disaggregated cells were exposed to complement and HBSS (control), pre-vaccine (pre-immune) or immune serum (1/8 dilution), and to HL-60 neutrophils in a 400:1 MOI (HL-60: bacteria). (B) OPA of MSSA monospecific biofilms. (C) OPA of MRSA monospecific biofilms. (D) Scheme for the OPA assay of the mixed biofilm containing MRSA and S. pneumoniae of serotype 19A. Briefly, the mixed biofilm of MRSA-S. pneumoniae was incubated following previous methodology (19). Then, biofilm was gently disaggregated with a pipette. Later, disaggregated cells were exposed to complement and PBS (control), pre-vaccine (pre-immune) or immune serum (1/8 dilution), and to HL-60 neutrophils in a 400:1 MOI (HL-60: bacteria). (E) Confocal microscopy image showing S. aureus in red and S. pneumoniae in green using the BacLight kit (Invitrogen) component B Scale bars, 25 μm. (F) OPA assay of the mixed biofilm containing MRSA in red hatched bars and S. pneumoniae of serotype 19A in green bars. To obtain OPA (%), viability data (CFU/ml) were normalized to control (OPA with HBSS and the source of complement). Diagrams were made with BioRender. Columns represent means and standard deviation are shown, and asterisks mark statistically significant results (two-tailed Student’s t test: ***P < 0.001) when comparing the biofilm exposed to immune sera versus the control.
In addition, we also evaluated the impact of PCV13 against a mixed biofilm formed by a MRSA strain and a multidrug-resistant strain of serotype 19A of pneumococcus (PCV13 serotype) (Figures 3D, E). Hence, we confirmed that vaccination with PCV13 did not have any effect against S. aureus in the polymicrobial biofilm whereas significantly reduced the pneumococcal population within the mixed biofilm (Figure 3F). Moreover, S. aureus population cells within the mixed biofilm did not increase when S. pneumoniae was killed in the presence of immune sera suggesting that PCV13 vaccination only targets pneumococcal cells of vaccine-covered serotypes without inducing replacement by MRSA of the niche left by pneumococcus (Figure 3F).
4 Discussion
Colonization of the upper respiratory tract is essential for the pneumococcal pathogenesis process as in many cases the bacterium can disseminate producing non-invasive diseases (acute otitis media, sinusitis, non-bacteraemic pneumonia) or even invasive pneumococcal disease (IPD) (22). The use of PCVs is the best prophylactic strategy to prevent the development of these diseases reducing the morbidity and mortality rates (4). Immunogenicity studies evaluating PCVs in children and adults are always based on their ability to induce functional antibodies that trigger the opsonophagocytosis process of the bacterium. The contribution of PCVs in the reduction of AOM, bronchiectasis, hospitalizations of COPD and even the carriage state is well known and in all these conditions and pathologies, the presence of biofilms is a generic event (1, 2). However, there are no studies demonstrating that antibodies elicited after vaccination with PCVs have the potential to recognize and induce the clearance of bacteria forming the biofilm. In pneumococcal pathogenesis, pediatric isolates are better biofilm formers than adult isolates and emerging serotypes causing IPD in adults can originally be from strains colonizing the pediatric nasopharyngeal tract and therefore, prevention of the carrier state may be a very useful strategy to induce herd protection in adults (23).
The in vitro biofilm model described in this study may be a good representation of an airways biofilm. In this sense, biofilms covering multiwell plates have proven to be a good platform for testing antimicrobial or antibiofilm compounds, both for dispersing and preventing biofilm formation (24). Similar results have been obtained when comparing antimicrobial treatments using in vitro biofilms and murine models of pneumonia (25). In vitro biofilms are also a valuable tool for predicting emerging serotypes or genotypes associated with colonization (26, 27). Finally, this in vitro biofilm system is a good platform for studying interactions between microorganisms that constitute the same biofilm (19).
Our results using an in vitro biofilm platform, human sera from vaccinated individuals and neutrophils derived from HL-60 cells, confirm for the first time that PCV13 is immunogenic against pneumococcal biofilms formed by vaccine-preventable serotypes including serotype 3. This is in agreement with epidemiological studies demonstrating that the introduction of PCV13 in pediatric immunization programs has reduced up to 38% of all IPD cases in children (4). Moreover, the introduction of PCV13 diminished the IPD cases associated with antimicrobial resistance such as those caused by serotypes 19F and 19A (9), that are also associated with the biofilm state (28). Our results are of great interest for public health because we demonstrate for the first time a beneficial preventive effect of PCV13 elicited antibodies against the biofilm state of both serotypes. Despite observing that immunized sera from adult patients provided protection against biofilms formed by the vaccine serotypes tested, the high prevalence of some of them such as serotype 3 in the adult population (5) can largely be attributed to insufficient vaccine coverage in adults (4, 5, 29).
The impact of PCVs on the nasopharyngeal niche is concerning, as the reduction in pneumococcal colonization could create an opportunity for other invasive pathogens to occupy the space left by the pneumococcus. Additionally, non-sterilizing immunization may be beneficial in preventing colonization by new pathogens that could cause similar or more severe diseases (30, 31). In terms of heterologous protection, there was no effect against S. aureus but antibodies to PCV13 reduced significantly the pneumococcal population within the polymicrobial biofilm. These results could shed light on the discrepancies found in literature, as different studies, mainly focus on carriage in children vaccinated or not with PCVs, have obtained different outcomes when evaluating the changes in S. aureus carriage due to PCVs (15–17). Our results align with previous studies demonstrating that the use of PCVs is effective in pneumococcal nasopharyngeal carriage in children suffering AOM without any change in S. aureus carriage (15–17).
Data availability statement
The raw data supporting the conclusions of this article will be made available by the authors, without undue reservation.
Ethics statement
This study involves sera from human participants, and all provided written informed consent (authorization approval of Ethics Committee: HULP: PI-1832). Participants gave informed consent to participate in the study before taking part. The project was approved by ISCIII Ethics Committee (Ref: CEI PI 45_2021-v2). The studies were conducted in accordance with the local legislation and institutional requirements. The participants provided their written informed consent to participate in this study.
Author contributions
JS: Writing – review & editing, Writing – original draft, Visualization, Validation, Methodology, Investigation, Formal analysis, Data curation, Conceptualization. JY: Writing – review & editing, Writing – original draft, Visualization, Validation, Supervision, Resources, Methodology, Funding acquisition, Formal analysis, Conceptualization. MD: Supervision, Writing – review & editing, Writing – original draft, Visualization, Validation, Methodology, Investigation, Formal analysis, Data curation, Conceptualization.
Funding
The author(s) declare that financial support was received for the research, authorship, and/or publication of this article. This work was supported by Ministerio de Ciencia e Innovación (MICINN) (grant PID2020-119298RB-100 and grant CPP2022-009574).
Acknowledgments
We thank Dr. Patricia Richi for coordinating the clinical trial.
Conflict of interest
JS and MD have participated in advisory boards from MSD. JY has participated in advisory boards from GSK, MSD, and Pfizer and has received funding from MSD and Pfizer that are not related to the current study.
Publisher’s note
All claims expressed in this article are solely those of the authors and do not necessarily represent those of their affiliated organizations, or those of the publisher, the editors and the reviewers. Any product that may be evaluated in this article, or claim that may be made by its manufacturer, is not guaranteed or endorsed by the publisher.
References
1. Huang YJ, Sethi S, Murphy T, Nariya S, Boushey HA, Lynch SV. Airway microbiome dynamics in exacerbations of chronic obstructive pulmonary disease. J Clin Microbiol. (2014) 52:2813–23. doi: 10.1128/JCM.00035-14
2. Martin C, Burgel PR, Lepage P, Andréjak C, De Blic J, Bourdin A, et al. Host-microbe interactions in distal airways: relevance to chronic airway diseases. Eur Respir Rev. (2015) 24:78–91. doi: 10.1183/09059180.00011614
3. Domenech M, Ramos-Sevillano E, Garcia E, Moscoso M, Yuste J. Biofilm formation avoids complement immunity and phagocytosis of Streptococcus pneumoniae. Infect Immun. (2013) 81:2606–15. doi: 10.1128/IAI.00491-13
4. De Miguel S, Domenech M, González-Camacho F, Sempere J, Vicioso D, Sanz JC, et al. Nationwide trends of invasive pneumococcal disease in Spain from 2009 through 2019 in children and adults during the pneumococcal conjugate vaccine era. Clin Infect Dis. (2021) 73:e3778–87. doi: 10.1093/cid/ciaa1483
5. Pérez-García C, Sempere J, De Miguel S, Hita S, Úbeda A, Vidal EJ, et al. Surveillance of invasive pneumococcal disease in Spain exploring the impact of the COVID-19 pandemic, (2019-2023). J Infect. (2024) 89:106204. doi: 10.1016/j.jinf.2024.106204
6. Moore MR, Link-Gelles R, Schaffner W, Lynfield R, Lexau C, Bennett NM, et al. Effect of use of 13-valent pneumococcal conjugate vaccine in children on invasive pneumococcal disease in children and adults in the USA: analysis of multisite, population-based surveillance. Lancet Infect Dis. (2015) 15:301–9. doi: 10.1016/S1473-3099(14)71081-3
7. Glikman D, Dagan R, Barkai G, Averbuch D, Guri A, Givon-Lavi N, et al. Dynamics of severe and non-severe invasive pneumococcal disease in young children in Israel following PCV7/PCV13 introduction. Pediatr Infect Dis J. (2018) 37:1048–53. doi: 10.1097/INF.0000000000002100
8. Ladhani SN, Collins S, Djennad A, Sheppard CL, Borrow R, Fry NK, et al. Rapid increase in non-vaccine serotypes causing invasive pneumococcal disease in England and Wales 2000-17: a prospective national observational cohort study. Lancet Infect Dis. (2018) 18:441–51. doi: 10.1016/S1473-3099(18)30052-5
9. Sempere J, Llamosí M, López Ruiz B, Del Río I, Pérez-García C, Lago D, et al. Effect of pneumococcal conjugate vaccines and SARS-CoV-2 on antimicrobial resistance and the emergence of Streptococcus pneumoniae serotypes with reduced susceptibility in Spain 2004-20: a national surveillance study. Lancet Microbe. (2022) 3:e744–52. doi: 10.1016/S2666-5247(22)00127-6
10. Nicholls TR, Leach AJ, Morris PS. The short-term impact of each primary dose of pneumococcal conjugate vaccine on nasopharyngeal carriage: Systematic review and meta-analyses of randomised controlled trials. Vaccine. (2016) 34:703–13. doi: 10.1016/j.vaccine.2015.12.048
11. Regev-Yochay G, Dagan R, Raz M, Carmeli Y, Shainberg B, Derazne E, et al. Association between carriage of Streptococcus pneumoniae and Staphylococcus aureus in Children. Jama. (2004) 292:716–20. doi: 10.1001/jama.292.6.716
12. Van Gils EJ, Hak E, Veenhoven RH, Rodenburg GD, Bogaert D, Bruin JP, et al. Effect of seven-valent pneumococcal conjugate vaccine on Staphylococcus aureus colonisation in a randomised controlled trial. PloS One. (2011) 6:e20229. doi: 10.1371/journal.pone.0020229
13. Spijkerman J, Prevaes SM, Van Gils EJ, Veenhoven RH, Bruin JP, Bogaert D, et al. Long-term effects of pneumococcal conjugate vaccine on nasopharyngeal carriage of S. pneumoniae, S. aureus, H. influenzae and M. catarrhalis. PloS One. (2012) 7:e39730. doi: 10.1371/journal.pone.0039730
14. Chien YW, Vidal JE, Grijalva CG, Bozio C, Edwards KM, Williams JV, et al. Density interactions among Streptococcus pneumoniae, Haemophilus influenzae and Staphylococcus aureus in the nasopharynx of young Peruvian children. Pediatr Infect Dis J. (2013) 32:72–7. doi: 10.1097/INF.0b013e318270d850
15. Cohen R, Levy C, Thollot F, de la Rocque F, Koskas M, Bonnet E, et al. Pneumococcal conjugate vaccine does not influence Staphylococcus aureus carriage in young children with acute otitis media. Clin Infect Dis. (2007) 45:1583–7. doi: 10.1086/523734
16. Lee GM, Huang SS, Rifas-Shiman SL, Hinrichsen VL, Pelton SI, Kleinman K, et al. Epidemiology and risk factors for Staphylococcus aureus colonization in children in the post-PCV7 era. BMC Infect Dis. (2009) 9:110. doi: 10.1186/1471-2334-9-110
17. Dunne EM, Smith-Vaughan HC, Robins-Browne RM, Mulholland EK, Satzke C. Nasopharyngeal microbial interactions in the era of pneumococcal conjugate vaccination. Vaccine. (2013) 31:2333–42. doi: 10.1016/j.vaccine.2013.03.024
18. Leach AJ, Wigger C, Beissbarth J, Woltring D, Andrews R, Chatfield MD, et al. General health, otitis media, nasopharyngeal carriage and middle ear microbiology in Northern Territory Aboriginal children vaccinated during consecutive periods of 10-valent or 13-valent pneumococcal conjugate vaccines. Int J Pediatr Otorhinolaryngol. (2016) 86:224–32. doi: 10.1016/j.ijporl.2016.05.011
19. Sempere J, Llamosí M, Román F, Lago D, González-Camacho F, Pérez-García C, et al. Clearance of mixed biofilms of Streptococcus pneumoniae and methicillin-susceptible/resistant Staphylococcus aureus by antioxidants N-acetyl-L-cysteine and cysteamine. Sci Rep. (2022) 12:6668. doi: 10.1038/s41598-022-10609-x
20. Burton RL, Nahm MH. Development and validation of a fourfold multiplexed opsonization assay (MOPA4) for pneumococcal antibodies. Clin Vaccine Immunol. (2006) 13:1004–9. doi: 10.1128/CVI.00112-06
21. Richi P, Yuste J, Navío T, González-Hombrado L, Salido M, Thuissard-Vasallo I, et al. Impact of biological therapies on the immune response after pneumococcal vaccination in patients with autoimmune inflammatory diseases. Vaccines (Basel). (2021) 9. doi: 10.3390/vaccines9030203
22. Weiser JN, Ferreira DM, Paton JC. Streptococcus pneumoniae: transmission, colonization and invasion. Nat Rev Microbiol. (2018) 16:355–67. doi: 10.1038/s41579-018-0001-8
23. Sempere J, De Miguel S, González-Camacho F, Yuste J, Domenech M. Clinical relevance and molecular pathogenesis of the emerging serotypes 22F and 33F of streptococcus pneumoniae in Spain. Front Microbiol. (2020) 11. doi: 10.3389/fmicb.2020.00309
24. Domenech M, Garcia E, Moscoso M. In vitro destruction of Streptococcus pneumoniae biofilms with bacterial and phage peptidoglycan hydrolases. Antimicrob Agents Chemother. (2011) 55:4144–8. doi: 10.1128/AAC.00492-11
25. Llamosi M, Sempere J, Coronel P, Gimeno M, Yuste J, Domenech M. Combination of Cefditoren and N-acetyl-l-Cysteine Shows a Synergistic Effect against Multidrug-Resistant Streptococcus pneumoniae Biofilms. Microbiol Spectr. (2022) 10:e0341522. doi: 10.1128/spectrum.03415-22
26. Aguinagalde L, Corsini B, Domenech A, Domenech M, Camara J, Ardanuy C, et al. Emergence of amoxicillin-resistant variants of Spain9V-ST156 pneumococci expressing serotype 11A correlates with their ability to evade the host immune response. PloS One. (2015) 10:e0137565. doi: 10.1371/journal.pone.0137565
27. Domenech M, Damian D, Ardanuy C, Linares J, Fenoll A, Garcia E. Emerging, non-PCV13 serotypes 11A and 35B of Streptococcus pneumoniae show high potential for biofilm formation in vitro. PloS One. (2015) 10:e0125636. doi: 10.1371/journal.pone.0125636
28. Domenech M, Araujo-Bazan L, Garcia E, Moscoso M. In vitro biofilm formation by Streptococcus pneumoniae as a predictor of post-vaccination emerging serotypes colonizing the human nasopharynx. Environ Microbiol. (2014) 16:1193–201. doi: 10.1111/emi.2014.16.issue-4
29. Milucky J, Carvalho MG, Rouphael N, Bennett NM, Talbot HK, Harrison LH, et al. Streptococcus pneumoniae colonization after introduction of 13-valent pneumococcal conjugate vaccine for US adults 65 years of age and older 2015-2016. Vaccine. (2019) 37:1094–100. doi: 10.1016/j.vaccine.2018.12.075
30. Mcdaniel LS, Swiatlo E. Should pneumococcal vaccines eliminate nasopharyngeal colonization? mBio. (2016) 7:e00545–16. doi: 10.1128/mBio.00545-16
Keywords: PCV13, biofilms, OPA, S. aureus, MRSA, S. pneumoniae
Citation: Sempere J, Yuste J and Domenech M (2024) PCV13 vaccine prevents pneumococcal biofilms without affecting Staphylococcus aureus population within the polymicrobial biofilm. Front. Immunol. 15:1495932. doi: 10.3389/fimmu.2024.1495932
Received: 13 September 2024; Accepted: 14 October 2024;
Published: 01 November 2024.
Edited by:
René Köffel, Zurich University of Applied Sciences, SwitzerlandReviewed by:
Elisa Ramos-Sevillano, University College London, United KingdomJonathan Lalsiamthara, University of Texas MD Anderson Cancer Center, United States
Copyright © 2024 Sempere, Yuste and Domenech. This is an open-access article distributed under the terms of the Creative Commons Attribution License (CC BY). The use, distribution or reproduction in other forums is permitted, provided the original author(s) and the copyright owner(s) are credited and that the original publication in this journal is cited, in accordance with accepted academic practice. No use, distribution or reproduction is permitted which does not comply with these terms.
*Correspondence: Mirian Domenech, bWlyaWRvbWVAdWNtLmVz; José Yuste, anl1c3RlQGlzY2lpaS5lcw==