- 1Senior Department of Hematology, the Fifth Medical Center of PLA General Hospital, Beijing, China
- 2Graduate School, Chinese PLA General Hospital, Beijing, China
- 3State Key Laboratory of Experimental Hematology, the Fifth Medical Center of PLA General Hospital, Beijing, China
The principle of immune checkpoint blockade therapy is based on the activation of T cells. Immune checkpoint inhibitors (ICIs), such as anti-PD-1/PD-L1 and anti-CTLA-4 antibodies, have demonstrated effectiveness in treating solid tumors by reinvigorating the immune system to recognize and eliminate malignant cells. In recent years, ICIs have shown promise in certain patients with relapsed or refractory lymphoma and myeloid malignancies. Allogeneic hematopoietic stem cell transplant (allo-HCT) currently remains the only curative immunotherapy option for eligible patients with these hematologic malignancies. An increasing number of patients with indications for allo-HCT have received treatment with ICIs either before the procedure or as a therapy for relapse after allo-HCT. Nevertheless, initial reports suggest that patients exposed to immune checkpoint inhibitors either before or after allo-HCT are at an increased risk of developing severe graft-versus-host disease and other immune-related adverse events, likely due to the persistent effects of immune checkpoint blocking. Maximizing therapeutic benefits while minimizing side effects of the combination of checkpoint blockade immunotherapy and allo-HCT is an active area of research aimed at improving the prognosis of relapsed or refractory hematologic malignancies. However, there is still a lack of rational design strategies to optimize the combined use of these two different types of immunotherapies. In this review, we addressed the scientific rationale behind ICIs for treating lymphoma and myeloid malignancies. We also summarized the evidence supporting the use of ICIs as salvage therapy before and after allo-HCT. Additionally, we offered insights into current approaches for preventing and treating graft-versus-host disease and other immune-related adverse events during the procedure.
Introduction
The immune system is regulated by a precise system of checks and balances that mediate protective immunity against invading pathogens while maintaining self-tolerance (1). The immune checkpoints consist of several stimulatory and inhibitory mechanisms that control the function of cells within the innate and adaptive immune system (2). Stimulatory checkpoint pathways promote activation and proliferation of T helper cells or effector CD8+ T cells. Conversely, inhibitory checkpoint pathways can regulate the extent of T cell activation and duration of immune responses, thereby preventing autoimmune reactions (3). Tumors can hijack inhibitory immune checkpoints to facilitate immune escape, making them attractive targets for cancer immunotherapy. For instance, many tumor cells inappropriately express programmed cell death 1 ligand 1 (PD-L1) protein, enabling them to evade attack from immune killer cells. Blocking the T cell checkpoint inhibitors programmed cell death protein 1 (PD-1) or signaling PD-L1 on cancer cells has demonstrated remarkably durable clinical responses in certain cancers, as it releases T cells from checkpoint inhibition to unleash antitumor activity (4–8). The primary therapeutic modalities for checkpoint blockade therapy involve using monoclonal antibodies engineered to block interactions between immune checkpoint receptors and their ligands. This prevents the off signal and allows immune cells to eliminate cancer cells.
Despite the promising clinical efficacy in multiple cancer types, currently approved immune checkpoint inhibitors (ICIs) only benefit a subset of patients with hematologic malignancies (9, 10). Even among those patients who initially responded, relapse may have occurred eventually due to acquired resistance, indicating that ICIs alone are unlikely to be curative for the majority of patients (11–13). Allogeneic hematopoietic stem cell transplant (allo-HCT) remains the only potentially curative therapeutic modality for most patients with prior checkpoint blockade therapy (14, 15). However, concerns have been raised about the safety of using ICIs either before or after allo-HCT due to potential increased rates and severity of subsequent toxicity, such as graft-versus-host disease (GVHD) and other immune-related adverse events (irAEs) (16–18). Many questions remain regarding the implications of immunomodulatory effects of checkpoint blockade, the optimal prophylactic and therapeutic strategy for GVHD, as well as the safety and efficacy of checkpoint blockade therapy in those patients experiencing relapse after allo-HCT. The lack of prospective data makes these treatment decisions challenging, but clinicians caring for patients with hematologic malignancies must address these important questions in their routine practice.
In this review, we discuss the mechanisms of action of ICIs in lymphoma and myeloid malignancies. We present a summary of the evidence that supports the use of these agents as salvage therapy before and after allo-HCT, with a specific focus on transplant outcomes and methods for preventing GVHD. Furthermore, based on available data and clinical experience, we also provide our recommendations to assist clinicians in guiding their practice until more definitive evidence is obtained.
Role of immune checkpoint inhibition in hematologic malignancies control
During the past decade, immune checkpoint blockade therapies, including anti-PD-1 (pembrolizumab, nivolumab, cemiplimab and tislelizumab), anti-PD-L1 (atezolizumab, avelumab, and durvalumab), and anti-CTLA-4 (ipilimumab), have significantly revolutionized the treatment approach for hematologic malignancies. Furthermore, the ongoing discovery of novel immune checkpoints has led to the emergence of drugs targeting new molecules such as T-cell immunoglobulin and mucin domain 3 (TIM-3), Lymphocyte-activation gene 3 (LAG-3), and T cell immunoreceptor with immunoglobulin and ITIM domain (TIGIT) on a continual basis (Figure 1).
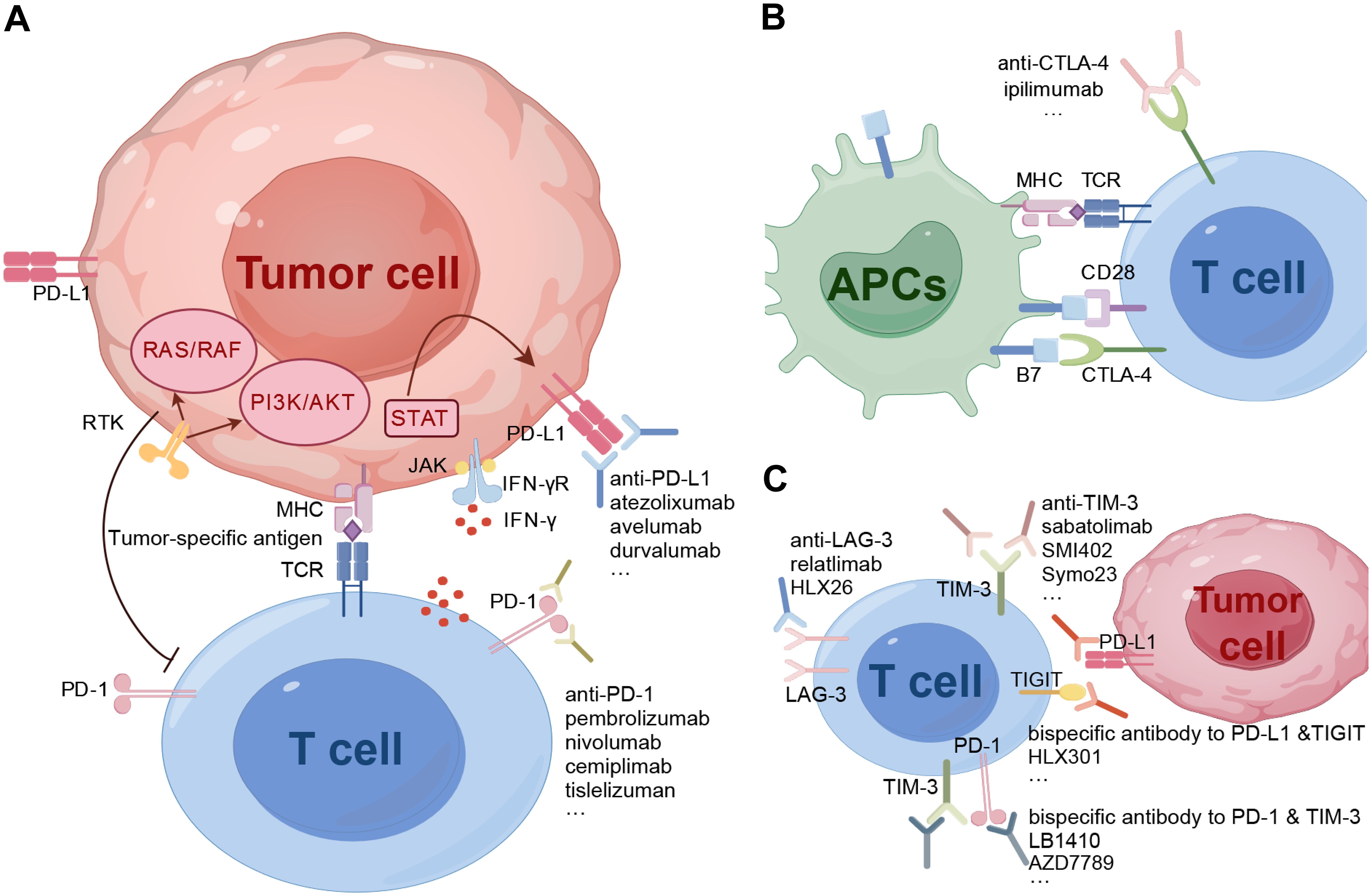
Figure 1. Inhibitory immune checkpoint molecules and emerging checkpoint inhibitors. (A), IFNγ produced from T cells binds to the IFNγ receptor, leading to the regulation of PD-L1 expression through the JAK-STAT pathway. Both the JAK-STAT pathway and RTKs pathway have immunosuppressive functions. (B), CTLA-4 competitively binds to B7, inhibiting the proliferation and activation of T cells. (C), In addition to classical molecules, other immune checkpoints on T cells such as LAG-3, TIM-3, and TIGIT also contribute to immune suppression. Mechanisms of checkpoint inhibitors involve targeting these inhibitory checkpoint molecules expressed by T- and antigen-presenting cells. By blocking these receptors, immune checkpoint inhibitors promote the proper induction and differentiation of T cell-mediated immunity. APCs, antigen presenting cells; CTLA-4, cytotoxic T lymphocyte-associated protein 4; LAG-3, lymphocyte activation gene-3; MHC, major histocompatibility complex; PD-1, programmed cell death protein 1; PD-L1, programmed cell death protein ligand 1; RTK, receptor tyrosine kinase; TCR, T cell receptor; TIGIT, T cell immunoreceptor with immunoglobulin and ITIM domain; TIM-3, T cell immunoglobulin and mucin-domain containing-3.
T cell activation is initiated by the recognition of peptides presented by major histocompatibility complex (MHC) molecules through their surface receptor, known as the T cell receptor (TCR). This recognition triggers the production of inflammatory cytokines and initiates the inflammatory response. The cytokines produced by activated T cells, such as interferon γ (IFN-γ) and tumor necrosis factor α (TNF-α), have the ability to up-regulate PD-L1 expression on tumor cells. The interaction between PD-L1 and PD-1 on immune cells leads to immune tolerance. Upon binding, the intracellular ITIM of PD-1 becomes phosphorylated and binds to the protein tyrosine phosphatase SHP-1, while the intracellular ITSM of PD-1 becomes phosphorylated and binds to the protein tyrosine phosphatase SHP-2. The recruited SHP-2 then dephosphorylates and inactivates downstream molecules in the TCR signaling pathway, including extracellular regulated protein kinase (ERK) and phosphatidylinositol-3-kinase (PI3K), thereby inhibiting TCR-mediated effector functions and cytotoxic T cell activity (19). The subsequent inhibition of TCR-mediated signaling activation and cell proliferation results in T-cell exhaustion. Zhou et al. have demonstrated that the coexpression of TIM-3 and PD-1 identifies a CD8+ T-cell exhaustion phenotype in mice with disseminated AML (20). Additionally, PD-1 has the ability to up-regulate genes that inhibit T-cell function, such as alkaline leucine zipper transcription factors ATF. This can ultimately lead to T cell depletion (21). Moreover, the activation of the PD-1/PD-L1 pathway can enhance the expression of IL-10 and result in dysfunction of T cell (22). Therefore, the inhibitory PD-1/PD-L1 checkpoint pathway plays an important role in the immune evasion of tumor cells. The strategies of anti-PD-1/PD-L1 immunotherapy aim to preserve the antitumor capacity of T cells by overcoming the PD-1/PD-L1 interaction, thereby inhibiting the immune escape of tumor cells. These strategies show promise in the treatment of R/R hematologic malignancies. In classic Hodgkin’s lymphoma (cHL), the common copy number gain of chromosome 9p24.1 leads to the upregulation of PD-L1 expression on Reed-Sternberg cells, prompting the systematic development of PD-1 checkpoint inhibitors in hematologic malignancies (23). Furthermore, the expression of PD-L1 was found to be up-regulated on murine leukemia C1498 cells when they were grown in vivo. When PD-1 knock-out mice were challenged with C1498 cells, they exhibited enhanced antitumor T-cell responses, reduced AML burden in the blood and tissues, and had significantly prolonged survival compared to wild-type mice. Similar outcomes were observed following treatment with anti-PD-L1 antibody therapy (24). Additionally, the frequency of PD-1+/CD8+ T cells was found to be higher in bone marrow samples obtained from patients with multiply relapsed AML compared to those with first relapsed or newly-diagnosed AML (25). Significantly, the high expression of PD-1, PD-L1, and PD-L2 in bone marrow samples from patients with AML has been linked to poor overall survival (OS) (26). These findings establish a scientific rationale for the utilization of PD-1 or PD-L1 antibodies in the treatment of AML patients.
CTLA-4 is a crucial immune checkpoint molecule predominantly expressed on activated T cells and regulatory T cells (Tregs). It plays a pivotal role in inhibiting T-cell activation and regulating immune homeostasis. In both in vivo and in vitro T cell responses, overexpression of CTLA-4 has been shown to inhibit T cell activation, which is dependent on the interaction between B7 and CD28. Furthermore, CTLA-4 on T cells has the ability to capture B7 molecules from antigen-presenting cells (APCs), leading to degradation of B7 ligands and subsequently inhibiting the CD28/B7 costimulatory pathway (27). CTLA-4 binds to B7 and triggers reverse signal transduction, leading to the activation of indoleamine 2,3-dioxygenase (IDO). This in turn induces tryptophan catabolism, thereby inhibiting T cell proliferation and activation (28, 29). Therefore, the CTLA-4/B7-mediated immune checkpoint in tumor microenvironment (TME) is another important component of the tumor immune escape. Therefore, the high expression of CTLA-4 in TME will inhibit proliferation and activation of T cells through multiple mechanisms, inducing tumor immune escape. Anti-CTLA-4 antibody can increase CD28 signaling in T cells, prevent Treg cell-mediated B7 endocytosis, and enable T cells to be activated. Saudemont et al. demonstrated that blocking B7/CTLA-4 interaction enhanced cytotoxic T cell-mediated killing of the leukemia cells in the DA1-3b murine AML model and prolonged the survival of mice (30). In cHL, there is a greater enrichment of CTLA-4 positive T cells, with their numbers exceeding that of PD-1+/LAG-3+ T cells (31).
Apart from CTLA-4 and PD-1, the upregulation of other immune checkpoint molecules such as LAG-3, TIM-3, and TIGIT has been confirmed (32). LAG-3, which shares structural similarities with CD4, competes for binding to MHC class II molecules, resulting in decreased efficiency of antigen presentation and thereby inhibiting the anti-tumor response. A study has demonstrated that T cell dysfunction could be reversed by removing the LAG-3 expressed Tregs in HL (33). TIM-3 expressed on myeloid leukemia cells interacts with ligands on T cells and suppresses anti-tumor immunity (34), which is correlated with poor clinical outcomes (35). Sabatolimab is an inhibitor of TIM-3 demonstrating effects against leukemia and is presently being studied in clinical trials (36).
The use of immune checkpoint blockade prior to Allo-HCT
Prior exposure to ICIs before allo-HCT is associated with better survival but also higher risk of GVHD
Immunotherapy has demonstrated efficacy in the treatment of malignant lymphomas, particularly in cHL and primary mediastinal B-cell lymphoma (PMBCL). A study involving 243 relapsed or refractory (R/R) cHL patients treated with nivolumab showed an overall response rate (ORR) of 71.2%, with a complete response (CR) rate of 21.4%. Notably, the median OS was not reached, and the 5-year OS was 71.4% (37). The phase 2 KEYNOTE-087 trial, which involved 210 participants with R/R cHL, reported an ORR of 71.9% and a CR rate of 27.6% with pembrolizumab treatment. The median OS was not reached, and the progression-free survival (PFS) was 13.7 months (38). Apart from PMBCL, the outcomes following ICIs in Non-Hodgkin Lymphoma (NHL) have been disappointing, with few durable responses. The phase 1b KEYNOTE-013 study reported an ORR of only 22% (19/86) in R/R NHL patients who received pembrolizumab. Furthermore, when considering disease type, the highest ORR was observed in PMBCL at 48% (10/21) (39). Despite the significant promise shown by PD-1 inhibition in enhancing ORR, a substantial proportion of patients do not experience lasting effects from this treatment. As a crucial therapeutic option for hematological malignancies, allo-HCT offers a viable choice for young patients who do not achieve CR after undergoing therapy with PD-1 blockade.
Allo-HCT following PD-1 blockade is linked to enhanced survival, but it also carries an increased risk of GVHD or other irAEs. Merryman RW et al. identified 31 patients with HL who underwent PD-1 blockade followed by allo-HCT. The 1-year OS and PFS were 90% and 74%, respectively. However, the cumulative incidence rates for grade 3-4 acute GVHD were found to be at 26%, with a rate of 13% for grade 4 acute GVHD, both figures markedly exceeding previous reports of 23% for grades 2-4 acute GVHD and 8% for grades 3-4 acute GVHD (40). Additionally, 3 patients developed severe hepatic veno-occlusive disease (VOD), and 7 patients experienced febrile syndrome (16). Another retrospective study reported on 13 patients with R/R cHL who underwent allo-HCT after PD-1 blockade therapy. At the time of allo-HCT, 4 patients achieved CR, 7 achieved partial remission (PR), and 2 had persistent disease. Interestingly, all patients achieved CR following allo-HCT. The OS and PFS at 57.4 months were 90.9% and 75.5%, respectively. However, acute GVHD developed in 5 patients (38.5%) within 3 to 5 weeks after transplantation, and non-infectious fever occurred in 7 patients (54%) (41).
Data regarding NHL patients undergoing allo-HCT following treatment with ICIs is scarce, as this information is primarily included in larger clinical studies focused on HL patients (16, 41). In investigations of ICIs for NHL, a limited number of patients proceed to allo-HCT; however, detailed data on these cases is not provided (42–45). In the KEYNOTE-013 and KEYNOTE-170 studies, 9 patients with R/R PMBCL underwent subsequent HCT following treatment with pembrolizumab. Among the 4 patients who received autologous HCT (auto-HCT), all were alive and in ongoing CR. Of the 5 patients who received allo-HCT, 3 remained alive with no evidence of disease, 1 survived but experienced disease recurrence, and 1 succumbed to disease progression at data cutoff (43). In another study, CheckMate 436, 12 patients with R/R PMBCL received allo-HCT (n=6) or auto-HCT (n=6) following treatment with nivolumab plus brentuximab vedotin (BV). At the 100-day after transplantation, 1 patient died due to neurological complications, while the remaining 11 patients were alive with CR. The 2-year CR rates were 80% for allo-HCT and 100% for auto-HCT. These results suggest that ICIs could be a viable strategy for bridging to auto-HCT or allo-HCT in patients with R/R PMBCL (45).
For many patients diagnosed with AML or myelodysplastic syndromes (MDS), allo-HCT remains the primary treatment strategy aimed at achieving a cure. However, there is limited data available on the effectiveness and safety of allo-HCT following checkpoint inhibition therapy in patients with AML or MDS (46). We have conducted a prospective study that included 27 patients with R/R AML. These patients received a combination of the PD-1 inhibitor tislelizumab, along with a hypomethylating agent (HMA) and the CAG (cytarabine, aclarubicin/idarubicin, granulocyte colony-stimulating factor) regimen. Among these patients, 11 proceeded to allo-HCT. In our study, 5 patients (45.5%) experienced acute GVHD (grade 1-2 acute GVHD n = 3, grade 3-4 acute GVHD n = 2), and 1 patient (9.1%) developed mild chronic GVHD. Unfortunately, 1 patient died from grade 4 gastrointestinal tract GVHD on day 36 after transplantation. A total of 5 patients remained alive and in remission at the follow-up time points ranging from 6.8 to 22 months post-transplantation (47). In a subsequent study, the safety and efficacy of allo-HSCT were assessed in 15 R/R AML patients (12 haploidentical [HIDs], 2 matched siblings, 1 unrelated donor) who received the tislelizumab + HMA + CAG regimen. Among the HIDs patients, four received GVHD prophylaxis with anti-thymocyte globulin and reduced-dose posttransplant cyclophosphamide (PTCy). With a median follow-up of 20.9 months, the 2-year OS and GVHD-free/relapse-free survival rates (GRFS) were found to be 54% and 48.6%, respectively. Notably, no deaths or relapses were observed in the PTCy group, suggesting a potential survival benefit associated with using PTCy for GVHD prophylaxis in HID-HCT. The regimen demonstrated tolerability along with promising efficacy for bridging to allo-HSCT in R/R AML (48).
PTCy-based GVHD prophylaxis mitigates GVHD associated with exposure to ICIs prior to allo-HCT
Multiple studies have demonstrated the efficacy of PTCy in preventing GVHD in individuals undergoing ICIs treatment (Table 1). Schoch LK et al. (17) conducted a retrospective study of 14 patients who underwent reduced-intensity conditioning (RIC) and received PTCy as GVHD prophylaxis, including 10 patients with HL, 2 patients with AML/MDS, and 2 patients with NHL. Importantly, none of the patients experienced grade 3 to 4 acute GVHD or chronic GVHD. At the end of the study, all 10 HL patients remained free from disease progression, with a median follow-up of 12.7 months. Similarly, a retrospective study compared 34 patients with R/R HL, of whom 10 received nivolumab before allo-HCT and 24 did not. Both groups received PTCy for GVHD prophylaxis. Only 1 patient in each group experienced grade 3 acute GVHD. The incidence of acute GVHD and chronic GVHD showed no statistically significant difference between the two groups. Furthermore, the OS in the nivolumab group was superior to that of the control group (80% vs 41.7%) (49). This study suggests that the use of PTCy alleviate the increased risk of GVHD associated with ICIs.
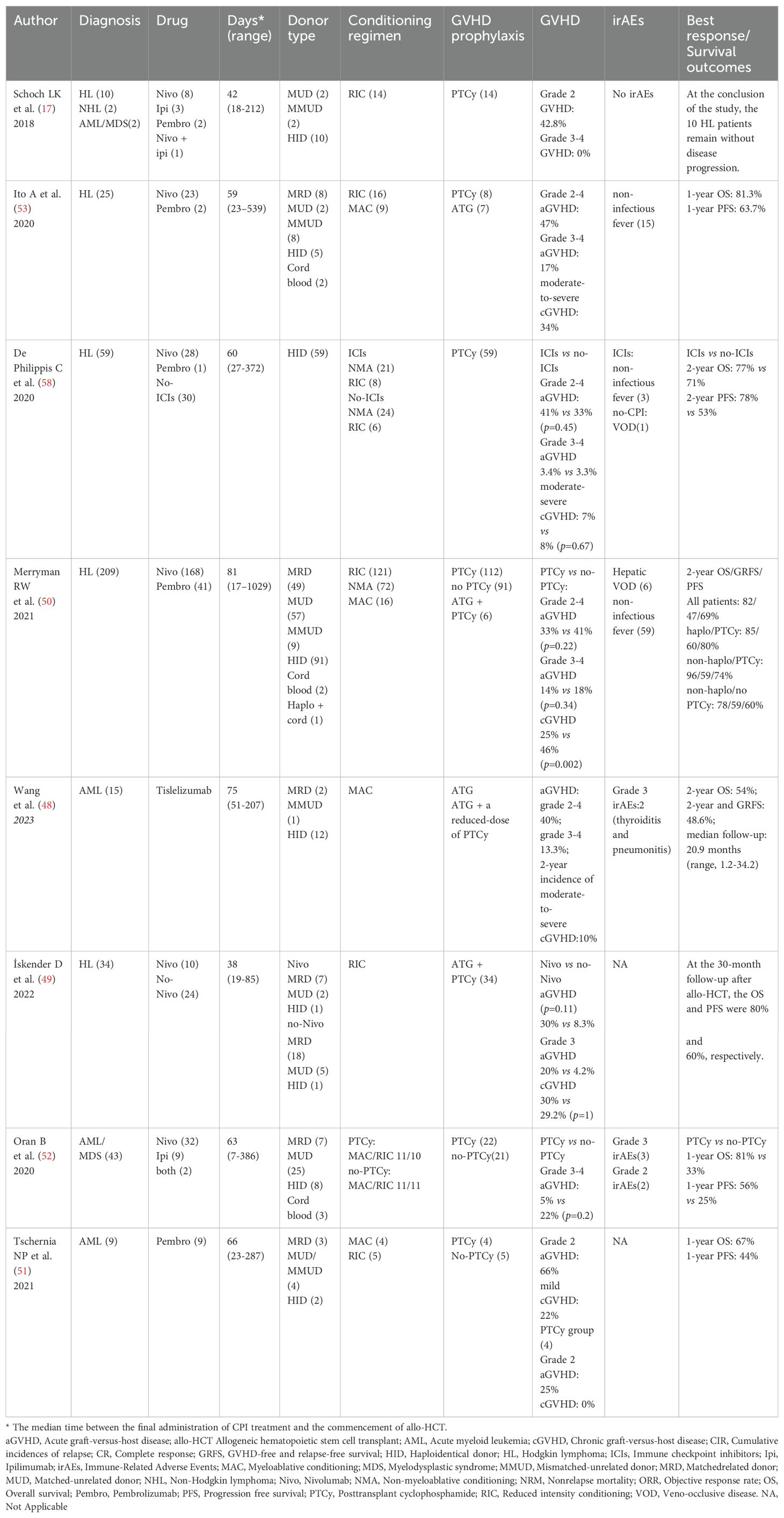
Table 1. Studies showing improved outcomes with PTCy-based GVHD prophylaxis in Allo-HCT with prior ICI exposure.
To determine whether the application of PTCy could reduce the incidence of GVHD in patients with HL, Merryman RW et al. conducted a study involving 209 HL patients who had undergone allo-HCT following prior treatment with PD-1 blockade. Of these patients, 112 (54%) received PTCy-based GVHD prophylaxis. Six patients developed grade 2-4 acute GVHD within the first 14 days following allo-HCT, and none of them had received PTCy prophylaxis. The incidence of grade 2-4 and grade 3-4 acute GVHD was 33% and 14%, respectively, in the patients who received PTCy. Multivariable analyses revealed that the use of PTCy for GVHD prophylaxis was associated with a significantly lower risk of chronic GVHD (25% vs 46%), but not with a reduced risk of acute GVHD (33% vs 41%). The only significant factor predicting the occurrence of acute GVHD was the time from the last dose of anti-PD-1 antibody to transplantation. Importantly, PTCy-based GVHD prophylaxis was associated with improved PFS and GRFS (50).
Similarly, PTCy-based GVHD prophylaxis mitigates severe GVHD in AML/MDS patients. In a retrospective analysis, 9 patients with R/R AML treated with pembrolizumab followed by allo-HCT, including 4 patients with PTCy-based GVHD prophylaxis. The 100-day mortality was 0%. Six patients (67%) developed grade 2 acute GVHD and no patients had grade 3-4 acute GVHD. The 1-year OS and PFS after allo-HCT were 67% and 44%, respectively. The median OS was 21 months with a median follow-up of 23 months (51). Oran B et al. conducted a study on the treatment outcomes of allo-HCT in 43 AML/MDS patients who received nivolumab (n=32), ipilimumab (n=9), or both (n=2) as part of their treatment regimen. The patients were divided into two groups based on the application of PTCy. In the PTCy group, consisting of 22 patients, the 100-day cumulative incidence of grade 3-4 acute GVHD was 5%, compared to 22% in the no-PTCy group. The results showed that in the PTCy group, the1-year OS and PFS rates were 81% and 56%, respectively. In contrast, the no-PTCy group had markedly inferior outcomes, with 1-year OS and PFS rates at 33% and 25%, respectively. Furthermore, only 5 patients experienced irAEs, including 3 grade 3 irAEs and 2 grade 2 irAEs (52). These findings suggest that the utilization of PTCy may improve the outcomes of transplantation without elevating the incidence of GVHD in AML/MDS patients who had received ICIs treatment prior to allo-HCT.
The above evidence (16, 17, 50, 53–56) demonstrates that allo-HCT following treatment with anti-PD-1 antibodies is associated with overall favorable outcomes. The majority of studies support the use of PTCy-based GVHD prophylaxis, which has been linked to prolonged survival and a reduced likelihood of developing GVHD (17, 50, 57, 58). Unlike PTCy, ex vivo T cell depletion (TCD) of the allograft represents an alternative strategy for the prevention of GVHD, obviating the need for post-transplant immunosuppressive therapy. There are limited reports regarding the incidence of GVHD in the TCD platform. Casadei B et al. (41) conducted a retrospective case series involving 13 patients with R/R cHL who were treated with an anti-PD-1 monoclonal antibody prior to allo-HCT. All but one patient received a T-cell-depleted graft, and ATG was included in the conditioning regimen for eight patients. The overall incidences of acute and chronic GVHD were found to be 38.5% and 23.1%, respectively. Notably, five out of the thirteen patients experienced acute GVHD, with only one patient presenting with grade II-III severity. A case report by Kim YE et al. (59) detailed the successful treatment of a patient with NK/T-cell lymphoma using pembrolizumab, followed by ex vivo TCRαβ-depleted haploidentical allo-HCT, resulting in sustained remission. The patient did not receive pharmacologic GVHD prophylaxis and did not experience acute GVHD. Given the limited sample size, we are currently unable to determine which of the two GVHD prophylaxis platforms has a lower incidence of GVHD at present, and further investigation and confirmation are required in prospective controlled trials. Additionally, since PTCy may be used in combination with ATG or followed by other immunosuppressive agents, we also cannot determine whether the reduction in GVHD incidence following the use of PTCy-based GVHD prophylaxis is related to other drugs. Larger prospective studies will be necessary to determine the effectiveness of PTCy in combination with other drugs in preventing GVHD, as well as the optimal interval between the last dose of PD-1 inhibitor administration and allo-HCT in order to minimize the occurrence of severe immune-related complications (58).
The mechanism by which PTCy alleviates GVHD
GVHD occurs when the immune cells from a donor recognize the recipient’s allogeneic peptide-HLA as foreign, and the risk of GVHD increases with greater HLA disparity between the donor and recipient. This can lead to an alloreactive response that induces GVHD (60). PD-1, functioning as a coinhibitory receptor, modulates the proliferation, differentiation, and survival of T-cells upon interaction with its ligand, PD-L1. Ikegawa et al. (61) demonstrated that PD-1 blockade promoted sustained proliferation of alloreactive T cells while also promoting a temporary increase in Tregs proliferation. However, within two weeks, the number of Tregs decreases due to elevated susceptibility to apoptosis. This dysregulation of T cell subsets leads to severe GVHD. The authors also observed that alloreactive T cells from PD-1-knockout donor grafts were aggressively proliferative on day 3 and vulnerable to the cytotoxic effects of Cy. However, Tregs were resistant to PTCy because of aldehyde dehydrogenase expression (62). In conclusion, PTCy-based GVHD prophylaxis alleviates GVHD by rebalancing the T cell subsets (57, 61).
In conclusion, the decision to offer allo-HCT as a consolidation treatment option is based on factors such as age, disease status, prior therapies, comorbidity index score (HCT-CI), and donor availability. PD-1 therapy should be withheld for at least 6 to 8 weeks before allo-HCT. Discontinuing PD-1 therapy before transplantation requires careful assessment of any immune-related complications of the patient during treatment, monitoring for signs of disease progression or recurrence, a well-timed cessation plan, and a refined transplant strategy. The transplant strategy, including conditioning intensity and GVHD prophylaxis, should be optimized to reduce posttransplant immune toxicity. We recommend using RIC regimens for patients aged 60 years or older, those with heavy pretreatment, or HCT-CI scores of ≥3 due to the increased risk of VOD and GVHD with ablative conditioning regimens. PTCy-containing GVHD prophylactic regimen is preferred for patients with previous exposure to ICIs. Close monitoring is necessary for these patients as they are more likely to develop severe GVHD and noninfectious febrile syndrome. In cases of noninfectious febrile syndrome, IV methylprednisolone at 1 mg/kg per day should be considered. Rapid initiation of IV methylprednisolone at 2 mg/kg per day is recommended if GVHD occurs. For cases with previous exposure to ICIs and lack of rapid response to systemic steroids, an earlier transition from steroids to second-line therapies for GVHD should be considered compared to a context without checkpoint blockade.
ICIs: an optional approach for relapse post allo-HCT
Recurrence remains the primary cause of transplant failure, primarily due to the loss of the graft-versus-tumor (GVT) effect, resulting in compromised outcomes (63, 64). The primary mechanism underlying immune escape involves the inhibition of allogeneic T cells, often through interaction with inhibitory receptors like PD-1 and CTLA-4 on their cell surfaces (63). Conventional treatments for relapse after allo-HCT, such as discontinuation of immunosuppressive drugs, donor lymphocyte infusion (DLI), salvage chemotherapy, and second transplantation, provide limited potential for achieving a cure and pose an increased risk of GVHD toxicity (65).
Previous reports suggest that ICIs after allo-HCT may reverse T-cell exhaustion and immune evasion, potentially enhancing the GVT effect (66). ICIs have shown significant clinical efficacy in the past decade, making them an attractive option for enhancing the GVT effect in managing post-transplant relapse after allo-HCT, especially in patients with classic cHL (67, 68). If used safely, ICIs could enhance GVT effects and reduce relapse after allo-HCT in lymphoma and potentially other hematological malignancies. Therefore, close monitoring is necessary for both irAEs and GVHD when using ICIs after allo-HCT (65). While most studies have focused on assessing ICIs after allo-HCT for HL, there is an increasing number of investigations into this strategy in other hematologic malignancies (Table 2). The majority of studies primarily focus on using one or two ICIs or their combination with demethylating agents for post-transplant relapse. There are few studies that explore the use of ICIs in combination with targeted drugs or DLI (69, 70).
Lymphomas
Early reports have indicated a high response rate with ICIs in HL patients relapsing after allo-HCT. However, it is important to note that an increased risk of sever GVHD and irAEs has been observed (69, 71–74). A retrospective study of 31 HL patients who underwent allo-HCT followed by treatment with nivolumab or pembrolizumab for relapsed disease showed an ORR of 77% and a CR rate of 50%. Notably, GVHD developed in 55% of the patients after receiving a median of 1 to 2 doses of treatment, including 5 patients without prior GVHD. Importantly, the majority of these patients showed poor response to systemic steroids, leading to complications related to GVHD and ultimately resulting in death for 26% or the cohort (75). Similarly, a multicenter retrospective analysis evaluated the efficacy of nivolumab at a dosage of 3 mg/kg every 2 weeks in HL patients who had relapsed after allo-HCT. Among the 20 identified patients, the ORR was 95%, and the median PFS had not been reached at a median follow-up of 370 days. Six out of the 20 patients (30%) experienced GVHD within one week following their initial dose of nivolumab, all with a history of acute GVHD, suggesting an increased level of allo-reactivity. Of these 6 patients, 3 were unresponsive to steroid treatment, and 2 ultimately succumbed to GVHD. Notably, the interval between allo-HCT and the first administration of nivolumab was shorter for those patients who developed GVHD compared to those who did not; with median times being 8 months versus 28 months, respectively (76). In contrast, another study found no instances of GVHD in 9 patients who received various ICIs after allo-HCT. It is noteworthy that the median time interval between allo-HCT and ICIs was 1.2 years, and all patients had received PTCy-based GVHD prophylaxis (77). Thus, it appears that a longer interval between the first administration of nivolumab and allo-HCT as well as PTCy-based GVHD prophylaxis are associated with reduced occurrence of GVHD.
While the potential association between GVHD and ICIs remains a significant concern, there have been documented cases demonstrating that effective management of GVHD can result in sustained remissions. A case report detailed the experience of a patient with refractory cHL who underwent two allo-HCTs (first from a matched unrelated donor, second from a haploidentical donor) following relapse on BV and nivolumab. Subsequently, BV-pembrolizumab combination therapy was administered for a relapse one year after the second transplant. Although moderate overlap GvHD emerged, necessitating treatment interruption, it was promptly brought under control. Notably, the patient maintained remission for over two years after just two cycles of BV-pembrolizumab combination therapy (78). A long-term study from the Early French Access Program revealed a nearly 40% CR rate in heavily pre-treated R/R HL patients, with 19 patients (25%) achieving remission without further consolidation after a median of 20 Nivolumab cycles. The median PFS was 41.9 months and the median OS was not reached. The 5year estimated PFS was 42.3%. Importantly, 36.8% of patients (7/19) received anti-PD-1 therapy post-allo-HCT, demonstrating its feasibility in this challenging salvage setting (79).
Combination therapies, including ipilimumab in conjunction with other agents, have demonstrated promise in the treatment of post-allo-HCT lymphoma relapses, extending beyond the use of single-agent ICIs. A phase I trial with 29 patients found that a single dose of ipilimumab (0.1 to 3 mg/kg) showed positive responses in lymphoma patients, with no signs of GVHD or severe irAEs (80). In the following phase I/Ib study, higher doses of ipilimumab (3 to 10 mg/kg every three weeks) were given. Only the group receiving 10 mg/kg showed a response rate of 32%. There were 4 cases of GVHD and 6 irAEs, including 1 fatal case involving hepatitis and pneumonitis (25). Notably, patients with a history of grade 3 or 4 acute GVHD or autoimmune disease were excluded from these studies. Khouri et al. found that the combination of ipilimumab and lenalidomide showed promising results in 10 lymphoma patients who relapsed after allo-HCT, with 4 cases of CR and 3 cases of partial remission (PR), and no instances of GVHD (81).
Myeloid malignancies
Treating relapsed AML after HCT remains challenging, as less than 20% of patients achieving lasting remission following chemotherapy combined with a second HCT or donor lymphocyte infusions (82). AML blasts from post-HCT relapse patients exhibit higher expression of inhibitory receptors (IRs) (83). In AML patients experiencing relapse after prolonged post-HSCT remission, functionally impaired CD8+ T cells expressing IRs such as PD-1, CTLA-4, and TIM-3 accumulate in the tumor microenvironment (84). This suggests the potential for ICIs to restore T cell activity and enhance anti-myeloid malignancy immune responses (85). Therefore, the utilization of ICIs for the management of recurrent myeloid malignancies following allo-HCT is increasingly recognized as a promising therapeutic strategy. Holderried et al. conducted a retrospective analysis of checkpoint blockade therapy for disease recurrence after allo-HCT other than HL, including 21 patients (MDS/AML n = 12, NHL n = 5, ALL n = 2, myelofibrosis n=2). The median follow-up was 59 days and the ORR was 43% (70). Patients who received DLI in combination with ICIs had a higher ORR of 80%. However, it is important to note that severe grade 3-4 acute GVHD and moderate to severe chronic GVHD were observed in 29% of all patients, with 83% being refractory to steroid treatment. Therefore, while the combination therapy of checkpoint blockade and DLI may be effective for relapsed patients after allo-HCT, it also poses a higher risk of severe GVHD. A recent Phase I study presented early results of pembrolizumab for treating relapse following allo-HCT (86). Among 9 patients with myeloid malignancies (8 AML, 1 MDS), pembrolizumab had limited effect, with only 2 patients showing stable disease (SD) (4 disease progression, 3 dis-continuation of pembrolizumab due to dose-limiting toxicity). These three participants (25%) with discontinuation of pembrolizumab experienced grade 3 to 4 irAEs, including pneumonitis in 2 patients and hyperthyroidism in 1 patient. All irAEs occurred after 1 to 2 cycles and resolved after discontinuation of pembrolizumab and corticosteroid treatment.
Ipilimumab-mediated CTLA-4 inhibition shows responses in about one-third of patients experiencing relapsed AML or other hematologic malignancies following allo-HCT, while maintaining a manageable safety profile (25, 87). In the phase I/Ib study, ipilimumab was given to 28 patients with relapsed hematological malignancies after allo-SCT, including 12 with AML. Among 22 patients with a dose of 10 mg per kilogram, 5 patients (23%) achieved a CR, including 3 with leukemia cutis, 1 with myeloid sarcoma, and 1 with AML secondary to MDS (25). A multicenter phase 1 trial (NCT02890329; CTEP 10026) is currently underway to investigate the combination of ICIs with decitabine treatment in patients with R/R MDS/AML, both in post-allo-HCT and transplant-naïve settings. Preliminary findings from this early study investigating the use of ICIs following allo-HCT for R/R AML suggest an ORR of 20% and an overall irAE rate of 44% (87). Transcriptomic analyses from the ETCTN/CTEP 10026 study, investigating the effects of decitabine and ipilimumab in AML/MDS patients post-HCT or in HCT-naive settings, have revealed a strong correlation between high baseline T cell-to-AML ratio and treatment response. Conversely, resistance is associated with inadequate clearance of diseased progenitor cells in post-HCT samples (88). Ipilimumab may facilitate regression of post-HSCT relapsed AML by recruiting cytotoxic CD8+ T cells to leukemic sites (88, 89). Further research is warranted to develop effective IPI-based treatment strategies that can achieve enduring responses while minimizing immune toxicity.
In AML patients with extramedullary disease relapse post-transplantation, nivolumab showed potential benefits in a multicenter prospective study. Nivolumab was administered every 2 weeks after allo-HCT for relapsed myeloid (n = 19) or lymphoid (n = 9) hematological malignancies. The initial dose of 1 mg/kg was de-escalated to 0.5 mg/kg due to observed toxicities. Among the patients treated at 1 mg/kg, 2 out of 6 experienced dose-limiting toxicity, while at the lower dose of 0.5 mg/kg, there were 4 cases of dose-limiting toxicity, including irAEs and fatal GVHD. The ORR in evaluable patients was 32% (8/25), with a one-year PFS and OS of 23% and 56%, respectively (90). A pilot study found unexpected severe toxicities in 4 patients with AML/MDS who received low-dose nivolumab as maintenance therapy post-allo-HCT. All 4 patients developed irAEs, with 2 experiencing serious adverse events, including grade 4 neutropenia and grade 3 autoimmune encephalopathy, leading to the termination of the study. It is noteworthy that the median time to drug administration from transplantation was 7.8 months, which may have contributed to increased toxicities (91). Even at low doses, nivolumab maintenance for myeloid malignancies in the post-allo-SCT setting may lead to serious irAEs beyond GVHD. Further research is needed to determine the appropriate dosage and timing of ICIs following allo-HCT (Table 2) (91). In a phase II, single-arm study, sixteen AML patients experiencing relapse following allo-HCT were administered HMA and nivolumab. The median number of nivolumab doses received was 2. At day 42, the ORR (CR/PR) was 25%, with an additional 25% achieving SD. The median OS was 15.6 months. Responders showed a higher frequency of activated (ICOS+, HLA-DR+), low-senescence (KLRG1−, CD57−) CD8+ effector T cells. AEs were consistent with previous studies, with GVHD being a common side effect. Five patients (31.25%) developed grade I-II aGVHD, and four (25%) had cGVHD, including one fatal case. This study suggests HMA/nivolumab treatment post-allo-HCT may dlicit anti-AML immune responses and could potentially serve as a bridge to a second allo-HCT (92).
A retrospective study was conducted on 21 patients with AML (n = 16) or MDS (n = 5) who received anti-PD-1 (16 patients) or anti-CTLA-4 (5 patients) therapy for disease relapse after allo-HCT. Despite early ICI therapy initiation, patients treated with PTCy had a lower observed cumulative incidence of grades 2 to 4 acute GVHD compared to those who did not receive PTCy (16% vs. 22%; p = 0.7). This suggests that PTCy may reduce the incidence of acute GVHD in ICI therapy for relapsed AML/MDS patients after allo-HCT (93). In theory, PTCy is thought to selectively eliminate primarily alloreactive activated T cells while preserving non-cross-reactive memory and naive T cells. It also promotes T regulatory cells and long-term tolerance through intrathymic clonal deletion of anti-host T cells (94–96).
In previous studies, ICIs were mainly used to treat hematologic relapse after allo-HCT. A recent study reported the first case of preemptive treatment using tislelizumab and azacitidine in a patient with MRD-positive AML. The patient received 100 mg of tislelizumab on day 1 and 100 mg of azacitidine on days 1-7 post-transplantation. After the combination therapy, RUNX1-RUNX1T1 transcripts became negative and complete donor chimerism was observed in bone marrow. However, the patient experienced moderate GVHD and irAEs affecting multiple organs, which were managed with immunosuppressive therapies. Using ICIs in MRD-positive patients may be a promising approach for preventing AML relapse post-transplantation, but safer clinical application principles need to be established (97).
In conclusion, the use of ICIs in the treatment of post-allo-HCT relapses holds promise, and their combination with HMAs may further enhance efficacy (98). However, it is important to note that these therapies can also carry the risk of severe side effects such as treatment-resistant GVHD and irAEs. Key risk factors for GVHD include prior history of GVHD, time since allo-HCT, ICI dosage, and transplant characteristics. Further investigation is warranted to determine the potential for improved OS or response rates. More comprehensive prospective studies are necessary to assess the safety and efficacy of ICIs in this context. Additionally, PTCy may provide a safer platform for administering ICIs after relapsed disease in both lymphomas and myeloid malignancies. Patients with a history of GVHD are at higher risk of developing GVHD after ICIs therapy. Additionally, checkpoint blocking before and shortly after transplantation increases the severity of GVHD (75, 99, 100). Considering the limited availability of alternative treatments and anticipated response rates, ICIs therapy may be considered for R/R patients, especially those without a history of GVHD who experience relapse more than 180 days after allo-HCT. Clinicians should carefully weigh the risks and benefits of using checkpoint blockade compared to other available options. Evaluating the expression of checkpoints and their ligands on immune and malignant cells is crucial for achieving positive outcomes (101). Dose optimization studies for ICIs or ICI combination therapy are currently underway in clinical trials focusing on relapse post-allo-HCT (NCT02890329, NCT03600155, NCT03912064, NCT04913922). It is recommended that the starting dose of ICIs outside of a clinical trial should be at a low dose, with close monitoring for evidence of GVHD. Immediate cessation of ICIs and rapid initiation of IV methylprednisolone at 2 mg/kg per day is recommended if GVHD occurs. If the patient does not respond rapidly to steroids, prompt initiation of second-line therapies for GVHD is advised (102). Combining two drugs may be beneficial for patients who do not respond to single ICI therapy, but it necessitates careful consideration of the potential increase in side effects (101).
Conclusion and future directions
Although most patients with R/R hematologic malignancies responding to ICIs will experience a recurrence, allo-HCT remains a viable option for selected patients to prolong response and improve survival. Despite the risk of severe GVHD in some patients who have received ICIs prior to transplantation, studies have shown that this procedure is effective and relatively safe. The use of PTCy-based GVHD prophylaxis strategies appears to offer promising outcomes in terms of both efficacy and safety for these patients. Additionally, ICIs have shown effectiveness in the post-transplant setting; however, an increased incidence of irAEs and fatal GVHD has been reported. Clinical trials evaluating ICIs in relapse after allo-HCT are currently ongoing. The prevailing trend is to integrate ICIs with a range of pharmacological agents and therapeutic modalities. Nevertheless, the lack of comprehensive understanding of resistance mechanisms to ICIs has hindered the identification of an optimal combination therapy. Consequently, high-quality randomized controlled trial data remains scarce in this area. To address this critical knowledge gap and challenge, it is imperative to first identify safe and effective approaches for combining immunotherapies and assess their safety when used in conjunction with allo-HCT. Subsequently, prospective multicenter biomarker-related trials should be conducted.
Author contributions
YH: Investigation, Visualization, Writing – original draft, Writing – review & editing. YW: Investigation, Visualization, Writing – original draft, Writing – review & editing. KM: Investigation, Visualization, Writing – original draft, Writing – review & editing. HZ: Investigation, Writing – original draft, Writing – review & editing. XG: Conceptualization, Funding acquisition, Project administration, Supervision, Validation, Writing – review & editing.
Funding
The author(s) declare financial support was received for the research, authorship, and/or publication of this article. This work was supported by the National Natural Science Foundation of China (No. 82070149 and 82470158 to X-NG).
Conflict of interest
The authors declare that the research was conducted in the absence of any commercial or financial relationships that could be construed as a potential conflict of interest.
Publisher’s note
All claims expressed in this article are solely those of the authors and do not necessarily represent those of their affiliated organizations, or those of the publisher, the editors and the reviewers. Any product that may be evaluated in this article, or claim that may be made by its manufacturer, is not guaranteed or endorsed by the publisher.
References
1. Sharpe AH. Introduction to checkpoint inhibitors and cancer immunotherapy. Immunol Rev. (2017) 276:5–8. doi: 10.1111/imr.12531
2. Sharafi F, Hasani SA, Alesaeidi S, Kahrizi MS, Adili A, Ghoreishizadeh S, et al. A comprehensive review about the utilization of immune checkpoint inhibitors and combination therapy in hepatocellular carcinoma: an updated review. Cancer Cell Int. (2022) 22:269. doi: 10.1186/s12935-022-02682-z
3. Meng L, Wu H, Wu J, Ding P, He J, Sang M, et al. Mechanisms of immune checkpoint inhibitors: insights into the regulation of circular RNAS involved in cancer hallmarks. Cell Death Dis. (2024) 15:3. doi: 10.1038/s41419-023-06389-5
4. Hodi FS, O’Day SJ, McDermott DF, Weber RW, Sosman JA, Haanen JB, et al. Improved survival with ipilimumab in patients with metastatic melanoma. N Engl J Med. (2010) 363:711–23. doi: 10.1056/NEJMoa1003466
5. McDermott DF, Drake CG, Sznol M, Choueiri TK, Powderly JD, Smith DC, et al. Survival, durable response, and long-term safety in patients with previously treated advanced renal cell carcinoma receiving nivolumab. J Clin Oncol. (2015) 33:2013–20. doi: 10.1200/JCO.2014.58.1041
6. Ribas A, Puzanov I, Dummer R, SChadendorf D, Hamid O, Robert C, et al. Pembrolizumab versus investigator-choice chemotherapy for ipilimumab-refractory melanoma (KEYNOTE-002): a randomised, controlled, phase 2 trial. Lancet Oncol. (2015) 16:908–18. doi: 10.1016/S1470-2045(15)00083-2
7. SChadendorf D, Hodi FS, Robert C, Weber JS, Margolin K, Hamid O, et al. Pooled analysis of long-term survival data from phase II and phase III trials of ipilimumab in unresectable or metastatic melanoma. J Clin Oncol. (2015) 33:1889–94. doi: 10.1200/JCO.2014.56.2736
8. Topalian SL, Hodi FS, Brahmer JR, Gettinger SN, Smith DC, McDermott DF, et al. Safety, activity, and immune correlates of anti-PD-1 antibody in cancer. N Engl J Med. (2012) 366:2443–54. doi: 10.1056/NEJMoa1200690
9. Doroshow DB, Bhalla S, Beasley MB, Sholl LM, Kerr KM, Gnjatic S, et al. PD-L1 as a biomarker of response to immune-checkpoint inhibitors. Nat Rev Clin Oncol. (2021) 18:345–62. doi: 10.1038/s41571-021-00473-5
10. Wang DR, Wu XL, Sun YL. Therapeutic targets and biomarkers of tumor immunotherapy: response versus non-response. Signal Transduct Target Ther. (2022) 7:331. doi: 10.1038/s41392-022-01136-2
11. Syn NL, Teng M, Mok T, Soo RA. De-novo and acquired resistance to immune checkpoint targeting. Lancet Oncol. (2017) 18:e731–731e741. doi: 10.1016/S1470-2045(17)30607-1
12. Armand P, Engert A, Younes A, Fanale M, Santoro A, Zinzani PL, et al. Nivolumab for relapsed/refractory classic hodgkin lymphoma after failure of autologous hematopoietic cell transplantation: extended follow-up of the multicohort single-arm phase II checkMate 205 trial. J Clin Oncol. (2018) 36:1428–39. doi: 10.1200/JCO.2017.76.0793
13. Younes A, Santoro A, Shipp M, Zinzani PL, Timmerman JM, Ansell S, et al. Nivolumab for classical Hodgkin’s lymphoma after failure of both autologous stem-cell transplantation and brentuximab vedotin: a multicentre, multicohort, single-arm phase 2 trial. Lancet Oncol. (2016) 17:1283–94. doi: 10.1016/S1470-2045(16)30167-X
14. Bobillo S, Nieto JC, Barba P. Use of checkpoint inhibitors in patients with lymphoid Malignancies receiving allogeneic cell transplantation: a review. Bone Marrow Transplant. (2021) 56:1784–93. doi: 10.1038/s41409-021-01268-z
15. Cieri N, Maurer K, Wu CJ. 60 years young: the evolving role of allogeneic hematopoietic stem cell transplantation in cancer immunotherapy. Cancer Res. (2021) 81:4373–84. doi: 10.1158/0008-5472.CAN-21-0301
16. Merryman RW, Kim HT, Zinzani PL, Carlo-Stella C, Ansell SM, Perales MA, et al. Safety and efficacy of allogeneic hematopoietic stem cell transplant after PD-1 blockade in relapsed/refractory lymphoma. Blood. (2017) 129:1380–8. doi: 10.1182/blood-2016-09-738385
17. Schoch LK, Cooke KR, Wagner-Johnston ND, Gojo I, Swinnen LJ, Imus P, et al. Immune checkpoint inhibitors as a bridge to allogeneic transplantation with posttransplant cyclophosphamide. Blood Adv. (2018) 2:2226–9. doi: 10.1182/bloodadvances.2018019208
18. Blazar BR, Carreno BM, Panoskaltsis-Mortari A, Carter L, Iwai Y, Yagita H, et al. Blockade of programmed death-1 engagement accelerates graft-versus-host disease lethality by an IFN-gamma-dependent mechanism. J Immunol. (2003) 171:1272–7. doi: 10.4049/jimmunol.171.3.1272
19. Williams P, Basu S, Garcia-Manero G, Hourigan CS, Oetjen KA, Cortes JE, et al. The distribution of T-cell subsets and the expression of immune checkpoint receptors and ligands in patients with newly diagnosed and relapsed acute myeloid leukemia. Cancer. (2019) 125:1470–81. doi: 10.1002/cncr.31896
20. Zhou Q, Munger ME, Veenstra RG, Weigel BJ, Hirashima M, Munn DH, et al. Coexpression of Tim-3 and PD-1 identifies a CD8+ T-cell exhaustion phenotype in mice with disseminated acute myelogenous leukemia. Blood. (2011) 117:4501–10. doi: 10.1182/blood-2010-10-310425
21. Bardhan K, Anagnostou T, Boussiotis VA. The PD1:PD-L1/2 pathway from discovery to clinical implementation. Front Immunol. (2016) 7:550. doi: 10.3389/fimmu.2016.00550
22. Liu J, Hamrouni A, Wolowiec D, Coiteux V, Kuliczkowski K, Hetuin D, et al. Plasma cells from multiple myeloma patients express B7-H1 (PD-L1) and increase expression after stimulation with IFN-{gamma} and TLR ligands via a MyD88-, TRAF6-, and MEK-dependent pathway. Blood. (2007) 110:296–304. doi: 10.1182/blood-2006-10-051482
23. Munir F, Hardit V, Sheikh IN, AlQahtani S, He J, Cuglievan B, et al. Classical hodgkin lymphoma: from past to future-A comprehensive review of pathophysiology and therapeutic advances. Int J Mol Sci. (2023) 24:10095. doi: 10.3390/ijms241210095
24. Zhang L, Gajewski TF, Kline J. PD-1/PD-L1 interactions inhibit antitumor immune responses in a murine acute myeloid leukemia model. Blood. (2009) 114:1545–52. doi: 10.1182/blood-2009-03-206672
25. Davids MS, Kim HT, Bachireddy P, Costello C, Liguori R, Savell A, et al. Ipilimumab for patients with relapse after allogeneic transplantation. N Engl J Med. (2016) 375:143–53. doi: 10.1056/NEJMoa1601202
26. Chen C, Liang C, Wang S, Chio CL, Zhang Y, Zeng C, et al. Expression patterns of immune checkpoints in acute myeloid leukemia. J Hematol Oncol. (2020) 13:28. doi: 10.1186/s13045-020-00853-x
27. Qureshi OS, Zheng Y, Nakamura K, Attridge K, Manzotti C, Schmidt EM, et al. Trans-endocytosis of CD80 and CD86: a molecular basis for the cell-extrinsic function of CTLA-4. Science. (2011) 332:600–3. doi: 10.1126/science.1202947
28. Boasso A, Herbeuval JP, Hardy AW, Winkler C, Shearer GM. Regulation of indoleamine 2,3-dioxygenase and tryptophanyl-tRNA-synthetase by CTLA-4-Fc in human CD4+ T cells. Blood. (2005) 105:1574–81. doi: 10.1182/blood-2004-06-2089
29. Grohmann U, Orabona C, Fallarino F, Vacca C, Calcinaro F, Falorni A, et al. CTLA-4-Ig regulates tryptophan catabolism. vivo. Nat Immunol. (2002) 3:1097–101. doi: 10.1038/ni846
30. Saudemont A, Quesnel B. In a model of tumor dormancy, long-term persistent leukemic cells have increased B7-H1 and B7.1 expression and resist CTL-mediated lysis. Blood. (2004) 104:2124–33. doi: 10.1182/blood-2004-01-0064
31. Patel SS, Weirather JL, Lipschitz M, Lako A, Chen PH, Griffin GK, et al. The microenvironmental niche in classic Hodgkin lymphoma is enriched for CTLA-4-positive T cells that are PD-1-negative. Blood. (2019) 134:2059–69. doi: 10.1182/blood.2019002206
32. Cai L, Li Y, Tan J, Xu L, Li Y. Targeting LAG-3, TIM-3, and TIGIT for cancer immunotherapy. J Hematol Oncol. (2023) 16:101. doi: 10.1186/s13045-023-01499-1
33. Aoki T, Chong LC, Takata K, Milne K, Hav M, Colombo A, et al. Single-cell transcriptome analysis reveals disease-defining T-cell subsets in the tumor microenvironment of classic hodgkin lymphoma. Cancer Discovery. (2020) 10:406–21. doi: 10.1158/2159-8290.CD-19-0680
34. Kikushige Y, Shima T, Takayanagi S, Urata S, Miyamoto T, Iwasaki H, et al. TIM-3 is a promising target to selectively kill acute myeloid leukemia stem cells. Cell Stem Cell. (2010) 7:708–17. doi: 10.1016/j.stem.2010.11.014
35. Tan J, Yu Z, Huang J, Chen Y, Huang S, Yao D, et al. Increased PD-1+Tim-3+ exhausted T cells in bone marrow may influence the clinical outcome of patients with AML. biomark Res. (2020) 8:6. doi: 10.1186/s40364-020-0185-8
36. Schwartz S, Patel N, Longmire T, Jayaraman P, Jiang X, Lu H, et al. Characterization of sabatolimab, a novel immunotherapy with immuno-myeloid activity directed against TIM-3 receptor. Immunother Adv. (2022) 2:ltac019. doi: 10.1093/immadv/ltac019
37. Ansell SM, Bröckelmann PJ, von Keudell G, Lee HJ, Santoro A, Zinzani PL, et al. Nivolumab for relapsed/refractory classical Hodgkin lymphoma: 5-year survival from the pivotal phase 2 CheckMate 205 study. Blood Adv. (2023) 7:6266–74. doi: 10.1182/bloodadvances.2023010334
38. Chen R, Zinzani PL, Lee HJ, Armand P, Johnson NA, Brice P, et al. Pembrolizumab in relapsed or refractory Hodgkin lymphoma: 2-year follow-up of KEYNOTE-087. Blood. (2019) 134:1144–53. doi: 10.1182/blood.2019000324
39. Kuruvilla J, Armand P, Hamadani M, Kline J, Moskowitz CH, Avigan D, et al. Pembrolizumab for patients with non-Hodgkin lymphoma: phase 1b KEYNOTE-013 study. Leuk Lymphoma. (2023) 64:130–9. doi: 10.1080/10428194.2022.2136956
40. Sureda A, Genadieva Stavrik S, Boumendil A, Finel H, Khvedelidze I, Dietricht S, et al. Changes in patients population and characteristics of hematopoietic stem cell transplantation for relapsed/refractory Hodgkin lymphoma: an analysis of the Lymphoma Working Party of the EBMT. Bone Marrow Transplant. (2020) 55:2170–9. doi: 10.1038/s41409-020-0929-y
41. Casadei B, Broccoli A, Stefoni V, Pellegrini C, Marangon M, Morigi A, et al. PD-1 blockade as bridge to allogeneic stem cell transplantation in relapsed/refractory Hodgkin lymphoma patients: a retrospective single center case series. Haematologica. (2019) 104:e521–521e522. doi: 10.3324/haematol.2019.215962
42. Zinzani PL, Ribrag V, Moskowitz CH, Michot JM, Kuruvilla J, Balakumaran A, et al. Safety and tolerability of pembrolizumab in patients with relapsed/refractory primary mediastinal large B-cell lymphoma. Blood. (2017) 130:267–70. doi: 10.1182/blood-2016-12-758383
43. Zinzani PL, Thieblemont C, Melnichenko V, Bouabdallah K, Walewski J, Majlis A, et al. Pembrolizumab in relapsed or refractory primary mediastinal large B-cell lymphoma: final analysis of KEYNOTE-170. Blood. (2023) 142:141–5. doi: 10.1182/blood.2022019340
44. Carreau NA, Armand P, Merryman RW, Advani RH, Spinner MA, Herrera AF, et al. Checkpoint blockade treatment sensitises relapsed/refractory non-Hodgkin lymphoma to subsequent therapy. Br J Haematol. (2020) 191:44–51. doi: 10.1111/bjh.16756
45. Zinzani PL, Santoro A, Gritti G, Brice P, Barr PM, Kuruvilla J, et al. Nivolumab combined with brentuximab vedotin for R/R primary mediastinal large B-cell lymphoma: a 3-year follow-up. Blood Adv. (2023) 7:5272–80. doi: 10.1182/bloodadvances.2023010254
46. Restelli C, Ruella M, Paruzzo L, Tarella C, Pelicci PG, Colombo E. Recent advances in immune-based therapies for acute myeloid leukemia. Blood Cancer Discovery. (2024) 5:234–48. doi: 10.1158/2643-3230.BCD-23-0202
47. Gao XN, Su YF, Li MY, Jing Y, Wang J, Xu L, et al. Single-center phase 2 study of PD-1 inhibitor combined with DNA hypomethylation agent + CAG regimen in patients with relapsed/refractory acute myeloid leukemia. Cancer Immunol Immunother. (2023) 72:2769–82. doi: 10.1007/s00262-023-03454-y
48. Wang YX, Wang A, Su YF, Wang J, Li YH, Li F, et al. Anti-PD-1 combined with hypomethylating agent and CAG regimen bridging to allogeneic hematopoietic stem cell transplantation: a novel strategy for relapsed/refractory acute myeloid leukemia. Front Immunol. (2024) 15:1409302. doi: 10.3389/fimmu.2024.1409302
49. İskender D, Çakar MK, Dal MS, Baysal NA, Merdin A, Bakırtas M, et al. Nivolumab as a bridge to allogeneic hematopoietic stem cell transplantation is associated with improved survival. Eur Rev Med Pharmacol Sci. (2022) 26:957–65. doi: 10.26355/eurrev_202202_28005
50. Merryman RW, Castagna L, Giordano L, Ho VT, Corradini P, Guidetti A, et al. Allogeneic transplantation after PD-1 blockade for classic Hodgkin lymphoma. Leukemia. (2021) 35:2672–83. doi: 10.1038/s41375-021-01193-6
51. Tschernia NP, Kumar V, Moore DT, Vincent BG, Coombs CC, Van Deventer H, et al. Safety and efficacy of pembrolizumab prior to allogeneic stem cell transplantation for acute myelogenous leukemia. Transplant Cell Ther. (2021) 27:1021.e1–1021.e5. doi: 10.1016/j.jtct.2021.08.022
52. Oran B, Garcia-Manero G, Saliba RM, Alfayez M, Al-Atrash G, Ciurea SO, et al. Posttransplantation cyclophosphamide improves transplantation outcomes in patients with AML/MDS who are treated with checkpoint inhibitors. Cancer. (2020) 126:2193–205. doi: 10.1002/cncr.32796
53. Ito A, Kim SW, Matsuoka KI, Kawakita T, Tanaka T, Inamoto Y, et al. Safety and efficacy of anti-programmed cell death-1 monoclonal antibodies before and after allogeneic hematopoietic cell transplantation for relapsed or refractory Hodgkin lymphoma: a multicenter retrospective study. Int J Hematol. (2020) 112:674–89. doi: 10.1007/s12185-020-02960-4
54. Covut F, Pinto R, Cooper BW, Tomlinson B, Metheny L, Malek E, et al. Nivolumab before and after allogeneic hematopoietic cell transplantation. Bone Marrow Transplant. (2017) 52:1054–6. doi: 10.1038/bmt.2017.44
55. El Cheikh J, Massoud R, Abudalle I, Haffar B, Mahfouz R, Kharfan-Dabaja MA, et al. Nivolumab salvage therapy before or after allogeneic stem cell transplantation in Hodgkin lymphoma. Bone Marrow Transplant. (2017) 52:1074–7. doi: 10.1038/bmt.2017.69
56. Martínez C, Carpio C, Heras I, Ríos-Herranz E, Buch J, Gutierrez A, et al. Potential survival benefit for patients receiving allogeneic hematopoietic stem cell transplantation after nivolumab therapy for relapse/refractory hodgkin lymphoma: real-life experience in Spain. Biol Blood Marrow Transplant. (2020) 26:1534–42. doi: 10.1016/j.bbmt.2020.02.003
57. Nieto JC, Roldán E, Jiménez I, Fox L, Carabia J, Ortí G, et al. Posttransplant cyclophosphamide after allogeneic hematopoietic cell transplantation mitigates the immune activation induced by previous nivolumab therapy. Leukemia. (2020) 34:3420–5. doi: 10.1038/s41375-020-0851-8
58. De Philippis C, Legrand-Izadifar F, Bramanti S, Giordano L, Montes de Oca C, Duléry R, et al. Checkpoint inhibition before haploidentical transplantation with posttransplant cyclophosphamide in Hodgkin lymphoma. Blood Adv. (2020) 4:1242–9. doi: 10.1182/bloodadvances.2019001336
59. Kim YE, Kim H, Shin J, Min SY, Kang SH, Suh JK, et al. Stage IV natural killer/T-cell lymphoma with chronic active Epstein-Barr virus, treated with pembrolizumab and TCRαβ-depleted haploidentical hematopoietic stem cell transplantation. Leuk Lymphoma. (2020) 61:2250–3. doi: 10.1080/10428194.2020.1757666
60. Handelsman S, Overbey J, Chen K, Lee J, Haj D, Li Y. PD-L1’s role in preventing alloreactive T cell responses following hematopoietic and organ transplant. Cells. (2023) 12:1609. doi: 10.3390/cells12121609
61. Ikegawa S, Meguri Y, Kondo T, Sugiura H, Sando Y, Nakamura M, et al. PTCy ameliorates GVHD by restoring regulatory and effector T-cell homeostasis in recipients with PD-1 blockade. Blood Adv. (2019) 3:4081–94. doi: 10.1182/bloodadvances.2019000134
62. Kanakry CG, Ganguly S, Zahurak M, Bolaños-Meade J, Thoburn C, Perkins B, et al. Aldehyde dehydrogenase expression drives human regulatory T cell resistance to posttransplantation cyclophosphamide. Sci Transl Med. (2013) 5:211ra157. doi: 10.1126/scitranslmed.3006960
63. Köhler N, Ruess DA, Kesselring R, Zeiser R. The role of immune checkpoint molecules for relapse after allogeneic hematopoietic cell transplantation. Front Immunol. (2021) 12:634435. doi: 10.3389/fimmu.2021.634435
64. Kolb HJ. Graft-versus-leukemia effects of transplantation and donor lymphocytes. Blood. (2008) 112:4371–83. doi: 10.1182/blood-2008-03-077974
65. Merryman RW, Armand P. Immune checkpoint blockade and hematopoietic stem cell transplant. Curr Hematol Malig Rep. (2017) 12:44–50. doi: 10.1007/s11899-017-0362-5
66. Norde WJ, Maas F, Hobo W, Korman A, Quigley M, Kester MG, et al. PD-1/PD-L1 interactions contribute to functional T-cell impairment in patients who relapse with cancer after allogeneic stem cell transplantation. Cancer Res. (2011) 71:5111–22. doi: 10.1158/0008-5472.CAN-11-0108
67. Atanackovic D, Luetkens T. Biomarkers for checkpoint inhibition in hematologic Malignancies. Semin Cancer Biol. (2018) 52:198–206. doi: 10.1016/j.semcancer.2018.05.005
68. Hashimoto D. Guest editorial: prophylaxis and treatment of relapse after allogeneic hematopoietic stem cell transplantation. Int J Hematol. (2022) 116:307–8. doi: 10.1007/s12185-022-03407-8
69. Godfrey J, Bishop MR, Syed S, Hyjek E, Kline J. PD-1 blockade induces remissions in relapsed classical Hodgkin lymphoma following allogeneic hematopoietic stem cell transplantation. J Immunother Cancer. (2017) 5:11. doi: 10.1186/s40425-017-0211-z
70. Holderried T, Fraccaroli A, Schumacher M, Heine A, Brossart P, Stelljes M, et al. The role of checkpoint blockade after allogeneic stem cell transplantation in diseases other than Hodgkin’s Lymphoma. Bone Marrow Transplant. (2019) 54:1662–7. doi: 10.1038/s41409-019-0498-0
71. Yared JA, Hardy N, Singh Z, Hajj S, Badros AZ, Kocoglu M, et al. Major clinical response to nivolumab in relapsed/refractory Hodgkin lymphoma after allogeneic stem cell transplantation. Bone Marrow Transplant. (2016) 51:850–2. doi: 10.1038/bmt.2015.346
72. Singh AK, Porrata LF, Aljitawi O, Lin T, Shune L, Ganguly S, et al. Fatal GvHD induced by PD-1 inhibitor pembrolizumab in a patient with Hodgkin’s lymphoma. Bone Marrow Transplant. (2016) 51:1268–70. doi: 10.1038/bmt.2016.111
73. Angenendt L, Schliemann C, Lutz M, Rebber E, Schulze AB, Weckesser M, et al. Nivolumab in a patient with refractory Hodgkin’s lymphoma after allogeneic stem cell transplantation. Bone Marrow Transplant. (2016) 51:443–5. doi: 10.1038/bmt.2015.266
74. McDuffee E, Aue G, Cook L, Ramos-Delgado C, Shalabi R, Worthy T, et al. Tumor regression concomitant with steroid-refractory GvHD highlights the pitfalls of PD-1 blockade following allogeneic hematopoietic stem cell transplantation. Bone Marrow Transplant. (2017) 52:759–61. doi: 10.1038/bmt.2016.346
75. Haverkos BM, Abbott D, Hamadani M, Armand P, Flowers ME, Merryman R, et al. PD-1 blockade for relapsed lymphoma post-allogeneic hematopoietic cell transplant: high response rate but frequent GVHD. Blood. (2017) 130:221–8. doi: 10.1182/blood-2017-01-761346
76. Herbaux C, Gauthier J, Brice P, Drumez E, Ysebaert L, Doyen H, et al. Efficacy and tolerability of nivolumab after allogeneic transplantation for relapsed Hodgkin lymphoma. Blood. (2017) 129:2471–8. doi: 10.1182/blood-2016-11-749556
77. Schoch L, Borrello I, Fuchs E, Bolanos-Meade J, Huo J, Gojo I, et al. Checkpoint inhibitor therapy and graft versus host disease in allogeneic bone marrow transplant recipients of haploidentical and matched products with post-transplant cyclophosphamide. Blood. (2016) 128:4571–1. doi: 10.1182/BLOOD.V128.22.4571.4571
78. Giannotti F, De Ramon Ortiz C, Simonetta F, Morin S, Bernardi C, Masouridi-Levrat S, et al. Remission of relapsed/refractory classical Hodgkin lymphoma induced by brentuximab vedotin and pembrolizumab combination after allogeneic hematopoietic stem cell transplantation: a case report. Front Immunol. (2024) 15:1360275. doi: 10.3389/fimmu.2024.1360275
79. Manson G, Herbaux C, Schiano JM, Casasnovas O, Stamatoullas A, Deau B, et al. Can nivolumab alone cure patients with relapse or refractory Hodgkin lymphoma? A 5-year analysis of the French early access program (EPA). Br J Haematol. (2022) 198:203–6. doi: 10.1111/bjh.18198
80. Bashey A, Medina B, Corringham S, Pasek M, Carrier E, Vrooman L, et al. CTLA4 blockade with ipilimumab to treat relapse of Malignancy after allogeneic hematopoietic cell transplantation. Blood. (2009) 113:1581–8. doi: 10.1182/blood-2008-07-168468
81. Khouri IF, Fernandez Curbelo I, Turturro F, Jabbour EJ, Milton DR, Bassett RL Jr, et al. Ipilimumab plus Lenalidomide after Allogeneic and Autologous Stem Cell Transplantation for Patients with Lymphoid Malignancies. Clin Cancer Res. (2018) 24:1011–8. doi: 10.1158/1078-0432.CCR-17-2777
82. Thol F, Döhner H, Ganser A. How I treat refractory and relapsed acute myeloid leukemia. Blood. (2024) 143:11–20. doi: 10.1182/blood.2023022481
83. Toffalori C, Zito L, Gambacorta V, Riba M, Oliveira G, Bucci G, et al. Immune signature drives leukemia escape and relapse after hematopoietic cell transplantation. Nat Med. (2019) 25:603–11. doi: 10.1038/s41591-019-0400-z
84. Noviello M, Manfredi F, Ruggiero E, Perini T, Oliveira G, Cortesi F, et al. Bone marrow central memory and memory stem T-cell exhaustion in AML patients relapsing after HSCT. Nat Commun. (2019) 10:1065. doi: 10.1038/s41467-019-08871-1
85. Vadakekolathu J, Rutella S. Escape from T-cell-targeting immunotherapies in acute myeloid leukemia. Blood. (2024) 143:2689–700. doi: 10.1182/blood.2023019961
86. Godfrey J, Liu H, Yu J, Tallarico M, Curran E, Artz A, et al. Pembrolizumab for the treatment of disease relapse after allogeneic hematopoietic stem cell transplantation. Blood Adv. (2023) 7:963–70. doi: 10.1182/bloodadvances.2022008403
87. Garcia JS, Flamand Y, Penter L, Keng M, Tomlinson BK, Mendez LM, et al. Ipilimumab plus decitabine for patients with MDS or AML in posttransplant or transplant-naïve settings. Blood. (2023) 141:1884–8. doi: 10.1182/blood.2022017686
88. Penter L, Liu Y, Yang L, Taing L, Jhaveri A, Southard J, et al. Mechanisms of response and resistance to combination decitabine and ipilimumab for transplant nave and post-transplant AML/MDS. Blood. (2022) 140(Supplement 1):10198–9. doi: 10.1182/blood-2022-157339
89. Penter L, Zhang Y, Savell A, Huang T, Wu CJ. Molecular and cellular features of CTLA-4 blockade for relapsed myeloid Malignancies after transplantation. Blood. (2021) 137:3212–7. doi: 10.1182/blood.2021010867
90. Davids MS, Kim HT, Costello C, Herrera AF, Locke FL, Maegawa RO, et al. A multicenter phase 1 study of nivolumab for relapsed hematologic Malignancies after allogeneic transplantation. Blood. (2020) 135:2182–91. doi: 10.1182/blood.2019004710
91. Wang AY, Kline J, Stock W, Kosuri S, Artz A, Larson RA, et al. Unexpected toxicities when nivolumab was given as maintenance therapy following allogeneic stem cell transplantation. Biol Blood Marrow Transplant. (2020) 26:1025–7. doi: 10.1016/j.bbmt.2020.01.021
92. Apostolova P, Kreutmair S, Toffalori C, Punta M, Unger S, Burk AC, et al. Phase II trial of hypomethylating agent combined with nivolumab for acute myeloid leukaemia relapse after allogeneic haematopoietic cell transplantation-Immune signature correlates with response. Br J Haematol. (2023) 203:264–81. doi: 10.1111/bjh.19007
93. Saberian C, Abdel-Wahab N, Abudayyeh A, Rafei H, Joseph J, Rondon G, et al. Post-transplantation cyclophosphamide reduces the incidence of acute graft-versus-host disease in patients with acute myeloid leukemia/myelodysplastic syndromes who receive immune checkpoint inhibitors after allogeneic hematopoietic stem cell transplantation. J Immunother Cancer. (2021) 9:e001818. doi: 10.1136/jitc-2020-001818
94. Mayumi H, Umesue M, Nomoto K. Cyclophosphamide-induced immunological tolerance: an overview. Immunobiology. (1996) 195:129–39. doi: 10.1016/S0171-2985(96)80033-7
95. Luznik L, O’Donnell PV, Fuchs EJ. Post-transplantation cyclophosphamide for tolerance induction in HLA-haploidentical bone marrow transplantation. Semin Oncol. (2012) 39:683–93. doi: 10.1053/j.seminoncol.2012.09.005
96. Cieri N, Peccatori J, Oliveira G, Greco R, Marktel S, Lunghi F, et al. Tracking T cell dynamics in the first month after haplo-HSCT with post-transplant cyclophosphamide reveals a predominant contribution of memory stem T cells to the early phase of immune reconstitution. Blood. (2013) 122:4615. doi: 10.1182/blood.V122.21.4615.4615
97. Tang Y, Zhou Z, Yan H, You Y. Case report: preemptive treatment with low-dose PD-1 blockade and azacitidine for molecular relapsed acute myeloid leukemia with RUNX1-RUNX1T1 after allogeneic hematopoietic stem cell transplantation. Front Immunol. (2022) 13:810284. doi: 10.3389/fimmu.2022.810284
98. Tameni A, Toffalori C, Vago L. Tricking the trickster: precision medicine approaches to counteract leukemia immune escape after transplant. Blood. (2024) 143:2710–21. doi: 10.1182/blood.2023019962
99. Saha A, Aoyama K, Taylor PA, Koehn BH, Veenstra RG, Panoskaltsis-Mortari A, et al. Host programmed death ligand 1 is dominant over programmed death ligand 2 expression in regulating graft-versus-host disease lethality. Blood. (2013) 122:3062–73. doi: 10.1182/blood-2013-05-500801
100. Hossain MS, Kunter GM, El-Najjar VF, Jaye DL, Al-Kadhimi Z, Taofeek OK, et al. PD-1 and CTLA-4 up regulation on donor T cells is insufficient to prevent GvHD in allo-HSCT recipients. PloS One. (2017) 12:e0184254. doi: 10.1371/journal.pone.0184254
101. Roshandel E, Tavakoli F, Parkhideh S, Akhlaghi SS, Ardakani MT, Soleimani M. Post-hematopoietic stem cell transplantation relapse: Role of checkpoint inhibitors. Health Sci Rep. (2022) 5:e536. doi: 10.1002/hsr2.536
Keywords: immune checkpoint inhibitors, programmed cell death protein 1, programmed cell death 1 ligand 1, allogeneic hematopoietic cell transplant, graft-versus-host disease, immune-related adverse events
Citation: Hu Y, Wang Y, Min K, Zhou H and Gao X (2024) The influence of immune checkpoint blockade on the outcomes of allogeneic hematopoietic stem cell transplantation. Front. Immunol. 15:1491330. doi: 10.3389/fimmu.2024.1491330
Received: 04 September 2024; Accepted: 04 November 2024;
Published: 20 November 2024.
Edited by:
Sina Naserian, Hôpital Paul Brousse, FranceReviewed by:
Lotte Van Der Wagen, University Medical Center Utrecht, NetherlandsStephen P. Persaud, Washington University in St. Louis, United States
Copyright © 2024 Hu, Wang, Min, Zhou and Gao. This is an open-access article distributed under the terms of the Creative Commons Attribution License (CC BY). The use, distribution or reproduction in other forums is permitted, provided the original author(s) and the copyright owner(s) are credited and that the original publication in this journal is cited, in accordance with accepted academic practice. No use, distribution or reproduction is permitted which does not comply with these terms.
*Correspondence: Xiaoning Gao, Z2FveG5AMjYzLm5ldA==
†These authors have contributed equally to this work and share first authorship