- 1Amgen R&D Postdoctoral Fellows Program, Amgen Inc, South San Francisco, CA, United States
- 2Amgen Research, Amgen Inc., South San Francisco, CA, United States
Tolerogenic vaccines represent a therapeutic approach to induce antigen-specific immune tolerance to disease-relevant antigens. As general immunosuppression comes with significant side effects, including heightened risk of infections and reduced anti-tumor immunity, antigen-specific tolerance by vaccination would be game changing in the treatment of immunological conditions such as autoimmunity, anti-drug antibody responses, transplantation rejection, and hypersensitivity. Tolerogenic vaccines induce antigen-specific tolerance by promoting tolerogenic antigen presenting cells, regulatory T cells, and regulatory B cells, or by suppressing or depleting antigen-specific pathogenic T and B cells. The design of tolerogenic vaccines vary greatly, but they all deliver a disease-relevant antigen with or without a tolerogenic adjuvant. Tolerogenic adjuvants are molecules which mediate anti-inflammatory or immunoregulatory effects and enhance vaccine efficacy by modulating the immune environment to favor a tolerogenic immune response to the vaccine antigen. Tolerogenic adjuvants act through several mechanisms, including immunosuppression, modulation of cytokine signaling, vitamin signaling, and modulation of immunological synapse signaling. This review seeks to provide a comprehensive examination of tolerogenic adjuvants currently utilized in tolerogenic vaccines, describing their mechanism of action and examples of their use in human clinical trials and animal models of disease.
1 Introduction
Immunological tolerance is a state of unresponsiveness or an anti-inflammatory response that promotes immune homeostasis and prevents detrimental immune reactions directed toward self-antigens and tissues (1). Tolerogenic vaccines aim to induce antigen-specific tolerance in conditions where tolerance has failed, or where there is an aberrant inflammatory response toward antigens not associated with danger. Tolerogenic vaccines differ from general immunosuppression or immunomodulation in that they aim to induce specific immune tolerance to the disease-relevant antigens, thereby suppressing the autoimmune response without affecting the immune system as a whole (2, 3). Autoimmune disease, transplantation rejection, anti-drug antibody responses, and hypersensitivity all represent conditions where tolerogenic vaccines are promising new therapeutic regimens (2, 3).
Tolerogenic vaccines can induce antigen-specific tolerance via effects on key players in peripheral tolerance. Mechanisms of immune tolerance are reviewed extensively elsewhere (1, 4, 5). In brief, master regulators of peripheral tolerance toward specific antigens are regulatory T cells (Tregs) and tolerogenic dendritic cells (tolDCs) (1, 3–6). Tregs exert their immunoregulatory effects via anti-inflammatory cytokines, direct suppression of conventional T cell (Tcon) proliferation, and by modulating DC maturation and function. TolDCs act by promoting Treg development, suppressing effector T cell responses, and by inducing antigen-specific T cell anergy. Many other cell types can contribute to immunological tolerance, of note are B cells producing anti-inflammatory cytokines (7) and type 1 regulatory T cells (Tr1 cells) (8).
Adjuvants are molecules used to enhance the effect of pharmacological treatments (9), therefore tolerogenic adjuvants aim to increase anti-inflammatory responses and enhance vaccine efficacy. A schematic overview of the main mode(s) of action for tolerogenic adjuvants described in this review are depicted in Figure 1. Tolerogenic vaccines use adjuvants in different ways depending on vaccine type. Antigen and adjuvants can be administered either separately or co-delivered, where the co-delivery can range from simultaneous administration of free antigen and adjuvant to intricate delivery systems, fusion molecules, or DNA vectors (Figure 2). Tolerogenic adjuvants can also be used to differentiate tolDCs for cell transfer of antigen-loaded tolDCs (Figure 2).
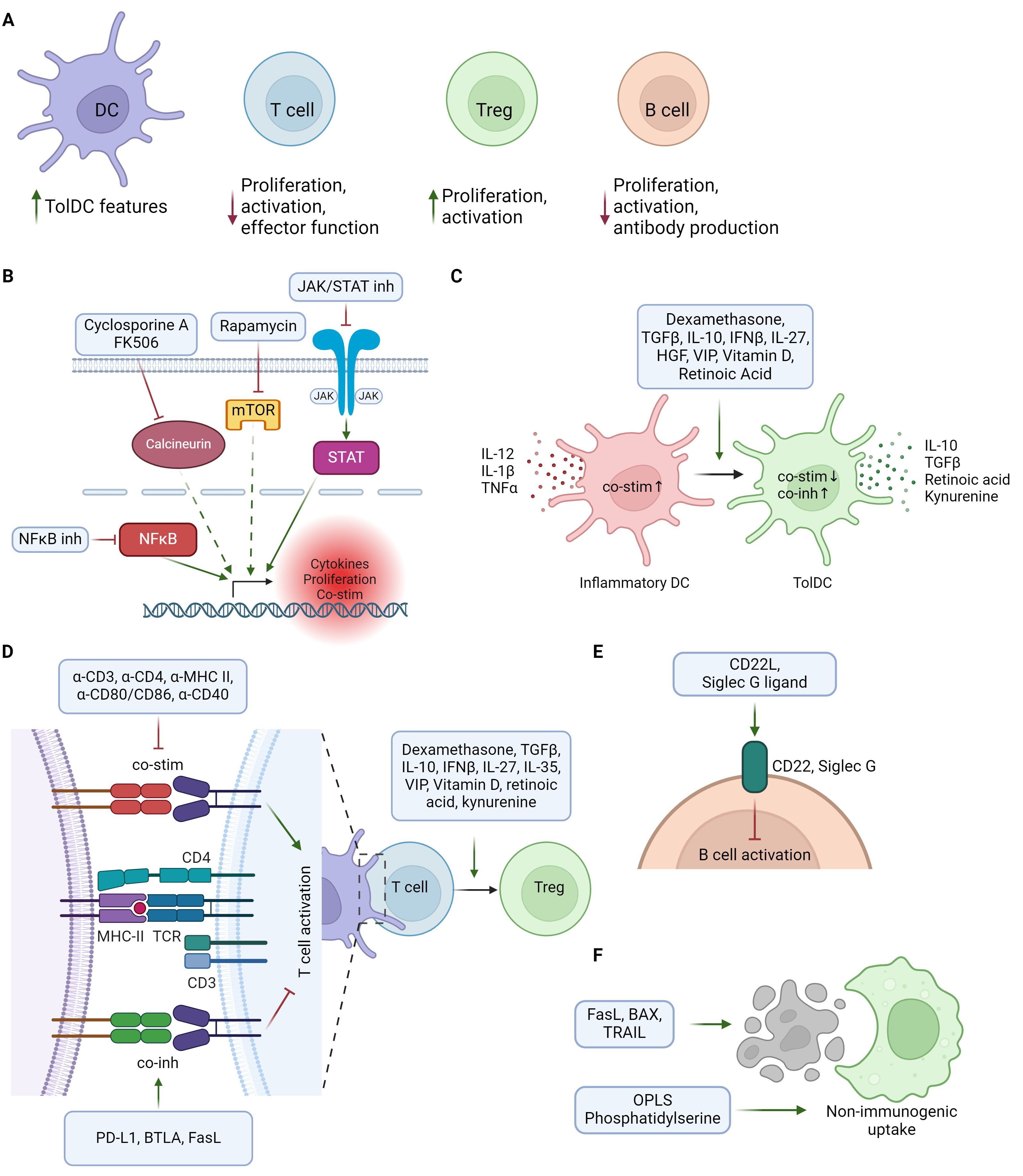
Figure 1. Schematic overview of tolerogenic adjuvants mechanisms of action. (A) Overview of main tolerogenic effects of adjuvants on different immune cell types, including suppression of immune activation and promotion of tolerogenic/immunoregulatory cells. (B) Immunoregulation through direct or indirect suppression of pro-inflammatory gene expression. (C) Adjuvants supporting differentiation from inflammatory DCs (red) to tolerogenic DCs (green). TolDCs are characterized by lower levels of co-stimulatory and increased levels of co-inhibitory molecules, together with release of Treg-promoting cytokines and metabolites. (D) Regulation of T cell responses by modulation of the immunological synapse or Treg promoting factors. Tolerogenic adjuvants can suppress T cell activation by inhibiting co-stimulation and/or promoting co-inhibition in the interaction between T cells and antigen presenting cells. Treg differentiation can be enhanced both by APC-T cell interactions and by Treg promoting factors acting on T cells. (E) Suppression of B cell activation by activation of inhibitory receptors. (F) Promoting non-immunogenic uptake of tolerogenic vaccine by mimicking or inducing apoptotic cells. Created in BioRender.
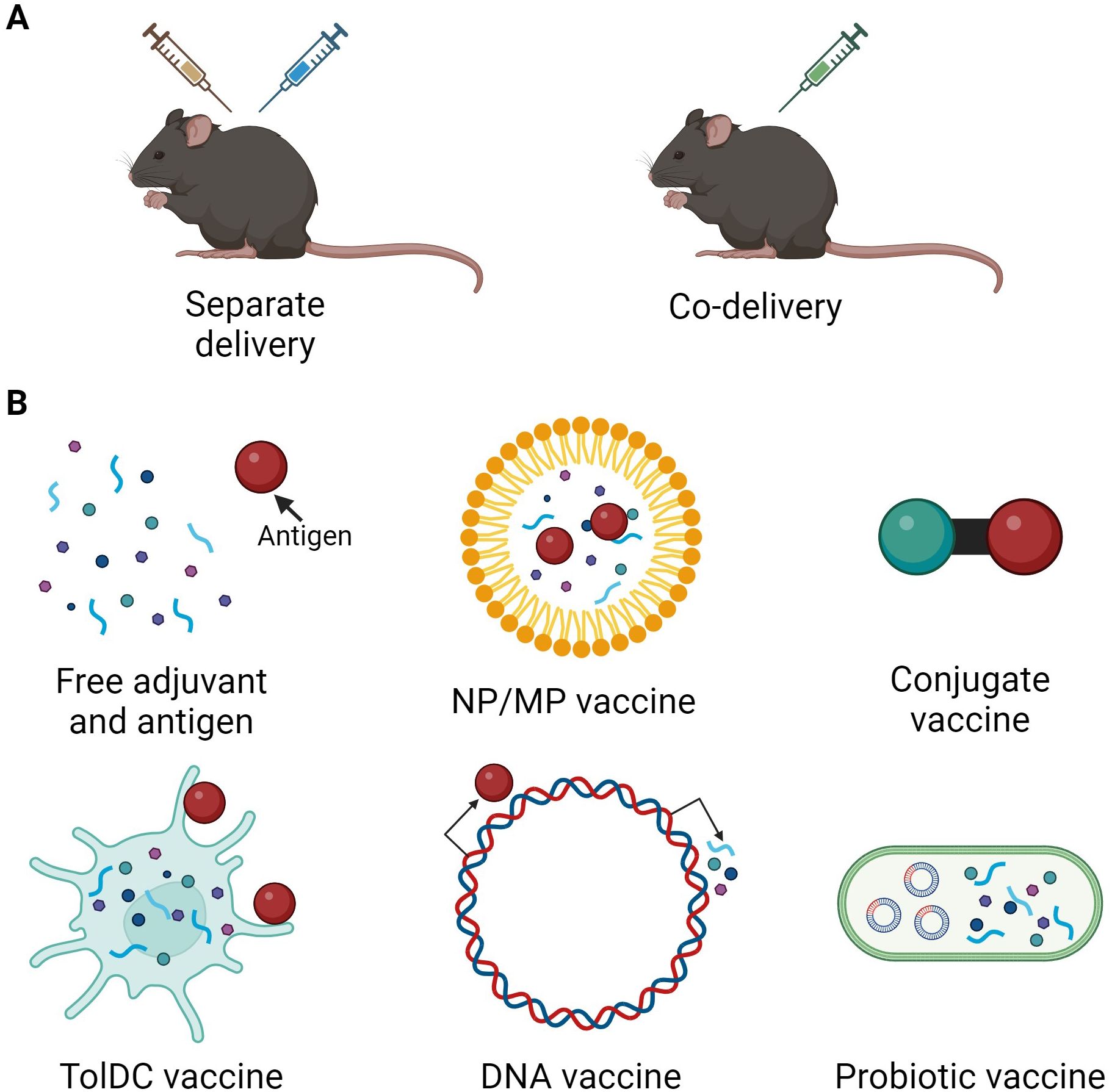
Figure 2. Overview of tolerogenic vaccine design and delivery. (A) Tolerogenic vaccine delivery types. (B) Different types of tolerogenic vaccines include administration of free adjuvant and antigen, incorporation of antigen and/or adjuvant in nanoparticles (NP) or microparticles (MP), conjugates or fusion molecules of antigen and adjuvant, antigen-loading of adjuvant-treated TolDCs, delivery of DNA vectors encoding antigen and/or adjuvant, and probiotic bacteria engineered to express antigen and/or adjuvant. Created in BioRender.
The tolerogenic adjuvants described in this review are grouped in five categories based on their properties and mechanism of action: general immunosuppressive agents (Table 1), cytokines and neuropeptides (Table 2), vitamins and vitamin derivatives (Table 2), modulators of contact-dependent immune cell signaling (Table 3), and other (Table 4). In tolerogenic vaccines using a combination of adjuvants, tolerance can be mediated by multiple mechanisms (Table 5). Tolerogenic vaccines in human clinical trials are discussed for each category and summarized in Table 6.
General immunosuppressive agents include molecules with broad immunosuppressive effects on multiple aspects of the immune system, often by suppressing intracellular signaling pathways to reduce inflammation-induced gene expression (Figure 1B). General immunosuppressive tolerogenic adjuvants are used both to promote tolerogenic features on antigen presenting cells (APCs) and to suppress effector T and B cell function.
Cytokines orchestrate the balance between immune activation and regulation, making them valuable tools as tolerogenic adjuvants to shape antigen-specific immune responses. Classical anti-inflammatory cytokines like transforming growth factor β (TGF-β), and interleukin (IL)-10 exert potent immunosuppressive functions affecting many aspects of the immune system, including the capacity to induce tolDCs and Tregs (Figures 1C, D). In addition to classical anti-inflammatory cytokines, cytokines affecting specific immune cell subsets or skewing immune balance can also be harnessed as tolerogenic adjuvants.
Many vitamins are important for maintaining a healthy immune system. Vitamins A and D signal in a hormone-like manner via nuclear receptors and are potent immunoregulators (10). Some of their immunomodulatory effects are inhibition of T effector cell activation and promotion of tolerogenic APCs (Figures 1C, D).
Modulating the immune synapse, the dynamic interface between APCs and T cells during antigen recognition, offers a promising approach to inducing immune tolerance (Figure 1D). By manipulating the immune synapse, one can modify T cell receptor (TCR) signaling strength to skew T cell responses or induce anergy or apoptosis (11). In addition to TCR signaling, modulation of APC co-stimulatory or co-inhibitory molecules in the immunological synapse can also be utilized to modify the outcome of APC-T cell interactions (12). (Figures 1D, E).
In addition to the adjuvant types described above, there are several other types of adjuvants. Described here are apoptotic remnant mimics (Figure 1F), Toll-like receptor (TLR) agonists and glycans and glycan-binding proteins which may promote tolerogenic immune responses.
Depot adjuvants and delivery systems including cell targeting moieties and nanoparticles/microparticles inherently act as adjuvants since they enhance the immune responses to incorporated antigens (2, 3, 13, 14). Cell targeting strategies which promote antigen delivery to dendritic cells often have tolerogenic properties (3, 14) and can be considered tolerogenic adjuvants. However, in this review we specifically explore adjuvants with tolerogenic properties that actively modify the immune milieu to facilitate shifts in immune cell subsets and/or phenotypes.
2 Tolerogenic adjuvants
2.1 Immunosuppressive agents
2.1.1 Dexamethasone
Glucocorticoids are potent anti-inflammatory and immunosuppressive agents used for treatment of autoimmune and inflammatory conditions (15–17). They mediate their effects through engagement with the nuclear glucocorticoid receptor inducing transcriptional regulation or rapid non-genomic effects (15, 17). In immune cells, the synthetic glucocorticoid dexamethasone suppresses production of most cytokines while increasing production of IL-10, inhibits lymphocyte activation and promotes lymphocyte apoptosis (15). Glucocorticoids dampen overall T cell activation by interfering with TCR signaling, and evidence suggests that glucocorticoids preferentially suppresses Th1 and Th17 T cells. Glucocorticoid treatment is also associated with increased circulating Tregs (15). In APCs, glucocorticoids induce tolerogenic features including attenuated DC maturation and reduced expression of MHC class II and co-stimulatory molecules (15, 18).
Tolerogenic vaccines consisting of antigen delivered with dexamethasone have been successful in murine models of experimental autoimmune encephalitis (EAE) (19–22), atherosclerosis (23), antigen-induced arthritis (AIA) (24) and type 1 diabetes (T1D) (25). Other tolerogenic vaccines use dexamethasone to induce tolDCs, which when loaded with disease relevant antigens and injected reduced disease in models of arthritis (26, 27), dust mite allergy (28) and T1D (29). The disease inhibition was often accompanied by an increase in Tregs over effector T cells and increase in anti-inflammatory cytokines. However, tolDC-mediated disease suppression in one arthritis model was independent of antigen (27) and antigen-loaded dexamethasone-derived tolDCs exacerbated a model of T1D while unloaded tolDCs suppressed disease (30) (Table 1).
Dexamethasone is often used together with other adjuvants to induce tolDCs. TolDC vaccines of dexamethasone in combination with one or more other adjuvants suppressed disease in models of EAE (31, 32) and systemic lupus erythematosus (SLE) (33) (Table 5).
Dexamethasone-containing tolerogenic vaccines have been investigated for human use. A phase I clinical trial investigated transfer of antigen-loaded dexamethasone-treated monocyte-derived DCs for treatment of multiple sclerosis and neuromyelitis optica spectrum disorders, by intravenous injection of tolDC every two weeks for a total of three doses. The tolDC vaccine was well tolerated and there was an increase of regulatory Tr1 cells at 12 weeks of follow-up (34). In another phase I trial, antigen-loaded autologous monocyte-derived DCs treated with a combination of dexamethasone, the vitamin D derivative calcitriol, and the TLR4 agonist MPLA were administered into inflamed knee joints. The treatment was well tolerated but did not result in reduction in disease severity or consistent immunoregulatory features (35) (Table 6).
2.1.2 Rapamycin
Rapamycin is a small molecule inhibitor of mTOR, a kinase that regulates cell growth and metabolism. Immunosuppressive effects of rapamycin includes suppression of the activation and proliferation of conventional T cells, promotion of T cell anergy or deletion, and enhancement of the development and function of Tregs (36). Additionally, rapamycin can inhibit the differentiation and maturation of DCs and promote tolDC features (37). Rapamycin is clinically used as an immunosuppressant for prevention of organ transplant rejection, as well as an anti-cancer drug.
Tolerogenic nanoparticle vaccines with antigen and rapamycin have successfully reduced disease in multiple models of autoimmunity including arthritis (38), Alzheimer’s disease (39), vitiligo (40), allergic airway disease (41, 42), T1D (43), and EAE (44, 45). Rapamycin-containing nanoparticle vaccines also prevented anti-drug antibody responses toward coagulation factor VIII (FVIII) (45, 46), uricase (47), adalimumab (anti-TNFα) (47), and adenoviral vectors used in gene therapy (48–50). Administration of rapamycin without nanoparticle carrier suppressed OVA-induced skin graft rejection (51) and anti-FVIII anti-drug antibodies in mice (52). Observed immunological alterations in studies of tolerogenic vaccines with rapamycin included increased Tregs, decreased levels of inflammatory cytokines, and/or decreased co-stimulatory molecules on DCs (Table 1).
Two phase Ia and phase Ib clinical trials were conducted investigating administration of rapamycin together with pegadricase, a biological drug used for the treatment of gout, finding that rapamycin suppressed the development of anti-drug antibodies in a dose dependent manner (53). Their following phase III trial demonstrated high response rate, safety, and clinically meaningful reduction in serum urate (54). Another phase I clinical trial administered rapamycin-containing nanoparticles together with AAV8 capsid used in adenoviral gene therapies, leading to reduced development of anti-AAV8 antibodies compared to subjects receiving capsid without rapamycin (press release) (Table 6).
2.1.3 Calcineurin inhibitors
Cyclosporine A and FK506 (tacrolimus) are calcineurin inhibitors which suppress downstream nuclear factor of activated T cell (NFAT) signaling and IL-2 production (55). Tolerogenic vaccination with cyclosporine A and autoantigens prevented the development of T1D in pre-diabetic mice and promoted Tregs and tolDCs (56). Likewise, tolerogenic vaccination with FK506 co-delivered with DNA encoding autoantigen suppressed EAE (57), and adoptive transfer of antigen-loaded FK506-induced tolDCs reduced disease in a model of CIA (58), in both studies with a reduction in Th17 responses (Table 1).
2.1.4 NFκB inhibitors
Nuclear factor kappa B (NFκB) is a transcription factor inducing expression of pro-inflammatory genes including cytokines, cell adhesion molecules, and immunoreceptors. Inhibition of NFκB suppresses T cell responses and induces immunoregulatory features in APCs (59).
Tolerogenic vaccination with antigen-loaded tolDCs treated with NFκB inhibitor BAY 11-7082 suppressed disease in AIA (60), and antigen-loaded tolDCs treated with NFκB inhibitor andrographolide reduced anti-drug antibodies in hemophilia A mice (61). A nanoparticle vaccine loaded with antigen and A20, an anti-inflammatory protein inhibiting NF-κB activation (62), suppressed Th2 responses and reduced disease in an asthma model (63) (Table 1).
A phase I clinical trial investigated antigen-loaded autologous Bay11-7082-treated tolDCs in rheumatoid arthritis. The vaccine reduced effector T cells and increased Tregs, together with a reduction in inflammatory cytokines. The treatment did not induce disease flares and lead to decreased rheumatoid arthritis DAS28 score (64) (Table 6).
2.1.5 Kynurenine and AhR agonists
Kynurenine is an immunoregulatory tryptophan metabolite signaling via the aryl hydrocarbon receptor (AhR). AhR signaling can influence T cell differentiation and function of APCs to favor expansion of Tregs (65, 66). Tolerogenic vaccination with nanoparticles loaded with autoantigen and the AhR agonist ITE (2-(1′H-indole-3′-carbonyl)-thiazole-4-carboxylic acid methyl ester), suppressed disease in models of EAE (67, 68) and T1D (69), and tolerogenic vaccination with kynurenine and antigen-expressing phages prevented hyperglycemia in a model of T1D (70) (Table 1).
2.1.6 Other immunosuppressive agents
Janus kinase (JAK) and signal transducer and activator of transcription proteins (STAT) signaling induces immune cell activation and cytokine production (71). Tolerogenic vaccination with autoantigen-loaded tolDCs treated with JAK/STAT inhibitors tofacitinib and BD750 suppressed disease, reduced Th1 and Th17 responses, and increased Tregs in models of EAE (72, 73) (Table 1).
Rosiglitazone is an anti-diabetic drug that activates peroxisome proliferator–activated receptor gamma (PPARγ). In immune cells, rosiglitazone can inhibit inflammatory cytokines and promote tolerogenic APCs (74). TolDC vaccination with antigen-loaded, rosiglitazone-treated tolDCs suppressed disease in CIA (75) (Table 1), and autoantigen-loaded tolDCs treated with rosiglitazone in combination with dexamethasone and cobalt (III) protoporphyrin suppressed a murine model of SLE (33) (Table 5).
Inhibition of the protein kinase glycogen synthase kinase 3 (GSK-3) in immune cells leads to reduced inflammatory cytokine production and increased IL-10 (76). Vaccination with antigen-loaded tolDCs treated with GSK-3β inhibitor K313 suppressed disease in EAE (77) (Table 1).
Prostaglandin I2 (PGI2) is a lipid signaling mediator most known for its vasodilating and anti-thrombotic effects. PGI2 also has anti-inflammatory properties and protective effects in allergy and asthma (78, 79). Tolerogenic vaccination with antigen-loaded tolDCs treated with PGI2 analog iloprost reduced disease in ovalbumin-induced asthma (80). Additionally, direct delivery of iloprost and antigen using a hydrogel suppressed antigen-induced lung inflammation and increased the frequency of antigen-specific Tregs (80) (Table 1).
2.2 Cytokines and chemokines
2.2.1 TGF-β
TGF-β is a strongly immunosuppressive cytokine, because genetic deficiency of TGF-β leads to fatal autoimmunity (81). TGF-β is an immunosuppressive cytokine with multiple effects on the immune system: it promotes Treg development and function, inhibits B and T cell proliferation, suppresses differentiation of Th1 and Th2 cells, and induces tolDCs (82). However, when combined with specific other cytokines, TGF-β may trigger T cells to differentiate into non-regulatory phenotypes such as Th17 effectors in presence of IL-6 and Th9 in presence of IL-4 (82).
A tolerogenic nanoparticle vaccine containing autoantigen and TGF-β reduced disease and immune cell activation in EAE (83). TolDC vaccination with autoantigen-loaded tolDCs cultured in presence of TGF-β or TGF-β receptor agonist suppressed disease in a CIA model (84) and reduced anti-drug antibodies toward FVIII (85), together with increases in Tregs and IL-10 (Table 2).
In combination with other adjuvants, tolerogenic vaccines using both TGF-β and IL-10 suppressed disease in CIA (86) and reduced anti-drug antibodies toward FVIII (87). Tolerogenic vaccination with microparticles containing GM-CSF and TGF-β1 alongside nanoparticles with antigen and vitamin D suppressed EAE and T1D (88–92) and microparticles loaded with TGF-β, retinoic acid, and autoantigens suppressed T1D (93) (Table 5).
A tolerogenic DNA vaccination with autoantigen-encoding plasmids in combination with plasmids for either TGF-β, IL-10, and or IL-2 suppressed disease in EAE (94) and T1D (95) (Table 5). A phase I clinical trial is registered for this tolerogenic DNA vaccine to evaluate vaccine safety in patients with T1D (Table 6).
2.2.2 IL-10
IL-10 is a powerful immunosuppressive and anti-inflammatory cytokine, absence of which causes spontaneous colitis in mice (96). IL-10 suppresses antigen presentation and inflammatory cytokine production by APCs and simultaneously increases their release of anti-inflammatory mediators. In CD4+ T cells, IL-10 inhibits proliferation and cytokine production and promotes the development of regulatory Tr1 cells (97).
Tolerogenic vaccination with antigen-loaded tolDCs engineered to express IL-10 suppressed disease in mouse models of T1D and asthma (98, 99), and antigen-loaded tolDCs cultured in presence of IL-10 reduced disease in EAE (100). Tolerogenic vaccination with nanoparticles containing IL-10 and antigen suppressed disease in EAE (101), and DNA vaccines encoding IL-10 and antigen suppressed disease in EAE and T1D models (102, 103) (Table 2).
A probiotic vaccine of Lactococcus lactis (L. lactis) genetically engineered to secrete IL-10 and pro-insulin administered together with anti-CD3 ameliorated disease and increase Tregs in models of T1D (104–106) (Table 5). The L. lactis probiotic vaccine has been studied in human clinical trials where results from phase Ib and IIa studies demonstrated treatment to be safe, metabolic variables were either stabilized or improved, and antigen-specific CD8+ T cells were reduced (107) (Table 6).
2.2.3 IL-2
IL-2 mediates T cell survival, differentiation, and proliferation. IL-2 is specifically required for Treg homeostasis and suppression of autoimmunity and genetic deletion results in systemic autoimmunity in mice (108). In addition, recent studies showed that low-dose IL-2 treatment induces the expansion of Treg cells and had efficacy in numerous mouse models and some early efficacy in clinical trials of T1D, graft-vs-host disease and SLE. Different types of tolerogenic vaccination with IL-2 treatment in combination with antigen exposure suppressed disease in models of EAE (109), experimental autoimmune uveitis (EAU) (110, 111), T1D (112), delayed-type hypersensitivity (DTH) (112), and reduced development of anti-drug antibodies toward FVIII in hemophilia A (113). Overall, the vaccines led to increased Tregs and anti-inflammatory cytokines (Table 2).
Tolerogenic vaccines using IL-2 in combination with rapamycin expanded Tregs and suppressed disease in models of T1D (114, 115), EAE (116), and primary biliary cholangitis (114). Furthermore IL-2 in combination with Retinoic Acid suppressed EAE and EAU (117). These combination vaccines expanded Tregs or induced antigen-specific Tr1 cells (Table 5).
2.2.4 IFN-β
Interferon beta (IFN-β) is a type I interferon with immunomodulatory properties, used therapeutically for multiple sclerosis (MS). IFN-β reduces T cell activation, promotes Tregs and induces tolDCs (118, 119). Tolerogenic vaccines comprised of autoantigen and IFN-β suppressed murine and rat models of EAE via the induction of neuroantigen-specific, suppressive CD25+ Tregs (120, 121) (Table 2). In addition, mice treated with IFN-β while receiving autoantigen-loaded vitamin D-treated tolDCs, further suppressed disease in a model of EAE (122) (Table 5).
2.2.5 GM-CSF
In addition to being a growth factor and chemokine, GM-CSF possesses anti-inflammatory effects. Administration of GM-CSF leads to a reduction in disease severity in several animal models of autoimmune disease (123), and GM-CSF promotes development and function of both tolDCs and Tregs (123, 124).
Tolerogenic vaccines with antigen-GM-CSF conjugates using neuropeptide autoantigens have been used to treat EAE in mice and rats, accompanied by increased Tregs (125–129). Additionally, GM-CSF is used for differentiation of DCs for most tolDC vaccines. Although most tolDC vaccines use additional adjuvants, also without other adjuvants transfer of antigen-loaded GM-CSF differentiated tolDCs suppressed murine EAU (130) (Table 2). A hydrogel/microparticle vaccine incorporating GM-CSF and TLR9 agonist CpG with autoantigen suppressed disease in a model of T1D (131) (Table 5).
2.2.6 Other cytokines and chemokines
IL-35 is a potent inducer of Tregs and regulatory B cells, and it can inhibit the proliferation and function of effector Th1 and Th17 cells. IL-35 has been shown to be protective against autoimmune disease and IL-35 treatment have been able to suppress disease in multiple models of autoimmunity and chronic inflammation (132). In tolerogenic vaccines, tolDCs engineered to overexpress IL-35 and loaded with disease relevant antigen suppressed EAE and DTH (133, 134) (Table 2).
IL-27 is an immunoregulatory cytokine which can promote tolerance by supporting development of Tregs and Tr1, antagonizing development of Th2 and Th17 cells, and by increasing co-inhibitory receptor expression on APCs (135). Tolerogenic vaccination by adoptive transfer of IL-27-conditioned antigen-loaded DCs led to a significant amelioration of disease and reduction in Th1 and Th17 cells in murine EAE (136) (Table 2).
IL-4 promotes type 2 immunity and suppresses Th1 polarization. Treatment with IL-4 suppressed disease severity in models of EAE and arthritis (137, 138). Tolerogenic DNA vaccines encoding IL-4 and antigen suppressed murine models of CIA, EAE, and T1D (139–142) (Table 2).
Hepatocyte growth factor (HGF) is a cytokine with pleiotropic effects, including the promotion of tolDCs (143). In a model of EAE, systemic HGF ameliorated disease and tolerogenic vaccination with HGF-treated antigen-loaded DCs mediated functional recovery in mice with established EAE and suppressed T cell mediated inflammation (144) (Table 2).
Vasoactive intestinal peptide (VIP) is a peptide functioning as a neurotransmitter in the central and peripheral nervous systems and has multiple effects, including immune modulation. VIP reduces the release of inflammatory cytokines, stimulates production of IL-10 and TGF-β, and decreases the co-stimulatory activity of APCs (145). Tolerogenic vaccination with antigen-loaded VIP-treated tolDCs led to amelioration of CIA and EAE, accompanied by increased levels of Tr1 cells (146) (Table 2).
TNF-related apoptosis-inducing ligand (TRAIL) is a cytokine that induces apoptosis and activation of NFκB. TRAIL has immunoregulatory effects demonstrated by the exacerbated development of autoimmunity in TRAIL-deficient mice (147). Tolerogenic vaccination with DCs engineered to co-express TRAIL and antigen reduced antigen-specific T cell responses and disease symptoms in models of EAE (148, 149) (Table 2).
2.2.7 Cytokine silencing
Just as the addition of anti-inflammatory or immunoregulatory cytokines can promote tolerogenic responses, silencing of inflammatory cytokines can also be effective. Silencing of B cell activating factor (BAFF), an essential cytokine for both T and B cell activation (150), in TolDCs using siRNA suppressed murine CIA and promoted Tregs (151) (Table 2).
2.3 Vitamins and vitamin derivates
2.3.1 Vitamin D
Vitamin D is primarily known for its role in calcium homeostasis and bone health, but it is also immunoregulatory. Having low levels of vitamin D is associated with increased susceptibility to a variety of infectious and autoimmune diseases. Vitamin D suppresses T cell activation, skews T cell differentiation away from Th17 while promoting Tregs and Th2 responses, and promotes tolerogenic DC features, including low surface expression of co-stimulatory molecules and decreased production of inflammatory cytokines (10, 152). Vitamin D signals via a nuclear receptor and exert immunoregulatory effects by regulating gene expression (10).
Many tolerogenic vaccines using vitamin D are tolDC vaccines. Vitamin D-treated tolDCs loaded with antigen or antigen-encoding mRNA reduced disease and promoted immunoregulatory cells and cytokines in murine models of EAE (153–156). The vitamin D-treated tolDCs had reduced expression of MHC class II, co-stimulatory molecules, and pro-inflammatory cytokines, and induced less T cell proliferation compared to DCs that were untreated (153, 154) (Table 2). TolDC vaccines using a combination of vitamin D and dexamethasone suppressed murine models of arthritis (26) and T1D (29) (Table 5).
Tolerogenic nanoparticle vaccines with vitamin D have been examined in T1D (157, 158), where the nanoparticles reduced disease incidence and increased Tregs or tolDCs in vivo. Separate delivery studies of vitamin D and antigen have been studied in EAE (159, 160) and DTH (161), in all studies reducing disease severity and inflammation (Table 2).
Vitamin D-containing tolerogenic vaccines have been investigated in human clinical trials. In a phase IIa trial of latent autoimmune diabetes in adult patients were treated with daily oral Vitamin D and monthly injections of antigen-alum. The trial demonstrated safety and stable β-cell function and metabolic control at 5 months follow-up (162). Two phase I clinical trials have been registered to test tolerogenic vaccination with antigen-loaded vitamin D-treated monocyte-derived DCs in multiple sclerosis (163). Likewise, a phase I clinical trial tested monocyte-derived DC loaded with proinsulin and treated with Vitamin D and dexamethasone in T1D. The study showed the treatment was safe and lead to reduce proinsulin specific CD8+ T cells and increased ICOS+ CCR4+ TIGIT+ Tregs (164). Another phase I trial investigated liposomes containing collagen II and calcitriol, the active form of vitamin D, for the treatment of rheumatoid arthritis. The calcitriol-antigen-liposomes led to reduced pathogenic T cells and expansion of antigen-specific PD1+ T cells (165) (Table 6).
2.3.2 Retinoic acid
Retinoic acid is an immunoregulatory vitamin A metabolite, which like vitamin D signals via a nuclear receptor (10). Retinoic acid promotes the development and function of Tregs while inhibiting the differentiation and activation of effector Th1 and Th17 cells. DCs and macrophages can produce retinoic acid to support Treg induction and maintenance, and the retinoic acid-producing capacity of DCs is further enhanced upon retinoic acid exposure (10, 166).
Tolerogenic vaccination with liposomes incorporating retinoic acid and autoantigen converted pathogenic autoantigen specific Th17 cells to Tr1 cells and suppressed disease in EAE (167). Tolerogenic vaccination with retinoic acid, IL-2, and autoantigen suppressed EAE and EAU and pathogenic Th17 and Th1 responses (117). Prophylactic tolerogenic vaccination with retinoic acid, TGF-β and autoantigen inhibited the incidence of T1D in mice (93), and in another study treatment of mice with autoantigen and retinoic acid in combination with IL-4 suppressed EAE (168) (Tables 2, 6).
2.4 Modulators of contact-dependent immune cell signaling
2.4.1 T cell modulation
CD3 is the invariant chain of the TCR. Anti-CD3 monoclonal antibodies suppresses disease in numerous animal models of autoimmunity, and anti-CD3 is an FDA approved treatment to delay early onset type 1 diabetes. The exact mechanism of anti-CD3-mediated immune suppression is unclear but proposed mechanisms include prevention of T cells from recognizing their antigens and the induction of anergy or apoptosis in activated T cells while sparing Tregs (169). Tolerogenic vaccination with anti-CD3 treatment in combination with an antigen-expressing lentiviral vector suppressed T1D and induced autoantigen-specific Tregs (170). Anti-CD3 is also a component of the previously described probiotic vaccine (104–106) (Tables 3, 5).
CD4 is a glycoprotein on helper T cells which primarily functions as a TCR co-receptor. Non-depleting anti-CD4 therapy has been shown to suppress autoimmunity and graft rejection by modulating the function of CD4+ T cells by blocking T cell activation and promoting Treg differentiation and suppressor functions (171). A tolerogenic vaccine comprised of aluminum hydroxide (alum), FVIII, and non-depleting anti-CD4 prevented development of anti-drug antibodies in mice (172). Another tolerogenic vaccine using treatment with depleting anti-CD4 antibodies followed by antigen administration suppressed disease in a murine model of EAU via the induction of antigen-specific Tregs (173) (Table 3).
“Tregitopes” are peptides derived from IgG that are recognized by a subset of natural Tregs. When presented in MHCII, these peptides activate Tregitope-specific Tregs and suppression of effector T cell responses to co-delivered antigens. Administration of nanoparticles with antigen and tregitopes decreased incidence and severity of T1D in mice (174). Likewise, co-administration of autontigen with Tregitope-albumin fusion proteins decreased incidence and reverse mild T1D (175) (Table 3).
Invariant natural killer T cells (iNKT cells) are immunoregulatory T cells important for preventing autoimmune reactions. The glycolipid α-galactosylceramide (α-GalCer) is a strong inducer of iNKT cells and has been shown to suppress disease in multiple animal models of autoimmunity (176). A tolerogenic vaccine comprised of lipid nanoparticles carrying autoantigen and α-GalCer prevented the development of diabetes in prediabetic mice (177) (Table 3).
2.4.2 Modulation of the immunological synapse
Including MHC class II-targeting molecules in a tolerogenic vaccine ensures delivery to APCs and may disrupt the immunological synapse. A tolerogenic vaccine with antigen conjugated to antibody fragments (nanobodies) targeting MHC class II suppressed disease in EAE. When combined with dexamethasone, the vaccine also overcame the inflammation associated with antigen exposure (178) (Table 5).
Co-stimulatory signals, such as CD80 and CD86, are necessary for T cell activation by APCs. T cell recognition of antigen on MHC II without co-stimulation results in anergy or apoptosis. Abatacept, a cytotoxic T-lymphocyte associated protein 4 (CTLA-4)-Fc fusion protein blocks CD80 and CD86 and is FDA approved for the treatment of autoimmune arthritis (179). Tolerogenic vaccination with nanoparticles displaying abatacept and carrying autoantigen and dexamethasone suppressed EAE (180) (Table 5).
Signaling via CD40-CD40L induces activation and pro-inflammatory cytokine production in both B cells, T cells, and APCs, and CD40L-blockade reduced disease in numerous animal models of autoimmunity (181). Tolerogenic vaccination with FVIII and anti-CD40 prevented subsequent development of anti-FVIII antibodies during rechallenge (182) (Table 3).
Other approaches include genetic modification of co-stimulatory signals. Antigen delivered with a CRISPR-Cas9 plasmid and guide RNAs toward CD80, CD86, and CD40 disrupted co-stimulation by DCs, reduced inflammatory cytokines, increased Tregs, and suppressed disease in a model of T1D (183) (Table 5). Administration of a DNA vector encoding membrane-bound autoantigen together with a B7.1/CD40L mutant fusion protein binding to CTLA-4 but not CD28, providing co-inhibitory but not co-stimulatory signals, reduced disease incidence in murine model of T1D (184) (Table 3).
Intercellular adhesion molecule 1 (ICAM-1) is a cell surface glycoprotein most known for its role in leukocyte migration. Inhibition of ICAM-1 can block T cell activation and induce tolerance by disrupting T cell-APC interactions, inhibiting co-stimulation, promoting PD-L1 expression, and by inducing T cell anergy or exhaustion (185). A tolerogenic vaccine comprised of hyaluronic acid with autoantigen and an ICAM-1 inhibitory peptide suppressed disease in EAE (186–188). In another tolerogenic vaccine, fusion molecules of antigen and ICAM-1 inhibitory peptide prevented the development of T1D (189) and suppressed EAE (190) (Table 3).
OX40 is a TNF receptor superfamily member expressed by activated T cells and resting Tregs, which acts as a co-stimulatory molecule promoting cell proliferation (191). Tolerogenic vaccination of prediabetic mice with antigen and an OX40 agonistic antibody reduced diabetes incidence and increased antigen specific Tregs (192) (Table 3).
2.4.3 Activation of inhibitory receptors
Programmed cell death protein 1 (PD-1) is an immune checkpoint molecule that induces T cell apoptosis and suppresses conventional T cell activation and favors development of Tregs (193). Treatment with tolDCs engineered to express PD-L1 and MOG reduced antigen-specific T cell responses and suppressed MOG induced EAE but not MBP induced EAE (148, 149) (Table 3).
B and T lymphocyte attenuator (BTLA) is an inhibitory receptor structurally related to PD-1, activation of which leads to the suppression of T cell activation (194), and BTLA-expressing DCs promoted Treg development (195). Adoptive transfer of bone marrow derived DCs treated with a nanoparticle containing antigen and a BTLA-encoding plasmid suppressed EAE (196) (Table 3).
Fas is a death receptor inducing apoptosis upon binding to Fas ligand (FasL). A tolerogenic vaccine using FasL-conjugated microparticles containing monocyte chemotactic protein-1 (MCP-1) recruited T cells and then induced their apoptosis. When coupled with respective antigens, the microparticles could suppress EAE and prevented development of T1D in pre-diabetic mice (197). (Table 5) A tolerogenic vaccine aiming for engagement of multiple inhibitory receptors for immune suppression used microparticles displaying surface PD-L1-Fc, anti-Fas, and self-marker CD47, and containing TGF-β. The vaccine led to reduced T cell infiltration and EAE suppression (198, 199) (Table 5).
CD22 and siglec G are inhibitory receptors inhibiting B cell receptor (BCR) signaling, thus suppressing B cell responses (200). Tolerogenic vaccination with liposomes displaying antigen and CD22/siglec G ligands induced antigen-specific tolerance in mice and reduce development of anti-drug antibodies toward FVIII in a model of hemophilia A (201) and reduced antigen-specific antibody production (202) (Table 3). Encapsulation of rapamycin in CD22L autoantigen liposomes suppressed arthritis in mice (203–205) (Table 5).
2.5 Other adjuvants
2.5.1 Modulators of apoptotic pathway signaling
Apoptotic cells are cleared by phagocytic cells via anti-inflammatory mechanisms, which in part is mediated by phosphatidylserine exposed on the apoptotic cell surface (206). Therefore, phosphatidylserine liposomes, O-phospho-L-serine (OPLS), or pro-apoptotic factors have been used as tolerogenic adjuvants to reduce antigen immunogenicity.
In hemophilia A, both co-delivery of FVIII with OPLS and tolerogenic nanoparticle vaccination with FVIII encapsulated in phosphatidylserine liposomes led to a reduction in anti-drug antibodies toward FVIII in mice (207, 208), and antigen-containing phosphatidylserine liposomes reduced disease incidence in a model of T1D (209). In addition, phosphatidylserine liposomes loaded with collagen peptide and the immunomodulator leflunomide, which inhibits the mitochondrial enzyme dihydroorotate dehydrogenase preventing uridine synthesis, suppressed CIA in mice (210). Furthermore, a DNA vaccine encoding antigen and the pro-apoptotic protein BAX, promoting apoptosis in cells expressing antigen, suppressed T1D via modulation of APC function and promotion of Treg development (211, 212) (Table 4).
2.5.2 TLR agonists
TLR agonists are well known inflammatory stimuli and often used as adjuvants in immunogenic vaccines to enhance the immune response to the target antigen (213). However, signaling through microbial pattern recognizing receptors might also protect from development of autoimmunity. Dose and administration of the TLR agonist affects if the response is immunogenic or tolerogenic, it is believed that a short-term, high-dose stimulation will result in immunogenic responses whereas low-dose, repeated stimulation results in tolerance (214).
TLR4 agonist LPS is often used in cultures of DCs to induce maturation and enhance their antigen presenting capacity. Antigen-loaded LPS-treated DCs suppressed EAE while non-treated DCs did not (215). A recombinant fusion protein of autoantigen and flagellin A, TLR5 agonist, induced production of IL-6 and IL-10 in DCs and reduced T cell-driven inflammation in a murine model of intestinal allergy (216, 217). Co-delivery of flagellin B and antigen reduced disease in models of allergy (218, 219) and a fusion protein of antigen and flagellin B reduced disease and IgE responses in allergy (220) (Table 4).
TLR9 agonist CpG DNA has been used in different tolerogenic vaccines in combination with other adjuvants. Treatment with a hydrogel vaccine containing CpG DNA, antigen, and GM-CSF prevented and delayed disease onset in pre-diabetic mice, and the inclusion of CpG DNA enhanced efficacy compared to GM-CSF alone (131). Tolerogenic vaccination with antigen, CpG DNA, and heat shock protein 60 induced an antigen-specific increase in IL-10 production and reduced disease severity in a model of arthritis (221) (Table 5).
2.5.3 Glycans and glycan-binding proteins
The use of glycans in tolerogenic vaccine design can mediate tolerance by targeting antigen to APCs expressing receptors for these glycans, promoting its uptake and processing, while concurrently inducing immunoregulatory effects in DCs (14, 222, 223).
β-glucan is a polysaccharide naturally occurring in cell walls of plants, bacteria, and fungi, and binds to Dectin-1 on myeloid cells. In a model of T1D, treatment with β-glucan and antigen led to increased protection from disease compared to treatment with β-glucan or antigen alone, and promoted tolDC features and increased Tregs (224). Conjugates of allergens to mannan, targeted these antigens to APCs expressing mannose and C-type lectin receptors, and promoted a tolerogenic response in comparison to native allergens in vitro and in vivo (222, 223). Skin-prick tests with mannan-conjugated grass pollen allergoids caused less inflammation than native allergens in patients with grass pollen allergy (225), and immunization of mice with mannan-allergoid conjugates led to tolerogenic responses and increase in Foxp3+ Tregs compared to native antigen (225, 226) (Table 4). Mannan-allergoid conjugates have been tested for dust mite and grass pollen allergens in two phase II clinical trials, showing improvement in nasal provocation test (227, 228) (Table 6).
Galectin-1 is a glycan-binding protein with diverse functions, including modulation of DCs and T cell responses (229). In a study of EAE, treatment of DCs with galectin-1 led to tolDC differentiation that suppressed EAE when loaded with relevant autoantigen (230). The disease suppression was dependent on IL-27 and IL-10 induced by galectin-1 (230) (Table 4).
3 Discussion
Tolerogenic vaccines are promising experimental treatments for a wide range of conditions, including autoimmune disease, anti-drug antibody responses, transplantation rejection, and hypersensitivity (3, 6). Successful reintroduction of immune tolerance via tolerogenic vaccination would have numerous benefits over traditional immunosuppression or immune modulation. First, tolerance could be durable as tolerogenic vaccines may deplete or inactivate pathogenic cells, while concurrently inducing long lived suppressive Tregs and/or regulatory B cells which can self-renew and persist (231, 232). Second, tolerogenic vaccines engaging antigen-specific Treg responses may engender bystander suppression and infectious tolerance to suppress autoimmune responses to unknown antigens (3, 233). Third, tolerogenic vaccines may have efficacy with minimized toxicity as they modulate the antigen-specific response, leaving the rest of the immune system intact. Together these characteristics could constitute a functional cure.
Although tolerogenic adjuvants are not always necessary in tolerogenic vaccines (3, 234, 235) addition of tolerogenic adjuvants have the potential to greatly enhance the efficacy of tolerogenic vaccines by several mechanisms. Immunosuppressive or anti-inflammatory tolerogenic adjuvants promote an anti-inflammatory environment upon antigen encounter, thereby reducing the risk of unwanted inflammatory responses, anaphylaxis or disease exacerbation when re-introducing disease-relevant antigens. Immunomodulatory tolerogenic adjuvants can steer the antigen-specific immune response in desired direction, and cell-targeting adjuvants ensure vaccine delivery to intended cell types and minimizes off-target effects. Additionally, tolDC transfer is a common tolerogenic vaccine modality, but this type of tolerogenic vaccine is associated with high costs and difficulties of standardization across patients. Therefore, tolerogenic vaccines using adjuvants to deliver antigen to and modulate DCs in vivo may represent a more feasible treatment option.
A major limitation to the development of tolerogenic vaccines is a lack of understanding of the autoantigen pools that drive autoimmune diseases. Few autoimmune diseases have limited and well-defined antigen pools, while most autoimmune disease have numerous, poorly defined or undefined antigen pool, and disease antigens may change through time or differ across patients. Therefore, initial clinical trials using tolerogenic vaccines have focused on conditions which have relatively defined antigen pools such as celiac disease, pemphigus vulgaris, T1D and anti-drug antibody responses. However, preclinical data suggest that induction of tissue-specific Tregs may circumvent the need-to-know exact antigens involved in disease as these vaccine-induced tissue-specific Tregs can traffic to the inflamed tissue and exert suppressive functions via bystander suppression or infectious tolerance to suppress immune responses to unknown antigens involved autoimmune disease (3, 233). Utilizing adjuvants to expand or enhance Treg responses, may therefore enable further application of tolerogenic vaccines also in autoimmune diseases with complex autoantigen pools.
Another unknown is if tolerogenic vaccines that induce tolDCs and/or Tregs can suppress preexisting pathogenic B cell that were licensed by CD4 T cell and have limited ongoing interactions with either Tregs or TolDCs. Therefore, tolerogenic vaccines might have to address B cells separately. This could be achieved by B cell targeting or B cell suppression, such as by adjuvants signaling via Siglec G and CD22 or using adjuvants with effects on both B and T cell responses. This approach could be combined with a tolerogenic vaccine design acting on tolDC and/or T cells to prevent further activation of novel pathogenic B cell clones.
In conclusion, tolerogenic vaccines may be the therapeutics of the future for autoimmune and inflammatory conditions. Tolerogenic adjuvants are powerful tools with capacity to both enhance antigen-specific tolerance as well as reduce the risk of unwanted inflammatory responses or off-target effects.
Author contributions
CM: Writing – original draft, Writing – review & editing. SA-B: Writing – original draft, Writing – review & editing, Visualization.
Funding
The author(s) declare financial support was received for the research, authorship, and/or publication of this article. The authors declare that this study received funding from Amgen Inc. The funder was not involved in the study design, collection, analysis, interpretation of data, the writing of this article or the decision to submit it for publication.
Acknowledgments
We acknowledge the use of ChatGPT, developed by OpenAI, for correcting grammatical structure and syntax in this article. All corrections and suggestions provided by the AI tool were reviewed and verified by the authors.
Conflict of interest
Authors SA-B and CM were employed by Amgen Inc.
Publisher’s note
All claims expressed in this article are solely those of the authors and do not necessarily represent those of their affiliated organizations, or those of the publisher, the editors and the reviewers. Any product that may be evaluated in this article, or claim that may be made by its manufacturer, is not guaranteed or endorsed by the publisher.
References
1. Waldmann H. Mechanisms of immunological tolerance. Clin Biochem. (2016) 49:324–8. doi: 10.1016/j.clinbiochem.2015.05.019
2. Liu Q, Chen G, Liu X, Tao L, Fan Y, Xia T. Tolerogenic nano-/microparticle vaccines for immunotherapy. ACS Nano. (2024) 18(7):5219–39. doi: 10.1021/acsnano.3c11647
3. Moorman CD, Sohn SJ, Phee H. Emerging therapeutics for immune tolerance: tolerogenic vaccines, T cell therapy, and IL-2 therapy. Front Immunol. (2021) 12:657768. doi: 10.3389/fimmu.2021.657768
4. Sakaguchi S, Yamaguchi T, Nomura T, Ono M. Regulatory T cells and immune tolerance. Cell. (2008) 133:775–87. doi: 10.1016/j.cell.2008.05.009
5. Audiger C, Rahman MJ, Yun TJ, Tarbell KV, Lesage S. The importance of dendritic cells in maintaining immune tolerance. J Immunol. (2017) 198:2223–31. doi: 10.4049/jimmunol.1601629
6. Kenison JE, Stevens NA, Quintana FJ. Therapeutic induction of antigen-specific immune tolerance. Nat Rev Immunol. (2024) 24:338–57. doi: 10.1038/s41577-023-00970-x
7. Rosser EC, Mauri C. Regulatory B cells: origin, phenotype, and function. Immunity. (2015) 42:607–12. doi: 10.1016/j.immuni.2015.04.005
8. Roncarolo MG, Gregori S, Bacchetta R, Battaglia M, Gagliani N. The biology of T regulatory type 1 cells and their therapeutic application in immune-mediated diseases. Immunity. (2018) 49:1004–19. doi: 10.1016/j.immuni.2018.12.001
9. Zhao T, Cai Y, Jiang Y, He X, Wei Y, Yu Y, et al. Vaccine adjuvants: mechanisms and platforms. Signal Transduct Target Ther. (2023) 8:283. doi: 10.1038/s41392-023-01557-7
10. Mora JR, Iwata M, von Andrian UH. Vitamin effects on the immune system: vitamins A and D take centre stage. Nat Rev Immunol. (2008) 8:685–98. doi: 10.1038/nri2378
11. Gascoigne NR, Rybakin V, Acuto O, Brzostek J. TCR signal strength and T cell development. Annu Rev Cell Dev Biol. (2016) 32:327–48. doi: 10.1146/annurev-cellbio-111315-125324
12. Zhang Q, Vignali DA. Co-stimulatory and co-inhibitory pathways in autoimmunity. Immunity. (2016) 44:1034–51. doi: 10.1016/j.immuni.2016.04.017
13. Puricelli C, Boggio E, Gigliotti CL, Stoppa I, Sutti S, Rolla R, et al. Cutting-edge delivery systems and adjuvants in tolerogenic vaccines: A review. Pharmaceutics. (2022) 14:1782. doi: 10.3390/pharmaceutics14091782
14. Castenmiller C, Keumatio-Doungtsop BC, van Ree R, de Jong EC, van Kooyk Y. Tolerogenic immunotherapy: targeting DC surface receptors to induce antigen-specific tolerance. Front Immunol. (2021) 12:643240. doi: 10.3389/fimmu.2021.643240
15. Cain DW, Cidlowski JA. Immune regulation by glucocorticoids. Nat Rev Immunol. (2017) 17:233–47. doi: 10.1038/nri.2017.1
16. van Staa TP, Leufkens HG, Abenhaim L, Begaud B, Zhang B, Cooper C. Use of oral corticosteroids in the United Kingdom. QJM. (2000) 93:105–11. doi: 10.1093/qjmed/93.2.105
17. Barnes PJ. How corticosteroids control inflammation: Quintiles Prize Lecture 2005. Br J Pharmacol. (2006) 148:245–54. doi: 10.1038/sj.bjp.0706736
18. Adorini L, Giarratana N, Penna G. Pharmacological induction of tolerogenic dendritic cells and regulatory T cells. Semin Immunol. (2004) 16:127–34. doi: 10.1016/j.smim.2003.12.008
19. Pickens CJ, Christopher MA, Leon MA, Pressnall MM, Johnson SN, Thati S, et al. Antigen-drug conjugates as a novel therapeutic class for the treatment of antigen-specific autoimmune disorders. Mol Pharm. (2019) 16:2452–61. doi: 10.1021/acs.molpharmaceut.9b00063
20. Sands RW, Tabansky I, Verbeke CS, Keskin D, Michel S, Stern J, et al. Steroid-peptide immunoconjugates for attenuating T cell responses in an experimental autoimmune encephalomyelitis murine model of multiple sclerosis. Bioconjug Chem. (2020) 31:2779–88. doi: 10.1021/acs.bioconjchem.0c00582
21. Phan NM, Nguyen TL, Shin H, Trinh TA, Kim J. ROS-scavenging lignin-based tolerogenic nanoparticle vaccine for treatment of multiple sclerosis. ACS Nano. (2023) 17:24696–709. doi: 10.1021/acsnano.3c04497
22. Peine KJ, Guerau-de-Arellano M, Lee P, Kanthamneni N, Severin M, Probst GD, et al. Treatment of experimental autoimmune encephalomyelitis by codelivery of disease associated Peptide and dexamethasone in acetalated dextran microparticles. Mol Pharm. (2014) 11:828–35. doi: 10.1021/mp4005172
23. Chen A, Geng Y, Ke H, Constant L, Yan Z, Pan Y, et al. Cutting edge: Dexamethasone potentiates the responses of both regulatory T cells and B-1 cells to antigen immunization in the ApoE(-/-) mouse model of atherosclerosis. J Immunol. (2014) 193:35–9. doi: 10.4049/jimmunol.1302469
24. Benne N, Ter Braake D, Porenta D, Lau CYJ, Mastrobattista E, Broere F. Autoantigen-dexamethasone conjugate-loaded liposomes halt arthritis development in mice. Adv Healthc Mater. (2024) 13(12):e2304238. doi: 10.1002/adhm.202304238
25. Zhang J, Gao W, Yang X, Kang J, Zhang Y, Guo Q, et al. Tolerogenic vaccination reduced effector memory CD4 T cells and induced effector memory Treg cells for type I diabetes treatment. PloS One. (2013) 8:e70056. doi: 10.1371/journal.pone.0070056
26. Stoop JN, Harry RA, von Delwig A, Isaacs JD, Robinson JH, Hilkens CM. Therapeutic effect of tolerogenic dendritic cells in established collagen-induced arthritis is associated with a reduction in Th17 responses. Arthritis Rheumatol. (2010) 62:3656–65. doi: 10.1002/art.v62.12
27. Jansen MAA, Spiering R, Ludwig IS, van Eden W, Hilkens CMU, Broere F. Matured tolerogenic dendritic cells effectively inhibit autoantigen specific CD4(+) T cells in a murine arthritis model. Front Immunol. (2019) 10:2068. doi: 10.3389/fimmu.2019.02068
28. de Aragao-Franca LS, Aragao-Franca LS, Rocha VCJ, Rocha VCJ, Cronemberger-Andrade A, da Costa FHB, et al. Tolerogenic dendritic cells reduce airway inflammation in a model of dust mite triggered allergic inflammation. Allergy Asthma Immunol Res. (2018) 10:406–19. doi: 10.4168/aair.2018.10.4.406
29. Danova K, Grohova A, Strnadova P, Funda DP, Sumnik Z, Lebl J, et al. Tolerogenic dendritic cells from poorly compensated type 1 diabetes patients have decreased ability to induce stable antigen-specific T cell hyporesponsiveness and generation of suppressive regulatory T cells. J Immunol. (2017) 198:729–40. doi: 10.4049/jimmunol.1600676
30. Funda DP, Golias J, Hudcovic T, Kozakova H, Spisek R, Palova-Jelinkova L. Antigen loading (e.g., glutamic acid decarboxylase 65) of tolerogenic DCs (tolDCs) reduces their capacity to prevent diabetes in the non-obese diabetes (NOD)-severe combined immunodeficiency model of adoptive cotransfer of diabetes as well as in NOD mice. Front Immunol. (2018) 9:290. doi: 10.3389/fimmu.2018.00290
31. Lee JH, Park CS, Jang S, Kim JW, Kim SH, Song S, et al. Tolerogenic dendritic cells are efficiently generated using minocycline and dexamethasone. Sci Rep. (2017) 7:15087. doi: 10.1038/s41598-017-15569-1
32. Deak P, Knight HR, Esser-Kahn A. Robust tolerogenic dendritic cells via push/pull pairing of toll-like-receptor agonists and immunomodulators reduces EAE. Biomaterials. (2022) 286:121571. doi: 10.1016/j.biomaterials.2022.121571
33. Funes SC, Rios M, Gomez-Santander F, Fernandez-Fierro A, Altamirano-Lagos MJ, Rivera-Perez D, et al. Tolerogenic dendritic cell transfer ameliorates systemic lupus erythematosus in mice. Immunology. (2019) 158:322–39. doi: 10.1111/imm.v158.4
34. Zubizarreta I, Florez-Grau G, Vila G, Cabezon R, Espana C, Andorra M, et al. Immune tolerance in multiple sclerosis and neuromyelitis optica with peptide-loaded tolerogenic dendritic cells in a phase 1b trial. Proc Natl Acad Sci U S A. (2019) 116:8463–70. doi: 10.1073/pnas.1820039116
35. Bell GM, Anderson AE, Diboll J, Reece R, Eltherington O, Harry RA, et al. Autologous tolerogenic dendritic cells for rheumatoid and inflammatory arthritis. Ann Rheum Dis. (2017) 76:227–34. doi: 10.1136/annrheumdis-2015-208456
36. Powell JD, Delgoffe GM. The mammalian target of rapamycin: linking T cell differentiation, function, and metabolism. Immunity. (2010) 33:301–11. doi: 10.1016/j.immuni.2010.09.002
37. Turnquist H, Raimondi G, Zahorchak AF, Fischer RT, Wang Z, Thomson AW. Rapamycin-conditioned dendritic cells are poor stimulators of allogeneic CD4+ T cells, but enrich for antigen-specific foxp3+ T regulatory cells and promote organ transplant tolerance1. J Immunol. (2007) 178:7018–31. doi: 10.4049/jimmunol.178.11.7018
38. Li C, Chen X, Luo X, Wang H, Zhu Y, Du G, et al. Nanoemulsions target to ectopic lymphoids in inflamed joints to restore immune tolerance in rheumatoid arthritis. Nano Lett. (2021) 21:2551–61. doi: 10.1021/acs.nanolett.0c05110
39. Jung M, Lee S, Park S, Hong J, Kim C, Cho I, et al. A therapeutic nanovaccine that generates anti-amyloid antibodies and amyloid-specific regulatory T cells for alzheimer’s disease. Adv Mater. (2023) 35:e2207719. doi: 10.1002/adma.202207719
40. Zhang X, Liu D, He M, Lin M, Tu C, Zhang B. Polymeric nanoparticles containing rapamycin and autoantigen induce antigen-specific immunological tolerance for preventing vitiligo in mice. Hum Vaccin Immunother. (2021) 17:1923–9. doi: 10.1080/21645515.2021.1872342
41. Liu Q, Wang X, Liu X, Liao YP, Chang CH, Mei KC, et al. Antigen- and epitope-delivering nanoparticles targeting liver induce comparable immunotolerance in allergic airway disease and anaphylaxis as nanoparticle-delivering pharmaceuticals. ACS Nano. (2021) 15:1608–26. doi: 10.1021/acsnano.0c09206
42. Zhao J, He P, Jiang M, He C, Zhao Y, Zhang Z, et al. Transdermally delivered tolerogenic nanoparticles induced effective immune tolerance for asthma treatment. J Control Release. (2024) 366:637–49. doi: 10.1016/j.jconrel.2024.01.018
43. Chen N, Kroger CJ, Tisch RM, Bachelder EM, Ainslie KM. Prevention of type 1 diabetes with acetalated dextran microparticles containing rapamycin and pancreatic peptide P31. Adv Healthc Mater. (2018) 7:e1800341. doi: 10.1002/adhm.201800341
44. LaMothe RA, Kolte PN, Vo T, Ferrari JD, Gelsinger TC, Wong J, et al. Tolerogenic nanoparticles induce antigen-specific regulatory T cells and provide therapeutic efficacy and transferrable tolerance against experimental autoimmune encephalomyelitis. Front Immunol. (2018) 9:281. doi: 10.3389/fimmu.2018.00281
45. Maldonado RA, LaMothe RA, Ferrari JD, Zhang AH, Rossi RJ, Kolte PN, et al. Polymeric synthetic nanoparticles for the induction of antigen-specific immunological tolerance. Proc Natl Acad Sci U S A. (2015) 112:E156–65. doi: 10.1073/pnas.1408686111
46. Zhang AH, Rossi RJ, Yoon J, Wang H, Scott DW. Tolerogenic nanoparticles to induce immunologic tolerance: Prevention and reversal of FVIII inhibitor formation. Cell Immunol. (2016) 301:74–81. doi: 10.1016/j.cellimm.2015.11.004
47. Kishimoto TK, Ferrari JD, LaMothe RA, Kolte PN, Griset AP, O’Neil C, et al. Improving the efficacy and safety of biologic drugs with tolerogenic nanoparticles. Nat Nanotechnol. (2016) 11:890–9. doi: 10.1038/nnano.2016.135
48. Meliani A, Boisgerault F, Hardet R, Marmier S, Collaud F, Ronzitti G, et al. Antigen-selective modulation of AAV immunogenicity with tolerogenic rapamycin nanoparticles enables successful vector re-administration. Nat Commun. (2018) 9:4098. doi: 10.1038/s41467-018-06621-3
49. Ilyinskii PO, Michaud AM, Rizzo GL, Roy CJ, Leung SS, Elkins SL, et al. ImmTOR nanoparticles enhance AAV transgene expression after initial and repeat dosing in a mouse model of methylmalonic acidemia. Mol Ther Methods Clin Dev. (2021) 22:279–92. doi: 10.1016/j.omtm.2021.06.015
50. Ilyinskii PO, Roy C, Michaud A, Rizzo G, Capela T, Leung SS, et al. Readministration of high-dose adeno-associated virus gene therapy vectors enabled by ImmTOR nanoparticles combined with B cell-targeted agents. PNAS Nexus. (2023) 2:pgad394. doi: 10.1093/pnasnexus/pgad394
51. Kang J, Huddleston SJ, Fraser JM, Khoruts A. De novo induction of antigen-specific CD4+CD25+Foxp3+ regulatory T cells in vivo following systemic antigen administration accompanied by blockade of mTOR. J Leukoc Biol. (2008) 83:1230–9. doi: 10.1189/jlb.1207851
52. Moghimi B, Sack BK, Nayak S, Markusic DM, Mah CS, Herzog RW. Induction of tolerance to factor VIII by transient co-administration with rapamycin. J Thromb Haemost. (2011) 9:1524–33. doi: 10.1111/j.1538-7836.2011.04351.x
53. Sands E, Kivitz A, DeHaan W, Leung SS, Johnston L, Kishimoto TK. Tolerogenic nanoparticles mitigate the formation of anti-drug antibodies against pegylated uricase in patients with hyperuricemia. Nat Commun. (2022) 13:272. doi: 10.1038/s41467-021-27945-7
54. Baraf HSB, Khanna PP, Kivitz AJ, Strand V, Choi HK, Terkeltaub R, et al. The COMPARE head-to-head, randomized controlled trial of SEL-212 (pegadricase plus rapamycin-containing nanoparticle, ImmTOR) versus pegloticase for refractory gout. Rheumatol (Oxford). (2024) 63:1058–67. doi: 10.1093/rheumatology/kead333
55. Matsuda S, Shibasaki F, Takehana K, Mori H, Nishida E, Koyasu S. Two distinct action mechanisms of immunophilin-ligand complexes for the blockade of T-cell activation. EMBO Rep. (2000) 1:428–34. doi: 10.1093/embo-reports/kvd090
56. Zhou X, Zhang S, Yu F, Zhao G, Geng S, Yu W, et al. Tolerogenic vaccine composited with islet-derived multipeptides and cyclosporin A induces pTreg and prevents Type 1 diabetes in murine model. Hum Vaccin Immunother. (2020) 16:240–50. doi: 10.1080/21645515.2019.1616504
57. Kang Y, Zhao J, Liu Y, Chen A, Zheng G, Yu Y, et al. FK506 as an adjuvant of tolerogenic DNA vaccination for the prevention of experimental autoimmune encephalomyelitis. J Gene Med. (2009) 11:1064–70. doi: 10.1002/jgm.v11:11
58. Ren Y, Yang Y, Yang J, Xie R, Fan H. Tolerogenic dendritic cells modified by tacrolimus suppress CD4(+) T-cell proliferation and inhibit collagen-induced arthritis in mice. Int Immunopharmacol. (2014) 21:247–54. doi: 10.1016/j.intimp.2014.05.004
59. Liu T, Zhang L, Joo D, Sun SC. NF-kappaB signaling in inflammation. Signal Transduct Target Ther. (2017) 2:17023–. doi: 10.1038/sigtrans.2017.23
60. Martin E, Capini C, Duggan E, Lutzky VP, Stumbles P, Pettit AR, et al. Antigen-specific suppression of established arthritis in mice by dendritic cells deficient in NF-kappaB. Arthritis Rheumatol. (2007) 56:2255–66. doi: 10.1002/art.22655
61. Qadura M, Othman M, Waters B, Chegeni R, Walker K, Labelle A, et al. Reduction of the immune response to factor VIII mediated through tolerogenic factor VIII presentation by immature dendritic cells. J Thromb Haemost. (2008) 6:2095–104. doi: 10.1111/j.1538-7836.2008.03165.x
62. Zhou Q, Wang H, Schwartz DM, Stoffels M, Park YH, Zhang Y, et al. Loss-of-function mutations in TNFAIP3 leading to A20 haploinsufficiency cause an early-onset autoinflammatory disease. Nat Genet. (2016) 48:67–73. doi: 10.1038/ng.3459
63. Luo XQ, Zhong JW, Qiu SY, Zhi M, Yang LQ, Zhou YL, et al. A20-OVA nanoparticles inhibit allergic asthma in a murine model. Inflammation. (2020) 43:953–61. doi: 10.1007/s10753-020-01181-5
64. Benham H, Nel HJ, Law SC, Mehdi AM, Street S, Ramnoruth N, et al. Citrullinated peptide dendritic cell immunotherapy in HLA risk genotype-positive rheumatoid arthritis patients. Sci Trans Med. (2015) 7:290ra87. doi: 10.1126/scitranslmed.aaa9301
65. Barroso A, Mahler JV, Fonseca-Castro PH, Quintana FJ. Therapeutic induction of tolerogenic dendritic cells via aryl hydrocarbon receptor signaling. Curr Opin Immunol. (2021) 70:33–9. doi: 10.1016/j.coi.2021.02.003
66. Gandhi R, Kumar D, Burns EJ, Nadeau M, Dake B, Laroni A, et al. Activation of the aryl hydrocarbon receptor induces human type 1 regulatory T cell-like and Foxp3(+) regulatory T cells. Nat Immunol. (2010) 11:846–53. doi: 10.1038/ni.1915
67. Kenison JE, Jhaveri A, Li Z, Khadse N, Tjon E, Tezza S, et al. Tolerogenic nanoparticles suppress central nervous system inflammation. Proc Natl Acad Sci U S A. (2020) 117:32017–28. doi: 10.1073/pnas.2016451117
68. Yeste A, Nadeau M, Burns EJ, Weiner HL, Quintana FJ. Nanoparticle-mediated codelivery of myelin antigen and a tolerogenic small molecule suppresses experimental autoimmune encephalomyelitis. Proc Natl Acad Sci U S A. (2012) 109:11270–5. doi: 10.1073/pnas.1120611109
69. Yeste A, Takenaka MC, Mascanfroni ID, Nadeau M, Kenison JE, Patel B, et al. Tolerogenic nanoparticles inhibit T cell-mediated autoimmunity through SOCS2. Sci Signal. (2016) 9:ra61. doi: 10.1126/scisignal.aad0612
70. Sun J, Shi J, Li J, Wu M, Li Y, Jia S, et al. The effect of immunosuppressive adjuvant kynurenine on type 1 diabetes vaccine. Front Immunol. (2021) 12:681328. doi: 10.3389/fimmu.2021.681328
71. Hu X, li J, Fu M, Zhao X, Wang W. The JAK/STAT signaling pathway: from bench to clinic. Signal Transduction Targeted Ther. (2021) 6:402. doi: 10.1038/s41392-021-00791-1
72. Zhou Y, Leng X, Luo S, Su Z, Luo X, Guo H, et al. Tolerogenic dendritic cells generated with tofacitinib ameliorate experimental autoimmune encephalomyelitis through modulation of th17/treg balance. J Immunol Res. (2016) 2016:5021537. doi: 10.1155/2016/5021537
73. Zhou Y, Leng X, Li H, Yang S, Yang T, Li L, et al. Tolerogenic dendritic cells induced by BD750 ameliorate proinflammatory T cell responses and experimental autoimmune encephalitis in mice. Mol Med. (2017) 23:204–14. doi: 10.2119/molmed.2016.00110
74. Martin H. Role of PPAR-gamma in inflammation. Prospects for therapeutic intervention by food components. Mutat Res. (2009) 669:1–7. doi: 10.1016/j.mrfmmm.2009.06.009
75. Byun SH, Lee JH, Jung NC, Choi HJ, Song JY, Seo HG, et al. Rosiglitazone-mediated dendritic cells ameliorate collagen-induced arthritis in mice. Biochem Pharmacol. (2016) 115:85–93. doi: 10.1016/j.bcp.2016.05.009
76. Martin M, Rehani K, Jope RS, Michalek SM. Toll-like receptor–mediated cytokine production is differentially regulated by glycogen synthase kinase 3. Nat Immunol. (2005) 6:777–84. doi: 10.1038/ni1221
77. Zhou Y, Leng X, Luo X, Mo C, Zou Q, Liu Y, et al. Regulatory dendritic cells induced by K313 display anti-inflammatory properties and ameliorate experimental autoimmune encephalitis in mice. Front Pharmacol. (2019) 10:1579. doi: 10.3389/fphar.2019.01579
78. Claar D, Hartert TV, Peebles RS Jr. The role of prostaglandins in allergic lung inflammation and asthma. Expert Rev Respir Med. (2015) 9:55–72. doi: 10.1586/17476348.2015.992783
79. Zhou W, Zhang J, Goleniewska K, Dulek DE, Toki S, Newcomb DC, et al. Prostaglandin I2 suppresses proinflammatory chemokine expression, CD4 T cell activation, and STAT6-independent allergic lung inflammation. J Immunol. (2016) 197:1577–86. doi: 10.4049/jimmunol.1501063
80. Wong TH, Gau RJ, Chen YF, Shen HH, Lin CT, Chen SL, et al. Dendritic cells treated with a prostaglandin I(2) analog, iloprost, promote antigen-specific regulatory T cell differentiation in mice. Int Immunopharmacol. (2020) 79:106106. doi: 10.1016/j.intimp.2019.106106
81. Kulkarni AB, Huh CG, Becker D, Geiser A, Lyght M, Flanders KC, et al. Transforming growth factor beta 1 null mutation in mice causes excessive inflammatory response and early death. Proc Natl Acad Sci. (1993) 90:770–4. doi: 10.1073/pnas.90.2.770
82. Travis MA, Sheppard D. TGF-beta activation and function in immunity. Annu Rev Immunol. (2014) 32:51–82. doi: 10.1146/annurev-immunol-032713-120257
83. Casey LM, Pearson RM, Hughes KR, Liu JMH, Rose JA, North MG, et al. Conjugation of transforming growth factor beta to antigen-loaded poly(lactide- co-glycolide) nanoparticles enhances efficiency of antigen-specific tolerance. Bioconjug Chem. (2018) 29:813–23. doi: 10.1021/acs.bioconjchem.7b00624
84. Oh JS, Hwang SU, Noh KE, Lee JH, Choi SY, Nam JH, et al. Synthetic TGF-beta signaling agonist-treated dendritic cells induce tolerogenicity and antirheumatic effects. Curr Issues Mol Biol. (2022) 44:3809–21. doi: 10.3390/cimb44090261
85. Ragni MV, Wu W, Liang X, Hsieh CC, Cortese-Hassett A, Lu L. Factor VIII-pulsed dendritic cells reduce anti-factor VIII antibody formation in the hemophilia A mouse model. Exp Hematol. (2009) 37:744–54. doi: 10.1016/j.exphem.2009.02.011
86. Yang J, Yang Y, Ren Y, Xie R, Zou H, Fan H. A mouse model of adoptive immunotherapeutic targeting of autoimmune arthritis using allo-tolerogenic dendritic cells. PloS One. (2013) 8:e77729. doi: 10.1371/journal.pone.0077729
87. Sule G, Suzuki M, Guse K, Cela R, Rodgers JR, Lee B. Cytokine-conditioned dendritic cells induce humoral tolerance to protein therapy in mice. Hum Gene Ther. (2012) 23:769–80. doi: 10.1089/hum.2011.225
88. Lewis JS, Stewart JM, Marshall GP, Carstens MR, Zhang Y, Dolgova NV, et al. Dual-sized microparticle system for generating suppressive dendritic cells prevents and reverses type 1 diabetes in the nonobese diabetic mouse model. ACS Biomater Sci Eng. (2019) 5:2631–46. doi: 10.1021/acsbiomaterials.9b00332
89. Cho JJ, Stewart JM, Drashansky TT, Brusko MA, Zuniga AN, Lorentsen KJ, et al. An antigen-specific semi-therapeutic treatment with local delivery of tolerogenic factors through a dual-sized microparticle system blocks experimental autoimmune encephalomyelitis. Biomaterials. (2017) 143:79–92. doi: 10.1016/j.biomaterials.2017.07.029
90. Kwiatkowski AJ, Helm EY, Stewart JM, Drashansky TT, Cho JJ, Avram D, et al. Treatment with an antigen-specific dual microparticle system reverses advanced multiple sclerosis in mice. Proc Natl Acad Sci U S A. (2022) 119:e2205417119. doi: 10.1073/pnas.2205417119
91. Lewis JS, Dolgova NV, Zhang Y, Xia CQ, Wasserfall CH, Atkinson MA, et al. A combination dual-sized microparticle system modulates dendritic cells and prevents type 1 diabetes in prediabetic NOD mice. Clin Immunol. (2015) 160:90–102. doi: 10.1016/j.clim.2015.03.023
92. Brusko MA, Stewart JM, Posgai AL, Wasserfall CH, Atkinson MA, Brusko TM, et al. Immunomodulatory dual-sized microparticle system conditions human antigen presenting cells into a tolerogenic phenotype in vitro and inhibits type 1 diabetes-specific autoreactive T cell responses. Front Immunol. (2020) 11:574447. doi: 10.3389/fimmu.2020.574447
93. Phillips BE, Garciafigueroa Y, Engman C, Liu W, Wang Y, Lakomy RJ, et al. Arrest in the progression of type 1 diabetes at the mid-stage of insulitic autoimmunity using an autoantigen-decorated all-trans retinoic acid and transforming growth factor beta-1 single microparticle formulation. Front Immunol. (2021) 12:586220. doi: 10.3389/fimmu.2021.586220
94. Castor T, Yogev N, Blank T, Barwig C, Prinz M, Waisman A, et al. Inhibition of experimental autoimmune encephalomyelitis by tolerance-promoting DNA vaccination focused to dendritic cells. PloS One. (2018) 13:e0191927. doi: 10.1371/journal.pone.0191927
95. Pagni PP, Chaplin J, Wijaranakula M, Wesley JD, Granger J, Cracraft J, et al. Multicomponent plasmid protects mice from spontaneous autoimmune diabetes. Diabetes. (2021) 71(1):157–69. doi: 10.2337/figshare.15142284
96. Kuhn R, Lohler J, Rennick D, Rajewsky K, Muller W. Interleukin-10-deficient mice develop chronic enterocolitis. Cell. (1993) 75:263–74. doi: 10.1016/0092-8674(93)80068-P
97. Sabat R, Grütz G, Warszawska K, Kirsch S, Witte E, Wolk K, et al. Biology of interleukin-10. Cytokine Growth Factor Rev. (2010) 21:331–44. doi: 10.1016/j.cytogfr.2010.09.002
98. Henry E, Desmet CJ, Garze V, Fievez L, Bedoret D, Heirman C, et al. Dendritic cells genetically engineered to express IL-10 induce long-lasting antigen-specific tolerance in experimental asthma. J Immunol. (2008) 181:7230–42. doi: 10.4049/jimmunol.181.10.7230
99. Passeri L, Andolfi G, Bassi V, Russo F, Giacomini G, Laudisa C, et al. Tolerogenic IL-10-engineered dendritic cell-based therapy to restore antigen-specific tolerance in T cell mediated diseases. J Autoimmun. (2023) 138:103051. doi: 10.1016/j.jaut.2023.103051
100. Perona-Wright G, Anderton SM, Howie SE, Gray D. IL-10 permits transient activation of dendritic cells to tolerize T cells and protect from central nervous system autoimmune disease. Int Immunol. (2007) 19:1123–34. doi: 10.1093/intimm/dxm084
101. Cappellano G, Woldetsadik AD, Orilieri E, Shivakumar Y, Rizzi M, Carniato F, et al. Subcutaneous inverse vaccination with PLGA particles loaded with a MOG peptide and IL-10 decreases the severity of experimental autoimmune encephalomyelitis. Vaccine. (2014) 32:5681–9. doi: 10.1016/j.vaccine.2014.08.016
102. Liu X, Zhang S, Li X, Zheng P, Hu F, Zhou Z. Vaccination with a co-expression DNA plasmid containing GAD65 fragment gene and IL-10 gene induces regulatory CD4(+) T cells that prevent experimental autoimmune diabetes. Diabetes Metab Res Rev. (2016) 32:522–33. doi: 10.1002/dmrr.v32.6
103. Schif-Zuck S, Wildbaum G, Karin N. Coadministration of plasmid DNA constructs encoding an encephalitogenic determinant and IL-10 elicits regulatory T cell-mediated protective immunity in the central nervous system. J Immunol. (2006) 177:8241–7. doi: 10.4049/jimmunol.177.11.8241
104. Cook DP, Cunha J, Martens PJ, Sassi G, Mancarella F, Ventriglia G, et al. Intestinal Delivery of Proinsulin and IL-10 via Lactococcus lactis Combined With Low-Dose Anti-CD3 Restores Tolerance Outside the Window of Acute Type 1 Diabetes Diagnosis. Front Immunol. (2020) 11:1103. doi: 10.3389/fimmu.2020.01103
105. Robert S, Gysemans C, Takiishi T, Korf H, Spagnuolo I, Sebastiani G, et al. Oral delivery of glutamic acid decarboxylase (GAD)-65 and IL10 by Lactococcus lactis reverses diabetes in recent-onset NOD mice. Diabetes. (2014) 63:2876–87. doi: 10.2337/db13-1236
106. Takiishi T, Korf H, Van Belle TL, Robert S, Grieco FA, Caluwaerts S, et al. Reversal of autoimmune diabetes by restoration of antigen-specific tolerance using genetically modified Lactococcus lactis in mice. J Clin Invest. (2012) 122:1717–25. doi: 10.1172/JCI60530
107. Mathieu C, Wiedeman A, Cerosaletti K, Long SA, Serti E, Cooney L, et al. A first-in-human, open-label Phase 1b and a randomised, double-blind Phase 2a clinical trial in recent-onset type 1 diabetes with AG019 as monotherapy and in combination with teplizumab. Diabetologia. (2024) 67:27–41. doi: 10.1007/s00125-023-06014-2
108. Abbas AK, Trotta E, Simeonov DR, Marson A, Bluestone JA. Revisiting IL-2: Biology and therapeutic prospects. Sci Immunol. (2018) 3:eaat1482–eaat1482. doi: 10.1126/sciimmunol.aat1482
109. Mannie MD, Clayson BA, Buskirk EJ, DeVine JL, Hernandez JJ, Abbott DJ. IL-2/neuroantigen fusion proteins as antigen-specific tolerogens in experimental autoimmune encephalomyelitis (EAE): correlation of T cell-mediated antigen presentation and tolerance induction. J Immunol. (2007) 178:2835–43. doi: 10.4049/jimmunol.178.5.2835
110. Izquierdo C, Ortiz AZ, Presa M, Malo S, Montoya A, Garabatos N, et al. Treatment of T1D via optimized expansion of antigen-specific Tregs induced by IL-2/anti-IL-2 monoclonal antibody complexes and peptide/MHC tetramers. Sci Rep. (2018) 8:8106. doi: 10.1038/s41598-018-26161-6
111. Rizzo LV, Miller-Rivero NE, Chan CC, Wiggert B, Nussenblatt RB, Caspi RR. Interleukin-2 treatment potentiates induction of oral tolerance in a murine model of autoimmunity. J Clin Invest. (1994) 94:1668–72. doi: 10.1172/JCI117511
112. Hamano R, Baba T, Sasaki S, Tomaru U, Ishizu A, Kawano M, et al. Ag and IL-2 immune complexes efficiently expand Ag-specific Treg cells that migrate in response to chemokines and reduce localized immune responses. Eur J Immunol. (2014) 44:1005–15. doi: 10.1002/eji.201343434
113. Liu CL, Ye P, Lin J, Djukovic D, Miao CH. Long-term tolerance to factor VIII is achieved by administration of interleukin-2/interleukin-2 monoclonal antibody complexes and low dosages of factor VIII. J Thromb Haemost. (2014) 12:921–31. doi: 10.1111/jth.12576
114. Kishimoto TK, Fournier M, Michaud A, Rizzo G, Roy C, Capela T, et al. Rapamycin nanoparticles increase the therapeutic window of engineered interleukin-2 and drive expansion of antigen-specific regulatory T cells for protection against autoimmune disease. J Autoimmun. (2023) 140:103125. doi: 10.1016/j.jaut.2023.103125
115. Manirarora JN, Wei CH. Combination therapy using IL-2/IL-2 monoclonal antibody complexes, rapamycin, and islet autoantigen peptides increases regulatory T cell frequency and protects against spontaneous and induced type 1 diabetes in nonobese diabetic mice. J Immunol. (2015) 195:5203–14. doi: 10.4049/jimmunol.1402540
116. Rhodes KR, Tzeng SY, Iglesias M, Lee D, Storm K, Neshat SY, et al. Bioengineered particles expand myelin-specific regulatory T cells and reverse autoreactivity in a mouse model of multiple sclerosis. Sci Adv. (2023) 9:eadd8693. doi: 10.1126/sciadv.add8693
117. Raverdeau M, Christofi M, Malara A, Wilk MM, Misiak A, Kuffova L, et al. Retinoic acid-induced autoantigen-specific type 1 regulatory T cells suppress autoimmunity. EMBO Rep. (2019) 20. doi: 10.15252/embr.201847121
118. Pennell LM, Fish EN. Interferon-beta regulates dendritic cell activation and migration in experimental autoimmune encephalomyelitis. Immunology. (2017) 152:439–50. doi: 10.1111/imm.2017.152.issue-3
119. Jakimovski D, Kolb C, Ramanathan M, Zivadinov R, Weinstock-Guttman B. Interferon β for multiple sclerosis. Cold Spring Harbor Perspect Med. (2018) 8. doi: 10.1101/cshperspect.a032003
120. Mannie MD, Abbott DJ, Blanchfield JL. Experimental autoimmune encephalomyelitis in Lewis rats: IFN-beta acts as a tolerogenic adjuvant for induction of neuroantigen-dependent tolerance. J Immunol. (2009) 182:5331–41. doi: 10.4049/jimmunol.0803756
121. Wang D, Ghosh D, Islam SM, Moorman CD, Thomason AE, Wilkinson DS, et al. IFN-beta facilitates neuroantigen-dependent induction of CD25+ FOXP3+ Regulatory T cells that suppress experimental autoimmune encephalomyelitis. J Immunol. (2016) 197:2992–3007. doi: 10.4049/jimmunol.1500411
122. Quirant-Sanchez B, Mansilla MJ, Navarro-Barriuso J, Presas-Rodriguez S, Teniente-Serra A, Fondelli F, et al. Combined therapy of vitamin D3-tolerogenic dendritic cells and interferon-beta in a preclinical model of multiple sclerosis. Biomedicines. (2021) 9:1758. doi: 10.3390/biomedicines9121758
123. Bhattacharya P, Thiruppathi M, Elshabrawy HA, Alharshawi K, Kumar P, Prabhakar BS. GM-CSF: An immune modulatory cytokine that can suppress autoimmunity. Cytokine. (2015) 75:261–71. doi: 10.1016/j.cyto.2015.05.030
124. Kared H, Leforban B, Montandon R, Renand A, Layseca Espinosa E, Chatenoud L, et al. Role of GM-CSF in tolerance induction by mobilized hematopoietic progenitors. Blood. (2008) 112:2575–8. doi: 10.1182/blood-2008-02-140681
125. Blanchfield JL, Mannie MD. A GMCSF-neuroantigen fusion protein is a potent tolerogen in experimental autoimmune encephalomyelitis (EAE) that is associated with efficient targeting of neuroantigen to APC. J Leukoc Biol. (2010) 87:509–21. doi: 10.1189/jlb.0709520
126. Islam SM, Curtis AD 2nd, Taslim N, Wilkinson DS, Mannie MD. GM-CSF-neuroantigen fusion proteins reverse experimental autoimmune encephalomyelitis and mediate tolerogenic activity in adjuvant-primed environments: association with inflammation-dependent, inhibitory antigen presentation. J Immunol. (2014) 193:2317–29. doi: 10.4049/jimmunol.1303223
127. Moorman CD, Bastian AG, DeOca KB, Mannie MD. A GM-CSF-neuroantigen tolerogenic vaccine elicits inefficient antigen recognition events below the CD40L triggering threshold to expand CD4(+) CD25(+) FOXP3(+) Tregs that inhibit experimental autoimmune encephalomyelitis (EAE). J Neuroinflamm. (2020) 17:180. doi: 10.1186/s12974-020-01856-8
128. Moorman CD, Curtis AD 2nd, Bastian AG, Elliott SE, Mannie MD. A GMCSF-neuroantigen tolerogenic vaccine elicits systemic lymphocytosis of CD4(+) CD25(high) FOXP3(+) regulatory T cells in myelin-specific TCR transgenic mice contingent upon low-efficiency T cell antigen receptor recognition. Front Immunol. (2018) 9:3119. doi: 10.3389/fimmu.2018.03119
129. Abbott DJ, Blanchfield JL, Martinson DA, Russell SC, Taslim N, Curtis AD, et al. Neuroantigen-specific, tolerogenic vaccines: GM-CSF is a fusion partner that facilitates tolerance rather than immunity to dominant self-epitopes of myelin in murine models of experimental autoimmune encephalomyelitis (EAE). BMC Immunol. (2011) 12:72. doi: 10.1186/1471-2172-12-72
130. Jiang HR, Muckersie E, Robertson M, Forrester JV. Antigen-specific inhibition of experimental autoimmune uveoretinitis by bone marrow-derived immature dendritic cells. Invest Ophthalmol Vis Sci. (2003) 44:1598–607. doi: 10.1167/iovs.02-0427
131. Yoon YM, Lewis JS, Carstens MR, Campbell-Thompson M, Wasserfall CH, Atkinson MA, et al. A combination hydrogel microparticle-based vaccine prevents type 1 diabetes in non-obese diabetic mice. Sci Rep. (2015) 5:13155. doi: 10.1038/srep13155
132. Choi J, Leung PS, Bowlus C, Gershwin ME. IL-35 and autoimmunity: a comprehensive perspective. Clin Rev Allergy Immunol. (2015) 49:327–32. doi: 10.1007/s12016-015-8468-9
133. Haller S, Duval A, Migliorini R, Stevanin M, Mack V, Acha-Orbea H. Interleukin-35-producing CD8alpha(+) dendritic cells acquire a tolerogenic state and regulate T cell function. Front Immunol. (2017) 8:98. doi: 10.3389/fimmu.2017.00098
134. Panfili E, Mondanelli G, Orabona C, Bianchi R, Gargaro M, Fallarino F, et al. IL-35Ig-expressing dendritic cells induce tolerance via Arginase 1. J Cell Mol Med. (2019) 23:3757–61. doi: 10.1111/jcmm.2019.23.issue-5
135. Yoshida H, Hunter CA. The immunobiology of interleukin-27. Annu Rev Immunol. (2015) 33:417–43. doi: 10.1146/annurev-immunol-032414-112134
136. Mascanfroni ID, Yeste A, Vieira SM, Burns EJ, Patel B, Sloma I, et al. IL-27 acts on DCs to suppress the T cell response and autoimmunity by inducing expression of the immunoregulatory molecule CD39. Nat Immunol. (2013) 14:1054–63. doi: 10.1038/ni.2695
137. Ishihara A, Ishihara J, Watkins EA, Tremain AC, Nguyen M, Solanki A, et al. Prolonged residence of an albumin-IL-4 fusion protein in secondary lymphoid organs ameliorates experimental autoimmune encephalomyelitis. Nat BioMed Eng. (2021) 5:387–98. doi: 10.1038/s41551-020-00627-3
138. von Kaeppler EP, Wang Q, Raghu H, Bloom MS, Wong H, Robinson WH. Interleukin 4 promotes anti-inflammatory macrophages that clear cartilage debris and inhibits osteoclast development to protect against osteoarthritis. Clin Immunol. (2021) 229:108784. doi: 10.1016/j.clim.2021.108784
139. Garren H, Ruiz PJ, Watkins TA, Fontoura P, Nguyen LT, Estline ER, et al. Combination of gene delivery and DNA vaccination to protect from and reverse Th1 autoimmune disease via deviation to the Th2 pathway. Immunity. (2001) 15:15–22. doi: 10.1016/S1074-7613(01)00171-6
140. Ho PP, Higgins JP, Kidd BA, Tomooka B, Digennaro C, Lee LY, et al. Tolerizing DNA vaccines for autoimmune arthritis. Autoimmunity. (2006) 39:675–82. doi: 10.1080/08916930601061603
141. Ma S, Huang Y, Yin Z, Menassa R, Brandle JE, Jevnikar AM. Induction of oral tolerance to prevent diabetes with transgenic plants requires glutamic acid decarboxylase (GAD) and IL-4. Proc Natl Acad Sci U S A. (2004) 101:5680–5. doi: 10.1073/pnas.0307420101
142. Tisch R, Wang B, Weaver DJ, Liu B, Bui T, Arthos J, et al. Antigen-specific mediated suppression of beta cell autoimmunity by plasmid DNA vaccination. J Immunol. (2001) 166:2122–32. doi: 10.4049/jimmunol.166.3.2122
143. Molnarfi N, Benkhoucha M, Funakoshi H, Nakamura T, Lalive PH. Hepatocyte growth factor: A regulator of inflammation and autoimmunity. Autoimmun Rev. (2015) 14:293–303. doi: 10.1016/j.autrev.2014.11.013
144. Benkhoucha M, Molnarfi N, Dunand-Sauthier I, Merkler D, Schneiter G, Bruscoli S, et al. Hepatocyte growth factor limits autoimmune neuroinflammation via glucocorticoid-induced leucine zipper expression in dendritic cells. J Immunol. (2014) 193:2743–52. doi: 10.4049/jimmunol.1302338
145. Pozo D, Gonzalez-Rey E, Chorny A, Anderson P, Varela N, Delgado M. Tuning immune tolerance with vasoactive intestinal peptide: a new therapeutic approach for immune disorders. Peptides. (2007) 28:1833–46. doi: 10.1016/j.peptides.2007.04.008
146. Chorny A, Gonzalez-Rey E, Fernandez-Martin A, Pozo D, Ganea D, Delgado M. Vasoactive intestinal peptide induces regulatory dendritic cells with therapeutic effects on autoimmune disorders. Proc Natl Acad Sci U S A. (2005) 102:13562–7. doi: 10.1073/pnas.0504484102
147. Lamhamedi-Cherradi SE, Zheng SJ, Maguschak KA, Peschon J, Chen YH. Defective thymocyte apoptosis and accelerated autoimmune diseases in TRAIL-/- mice. Nat Immunol. (2003) 4:255–60. doi: 10.1038/ni894
148. Hirata S, Matsuyoshi H, Fukuma D, Kurisaki A, Uemura Y, Nishimura Y, et al. Involvement of regulatory T cells in the experimental autoimmune encephalomyelitis-preventive effect of dendritic cells expressing myelin oligodendrocyte glycoprotein plus TRAIL. J Immunol. (2007) 178:918–25. doi: 10.4049/jimmunol.178.2.918
149. Hirata S, Senju S, Matsuyoshi H, Fukuma D, Uemura Y, Nishimura Y. Prevention of experimental autoimmune encephalomyelitis by transfer of embryonic stem cell-derived dendritic cells expressing myelin oligodendrocyte glycoprotein peptide along with TRAIL or programmed death-1 ligand. J Immunol. (2005) 174:1888–97. doi: 10.4049/jimmunol.174.4.1888
150. Vincent FB, Saulep-Easton D, Figgett WA, Fairfax KA, Mackay F. The BAFF/APRIL system: emerging functions beyond B cell biology and autoimmunity. Cytokine Growth Factor Rev. (2013) 24:203–15. doi: 10.1016/j.cytogfr.2013.04.003
151. Zhao Y, Sun X, Yang X, Zhang B, Li S, Han P, et al. Tolerogenic dendritic cells generated by BAFF silencing ameliorate collagen-induced arthritis by modulating the th17/regulatory T cell balance. J Immunol. (2020) 204:518–30. doi: 10.4049/jimmunol.1900552
152. Aranow C. Vitamin D and the immune system. J Invest Med. (2011) 59:881–6. doi: 10.2310/JIM.0b013e31821b8755
153. Mansilla MJ, Contreras-Cardone R, Navarro-Barriuso J, Cools N, Berneman Z, Ramo-Tello C, et al. Cryopreserved vitamin D3-tolerogenic dendritic cells pulsed with autoantigens as a potential therapy for multiple sclerosis patients. J Neuroinflamm. (2016) 13:113. doi: 10.1186/s12974-016-0584-9
154. Mansilla MJ, Selles-Moreno C, Fabregas-Puig S, Amoedo J, Navarro-Barriuso J, Teniente-Serra A, et al. Beneficial effect of tolerogenic dendritic cells pulsed with MOG autoantigen in experimental autoimmune encephalomyelitis. CNS Neurosci Ther. (2015) 21:222–30. doi: 10.1111/cns.2015.21.issue-3
155. Xie Z, Chen J, Zheng C, Wu J, Cheng Y, Zhu S, et al. 1,25-dihydroxyvitamin D(3) -induced dendritic cells suppress experimental autoimmune encephalomyelitis by increasing proportions of the regulatory lymphocytes and reducing T helper type 1 and type 17 cells. Immunology. (2017) 152:414–24. doi: 10.1111/imm.2017.152.issue-3
156. Derdelinckx J, Mansilla MJ, De Laere M, Lee WP, Navarro-Barriuso J, Wens I, et al. Clinical and immunological control of experimental autoimmune encephalomyelitis by tolerogenic dendritic cells loaded with MOG-encoding mRNA. J Neuroinflamm. (2019) 16:167. doi: 10.1186/s12974-019-1541-1
157. Bergot AS, Buckle I, Cikaluru S, Naranjo JL, Wright CM, Zheng G, et al. Regulatory T cells induced by single-peptide liposome immunotherapy suppress islet-specific T cell responses to multiple antigens and protect from autoimmune diabetes. J Immunol. (2020) 204:1787–97. doi: 10.4049/jimmunol.1901128
158. Buckle I, Loaiza Naranjo JD, Bergot AS, Zhang V, Talekar M, Steptoe RJ, et al. Tolerance induction by liposomes targeting a single CD8 epitope IGRP(206-214) in a model of type 1 diabetes is impeded by co-targeting a CD4(+) islet epitope. Immunol Cell Biol. (2022) 100:33–48. doi: 10.1111/imcb.v100.1
159. Chiuso-Minicucci F, Ishikawa LL, Mimura LA, Fraga-Silva TF, Franca TG, Zorzella-Pezavento SF, et al. Treatment with vitamin D/MOG association suppresses experimental autoimmune encephalomyelitis. PloS One. (2015) 10:e0125836. doi: 10.1371/journal.pone.0125836
160. Mimura LA, Chiuso-Minicucci F, Fraga-Silva TF, Zorzella-Pezavento SF, Franca TG, Ishikawa LL, et al. Association of myelin peptide with vitamin D prevents autoimmune encephalomyelitis development. Neuroscience. (2016) 317:130–40. doi: 10.1016/j.neuroscience.2015.12.053
161. Ghoreishi M, Bach P, Obst J, Komba M, Fleet JC, Dutz JP. Expansion of antigen-specific regulatory T cells with the topical vitamin d analog calcipotriol. J Immunol. (2009) 182:6071–8. doi: 10.4049/jimmunol.0804064
162. Bjorklund A, Hals IK, Grill V, Ludvigsson J. Latent autoimmune diabetes in adults: background, safety and feasibility of an ongoing pilot study with intra-lymphatic injections of GAD-alum and oral vitamin D. Front Endocrinol (Lausanne). (2022) 13:926021. doi: 10.3389/fendo.2022.926021
163. Willekens B, Presas-Rodriguez S, Mansilla MJ, Derdelinckx J, Lee WP, Nijs G, et al. Tolerogenic dendritic cell-based treatment for multiple sclerosis (MS): a harmonised study protocol for two phase I clinical trials comparing intradermal and intranodal cell administration. BMJ Open. (2019) 9:e030309. doi: 10.1136/bmjopen-2019-030309
164. Nikolic T, Suwandi JS, Wesselius J, Laban S, Joosten AM, Sonneveld P, et al. Tolerogenic dendritic cells pulsed with islet antigen induce long-term reduction in T-cell autoreactivity in type 1 diabetes patients. Front Immunol. (2022) 13:1054968. doi: 10.3389/fimmu.2022.1054968
165. Sonigra A, Nel HJ, Wehr P, Ramnoruth N, Patel S, van Schie KA, et al. Randomized phase I trial of antigen-specific tolerizing immunotherapy with peptide/calcitriol liposomes in ACPA+ rheumatoid arthritis. JCI Insight. (2022) 7. doi: 10.1172/jci.insight.160964
166. Erkelens MN, Mebius RE. Retinoic acid and immune homeostasis: A balancing act. Trends Immunol. (2017) 38:168–80. doi: 10.1016/j.it.2016.12.006
167. Worzner K, Zimmermann J, Buhl R, Desoi A, Christensen D, Dietrich J, et al. Repeated immunization with ATRA-containing liposomal adjuvant transdifferentiates Th17 cells to a Tr1-like phenotype. J Autoimmun. (2024) 144:103174. doi: 10.1016/j.jaut.2024.103174
168. Zhu B, Buttrick T, Bassil R, Zhu C, Olah M, Wu C, et al. IL-4 and retinoic acid synergistically induce regulatory dendritic cells expressing Aldh1a2. J Immunol. (2013) 191:3139–51. doi: 10.4049/jimmunol.1300329
169. Kuhn C, Weiner HL. Therapeutic anti-CD3 monoclonal antibodies: from bench to bedside. Immunotherapy. (2016) 8:889–906. doi: 10.2217/imt-2016-0049
170. Russo F, Citro A, Squeri G, Sanvito F, Monti P, Gregori S, et al. InsB9-23 gene transfer to hepatocyte-based combined therapy abrogates recurrence of type 1 diabetes after islet transplantation. Diabetes. (2021) 70:171–81. doi: 10.2337/db19-1249
171. Becker C, Bopp T, Jonuleit H. Boosting regulatory T cell function by CD4 stimulation enters the clinic. Front Immunol. (2012) 3:164. doi: 10.3389/fimmu.2012.00164
172. Oliveira VG, Agua-Doce A, Curotto de Lafaille MA, Lafaille JJ, Graca L. Adjuvant facilitates tolerance induction to factor VIII in hemophilic mice through a Foxp3-independent mechanism that relies on IL-10. Blood. (2013) 121:3936–45, S1. doi: 10.1182/blood-2012-09-457135
173. Chen Z, Zhang T, Kam HT, Qiao D, Jin W, Zhong Y, et al. Induction of antigen-specific Treg cells in treating autoimmune uveitis via bystander suppressive pathways without compromising anti-tumor immunity. EBioMedicine. (2021) 70:103496. doi: 10.1016/j.ebiom.2021.103496
174. Cousens LP, Su Y, McClaine E, Li X, Terry F, Smith R, et al. Application of IgG-derived natural Treg epitopes (IgG Tregitopes) to antigen-specific tolerance induction in a murine model of type 1 diabetes. J Diabetes Res. (2013) 2013:621693. doi: 10.1155/2013/621693
175. De Groot AS, Skowron G, White JR, Boyle C, Richard G, Serreze D, et al. Therapeutic administration of Tregitope-Human Albumin Fusion with Insulin Peptides to promote Antigen-Specific Adaptive Tolerance Induction. Sci Rep. (2019) 9:16103. doi: 10.1038/s41598-019-52331-1
176. Van Kaer L. alpha-Galactosylceramide therapy for autoimmune diseases: prospects and obstacles. Nat Rev Immunol. (2005) 5:31–42. doi: 10.1038/nri1531
177. Akimoto H, Fukuda-Kawaguchi E, Duramad O, Ishii Y, Tanabe K. A novel liposome formulation carrying both an insulin peptide and a ligand for invariant natural killer T cells induces accumulation of regulatory T cells to islets in nonobese diabetic mice. J Diabetes Res. (2019) 2019:9430473. doi: 10.1155/2019/9430473
178. Pishesha N, Harmand T, Smeding LY, Ma W, Ludwig LS, Janssen R, et al. Induction of antigen-specific tolerance by nanobody-antigen adducts that target class-II major histocompatibility complexes. Nat BioMed Eng. (2021) 5:1389–401. doi: 10.1038/s41551-021-00738-5
179. Moreland L, Bate G, Kirkpatrick P. Abatacept. Nat Rev Drug Discovery. (2006) 5:185–6. doi: 10.1038/nrd1989
180. Park J, Le QV, Wu Y, Lee J, Oh YK. Tolerogenic nanovaccine for prevention and treatment of autoimmune encephalomyelitis. Adv Mater. (2023) 35:e2202670. doi: 10.1002/adma.202202670
181. Karnell JL, Rieder SA, Ettinger R, Kolbeck R. Targeting the CD40-CD40L pathway in autoimmune diseases: Humoral immunity and beyond. Adv Drug Delivery Rev. (2019) 141:92–103. doi: 10.1016/j.addr.2018.12.005
182. Rossi G, Sarkar J, Scandella D. Long-term induction of immune tolerance after blockade of CD40-CD40L interaction in a mouse model of hemophilia A. Blood. (2001) 97:2750–7. doi: 10.1182/blood.V97.9.2750
183. Luo YL, Liang LF, Gan YJ, Liu J, Zhang Y, Fan YN, et al. An all-in-one nanomedicine consisting of CRISPR-cas9 and an autoantigen peptide for restoring specific immune tolerance. ACS Appl Mater Interfaces. (2020) 12:48259–71. doi: 10.1021/acsami.0c10885
184. Chang Y, Yap S, Ge X, Piganelli J, Bertera S, Giannokakis N, et al. DNA vaccination with an insulin construct and a chimeric protein binding to both CTLA4 and CD40 ameliorates type 1 diabetes in NOD mice. Gene Ther. (2005) 12:1679–85. doi: 10.1038/sj.gt.3302578
185. Yusuf-Makagiansar H, Anderson ME, Yakovleva TV, Murray JS, Siahaan TJ. Inhibition of LFA-1/ICAM-1 and VLA-4/VCAM-1 as a therapeutic approach to inflammation and autoimmune diseases. Med Res Rev. (2002) 22:146–67. doi: 10.1002/med.10001
186. Sestak J, Mullins M, Northrup L, Thati S, Forrest ML, Siahaan TJ, et al. Single-step grafting of aminooxy-peptides to hyaluronan: a simple approach to multifunctional therapeutics for experimental autoimmune encephalomyelitis. J Control Release. (2013) 168:334–40. doi: 10.1016/j.jconrel.2013.03.015
187. Sestak JO, Fakhari A, Badawi AH, Siahaan TJ, Berkland C. Structure, size, and solubility of antigen arrays determines efficacy in experimental autoimmune encephalomyelitis. AAPS J. (2014) 16:1185–93. doi: 10.1208/s12248-014-9654-z
188. Sestak JO, Sullivan BP, Thati S, Northrup L, Hartwell B, Antunez L, et al. Codelivery of antigen and an immune cell adhesion inhibitor is necessary for efficacy of soluble antigen arrays in experimental autoimmune encephalomyelitis. Mol Ther Methods Clin Dev. (2014) 1:14008. doi: 10.1038/mtm.2014.8
189. Murray JS, Oney S, Page JE, Kratochvil-Stava A, Hu Y, Makagiansar IT, et al. Suppression of type 1 diabetes in NOD mice by bifunctional peptide inhibitor: modulation of the immunological synapse formation. Chem Biol Drug Des. (2007) 70:227–36. doi: 10.1111/j.1747-0285.2007.00552.x
190. Badawi AH, Siahaan TJ. Suppression of MOG- and PLP-induced experimental autoimmune encephalomyelitis using a novel multivalent bifunctional peptide inhibitor. J Neuroimmunol. (2013) 263:20–7. doi: 10.1016/j.jneuroim.2013.07.009
191. Webb GJ, Hirschfield GM, Lane PJ. OX40, OX40L and autoimmunity: a comprehensive review. Clin Rev Allergy Immunol. (2016) 50:312–32. doi: 10.1007/s12016-015-8498-3
192. Bresson D, Fousteri G, Manenkova Y, Croft M, von Herrath M. Antigen-specific prevention of type 1 diabetes in NOD mice is ameliorated by OX40 agonist treatment. J Autoimmun. (2011) 37:342–51. doi: 10.1016/j.jaut.2011.10.001
193. Patsoukis N, Wang Q, Strauss L, Boussiotis VA. Revisiting the PD-1 pathway. Sci Adv. (2020) 6:eabd2712. doi: 10.1126/sciadv.abd2712
194. Xu X, Hou B, Fulzele A, Masubuchi T, Zhao Y, Wu Z, et al. PD-1 and BTLA regulate T cell signaling differentially and only partially through SHP1 and SHP2. J Cell Biol. (2020) 219. doi: 10.1083/jcb.201905085
195. Jones A, Bourque J, Kuehm L, Opejin A, Teague RM, Gross C, et al. Immunomodulatory functions of BTLA and HVEM govern induction of extrathymic regulatory T cells and tolerance by dendritic cells. Immunity. (2016) 45:1066–77. doi: 10.1016/j.immuni.2016.10.008
196. Yuan B, Zhao L, Fu F, Liu Y, Lin C, Wu X, et al. A novel nanoparticle containing MOG peptide with BTLA induces T cell tolerance and prevents multiple sclerosis. Mol Immunol. (2014) 57:93–9. doi: 10.1016/j.molimm.2013.08.006
197. Chen X, Yang X, Yuan P, Jin R, Bao L, Qiu X, et al. Modular immune-homeostatic microparticles promote immune tolerance in mouse autoimmune models. Sci Trans Med. (2021) 13:eaaw9668. doi: 10.1126/scitranslmed.aaw9668
198. Pei W, Wan X, Shahzad KA, Zhang L, Song S, Jin X, et al. Direct modulation of myelin-autoreactive CD4(+) and CD8(+) T cells in EAE mice by a tolerogenic nanoparticle co-carrying myelin peptide-loaded major histocompatibility complexes, CD47 and multiple regulatory molecules. Int J Nanomed. (2018) 13:3731–50. doi: 10.2147/IJN.S164500
199. Wan X, Pei W, Shahzad KA, Zhang L, Song S, Jin X, et al. A tolerogenic artificial APC durably ameliorates experimental autoimmune encephalomyelitis by directly and selectively modulating myelin peptide-autoreactive CD4(+) and CD8(+) T cells. J Immunol. (2018) 201:1194–210. doi: 10.4049/jimmunol.1800108
200. Muller J, Nitschke L. The role of CD22 and Siglec-G in B-cell tolerance and autoimmune disease. Nat Rev Rheumatol. (2014) 10:422–8. doi: 10.1038/nrrheum.2014.54
201. Macauley MS, Pfrengle F, Rademacher C, Nycholat CM, Gale AJ, von Drygalski A, et al. Antigenic liposomes displaying CD22 ligands induce antigen-specific B cell apoptosis. J Clin Invest. (2013) 123:3074–83. doi: 10.1172/JCI69187
202. Pfrengle F, Macauley MS, Kawasaki N, Paulson JC. Copresentation of antigen and ligands of Siglec-G induces B cell tolerance independent of CD22. J Immunol. (2013) 191:1724–31. doi: 10.4049/jimmunol.1300921
203. Brzezicka KA, Arlian BM, Wang S, Olmer M, Lotz M, Paulson JC. Suppression of autoimmune rheumatoid arthritis with hybrid nanoparticles that induce B and T cell tolerance to self-antigen. ACS Nano. (2022) 16:20206–21. doi: 10.1021/acsnano.2c05643
204. Srivastava A, Arlian BM, Pang L, Kishimoto TK, Paulson JC. Tolerogenic nanoparticles impacting B and T lymphocyte responses delay autoimmune arthritis in K/bxN mice. ACS Chem Biol. (2021) 16:1985–93. doi: 10.1021/acschembio.1c00212
205. Pang L, Macauley MS, Arlian BM, Nycholat CM, Paulson JC. Encapsulating an immunosuppressant enhances tolerance induction by siglec-engaging tolerogenic liposomes. Chembiochem. (2017) 18:1226–33. doi: 10.1002/cbic.201600702
206. Birge RB, Boeltz S, Kumar S, Carlson J, Wanderley J, Calianese D, et al. Phosphatidylserine is a global immunosuppressive signal in efferocytosis, infectious disease, and cancer. Cell Death Differ. (2016) 23:962–78. doi: 10.1038/cdd.2016.11
207. Fathallah AM, Ramakrishnan R, Balu-Iyer SV. O-phospho-l-serine mediates hyporesponsiveness toward FVIII in hemophilia A-murine model by inducing tolerogenic properties in dendritic cells. J Pharm Sci. (2014) 103:3457–63. doi: 10.1002/jps.24173
208. Glassman FY, Balu-Iyer SV. Subcutaneous administration of Lyso-phosphatidylserine nanoparticles induces immunological tolerance towards Factor VIII in a Hemophilia A mouse model. Int J Pharm. (2018) 548:642–8. doi: 10.1016/j.ijpharm.2018.07.018
209. Pujol-Autonell I, Serracant-Prat A, Cano-Sarabia M, Ampudia RM, Rodriguez-Fernandez S, Sanchez A, et al. Use of autoantigen-loaded phosphatidylserine-liposomes to arrest autoimmunity in type 1 diabetes. PloS One. (2015) 10:e0127057. doi: 10.1371/journal.pone.0127057
210. Mai Y, Yu X, Gao T, Wei Y, Meng T, Zuo W, et al. Autoantigenic peptide and immunomodulator codelivery system for rheumatoid arthritis treatment by reestablishing immune tolerance. ACS Appl Mater Interfaces. (2024) 16(16):20119–31. doi: 10.1021/acsami.4c00296
211. Li A, Ojogho O, Franco E, Baron P, Iwaki Y, Escher A. Pro-apoptotic DNA vaccination ameliorates new onset of autoimmune diabetes in NOD mice and induces foxp3+ regulatory T cells in vitro. Vaccine. (2006) 24:5036–46. doi: 10.1016/j.vaccine.2006.03.041
212. Li AF, Hough J, Henderson D, Escher A. Co-delivery of pro-apoptotic BAX with a DNA vaccine recruits dendritic cells and promotes efficacy of autoimmune diabetes prevention in mice. Vaccine. (2004) 22:1751–63. doi: 10.1016/j.vaccine.2003.10.049
213. Pulendran B, SA P, O’Hagan DT. Emerging concepts in the science of vaccine adjuvants. Nat Rev Drug Discovery. (2021) 20:454–75. doi: 10.1038/s41573-021-00163-y
214. Bach JF. The hygiene hypothesis in autoimmunity: the role of pathogens and commensals. Nat Rev Immunol. (2018) 18:105–20. doi: 10.1038/nri.2017.111
215. Zhou F, Zhang GX, Rostami A. LPS-treated bone marrow-derived dendritic cells induce immune tolerance through modulating differentiation of CD4(+) regulatory T cell subpopulations mediated by 3G11 and CD127. Immunol Res. (2017) 65:630–8. doi: 10.1007/s12026-016-8881-z
216. Schulke S, Burggraf M, Waibler Z, Wangorsch A, Wolfheimer S, Kalinke U, et al. A fusion protein of flagellin and ovalbumin suppresses the TH2 response and prevents murine intestinal allergy. J Allergy Clin Immunol. (2011) 128:1340–8 e12. doi: 10.1016/j.jaci.2011.07.036
217. Schulke S, Wolfheimer S, Gadermaier G, Wangorsch A, Siebeneicher S, Briza P, et al. Prevention of intestinal allergy in mice by rflaA:Ova is associated with enforced antigen processing and TLR5-dependent IL-10 secretion by mDC. PloS One. (2014) 9:e87822. doi: 10.1371/journal.pone.0087822
218. Lee SE, Koh YI, Kim MK, Kim YR, Kim SY, Nam JH, et al. Inhibition of airway allergic disease by co-administration of flagellin with allergen. J Clin Immunol. (2008) 28:157–65. doi: 10.1007/s10875-007-9138-3
219. Shim JU, Lee SE, Hwang W, Lee C, Park JW, Sohn JH, et al. Flagellin suppresses experimental asthma by generating regulatory dendritic cells and T cells. J Allergy Clin Immunol. (2016) 137:426–35. doi: 10.1016/j.jaci.2015.07.010
220. Tan W, Zheng JH, Duong TMN, Koh YI, Lee SE, Rhee JH. A fusion protein of derp2 allergen and flagellin suppresses experimental allergic asthma. Allergy Asthma Immunol Res. (2019) 11:254–66. doi: 10.4168/aair.2019.11.2.254
221. Zonneveld-Huijssoon E, van Wijk F, Roord S, Delemarre E, Meerding J, de Jager W, et al. TLR9 agonist CpG enhances protective nasal HSP60 peptide vaccine efficacy in experimental autoimmune arthritis. Ann Rheum Dis. (2012) 71:1706–15. doi: 10.1136/annrheumdis-2011-201131
222. Benito-Villalvilla C, Soria I, Subiza JL, Palomares O. Novel vaccines targeting dendritic cells by coupling allergoids to mannan. Allergo J Int. (2018) 27:256–62. doi: 10.1007/s40629-018-0069-8
223. Zhang W, Cheng H, Gui Y, Zhan Q, Li S, Qiao W, et al. Mannose treatment: A promising novel strategy to suppress inflammation. Front Immunol. (2021) 12:756920. doi: 10.3389/fimmu.2021.756920
224. Karumuthil-Melethil S, Gudi R, Johnson BM, Perez N, Vasu C. Fungal beta-glucan, a Dectin-1 ligand, promotes protection from type 1 diabetes by inducing regulatory innate immune response. J Immunol. (2014) 193:3308–21. doi: 10.4049/jimmunol.1400186
225. Sirvent S, Soria I, Cirauqui C, Cases B, Manzano AI, Diez-Rivero CM, et al. Novel vaccines targeting dendritic cells by coupling allergoids to nonoxidized mannan enhance allergen uptake and induce functional regulatory T cells through programmed death ligand 1. J Allergy Clin Immunol. (2016) 138:558–67 e11. doi: 10.1016/j.jaci.2016.02.029
226. Soria I, Lopez-Relano J, Vinuela M, Tudela JI, Angelina A, Benito-Villalvilla C, et al. Oral myeloid cells uptake allergoids coupled to mannan driving Th1/Treg responses upon sublingual delivery in mice. Allergy. (2018) 73:875–84. doi: 10.1111/all.2018.73.issue-4
227. Ojeda P, Barjau MC, Subiza J, Moreno A, Ojeda I, Solano E, et al. Grass pollen allergoids conjugated with mannan for subcutaneous and sublingual immunotherapy: a dose-finding study. Front Immunol. (2024) 15:1431351. doi: 10.3389/fimmu.2024.1431351
228. Nieto A, Mazon A, Nieto M, Ibanez E, Jang DT, Calaforra S, et al. First-in-human phase 2 trial with mite allergoids coupled to mannan in subcutaneous and sublingual immunotherapy. Allergy. (2022) 77:3096–107. doi: 10.1111/all.v77.10
229. Camby I, Le Mercier M, Lefranc F, Kiss R. Galectin-1: a small protein with major functions. Glycobiology. (2006) 16:137R–57R. doi: 10.1093/glycob/cwl025
230. Ilarregui JM, Croci DO, Bianco GA, Toscano MA, Salatino M, Vermeulen ME, et al. Tolerogenic signals delivered by dendritic cells to T cells through a galectin-1-driven immunoregulatory circuit involving interleukin 27 and interleukin 10. Nat Immunol. (2009) 10:981–91. doi: 10.1038/ni.1772
231. Macallan DC, Borghans JA, Asquith B. Human T cell memory: A dynamic view. Vaccines (Basel). (2017) 5:5. doi: 10.3390/vaccines5010005
232. Rubtsov YP, Niec RE, Josefowicz S, Li L, Darce J, Mathis D, et al. Stability of the regulatory T cell lineage in vivo. Science. (2010) 329:1667–71. doi: 10.1126/science.1191996
233. Mannie MD, DeOca KB, Bastian AG, Moorman CD. Tolerogenic vaccines: Targeting the antigenic and cytokine niches of FOXP3(+) regulatory T cells. Cell Immunol. (2020) 355:104173. doi: 10.1016/j.cellimm.2020.104173
234. Weiner HL, Mackin GA, Matsui M, Orav EJ, Khoury SJ, Dawson DM, et al. Double-blind pilot trial of oral tolerization with myelin antigens in multiple sclerosis. Science. (1993) 259:1321–4. doi: 10.1126/science.7680493
Keywords: tolerogenic adjuvant, tolerogenic vaccine, autoimmune disease, immune tolerance, regulatory T cells, tolerogenic dendritic cells, immunoregulation, adjuvant
Citation: Arve-Butler S and Moorman CD (2024) A comprehensive overview of tolerogenic vaccine adjuvants and their modes of action. Front. Immunol. 15:1494499. doi: 10.3389/fimmu.2024.1494499
Received: 11 September 2024; Accepted: 29 November 2024;
Published: 20 December 2024.
Edited by:
Guobao Chen, AbbVie’s Cambridge Research Center, United StatesReviewed by:
Salvador Iborra, Inmunotek SL, SpainKrzysztof Bryniarski, Jagiellonian University Medical College, Poland
Copyright © 2024 Arve-Butler and Moorman. This is an open-access article distributed under the terms of the Creative Commons Attribution License (CC BY). The use, distribution or reproduction in other forums is permitted, provided the original author(s) and the copyright owner(s) are credited and that the original publication in this journal is cited, in accordance with accepted academic practice. No use, distribution or reproduction is permitted which does not comply with these terms.
*Correspondence: Cody Deumont Moorman, Y21vb3JtYW5AYW1nZW4uY29t