- 1Institute of Immunology, Centre for Infectious Diseases, Faculty of Veterinary Medicine, Centre for Biotechnology and Biomedicine, Leipzig University, Leipzig, Germany
- 2Clinic for Ruminants and Swine, Faculty of Veterinary Medicine, Leipzig University, Leipzig, Germany
- 3Institute of Bacteriology and Mycology, Centre for Infectious Diseases, Faculty of Veterinary Medicine, Leipzig University, Leipzig, Germany
Introduction: Streptococcus suis serotype (cps) 2 is an important bacterial pathogen in piglet nurseries and an emerging zoonotic agent without effective vaccines available. Immunoglobulin (Ig)M plays an essential role in host defense against S. suis. In mice, non-conventional B-1 cells are a major source of protective IgM against encapsulated bacterial pathogens, such as S. pneumoniae. Two IgM+CD21− B-1-like cell subpopulations, distinguishable by CD11R1 expression, were described in pigs, but their properties and functions are poorly understood. This study aimed at a first characterization of the porcine early IgM B cell response against S. suis cps 2.
Methods: We analyzed the same healthy pigs, naturally colonized by different S. suis serotypes, including cps 2, at four and eight weeks of age serologically and determined the frequency of different peripheral B cell subpopulations by flow cytometry. Furthermore, we isolated conventional IgM+CD21+ B-2 cells as well as non-conventional B-1-like cell subpopulations from peripheral blood of eight-weeks-old pigs to evaluate their potential of IgM secretion in response to innate and adaptive stimuli in vitro.
Results: Between the fourth and eighth week of life, a characteristic increase of S. suis cps 2-binding serum IgM antibodies, restricting bacterial growth, was observed. Moreover, we show for the first time that the significant increase of anti-S. suis serum IgM is associated with a relative increase of peripheral non-conventional IgM+CD21− B-1-like cells in vivo, particularly of the IgM+CD21− CD11R1− subpopulation. Noteworthy, sorted IgM+CD21− CD11R1− B-1-like cells from eight-weeks-old pigs spontaneously secreted IgM in vitro. In addition, both non-conventional IgM+CD21− B cell subpopulations, in contrast to conventional IgM+CD21+ B-2 cells, produced anti-S. suis IgM upon toll-like receptor (TLR) stimulation underlining their innate-like characteristics. We furthermore observed that both B-1-like subpopulations secrete S. suis cps 2-binding IgM upon stimulation with T cell-associated factors with highest amounts in IgM+CD21−CD11R1− B-1-like cells even exceeding anti-S. suis IgM levels produced by B-2 cells.
Conclusion: Porcine non-conventional B-1-like cells are a potent source of S. suis-binding IgM indicating a role in immunity during a critical phase of piglet rearing.
1 Introduction
Streptococcus suis is an encapsulated, Gram-positive, zoonotic bacterial pathogen (1). As a natural colonizer, S. suis can be ubiquitously found on tonsils and mucosal surfaces of healthy pigs (2). However, invasive S. suis infection causes life-threatening disease, including meningitis and septicemia in pigs and also in humans (3–5). In piglet nurseries, S. suis is one of the most important pathogens and is associated with high economic losses (5, 6). Pigs at the age of five to ten weeks are predominantly affected (7). Due to the lack of effective vaccines, S. suis is one of the main reasons for the extensive use of antimicrobials in pigs (6). Based on differences in capsular polysaccharides (cps), S. suis is classified into 29 serotypes (8, 9). However, this number does not account for several novel capsular types that have recently been recognized using molecular methods (9). While the serotype distribution of S. suis varies locally, S. suis serotype 2 (cps 2) is the most relevant serotype in pigs and humans worldwide (10).
Bacteremia is considered a crucial step in the pathogenesis of invasive S. suis infections and the bacterial polysaccharide capsule serves as a virulence factor providing protection against opsonophagocytosis in the blood (11–14). Previous studies demonstrated a role of IgM in host defense (15, 16) (IgM-complement-oxidative burst-axis), which is particularly important in the absence of or at low levels of S. suis-binding IgG (16). S. suis secretes an immunoglobulin M-degrading enzyme (IdeSsuis), which serves as a complement evasion mechanism (17). Murine monoclonal IgM antibodies directed against S. suis cps 2 were shown to mediate the killing of S. suis serotype 2 in vitro by opsonization triggering phagocytosis and/or agglutination. This correlated with protection after passive immunization in the mouse model (18).
Conventional B cells, also termed B-2 cells, are an essential component of the adaptive immune system providing antibody-mediated protection against infections. Conventional B-2 cells recognize non-self protein antigens and secrete specific IgM for a short time after activation before usually switching to the production of IgG (19, 20). In addition, B-1 cells with innate functions have been described as a distinct non-conventional B cell lineage in mice and humans. B-1 cells are the main producers of natural IgM antibodies with polyspecific reactivity against self and microbial antigens. Antibodies constitutively secreted by B-1 cells assist in the disposition of cellular debris and provide a first line defense against a variety of microbial pathogens (20). In addition to providing a steady-state level of natural IgM, B-1 cells have also been shown to actively respond to infections with induced IgM production (21). Activation of B-1 cells is induced by repetitive sequences, like capsule polysaccharides, as demonstrated in human immunization studies with pneumococcal polysaccharide (22). In mice, non-conventional B-1 cells have been shown to release large amounts of protective capsule-specific IgM following recognition of polysaccharides of bacterial pathogens, such as S. pneumoniae (23). Although B-1-like cells have been described in pigs (24), little is known about their properties and function.
This study aimed at a first characterization of the early porcine IgM B cell response against S. suis cps 2 and included the analysis of non-conventional B-1-like subpopulations in comparison to conventional B-2 cells.
In pigs between four and eight weeks of age we observed a characteristic increase of S. suis cps 2-binding serum IgM, which restricts survival of streptococci in porcine blood in vitro. Moreover, we show that this IgM increase is associated with the relative increase of IgM+CD21− B-1-like cells in peripheral blood in vivo. CD21−CD11R1− B-1-like cells of 8-weeks-old pigs, capable of constitutive IgM production, are the major source of anti-S. suis IgM in vitro in response to toll-like receptor (TLR) stimulation and T cell-associated signals. Our study characterizes the early porcine IgM B cell response and demonstrates its importance in a critical phase of piglet rearing.
2 Material and methods
2.1 S. suis serotype (cps) 2 strain 10 growth conditions
S. suis serotype (cps) 2 strain 10 is a virulent strain of sequence type 1 that has previously been used for pathogenesis studies by different groups (25, 26), kindly provided by H. Smith (DLO-Lelystad, The Netherlands) (27). Bacteria were grown overnight at 37 °C in Bacto™ Todd-Hewitt-Broth (THB) (BD, Heidelberg, Germany) or on Columbia agar plates with 6% sheep blood (Thermo Oxoid, Schwerte, Germany). S. suis glycerol stocks were prepared at the exponential growth phase (optical densitiy (OD)600 nm = 0.5) and stored at −80 °C in 15% glycerol as single-use aliquots.
2.2 Expression and purification of recombinant IdeSsuis_homologue
The recombinant IdeSsuis_homologue (rIdeSsuis_h) is a truncated variant of the enzyme including the conserved IgM protease domain. Functionally it is equivalent to wild-type full-length IdeSsuis (25). Expression was induced in Escherichia coli BL21 (DE3), carrying the pETideSsuis_homologue plasmid, by the addition of 1 mM isopropyl-β-D-thiogalactopyranoside (IPTG) during the exponential growth phase and the protein was subsequently purified using Ni-affinity chromatography as described previously (16, 25).
2.3 Pigs, blood sample collection, and isolation of porcine peripheral blood mononuclear cells
Blood collection from conventional healthy post-weaning pigs (boar: Hypor Libra × sow: PIC408) naturally colonized by different S. suis serotypes, including cps 2, was approved under the permit number 81-02.04.40.2023.VG034 by North Rhine-Westphalia State Agency for Nature, Environment and Consumer Protection (Landesamt für Natur, Umwelt und Verbraucherschutz Nordrhein-Westfalen). Whole blood and serum samples from the same pigs at four and eight weeks were collected via the Vena cava cranialis into heparinized tubes (Monovette®, Sarstedt, Nümbrecht, Germany) or serum tubes (Monovette®, Sarstedt), respectively. Whole blood samples were centrifuged at 400×g for 10 min, and the plasma was removed. For isolation of peripheral blood mononuclear cells (PBMC), blood was diluted with an equal volume of phosphate buffered saline (PBS), layered onto Pancoll® Separating Solution (density 1.077 g/mL, PAN-Biotech, Aidenbach, Germany) and centrifuged (900×g, 35 min at room temperature (RT), minimal acceleration and deceleration). Mononuclear cells from the interphase were collected and washed with medium (IMDM with L-Glutamine, 25 mM HEPES and 3.024 g/L NaHCO3; PAN-Biotech) supplemented with 10% heat-inactivated fetal bovine serum (FBS, PAN-Biotech) (500×g, 12 min, RT), followed by another washing step with PBS (400×g, 10 min, RT). If required, lysis of remaining erythrocytes was performed by incubating cells at RT in 1-2 mL of lysis buffer (150 mM NH4Cl, 8 mM KHCO3, 2 mM EDTA in PBS, pH 7.0) for 2-3 min. Medium containing 10% FBS was added to stop the reaction. Following two washing steps (400×g, 6 min, RT), the cell number was determined using a Neubauer chamber (Laboroptik Lancing, UK), excluding dead cells via trypan blue staining (Merck-Sigma, Darmstadt, Germany). PBMC were used immediately for phenotyping of B cell subpopulations by flow cytometric analysis or cryo-preserved in liquid nitrogen until B cell sorting [freezing medium: 50% FBS, 40% IMDM, 10% dimethyl sulfoxide (DMSO, Merck-Sigma)].
2.4 Enzyme-linked immunosorbent assay (ELISA)
Detection of anti-S. suis IgM and IgG in serum samples was performed as described previously (17). Briefly, Nunc MaxiSorp™ flat bottom plates (ThermoFisher, Darmstadt, Germany) were coated overnight (O/N) at 4°C with 1×107 colony-forming units (CFU) of inactivated (0.2% paraformaldehyde O/N) S. suis cps 2 strain 10 per well in PBS. All incubations described below were followed by washing steps with PBST (PBS, 0.05% v/v Tween20, Carl Roth). After blocking with a combination of 0.5% w/v BSA and 0.1% v/v gelatine (Carl Roth) in PBS for 1 hour at 37°C, four-step serial dilutions of serum samples were added in duplicates and incubated for 1.5 hours at 37°C. Anti-S. suis IgG was detected using peroxidase-conjugated goat anti-swine IgG (50 ng/mL, Bethyl Laboratories, Montgomery, Texas), while IgM was detected using peroxidase-conjugated goat anti-swine IgM (100 ng/mL, Bethyl Laboratories). 3,3’,5,5’-Tetramethylbenzidine substrate (TMB, KPL, medac, Wedel, Germany) was added for colorimetric development. After 15 min (assay-optimized), the reaction was stopped with 1 M phosphoric acid (Carl Roth). The OD at 450 nm was measured using a Spectra-Max 340 ELISA reader with SoftMax Pro software v5.0 (Molecular devices, Munich, Germany). Blank-reduced ODs were converted to ELISA units using a standard serum from a pig prime-booster-vaccinated with S. suis cps 2 strain 10 as internal reference defined to include 100 ELISA units. The standard serum as well as the colostrum-deprived serum serving as negative control have been used previously (28).
Anti-S. suis IgM was determined in supernatants of stimulated B cell subpopulations (see sections 2.7 and 2.8) as described above for serum samples. Supernatants were used undiluted. Blank-reduced ODs were reported and compared.
For determination of total IgM in serum and supernatants of medium-incubated/stimulated B cell subpopulations, ELISA plates were coated with 2.4 µg/mL of monoclonal anti-porcine IgM (clone: K521C3) Biorad, Feldkirchen, Germany) in PBS. Supernatants of cells incubated in medium without stimuli were diluted 1:2, while serial dilutions of sera (starting from 1:10,000) as well as of supernatants from stimulated cells (CD40L plus IL-21 starting from 1:100; Pam3Cys-SK4 starting from 1:10) were used. Detection was performed as described above. Swine IgM whole molecule serum (Rockland Immunochemicals, Limerick, Pottstown) was used as a standard and total IgM concentrations were calculated based on the standard curve.
2.5 S. suis cps 2 whole blood bacterial survival assay
Survival of S. suis cps 2 strain 10 in porcine blood ex vivo was determined following the protocol of Seele et al., 2013 (25). Briefly, 500 μL of heparinized blood was mixed with 1×106 CFU of exponentially grown bacteria. CFU were determined by serial dilutions at time zero and after incubation of the samples in a rotator for 2 hours at 37°C. The survival factor was defined as the ratio of CFU at 120 min to CFU at time zero. Where indicated, 10 µg of rIdeSsuis_h was added to evaluate the impact of IgM on bacterial survival in blood of pigs at four and eight weeks of age.
2.6 Phenotyping of B cell subpopulations by flow cytometric analysis
The frequency of conventional and non-conventional B cell subpopulations in peripheral blood was determined in the same pigs at four and eight weeks of age by flow cytometry. A fixable viability dye (eFluor 506, Thermo Fisher Scientific, Carlsbad, USA) was used according to the manufacturer’s protocol to allow for discrimination between viable and dead cells. To prevent binding via Fc receptors, cells were incubated with heat-inactivated normal serum derived from pig (30% in PBS). All surface staining steps described below were performed for 20 min on ice in the dark and separated by washing steps with FACS buffer (PBS, 3% FBS, 0.1% sodium azide (Merck-Sigma); 500×g, 3 min, 4°C). Detailed information regarding the applied primary and secondary antibodies for flow cytometric staining is provided in Table 1. Initially, cells were incubated with anti-porcine CD11R1, anti-porcine IgG, and anti-porcine IgM. Detection of CD11R1 was achieved using a fluorescently labeled anti-mouse IgG1 secondary antibody (Table 1). In the final surface staining step, cells were incubated with a mixture containing the remaining primary antibodies (anti-CD21, anti-CD3) as well as secondary antibodies for detection of IgM and IgG (Table 1). For intracellular staining of the pan-B-cell marker CD79a (Table 1), cells were permeabilized using the FoxP3/Transcription Factor Staining Buffer Set (Thermo Fisher Scientific, Carlsbad, USA) according to the manufacturer’s protocol. After permeabilization, an additional blocking step with 30% heat-inactivated porcine normal serum was done and cells were incubated with the staining antibody for 30 min at RT. Following acquisition with a BD LSR Fortessa™ flow cytometer (Becton Dickinson (BD), Heidelberg, Germany) equipped with BD FACS Diva ™ software version 6.1.3 (BD), data were analyzed using the FlowJo™10.10 software (BD). After exclusion of doublets and dead cells, PBMC were gated on CD79a+IgM+ B lymphocytes and the frequency of non-conventional IgM+CD21-CD11R1+ and IgM+CD21-CD11R1- B-1-like subpopulations as well as of conventional IgM+CD21+ B-2 cells was analyzed. The frequency of IgG+ B cells was analyzed for comparison. Adequate gating was performed according to Fluorescence Minus One (FMO) controls included in the experiments.
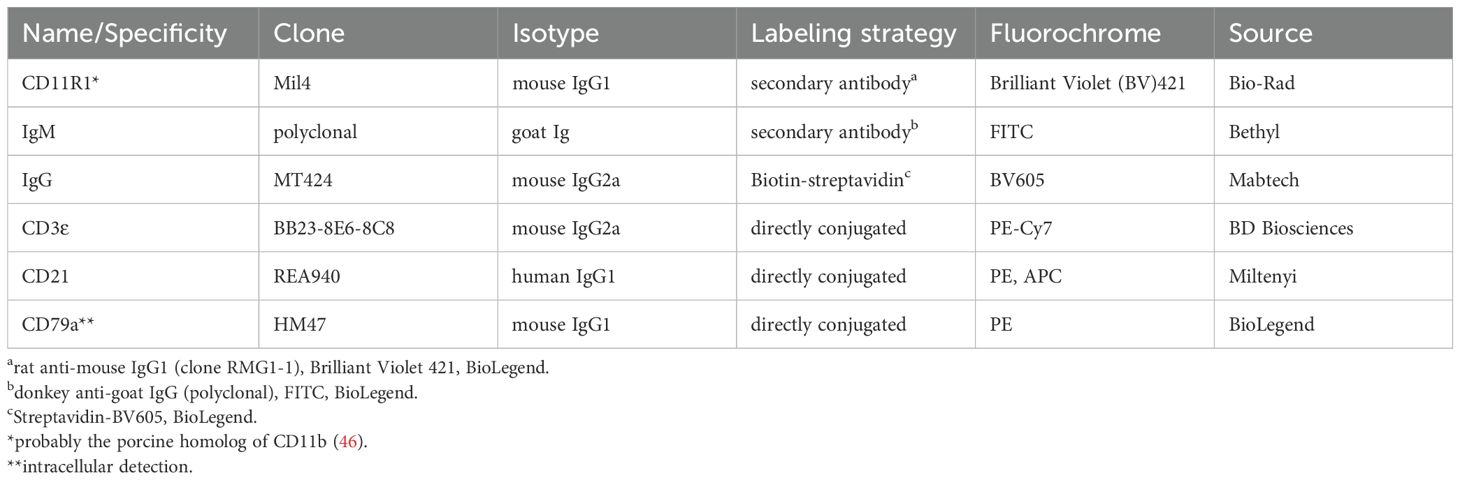
Table 1. Antibodies and reagents used for flow cytometric analysis and fluorescent activated cell sorting.
2.7 Sorting of B cell subpopulations
The different conventional and non-conventional B cell subpopulations were isolated from PBMC of the same individual pigs at four and eight weeks of age by fluorescence-activated cell sorting (FACS). Viability dye and surface staining (Table 1) were performed as described above, except for that FACS buffer was replaced by PBS, 3% FCS (without sodium azide) in the protocol. Stained cells were passed through a 30 µm filter (Sysmex Germany GmbH, Norderstedt, Germany) and immediately sorted through a 85 μm nozzle using a BD FACSAria™ III Cell Sorter (BD, Heidelberg, Germany) equipped with BD FACS Diva ™ software version 6.1.3. Four B cell subpopulations were collected: IgG+, conventional IgM+CD21+ B-2 as well as non-conventional IgM+CD21-CD11R1+ and IgM+CD21-CD11R1- B-1-like cells. Purity was determined by post-sort re-analysis using FlowJo™ 10.10 software (BD).
2.8 Stimulation of sorted B cell subpopulations
The four sorted B cell subpopulations were seeded at a density of 5×104 per well in culture medium (IMDM with L-Glutamine, 25 mM HEPES and 3.024 g/L NaHCO3; PAN-Biotech) supplemented with 10% heat-inactivated FBS, 100 U/mL penicillin, and 100 μg/mL streptomycin (both purchased from PAA Laboratories, Cölbe, Germany) containing 50 ng/µL porcine IL-2 (BioLegend, San Diego, United States) into a round-bottom 96-well cell culture plate (TPP, Trasadingen, Switzerland). To evaluate the IgM response of sorted B cell subpopulations to Toll-like-receptor (TLR) stimulation and T cell-associated signals, cells were stimulated with 10 µg/mL Pam3Cys-SK4 (InvivoGen, Toulouse, France) or 1 µg/mL human CD40L plus 50 ng/mL human IL-21 (both: BioLegend), respectively. To promote cell sedimentation, plates were centrifuged at 400×g for 2 min before start of culture (29). B cell subpopulations were incubated for 3 days at 37°C and 5% CO2. The viability of the different B cell subpopulations was determined using a viability dye by flow cytometry after culture. Importantly, the viability of the different B cell subpopulations was comparable for the individual stimulation conditions allowing for a comparison of IgM secretion.
2.9 Statistical analysis
Statistical analysis was performed using GraphPad Prism 10.2.0 (GraphPad Software Inc., San Diego, USA). The Shapiro-Wilk test was applied to test for normal distribution. Normally distributed data are presented with means. Nonparametric data are shown with medians. Depending on normal distribution, the paired t-test or the Wilcoxon matched-pairs signed-rank test (both: two-tailed) were used for statistical analysis. Significance of stimulation-induced effects was calculated for each subpopulation by direct comparison of medium versus the CD40L plus IL-21 or Pam3Cys-SK4-stimulated equivalent. A Pearson correlation analysis was conducted between S. suis-binding IgM and total IgM. The level of confidence for significance is shown in figure legends.
3 Results
3.1 S. suis cps 2-binding serum IgM increases in pigs between four and eight weeks of age and restricts survival of streptococci
Since invasive infections of S. suis mainly affects pigs at the age of five to ten weeks, understanding changes in the immune status after the forth week of life is of great importance. Here, in healthy, weaned pigs naturally colonized with different serotypes of S. suis, including cps 2, we analyzed anti-S. suis cps 2 antibody levels during this critical period.
While S. suis cps 2-binding IgM was low in 4-weeks-old pigs, it significantly increased until eight weeks of age (Figure 1A). In contrast, anti-S. suis cps 2 IgG decreased over the same period (Figure 1A), indicating stable IgM production without indication of isotype switching to IgG between four and eight weeks. These findings are in line with previous reports showing a similar course of anti-S. suis IgM and IgG levels in porcine blood for other serotypes (cps 1, 7, 14/1) (30, 31). Using an established in vitro whole blood bacterial survival assay (30), comparable survival of S. suis cps 2 was observed in the blood of the same pigs at four and eight weeks of age (Supplementary Figure 1A). To elucidate the role of IgM in control of S. suis cps 2 in porcine blood in vitro, IdeSsuis, a highly specific protease cleaving porcine IgM, but not IgG (25), was added in the whole blood bacterial survival assay. As expected from the low serum IgM levels (Figure 1A), incubation with the IgM protease did not alter the survival of S. suis cps 2 in blood of 4-weeks-old pigs (Supplementary Figure 1B). In contrast, presence of IdeSsuis significantly increased the survival of cps 2 in blood of the same pigs at the age of eight weeks (Figure 1B). In blood samples of five out of six 8-week-old pigs, even an increase in the survival factors above values of one was observed after addition of the IgM protease, indicating proliferation of S. suis cps 2 upon IgM cleavage (Figure 1B). Comparable survival of S. suis cps 2 in the blood of the same pigs at four and eight weeks of age (Supplementary Figure 1A) likely can be attributed to higher maternal anti-S. suis IgG levels in the former and higher anti-S. suis IgM levels in the latter (Figure 1A). In serum from 8-weeks-old pigs, levels of anti-S. suis IgM and total IgM correlated (Supplementary Figure 2).
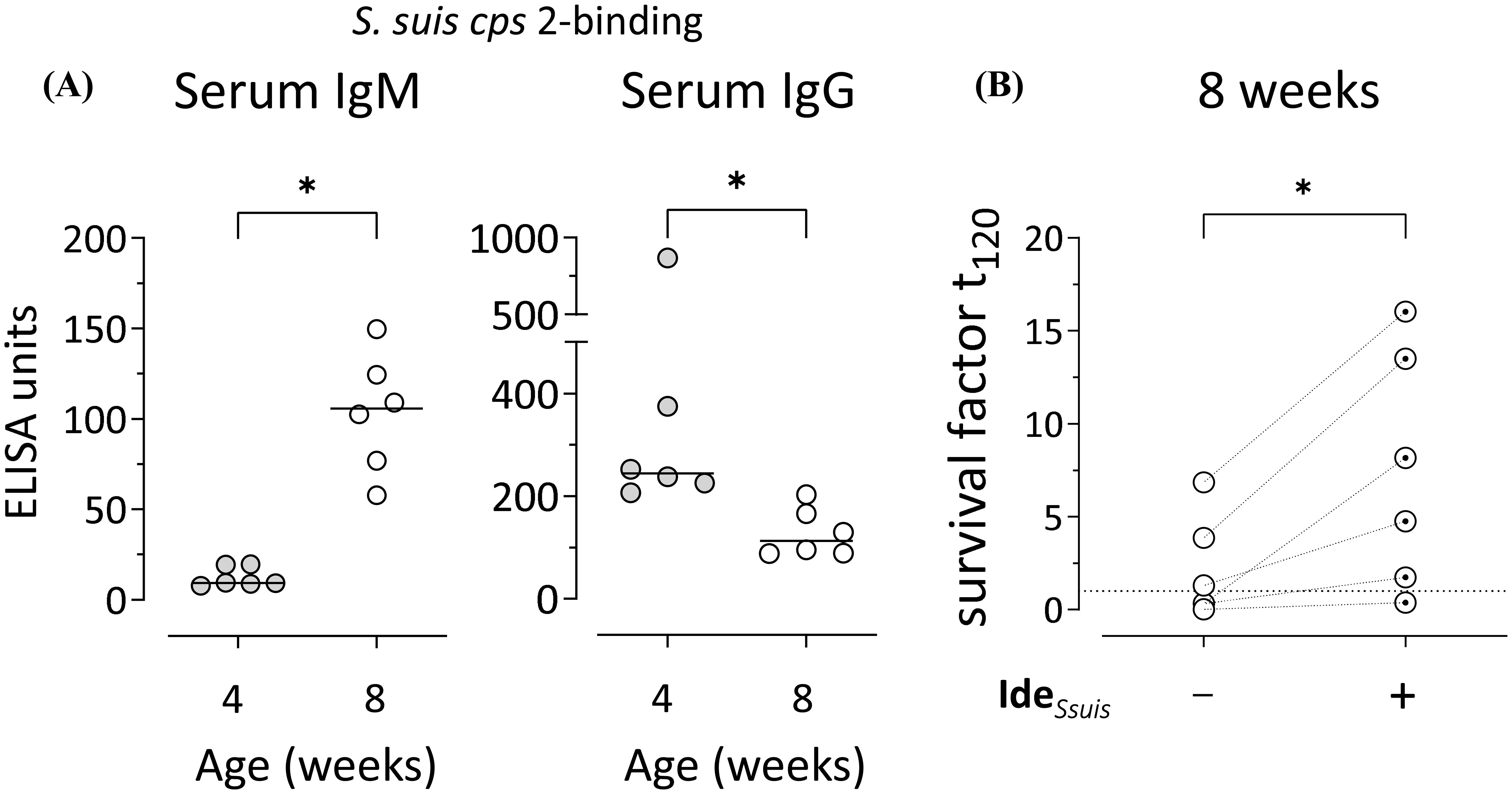
Figure 1. S. suis-binding serum IgM increases between four and eight weeks of age and restricts bacterial survival in vitro. (A) S. suis cps 2-binding serum IgM and IgG were analyzed in the same pigs at four and eight weeks of age by ELISA. Plates were coated with whole inactivated bacteria of S. suis. A hyperimmune serum pool defined to include 100 ELISA units served as internal reference. Data of one out of two independent experiments are shown and presented with group medians. Statistical analysis was performed using Wilcoxon matched-pairs signed-rank test. (two-tailed, *p<0.05). (B) Survival of S. suis cps 2 strain 10 was assessed in the blood drawn from eight-weeks-old pigs in the presence/absence of the specific IgM protease IdeSsuis. The survival factor represents the ratio of CFU at 120 min to CFU at time zero. Survival factors above one (dotted line) indicate proliferation of S. suis. Data of one out of two independent experiments are shown. Each dot represents one individual pig. Statistical analysis was performed using paired t-test. (two-tailed, *p<0.05).
Taken together, our data indicate that the increase of anti-S. suis cps 2 IgM in porcine serum after the fourth week of life contributes to the restriction of survival and proliferation of the bacterial pathogen.
3.2 Relative increase of non-conventional B-1-like subpopulations in the peripheral blood of pigs between four and eight weeks of age
Next we were interested in the cellular source of anti-S. suis IgM. In mice, non-conventional B-1 cells produce protective IgM antibodies against S. pneumoniae (23). Here, we applied a multicolor flow cytometric staining protocol (24) to identify conventional and non-conventional B cell subpopulations in the peripheral blood of the same pigs at four and eight weeks of age (Figure 2A). PBMC from the same pigs whose serum IgM and IgG data are shown in Figure 1 were used. After excluding doublets and dead cells in flow cytometric analysis, we identified B cells using CD79a as a marker and analyzed four B cell subpopulations: non-conventional IgM+CD21−CD11R1+ and IgM+CD21−CD11R1− B-1-like cells as well as conventional IgM+CD21+, and IgG+ B-2 cells. Our analysis revealed an increase in the frequency of non-conventional IgM+CD21−CD11R1+ (mean 1.8-fold) and IgM+CD21−CD11R1− (mean 3.0-fold) B-1-like cells in porcine blood between four and eight weeks of age, while a relative decrease of conventional IgM+CD21+ B-2 cells was observed (Figure 2). The relative proportion of IgG+ B-2 cells also decreased in this period (Figure 2). Thus, the significant increase of S. suis cps 2-binding IgM in peripheral blood of four to eight weeks old pigs (Figure 1) is associated with an increase of the frequency of non-conventional IgM+CD21− B-1-like cells, most pronounced for the CD11R1− subpopulation (Figure 2B).
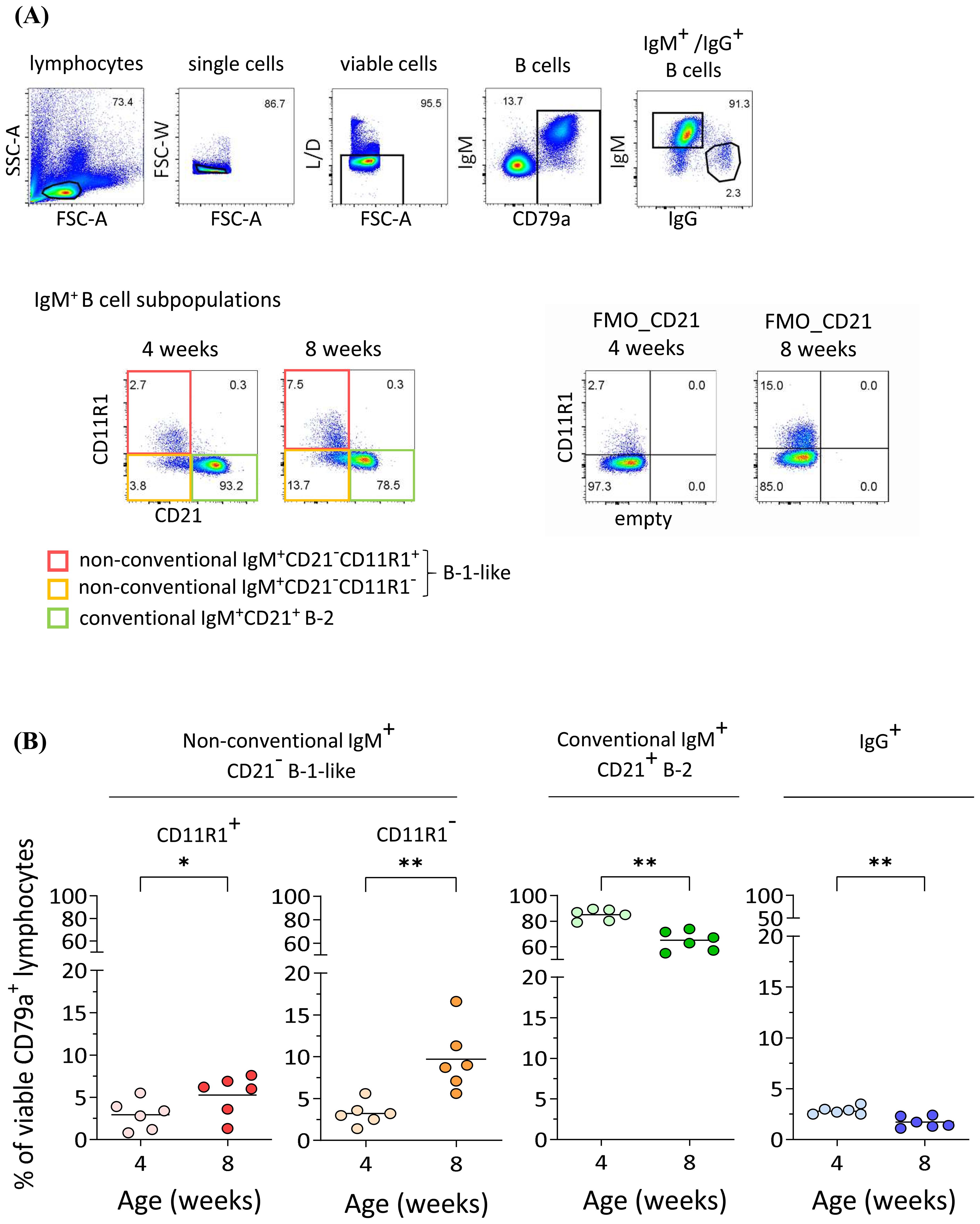
Figure 2. Increase of non-conventional IgM+CD21- B-1-like cell populations in peripheral blood of pigs between four and eight weeks of age. (A) The frequency of conventional and non-conventional IgM+ B cell subpopulations in peripheral blood in the same pigs at four and eight weeks of age was determined by flow cytometry. Representative pseudocolor plots of PBMC gated on CD79a+IgM+ B lymphocytes after exclusion of doublets and dead cells show an increase in the frequency of both, non-conventional IgM+CD21-CD11R1+ and IgM+CD21-CD11R1- B-1-like subpopulations, and a relative decrease in conventional IgM+CD21+ B-2 cells. The frequency of IgG+ B cells was analyzed for comparison. FMO: fluorescence minus one control. (B) Quantification of indicated B cell subpopulations in peripheral blood of the same pigs at four and eight weeks of age. Data of one out of two independent experiments are shown. Each dot represents one individual pig, the horizontal bars indicate mean values. Paired t-test was performed for statistical analysis (two-tailed, *p<0.05, **p < 0.01).
3.3 Porcine IgM+CD21−CD11R1− B-1-like cells constitutively secrete IgM and are highly reactive to toll-like receptor (TLR) stimulation and T cell-associated signals
To assess the potential of IgM secretion by the individual IgM+ B cell subpopulations, conventional IgM+CD21+ B-2 cells as well as non-conventional IgM+CD21−CD11R1+ and IgM+CD21−CD11R1− B-1-like cell subpopulations were isolated by fluorescence-activated cell sorting from PBMC of 8-weeks-old pigs with high purity (Figure 3A). IgG+ B cells were isolated for control (Figure 3A). The sorted B cell subpopulations were incubated in medium for three days or stimulated with either the Toll-like receptor (TLR) 1/2 ligand Pam3Cys-SK4 to analyze innate B cell activation or, to characterize adaptive T cell dependent B cell activation, with the T cell-associated factors CD40L and IL-21 (32). Responsiveness to TLR stimulation is a well-known feature of B-1 cells (24, 33, 34) and murine B-1 cells have also been shown to respond to T cell signals in vitro (35). Of note, analysis of total IgM in supernatants of purified B cell subpopulations by ELISA revealed spontaneous IgM secretion under medium condition only by non-conventional IgM+CD21−CD11R1− B-1-like cells (Figure 3B). Moreover, IgM+CD21−CD11R1− B-1-like cells responded to TLR stimulation with highest IgM production (Figure 3B). Conventional IgM+CD21+ B-2 and non-conventional IgM+CD21−CD11R+ B-1-like cells also secreted IgM following TLR stimulation, albeit to a slightly lesser extent (Figure 3B). Similar to conventional IgM+CD21+ B-2 cells and in contrast to the IgM+CD21−CD11R1+ B-1-like subpopulation, IgM+CD21−CD11R− B-1-like cells secreted high amounts of IgM in response to the T cell-associated signals CD40L and IL-21 (Figure 3B). As expected IgM was not detectable in supernatants from sorted IgG+ B cells (Figure 3B). Thus, IgM+CD21−CD11R1− B-1-like cells are not only a potent source of induced IgM under innate and adaptive B cell stimulation conditions, but also constitutively secrete IgM conceivable with pronounced innate features of this B cell subpopulation.
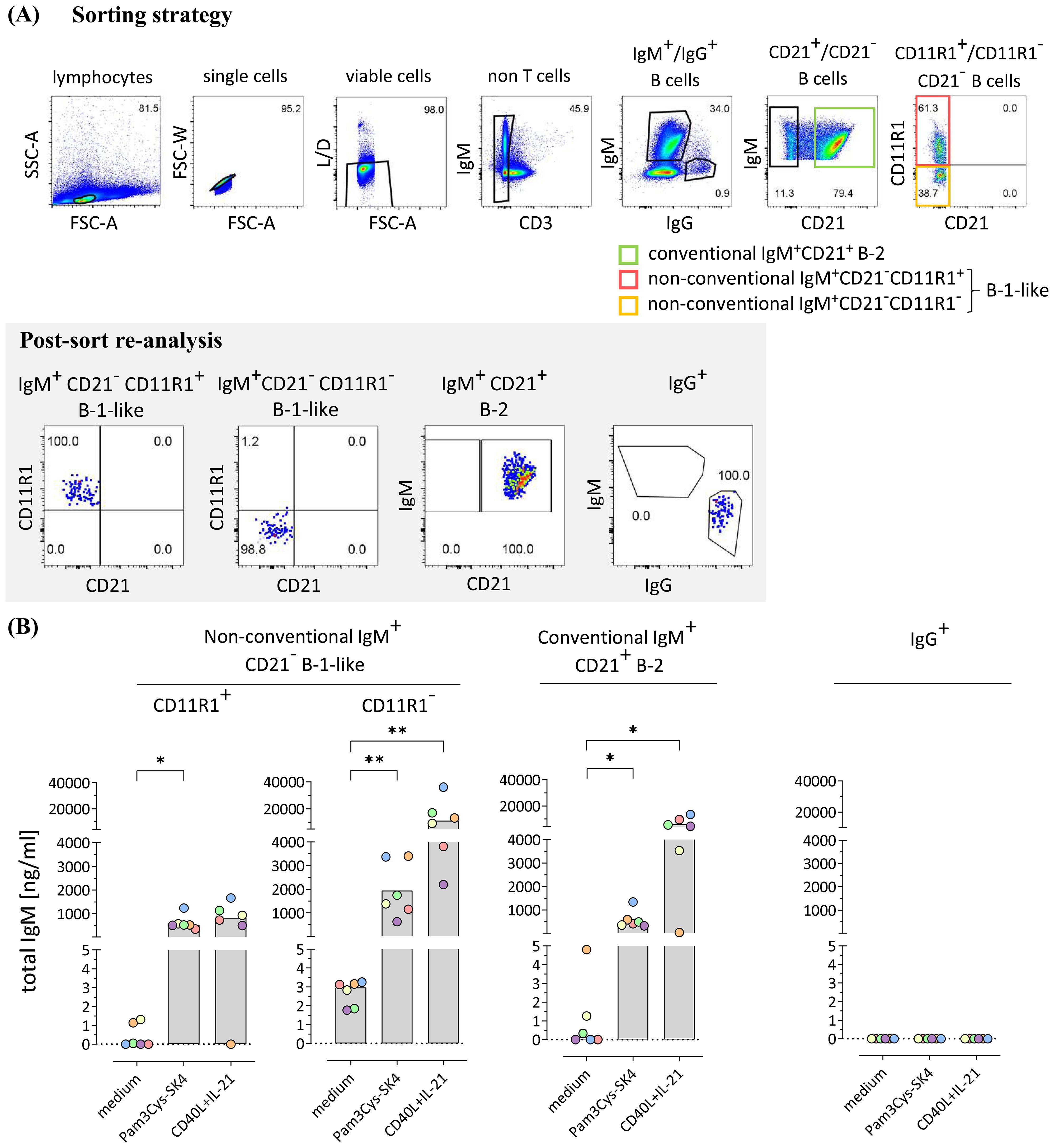
Figure 3. Porcine CD21-CD11R1- B-1-like cells are capable of constitutive IgM production and secrete high amounts of IgM in response to toll-like receptor (TLR) stimulation and T cell-associated signals (A) The strategy for isolation of different B cell subpopulations from peripheral blood mononuclear cells of 8-weeks-old pigs by fluorescence-activated cell sorting is shown. After gating on single viable CD3- lymphocytes, conventional IgG+ as well as IgM+CD21+ B-2 and non-conventional IgM+CD21− B-1-like B cell subpopulations were selected. The latter were further divided according to CD11R1 expression to obtain four different fractions: (1) IgM+CD21− CD11R1+, (2) IgM+CD21− CD11R1−, (3) IgM+CD21+, and (4) IgG+. High purity (>98%) of the isolated populations was confirmed by re-analysis. Representative data of one pig are shown. Numbers in gates depict percentages. (B) The sorted conventional and non-conventional B cell subpopulations were incubated for three days in medium or stimulated with either the TLR ligand Pam3Cys-SK4 or the T cell-associated factors CD40L and IL-21 in the presence of IL-2. Secretion of total IgM was analyzed by ELISA. Depending on normal distribution, data sets are presented with the mean (CD11R1+) or the median. Significance of stimulation-induced effects was calculated for each subpopulation by direct comparison of medium versus the Pam3Cys-SK4 or CD40L plus IL-21 stimulated equivalent using the paired t test or the Wilcoxon matched-pairs signed-rank test, respectively (both: two-tailed, *p<0.05, **p<0.01).
3.4 Non-conventional IgM+CD21− B-1-like cells secrete S. suis-binding IgM upon TLR stimulation in contrast to conventional IgM+CD21+ B-2 cells, with highest levels in the CD11R1− subpopulation
Next, we investigated whether the constitutively produced IgM detected in the supernatant of IgM+CD21−CD11R1− B-1-like cells and the stimulation-induced IgM detected in supernatants of conventional and non-conventional B cell subpopulations bind to S. suis cps 2. For that purpose, ELISA plates were coated with whole inactivated bacteria and supernatants analyzed for total IgM (Figure 3B) were additionally analyzed for binding to S. suis cps 2. As shown in Figure 4, constitutively produced IgM binding to S. suis cps 2 was not detectable (Figure 4) likely due to limited amounts of total IgM as compared with the >700-fold higher stimulation-dependent levels (see Figure 3B for difference between constitutive and induced total IgM secretion). Noteworthy, in contrast to conventional IgM+CD21+ B-2 cells, both non-conventional IgM+CD21− B-1-like cell subpopulations secreted anti-S. suis IgM following stimulation with the TLR 1/2 ligand Pam3Cys-SK4 (Figure 4). Furthermore, upon stimulation with the T cell-associated factors CD40L and IL-21, the non-conventional IgM+CD21−CD11R1− B-1-like cell subpopulation demonstrated a higher potential to produce IgM that binds to S. suis cps 2 compared to non-conventional IgM+CD21−CD11R1+ and to conventional IgM+CD21+ cells (Figure 4). Taken together, conventional and non-conventional B cell subpopulations of eight-weeks-old pigs are able to secrete anti-S. suis IgM with highest levels produced by IgM+CD21−CD11R1− B-1-like cells.
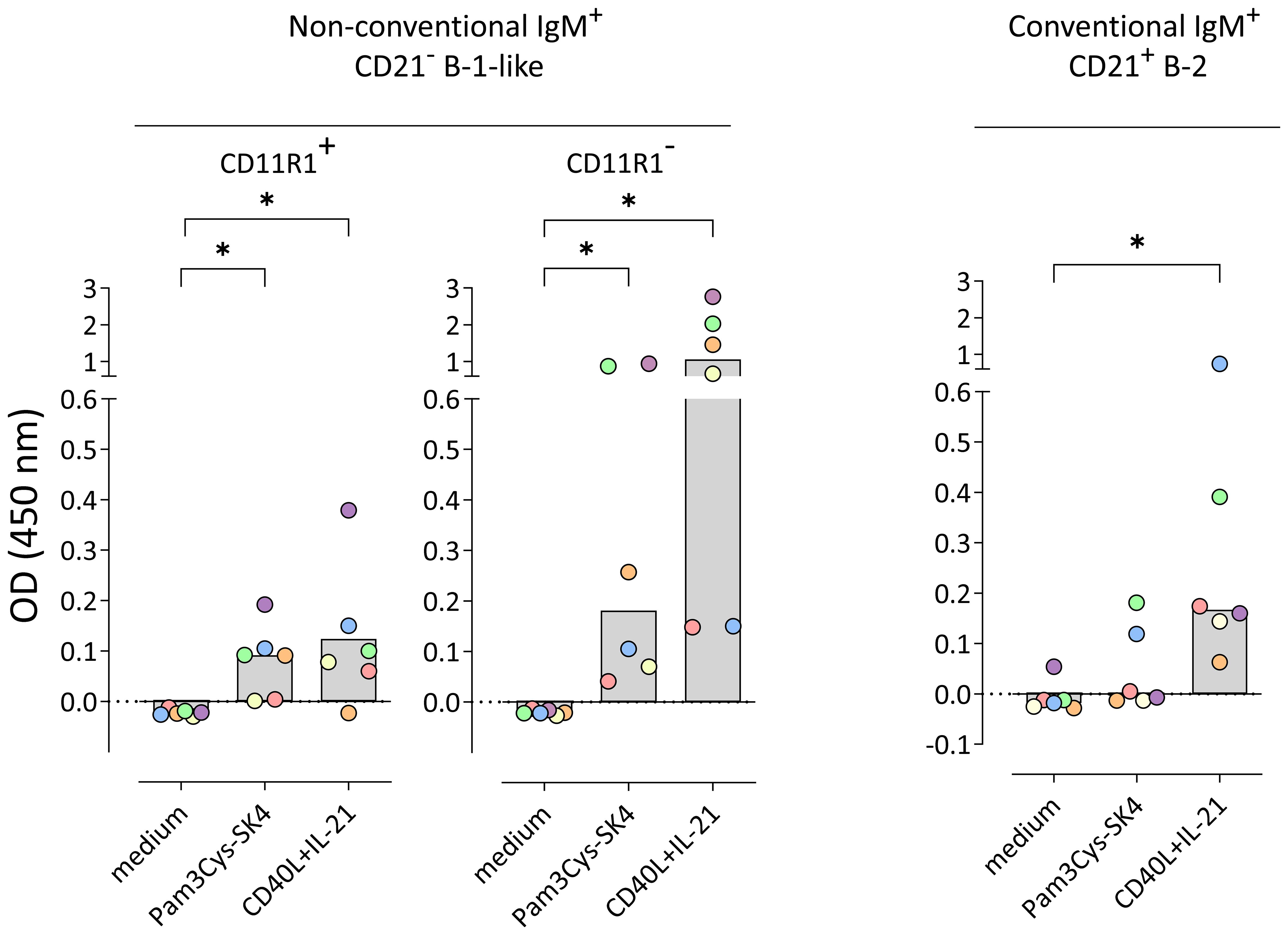
Figure 4. Non-conventional IgM+CD21− B-1-like cells but not conventional IgM+CD21+ B-2 cells isolated from peripheral blood of 8-weeks-old pigs secrete S. suis cps 2-binding IgM in response to toll-like receptor (TLR) stimulation, with highest levels in the CD11R1− subpopulation. Conventional and non-conventional IgM+ B cell subpopulations were sorted in four independent experiments from a total of six 8-week-old pigs. Isolated B cell subpopulations were incubated for three days in medium or stimulated with either the TLR ligand Pam3Cys-SK4 or the T cell-associated factors CD40L and IL-21 in the presence of IL-2. The amount of S. suis cps 2-binding IgM in supernatants was determined using whole inactivated bacteria as coating antigen. Blank-reduced optical densities (OD) are shown. Each colored dot represents one individual pig. Depending on normal distribution, data sets are presented with the mean or the median. Significance of stimulation-induced effects was calculated for each subpopulation by direct comparison of medium versus the Pam3Cys-SK4 or CD40L plus IL-21 stimulated equivalent using the paired t test or the Wilcoxon matched-pairs signed-rank test, respectively (both: two-tailed, *p<0.05).
4 Discussion
S. suis cps 2 is one of the most important bacterial pathogens in piglet nurseries. IgM antibodies are known to be crucial for host defense against S. suis during the critical phase after weaning (15, 16, 30). The characteristic course of anti-S. suis-binding IgM antibodies crucial for bacterial clearance, published for different serotypes (cps 1, 7, 14/1) previously (30, 31), was confirmed for cps 2 in the present study: in the 4th week of life, levels of anti-S. suis cps 2 IgM are very low in porcine blood. In the following four weeks, there is an increase in IgM, which restricts bacterial growth in vitro. Furthermore, we show for the first time, that in pigs, the significant increase of S. suis-binding serum IgM between four and eight weeks of age is associated with a relative increase of peripheral non-conventional IgM+CD21− B-1-like cells in vivo, particularly of the IgM+CD21 CD11R1− subpopulation.
The two distinct subpopulations of porcine B-1-like cells, that differ with respect to the expression of CD11R1 (probably the porcine homolog of CD11b), have been described previously (24). In the present study the porcine IgM+CD21−CD11R1− subpopulation, isolated from peripheral blood of 8-weeks-old pigs, was shown to constitutively secrete IgM, as described for human and murine innate B-1 cells (36, 37). Interestingly, despite the fact that comparable levels of total IgM were produced in response to TLR stimulation in porcine conventional and non-conventional B cell subpopulations, only TLR-induced IgM secreted by the two non-conventional IgM+CD21− B-1-like cell subpopulations bound to S. suis cps 2. This highlights differences in reactivities of IgM antibodies secreted by TLR-activated B-1 versus B-2 cells likely due to distinct IgM BCR repertoires expressed by B-1 and B-2 cells. As observed in mouse B-1 cells (38), the concentrations of total IgM spontaneously secreted by porcine IgM+CD21−CD11R1− B-1-like cells were very low, which might be the reason why S. suis cps 2-binding IgM was undetectable in their supernatants. Taken together, in contrast to conventional IgM+CD21+ B-2 cells, the IgM+CD21− CD11R1− B-1-like cell subpopulation constitutively secretes IgM and both non-conventional IgM+CD21− B cell subpopulations (but not B-2 cells) produce anti-S. suis IgM upon TLR stimulation underlining their innate-like characteristics. Future experiments, including a detailed transcriptome and repertoire analysis will provide further insights into specific features of the two non-conventional B-1-like subpopulations in comparison to conventional B-2 cells. This can reveal whether porcine non-conventional B-1-like cells express a skewed variable heavy chain (VH) repertoire as shown for murine and human B-1 cells (39–41).
Interestingly, the increase in serum IgM between the fourth and eighth week of age without a corresponding rise in IgG indicates the absence of isotype class switch during this period. Stable IgM production without class switch is a characteristic feature of B-1 cells in mice (42). After experimental infection with S. suis there is an immediate increase in serum IgM, yet even 21 days post infection serum IgG does not increase significantly (43). In mice experimentally infected with S. suis cps 2, control of infection was mediated by S. suis-binding IgM and independent of germinal center reaction and of isotype switching. Nevertheless, production of anti-bacterial IgM antibodies was shown to be T cell-dependent, as T cell deficient mice could not control bacteremia, produce optimal anti-S. suis IgM titers, or elicit antibodies with opsonophagocytic activity (15). Interestingly, in light of this murine study indicating T cell dependent extrafollicular induction of anti-S. suis IgM, we observed that porcine B-1-like as well as B-2 cell subpopulations are capable of secreting S. suis-binding IgM upon stimulation with T cell-associated factors with highest levels in IgM+CD21−CD11R1− B-1-like cells. Secreted IgM from all three subpopulations binds to S. suis and may therefore also play a role in bacterial killing in vivo.
Presently, we can only speculate on the specificity of the S. suis cps 2-binding IgM. A recent study indicates biological relevance of cross-reactivity of antibodies between the different S. suis serotypes cps 1 and cps 14 (31). Given that the capsule of S. suis cps 2 shares similarities with that of cps 1 and cps 14 (44), we hypothesize that the S. suis cps 2-binding IgM is not exclusively specific for cps 2 but may also bind to other S. suis serotypes. Noteworthy, the characteristic increase of serum IgM antibodies critical for bacterial clearance in pigs between four and eight weeks of age has not only been shown for different S. suis serotypes, but also for Klebsiella pneumoniae (45). Murine and human B-1 cells are the main producers of polyreactive IgM antibodies (20). It could be speculated that in pigs between the fourth and eighth week of life, B-1-like cells with innate features are also a major source of polyreactive IgM and that the antibacterial activity is not restricted to S. suis only but contributes to protection against different bacterial pathogens. However, this needs further investigation.
In conclusion, this is the first characterization of the porcine early IgM B cell response which identifies an increase of B-1-like cells in porcine peripheral blood between four and eight weeks of age concomitant with the increase of anti-S. suis IgM restricting bacterial growth in vitro. Our study provides the basis for future experiments which should reveal novel insights into the regulation of non-conventional and conventional B cell subpopulations in porcine early life host-pathogen interaction.
Data availability statement
The original contributions presented in the study are included in the article/Supplementary Material. Further inquiries can be directed to the corresponding author.
Ethics statement
The animal study was approved by North Rhine-Westphalia State Agency for Nature, Environment and Consumer Protection (Landesamt für Natur, Umwelt und Verbraucherschutz Nordrhein-Westfalen) under the approval number 81-02.04.40.2023.VG034. The study was conducted in accordance with the local legislation and institutional requirements.
Author contributions
A-MS: Data curation, Formal analysis, Funding acquisition, Investigation, Methodology, Visualization, Writing – original draft, Writing – review & editing. JK: Resources, Writing – review & editing. MP: Methodology, Writing – review & editing. CB: Conceptualization, Funding acquisition, Project administration, Resources, Writing – review & editing. GA: Conceptualization, Funding acquisition, Project administration, Resources, Supervision, Writing – review & editing. ME: Data curation, Formal analysis, Investigation, Methodology, Supervision, Writing – original draft, Writing – review & editing.
Funding
The author(s) declare financial support was received for the research, authorship, and/or publication of this article. This project was funded by grants from the German Research Foundation (Deutsche Forschungsgemeinschaft, DFG), AL 371/10-2 to UM, GA and BA 4730/3-2 to CB and by a doctoral stipend from the Heinrich-Wilhelm Schaumann Fondation to A-MS. The authors acknowledge support from the German Research Foundation (DFG) and Leipzig University within the program of Open Access Publishing.
Acknowledgments
The authors thank Dr. H. Smith (Wageningen University and Research, Lelystad, Netherlands) for providing S. suis strain 10. Theresa Middendorf from the Institute of Bacteriology and Mycology, College of Veterinary Medicine, Leipzig University is thanked for support in collection of blood samples. The authors thank Aileen Wingenfeld for her excellent technical assistance. We acknowledge Dr. C. Schnabel, Dr. U. Müller, and Dr. M. Büttner for critical reading of the manuscript. Flow cytometry was conducted at the core unit for flow cytometry (CUDZ) of the Faculty of Veterinary Medicine, Leipzig University, maintained by Dr. U. Müller.
Conflict of interest
The authors declare that the research was conducted in the absence of any commercial or financial relationships that could be construed as a potential conflict of interest.
Publisher’s note
All claims expressed in this article are solely those of the authors and do not necessarily represent those of their affiliated organizations, or those of the publisher, the editors and the reviewers. Any product that may be evaluated in this article, or claim that may be made by its manufacturer, is not guaranteed or endorsed by the publisher.
Supplementary material
The Supplementary Material for this article can be found online at: https://www.frontiersin.org/articles/10.3389/fimmu.2024.1495359/full#supplementary-material
References
2. Vötsch D, Willenborg M, Weldearegay YB, Valentin-Weigand P. Streptococcus suis - the “Two faces” of a pathobiont in the porcine respiratory tract. Front Microbiol. (2018) 9:480. doi: 10.3389/fmicb.2018.00480
3. Wertheim HF, Nghia HD, Taylor W, Schultsz C. Streptococcus suis: an emerging human pathogen. Clin Infect Dis. (2009) 48:617–25. doi: 10.1086/596763
4. Sanford SE, Tilker ME. Streptococcus suis type II-associated diseases in swine: observations of a one-year study. J Am Vet Med Assoc. (1982) 181:673–6.
5. Gottschalk M, Xu J, Calzas C, Segura M. Streptococcus suis: a new emerging or an old neglected zoonotic pathogen? Future Microbiol. (2010) 5:371–91. doi: 10.2217/fmb.10.2
6. Neila-Ibáñez C, Casal J, Hennig-Pauka I, Stockhofe-Zurwieden N, Gottschalk M, Migura-García L, et al. Stochastic assessment of the economic impact of streptococcus suis-associated disease in german, dutch and spanish swine farms. Front Vet Sci. (2021) 8:676002. doi: 10.3389/fvets.2021.676002
7. Goyette-Desjardins G, Calzas C, Shiao TC, Neubauer A, Kempker J, Roy R, et al. Protection against Streptococcus suis Serotype 2 Infection Using a Capsular Polysaccharide Glycoconjugate Vaccine. Infect Immun. (2016) 84:2059–75. doi: 10.1128/IAI.00139-16
8. Okura M, Osaki M, Nomoto R, Arai S, Osawa R, Sekizaki T, et al. Current taxonomical situation of streptococcus suis. Pathogens. (2016) 5:45. doi: 10.3390/pathogens5030045
9. Li K, Lacouture S, Lewandowski E, Thibault E, Gantelet H, Gottschalk M, et al. Molecular characterization of Streptococcus suis isolates recovered from diseased pigs in Europe. Vet Res. (2024) 55:117. doi: 10.1186/s13567-024-01366-y
10. Goyette-Desjardins G, Auger J-P, Xu J, Segura M, Gottschalk M. Streptococcus suis, an important pig pathogen and emerging zoonotic agent-an update on the worldwide distribution based on serotyping and sequence typing. Emerg Microbes Infect. (2014) 3:e45. doi: 10.1038/emi.2014.45
11. Dolbec D, Lehoux M, Okura M, Takamatsu D, Gottschalk M, Segura M. Streptococcus suis surface-antigen recognition by antibodies and bacterial elimination is influenced by capsular polysaccharide structure. Front Cell Infect Microbiol. (2023) 13:1228496. doi: 10.3389/fcimb.2023.1228496
12. Segura M, Calzas C, Grenier D, Gottschalk M. Initial steps of the pathogenesis of the infection caused by Streptococcus suis: fighting against nonspecific defenses. FEBS Lett. (2016) 590:3772–99. doi: 10.1002/1873-3468.12364
13. Benga L, Fulde M, Neis C, Goethe R, Valentin-Weigand P. Polysaccharide capsule and suilysin contribute to extracellular survival of Streptococcus suis co-cultivated with primary porcine phagocytes. Vet Microbiol. (2008) 132:211–9. doi: 10.1016/j.vetmic.2008.05.005
14. Fittipaldi N, Segura M, Grenier D, Gottschalk M. Virulence factors involved in the pathogenesis of the infection caused by the swine pathogen and zoonotic agent Streptococcus suis. Future Microbiol. (2012) 7:259–79. doi: 10.2217/fmb.11.149
15. Dolbec D, Lehoux M, Beauville AA, Zahn A, Di Noia JM, Segura M. Unmutated but T cell dependent IgM antibodies targeting Streptococcus suis play an essential role in bacterial clearance. PloS Pathog. (2024) 20:e1011957. doi: 10.1371/journal.ppat.1011957
16. Rungelrath V, Weiße C, Schütze N, Müller U, Meurer M, Rohde M, et al. IgM cleavage by Streptococcus suis reduces IgM bound to the bacterial surface and is a novel complement evasion mechanism. Virulence. (2018) 9:1314–37. doi: 10.1080/21505594.2018.1496778
17. Seele J, Beineke A, Hillermann L-M, Jaschok-Kentner B, Pawel-Rammingen UV, Valentin-Weigand P, et al. The immunoglobulin M-degrading enzyme of Streptococcus suis, IdeSsuis, is involved in complement evasion. Vet Res. (2015) 46:45. doi: 10.1186/s13567-015-0171-6
18. Goyette-Desjardins G, Lacouture S, Auger JP, Roy R, Gottschalk M, Segura M. Characterization and Protective Activity of Monoclonal Antibodies Directed against Streptococcus suis Serotype 2 Capsular Polysaccharide Obtained Using a Glycoconjugate. Pathogens. (2019) 8:139. doi: 10.3390/pathogens8030139
19. Montecino-Rodriguez E, Dorshkind K. B-1 B cell development in the fetus and adult. Immunity. (2012) 36:13–21. doi: 10.1016/j.immuni.2011.11.017
20. Baumgarth N. The double life of a B-1 cell: self-reactivity selects for protective effector functions. Nat Rev Immunol. (2011) 11:34–46. doi: 10.1038/nri2901
21. Smith FL, Baumgarth N. B-1 cell responses to infections. Curr Opin Immunol. (2019) 57:23–31. doi: 10.1016/j.coi.2018.12.001
22. Leggat DJ, Khaskhely NM, Iyer AS, Mosakowski J, Thompson RS, Weinandy JD, et al. Pneumococcal polysaccharide vaccination induces polysaccharide-specific B cells in adult peripheral blood expressing CD19+CD20+CD3-CD70-CD27+IgM+CD43+CD5+/-. Vaccine. (2013) 31:4632–40. doi: 10.1016/j.vaccine.2013.07.030
23. Haas KM, Poe JC, Steeber DA, Tedder TF. B-1a and B-1b cells exhibit distinct developmental requirements and have unique functional roles in innate and adaptive immunity to S. pneumoniae. Immunity. (2005) 23:7–18. doi: 10.1016/j.immuni.2005.04.011
24. Braun RO, Python S, Summerfield A. Porcine B cell subset responses to toll-like receptor ligands. Front Immunol. (2017) 8:1044. doi: 10.3389/fimmu.2017.01044
25. Seele J, Singpiel A, Spoerry C, Pawel-Rammingen UV, Valentin-Weigand P, Baums CG. Identification of a novel host-specific IgM protease in Streptococcus suis. J Bacteriol. (2013) 195:930–40. doi: 10.1128/JB.01875-12
26. Buhr ND, Reuner F, Neumann A, Stump-Guthier C, Tenenbaum T, Schroten H, et al. Neutrophil extracellular trap formation in the Streptococcus suis-infected cerebrospinal fluid compartment. Cell Microbiol. (2017) 19:e12649. doi: 10.1111/cmi.12649
27. Smith HE, Damman M, van der Velde J, Wagenaar F, Wisselink HJ, Stockhofe-Zurwieden N, et al. Identification and Characterization of thecps Locus of Streptococcus suis Serotype 2: the Capsule Protects against Phagocytosis and Is an Important Virulence Factor. Infect Immun. (1999) 67:1750–6. doi: 10.1128/iai.67.4.1750-1756.1999
28. Rungelrath V, Öhlmann S, Alber G, Schrödl W, Köckritz-Blickwede MV, Buhr ND, et al. Survival of streptococcus suis in porcine blood is limited by the antibody- and complement-dependent oxidative burst response of granulocytes. Infect Immun. (2020) 88:e00598-19. doi: 10.1128/iai.00598-19
29. Marsman C, Verhoeven D, Koers J, Rispens T, ten BA, SM vH, et al. Optimized protocols for in-vitro T-cell-dependent and T-cell-independent activation for B-cell differentiation studies using limited cells. Front Immunol. (2022) 13:815449. doi: 10.3389/fimmu.2022.815449
30. Rieckmann K, Seydel A, Szewczyk K, Klimke K, Rungelrath V, Baums CG. Streptococcus suis cps7: an emerging virulent sequence type (ST29) shows a distinct, IgM-determined pattern of bacterial survival in blood of piglets during the early adaptive immune response after weaning. Vet Res. (2018) 49:48. doi: 10.1186/s13567-018-0544-8
31. Mayer L, Bornemann N, Lehnert S, Greeff AD, Strutzberg-Minder K, Rieckmann K, et al. Survival patterns of Streptococcus suis serotypes 1 and 14 in porcine blood indicate cross-reactive bactericidal antibodies in naturally infected pigs. Vet Microbiol. (2021) 260:109183. doi: 10.1016/j.vetmic.2021.109183
32. Rahe MC, Murtaugh MP. Interleukin-21 drives proliferation and differentiation of porcine memory B cells into antibody secreting cells. PloS One. (2017) 12:e0171171. doi: 10.1371/journal.pone.0171171
33. Ray A, Karmakar P, Biswas T. Up-regulation of CD80-CD86 and IgA on mouse peritoneal B-1 cells by porin of Shigella dysenteriae is Toll-like receptors 2 and 6 dependent. Mol Immunol. (2004) 41:1167–75. doi: 10.1016/j.molimm.2004.06.007
34. Ma K, Du W, Wang S, Xiao F, Li J, Tian J, et al. B1-cell-produced anti-phosphatidylserine antibodies contribute to lupus nephritis development via TLR-mediated Syk activation. Cell Mol Immunol. (2023) 20:881–94. doi: 10.1038/s41423-023-01049-2
35. Erickson LD, Foy TM, Waldschmidt TJ. Murine B1 B cells require IL-5 for optimal T cell-dependent activation. J Immunol. (2001) 166:1531–9. doi: 10.4049/jimmunol.166.3.1531
36. Genestier L, Taillardet M, Mondiere P, Gheit H, Bella C, DeFrance T. TLR agonists selectively promote terminal plasma cell differentiation of B cell subsets specialized in thymus-independent responses. J Immunol. (2007) 178:7779–86. doi: 10.4049/jimmunol.178.12.7779
37. She Z, Li C, Wu F, Mao J, Xie M, Hun M, et al. The role of B1 cells in systemic lupus erythematosus. Front Immunol. (2022) 13:814857. doi: 10.3389/fimmu.2022.814857
38. Suchanek O, Clatworthy MR. Homeostatic role of B-1 cells in tissue immunity. Front Immunol. (2023) 14:1106294. doi: 10.3389/fimmu.2023.1106294
39. Griffin DO, Holodick NE, Rothstein TL. Human B1 cells in umbilical cord and adult peripheral blood express the novel phenotype CD20+CD27+CD43+CD70–. J Exp Med. (2011) 208:67–80. doi: 10.1084/jem.20101499
40. Zhong X, Lau S, Bai C, Degauque N, Holodick NE, Steven SJ, et al. A novel subpopulation of B-1 cells is enriched with autoreactivity in normal and lupus-prone mice. Arthritis Rheum. (2009) 60:3734–43. doi: 10.1002/art.25015
41. Silverman GJ, Shaw PX, Luo L, Dwyer D, Chang M, Horkko S, et al. Neo-self antigens and the expansion of B-1 cells: lessons from atherosclerosis-prone mice. Curr Top Microbiol Immunol. (2000) 252:189–200. doi: 10.1007/978-3-642-57284-5_20
42. Tarlinton DM, McLean M, Nossal GJ. B1 and B2 cells differ in their potential to switch immunoglobulin isotype. Eur J Immunol. (1995) 25:3388–93. doi: 10.1002/eji.1830251228
43. Liedel C, Mayer L, Einspanier A, Völker I, Ulrich R, Rieckmann K, et al. A new S. suis serotype 3 infection model in pigs: lack of effect of buprenorphine treatment to reduce distress. BMC Vet Res. (2022) 18:435. doi: 10.1186/s12917-022-03532-w
44. van Calsteren MR, Goyette-Desjardins G, Gagnon F, Okura M, Takamatsu D, Roy R, et al. Explaining the serological characteristics of streptococcus suis serotypes 1 and 1/2 from their capsular polysaccharide structure and biosynthesis. J Biol Chem. (2016) 291:8387–98. doi: 10.1074/jbc.M115.700716
45. Krieger A-K, Öhlmann S, Mayer L, Weiße C, Rieckmann K, Baums CG. Porcine iucA+ but rmpA- Klebsiella pneumoniae strains proliferate in blood of young piglets but are killed by IgM and complement dependent opsonophagocytosis when these piglets get older. Vet Microbiol. (2022) 266:109361. doi: 10.1016/j.vetmic.2022.109361
Keywords: Streptococcus suis, IgM, pig, swine, non-conventional B cells, innate B-1 cells, B-2 cells, cell culture
Citation: Seidel A-M, Kauffold J, Protschka M, Baums CG, Alber G and Eschke M (2024) Porcine non-conventional B-1-like cells are a potent source of Streptococcus suis-binding IgM. Front. Immunol. 15:1495359. doi: 10.3389/fimmu.2024.1495359
Received: 12 September 2024; Accepted: 28 October 2024;
Published: 18 November 2024.
Edited by:
Falko Steinbach, University of Surrey, United KingdomCopyright © 2024 Seidel, Kauffold, Protschka, Baums, Alber and Eschke. This is an open-access article distributed under the terms of the Creative Commons Attribution License (CC BY). The use, distribution or reproduction in other forums is permitted, provided the original author(s) and the copyright owner(s) are credited and that the original publication in this journal is cited, in accordance with accepted academic practice. No use, distribution or reproduction is permitted which does not comply with these terms.
*Correspondence: Maria Eschke, bWFyaWEuZXNjaGtlQGJiei51bmktbGVpcHppZy5kZQ==