- 1Centre of Experimental Medicine and Surgery, Institute of Medical Sciences, Banaras Hindu University, Varanasi, India
- 2Department of Medicine, Institute of Medical Sciences, Banaras Hindu University, Varanasi, India
- 3Department of Microbiology Tumor and Cell Biology, Karolinska Institutet, Stockholm, Sweden
- 4Infection and Inflammation Division, QIMR Berghofer Medical Research Institute, Brisbane, QLD, Australia
Understanding the development and maintenance of immunological memory is important for efforts to eliminate parasitic diseases like leishmaniasis. Leishmaniasis encompasses a range of pathologies, resulting from infection with protozoan parasites belonging to the subgenera Leishmania and Viannia of the genus Leishmania. A striking feature of these infections is that natural or drug-mediated cure of infection generally confers life-long protection against disease. The generation of protective T cell responses are necessary to control Leishmania infections. CD4+ T helper (Th) cells orchestrate immune responses in leishmaniasis and IFNγ+ Tbet+ CD4+ T (Th1) cells are required for the activation of phagocytes to kill captured or resident parasites, while other Th cell subset, including FoxP3+ natural regulatory T cells and Th2 cells can promote disease progression by suppressing the activities of Th1 cells. Upon resolution of a primary Leishmania infection, different subsets of CD4+ T cells, including tissue-resident memory T cells, effector memory T cells, central memory T cells, and short-lived effector T cells, help to confer resistance against reinfection. To maintain long-term protective Leishmania-specific CD4+ T cells responses, it is believed that persistent parasites or re-exposure to parasites at regular intervals is required (concomitant immunity). Despite the advances in our understanding about the immune responses during leishmaniasis, the generation of long-lasting protective immunity via vaccination has yet to be achieved. In this review, we summarize our current understanding about the formation and maintenance of immunological memory and control of leishmaniasis at the individual and population level. We will focus on Indian visceral leishmaniasis and discuss T cell responses that contribute to susceptibility to leishmaniasis, parasite persistence in populations and the environment, as well as describing advances in the development of leishmaniasis vaccines aimed at inducing protective CD4+ T cell responses.
Introduction
Leishmaniasis represents a diverse array of clinical manifestations resulting from infection with intracellular protozoan parasites of the subgenera Leishmania and Viannia of genus Leishmania. Parasites are transmitted to humans through the bite of infected sand fly vectors belonging to the genus Phlebotomus in the Old World (Africa, Asia, and Southern Europe) and the genus Lutzomia in the New World (The Americas). Disease symptoms range from relatively benign lesions in cutaneous leishmaniasis (CL), to mucosal membrane destruction in mucocutaneous leishmaniasis (MCL), to lethal infection of visceral organs in visceral leishmaniasis (VL), also known as Kala-azar in the Indian sub-continent. The different disease pathologies depend primarily on the parasite species involved, but also on the type of host to immune response generated following infection (1). With an extremely varied epidemiology, leishmaniasis is endemic in 99 nations, and 9 of these are endemic for VL alone, 9 for CL, and 71 for both VL and CL (https://www.who.int/publications/i/item/who-wer9840-471-487). CL, the most prevalent form of the disease, presents as skin lesions, predominantly ulcers, typically on exposed areas of the body. These lesions can lead to significant disabilities and may result in lifelong scarring (1, 2). Approximately 95% of CL cases are reported in the Americas, the Mediterranean basin, the Middle East, and central Asia. It is estimated that between 600,000 to 1 million new cases of CL are reported worldwide annually. VL is the most severe form of leishmaniasis and is caused by Leishmania (Leishmania) donovani (in Asia), Leishmania (Leishmania) infantum (South America and around the Mediterranean basin) and Leishmania donovani complex (in Africa) (3). VL continues to pose a substantial public health challenge in numerous countries, with the majority of reported cases originating from Brazil, east Africa, and India, particularly affecting socioeconomically disadvantaged people With an estimated annual disease burden of 50,000 to 90,000 cases, the World Health Organization (WHO) considers VL as a disease of outbreak potential, (Leishmaniasis, 12 January, 2023, https://www.who.int/news-room/fact-sheets/detail/leishmaniasis). In VL patients, visceral organs are parasitized and clinical manifestations are systemic, including long-term fever, enlargement of the spleen and liver, weight loss, progressive anemia, pancytopenia and elevated antibody titers (4). Lymphadenopathy and skin darkening can also be observed if the disease is allowed to progress. Comorbidities in VL patients can include concomitant bacterial and HIV infections, which can affect clinical presentations and impair the accuracy of early diagnostic tests (5, 6). These are important because failed diagnosis can have severe consequences as fatality rate is over 95% unless VL is treated (4, 7). It is important to understand that Leishmania infections do not always result in disease and that the majority of infected individuals remain asymptomatic with no clinical signs (5). Factors influencing these different outcomes of infection include host genetics (6), nutritional status (5), and co-infections (8, 9), which affect host immune responses.
The reservoir host for the Leishmania parasite exhibits regional variation, and both anthroponotic and zoonotic transmission of leishmaniasis can occur. Zoonotic transmission is considered to dominate in Mediterranean regions and South American endemic foci, while VL is predominantly anthroponotic in India and Bangladesh (10–12). Infection begins when a carrier sand fly lacerates a blood vessel and feeds on the pool of blood released. During this process, the fly releases a mixture of salivary factors with anticoagulant, vasodilatory, and complement inhibitory activities. As the sand fly feeds, it regurgitates and transmits the infectious motile metacyclic promastigotes into the mammalian host (13). Inside the host, promastigotes convert into non-motile amastigotes, that take up permanent residence in macrophages of tissues, which in case of VL is the spleen, liver and bone marrow (14). VL presents as complex immunological responses involving both innate and adaptive responses. Experimental infections have demonstrated that the first immune cells to respond to the insult of an infectious bite are infiltrating neutrophils followed by monocytes (15–17). These cells can kill the parasites if activated but can also act as host cells allowing the establishment of disease. Macrophages, and in particular dendritic cells (DC), are involved in activating the adaptive immune response and shaping it through the cytokines secreted when these antigen presenting cells (APC) are in contact with T cells. These interactions shape the T cell immune response and determine disease outcome.
Concomitant immunity provided by memory T cells is thought to develop following primary infection with Leishmania parasites, and complete parasite elimination can impair protection against reinfection (18, 19). Memory T cells can develop at different time following infection and have varying life spans and functions (20). Defining the processes underlying the generation of effective immune memory responses is needed to help design vaccine approaches that produce long-lasting cell-mediated immunity. However, despite advances in our understanding of these processes, significant gaps remain in our understanding of how memory T cells are generated, and in particular, how to generate these responses by vaccination.
T cell memory response
Immunological memory is a feature of the adaptive immune system and includes the development of long-lived memory T cell and B cells (along with long lived plasma cells) and their different subsets, either located in secondary lymphoid organs (SLOs) or in peripheral tissue (21). As the primary immune response contracts, only around 5-10% of antigen-specific T cells survive and become memory T cells. These cells have less rigorous requirements for re-activation and can respond to suboptimal T cell receptor (TCR) and co-stimulatory signaling with greater proliferative and effector capacity, as well as tissue homing capabilities, compared with naïve T cells (reviewed in (22). Commitment of antigen-specific T cells to effector or memory lineages is determined by priming conditions, such as the duration and strength of antigenic exposer or stimulation, co-stimulatory signals, and local cytokine production (23–25). Memory T cells were first identified as CD45RO+and CD45RA- in humans (26) and were further divided into central memory cells (Tcm) and effector memory cells (Tem) on the basis of CCR7 and CD62L expression in mice and humans, which are constitutively expressed on naïve T cells enabling their localization in secondary lymphoid organs (SLO). Tcm are highly proliferative, interleukin (IL)-2 secreting CD45RA-CCR7+CD62L+ cells present in SLO, whereas Tem is efficient effector cytokine producing CD45RA-CCR7-CD62L- cells present in peripheral tissue and recirculate via the blood. A more recently described memory subset is tissue resident memory T (Trm) cell and is important as a first line of defense following re-exposure to a pathogen in non-lymphoid tissues. Trm cells express high levels of CD69 and lower levels of CD62L, facilitating their tissue homing and retention capacity (21, 27). Although memory T cells respond rapidly to parasites following re-exposure, little is known about their persistence after cure of visceral disease, despite numerous studies (28, 29).
T cell responses in leishmaniasis
Early studies of Leishmania infection in mice helped develop the paradigm for T helper cell differentiation and committed effector functions (30). A central cytokine produced by DC in priming protective immune responses is IL-12. Production of IL-12 during CD4+ T cell priming drives the generation of IFN-γ producing Th1 cells that are essential for macrophage activation and control of Leishmania parasites (31, 32). The CD4+ T cell responses seen in leishmaniasis patients depend on the causative species and the site of infection. In VL, which is a progressive systemic disease, patients present a highly mixed immune profile, where pro-inflammatory mediators like IL-1, IL-6, IL-8, IL-12, IL-15, IFN-γ and TNF-Α, along with IL-10 and IL-4 are increased in the plasma (33, 34). In spleen, liver and bone marrow, CD4+ T cells express elevated levels of IFN-γ along with IL-10 (34) (reviewed in (35). Interestingly, VL does not lead to the expansion of FoxP3+ Treg cells (36). Instead, chronic infection with L. (L.) donovani is associated with the development of type I regulatory (Tr1) cells, which were defined as IL-10 producing-CD4+CD25-Foxp3- T cells in VL patients (34). Subsequent studies in mice showed that the transcription factor Blimp1 was crucial for Tr1 cell development and IL-10 production (37). These Tr1 cells are believed to develop from Th1 cells and suppress pro-inflammatory immune responses, serving to protect the patient from tissue pathology (38). However, by doing so, they may also promote parasite survival. In mice infected with L. donovani, a distinct population of LAG3+CXCR5+ CD4+ T cells have also been observed recently. This subset is thought to play a role in the maintenance of T cell responses. These cells can differentiate into regulatory and effector cells and can confer protection on adoptive transfer (39).
CD8+ T cells in human leishmaniasis can play both protective and exacerbating roles, depending on their functional characteristics and state (40). On one hand, an IFNγ-dominant CD8+ T cell response is suggested to contribute to protective immunity in both murine and human VL, potentially aiding in control of the infection (40). Conversely, upregulation of IL-10 within CD8+ T cells has been associated with VL progression (41, 42). These cells can exhibit cytotoxic T lymphocyte (CTL) features, which are associated with increased IFN-γ production in plasma. However, CD8+ T cells in chronic leishmaniasis often also display markers of exhaustion or anergy, such as elevated expression of inhibitory receptors CTLA-4 and PD-1, which can impair their responsiveness to Leishmania antigens, as seen in whole blood assays and PBMC cultures (42, 43). Following successful treatment, CD8+ T cells can regain responsiveness, potentially providing protection against re-infection (42) (Figure 1). Additionally, experimental VL models indicate that blocking the B7-H1 (PD-1 ligand) pathway can reduce splenic parasite burden and alleviate CD8+ T cell exhaustion and apoptosis (44).
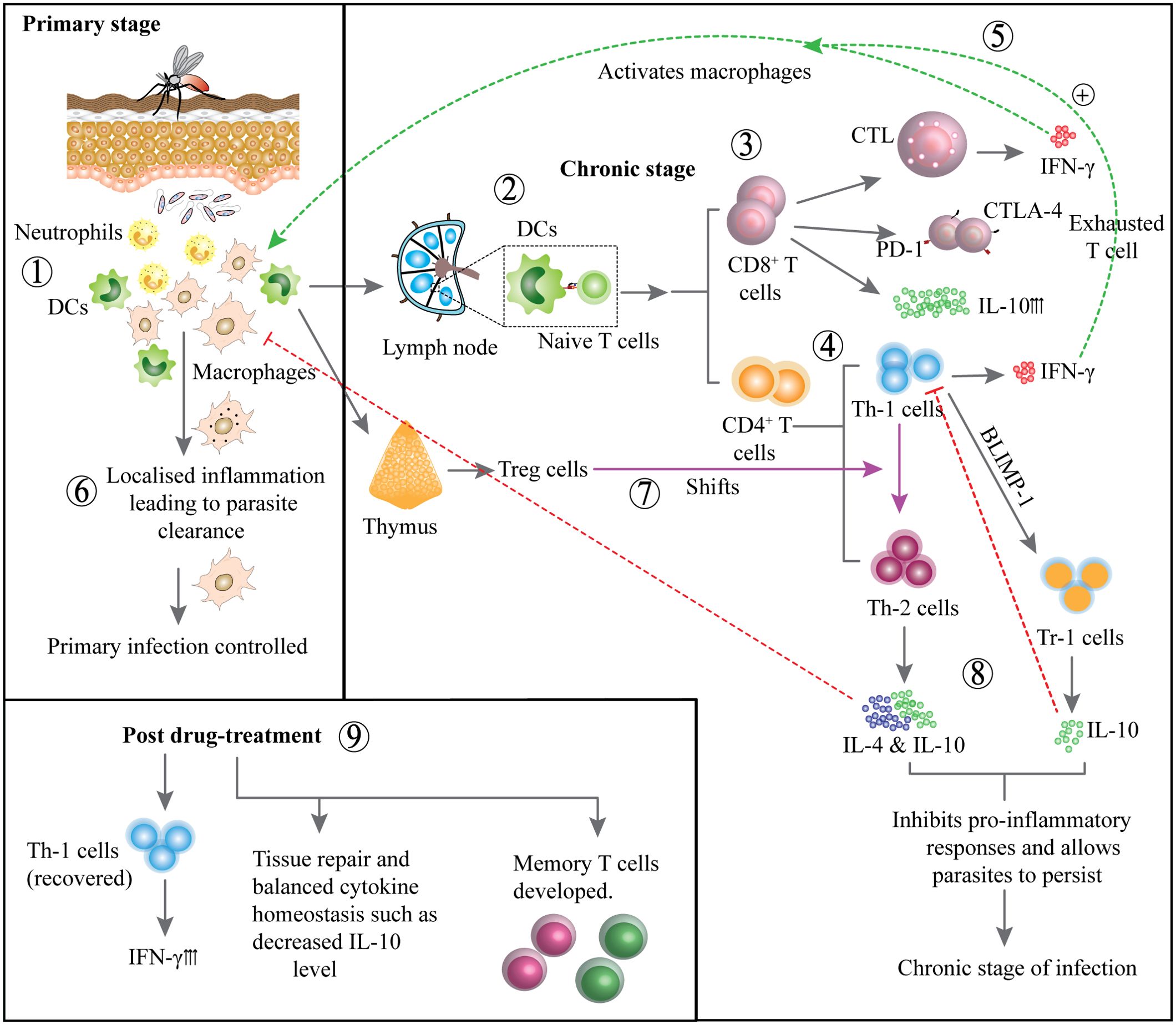
Figure 1. Development of immune responses during primary and chronic stages and post treatment: Neutrophils and DCs are activated at the infection site, initiating the immune response (1). DCs migrate to lymph nodes to present antigens to naïve T cells (2). CD8+ T cells become activated but may display exhaustion markers CTLA-4, PD-1 and produce IL-10 (3). CD4+ T cells differentiate into Th1 cells, that produces IFN-γ to activate macrophages (4). IFN-γ production enhances macrophage activation, contributing to parasite control (5). Primary infection is controlled by intracellular parasite clearance and localized inflammation (6). In contrast, Treg cells may suppress immune responses, promoting parasite persistence (7). T helper cells may shift towards Th2 phenotype producing IL-4 and IL-10 or Th1 can gradually develop into Tr-1 cells producing IL-10, inhibiting pro-inflammatory responses and promoting chronic infection (8). Following treatment, Th1 cell activity is restored, IFN-γ production increases, cytokine balance is re-established and memory T cells develop to support long term immunity (9).
The lack of leptins, which is a feature of malnourished individuals and VL patients (45) has been suggested as a reason for the impaired and exhausted T cell phenotype seen in experimental VL (46). Experimental studies in mice show that CD8+ T cells contribute to the protective immunity. Murray et al. found that infection promoted the generation of CD8+ T cells that could protect against re-infection in experimental VL (47). Furthermore, a vaccine-induced CD8+ T cell response could confer protection against L. (L.) donovani in mice (48). CD8+ T cells can mediate protection via the secretion of IFN-γ, perforin and granzymes (49), and depleting CD8+ T cells was found to impair hepatic granuloma formation and was associated with a failure to curb liver infection in L. (L.) donovani infected mice (50). In human VL, Th17 cells have been associated with protection against L. (L.) donovani infection by mediating intracellular pathogen clearance along with Th1 cytokines (51). Moreover, experimental sensitization with live attenuated L. (L.) donovani has been reported to promote an efficient IL-17-dominant immune response that confers protection to the host (52) suggesting role for Th17 cells in protective memory responses.
Generation and maintenance of CD4+ T cell memory responses in VL
Both CD8+ and CD4+ memory T cells generated after natural infection or vaccination are important for parasite control. But the generation and maintenance of these memory cells differ significantly. The generation and maintenance of CD4+ memory T cells likely requires persistence of parasite antigen or frequent re-exposure to parasites (18), while CD8+ memory T cells can be maintained without antigen persistence (53). However, there are some reports suggesting that CD4+ memory T cells can also be maintained after antigen clearance, but only when there was limited competition from other T cell clones (54, 55). A recent study from Brazil reported protective roles of Tcm and Tem positively correlated with IL-2, IFN-γ and TNF production by these cells in VL patients following drug treatment (56). These results indicate CD4+ memory T cell generation, but how long it was maintained and the role of these cells following reinfection needs further evaluation. However, a previous study evaluating cell mediated immune response in terms of lymphoproliferation and activation upon in vitro stimulation with leishmanial antigens in active VL, cured VL, and naive groups showed significantly high lymphoproliferation in cured VL group and a significant proportion of CD4+ and CD8+ T cells expressed activation markers. Individuals with a history of VL ranging from one to twenty years were included in this study, suggesting the presence of circulating memory T cells specific to Leishmania. A predominant Th1 type cytokine response was also observed (49). In line with the above findings another similar study reported maintenance of CD4+ T cells memory after recovery from VL. Interestingly, even leishmania skin test positive individuals showed similar memory T cell response on in vivo exposer to leishmanial antigens suggesting even asymptomatic infection can provide long term protection (57).
A proportion of Tcm cells may also include T follicular helper (Tfh) cells (CXCR-5+ Bcl-6+) which are critical for B cell antibody responses during infections. These CD4+ T cells retain their Tfh cell commitment during the resting memory stage and following reinfection, remain committed to providing help to memory B cells in germinal center (GC) of secondary lymphoid tissues (58, 59). The long-term maintenance of CD4+ memory T cell requires expression of IL-7RΑ, which is low on effector cells. CD4+ memory T cells have a distinct advantage over effector cells during the contraction phase owing to their ability to respond to IL-7, which stimulates anti-apoptotic bcl-6 expression. CD4+ memory T cells are maintained by IL-7 and IL-15, which also support the periodic replenishment of these cells (60) (Figure 2). In contrast, IL-2 favors effector CD4+ T cell formation (61).
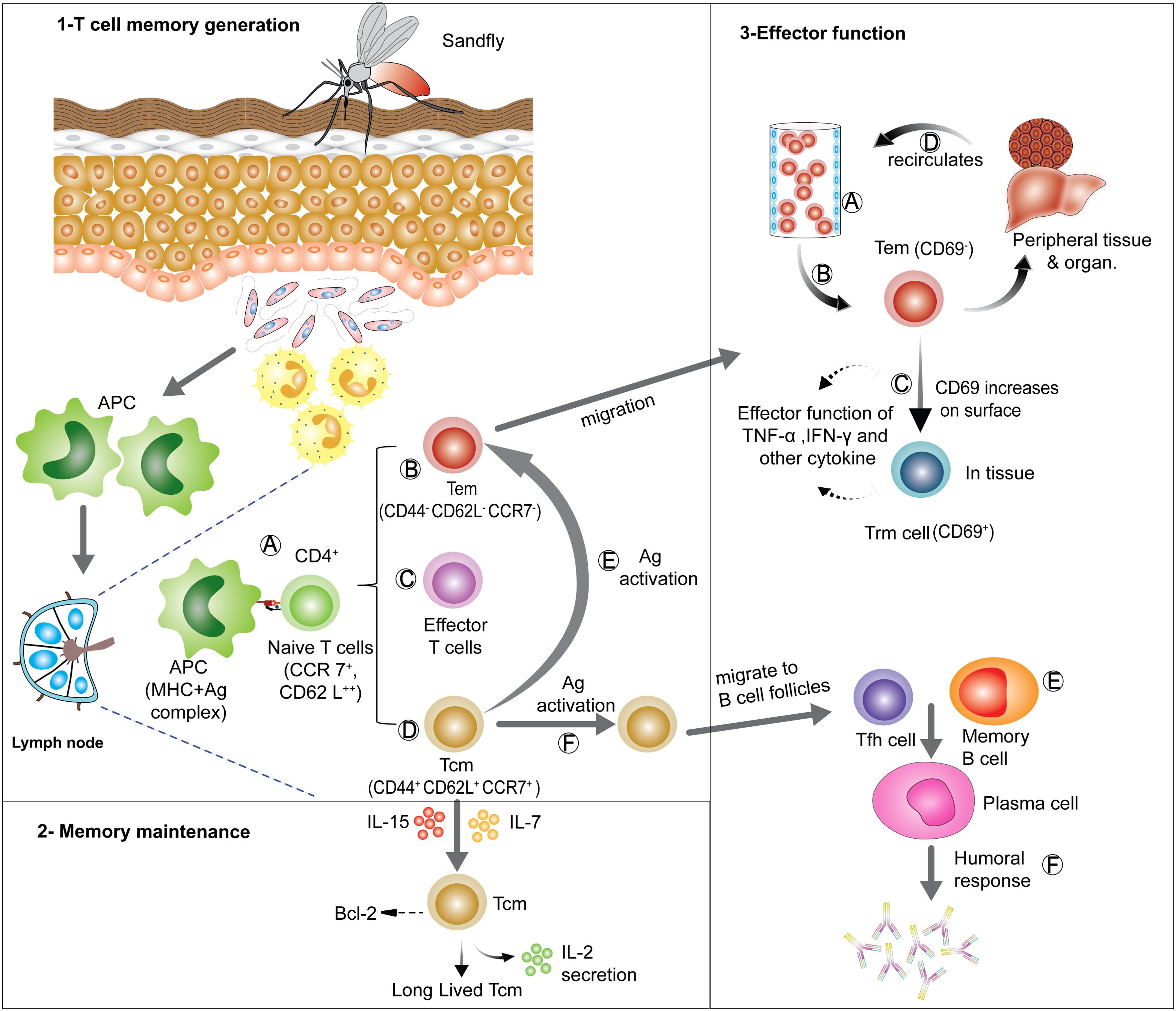
Figure 2. Generation, maintenance and effector functions of CD4+ T cell memory: (1) T cell memory generation- antigen presenting cells (APCs) take up parasites or parasite- infected neutrophils and moves to local lymph node for antigen presentation to naïve T cells. On recognizing the MHC II-peptide complex presented by APCs, naïve T cells get activated (A) and can differentiate into various subsets, such as Tem (B), short lived effector T cells (C) and Tcm (D). On re-exposer to antigen Tcm can re-differentiate into Tem (E) and Tfh (F). (2) Memory maintenance- Tcm cells can be long-lived in lymphoid organs in presence of IL-7 and IL-15 signaling, which sustains anti-apoptotic Bcl-6 expression. (3) Effector functions- Tem can migrate via blood (A) to various tissues and organs performing effector functions (B), Tem can also start expressing CD69 and can convert into tissue resident memory (Trm) cells, which can then act as a first line of defense on Ag re-exposer (C). Tem can also migrate through various organs and tissues and scan them for Ag (D). Tfh generated from Tcm cells can provide help to memory B cells (E) assisting early humoral (antibody) responses (F). [APCs, Antigen presenting cells; Ag, Antigen; Tcm, central memory T cells; Tem, effector memory T cells; Tfh, follicular helper T cells; Trm, tissue resident memory T cells].
VL generates an organ specific immune response in mice where Th1 cell-mediated responses and granuloma formation contribute to infection resolution in the liver and resistance to reinfection (62). In dogs formation of structured granulomas formation has been associated with disease control, while unstructured granulomas were associated with disease (63). Interestingly, in mice, parasites are controlled in the liver, but they persist and propagate slowly in the spleen (64, 65). Tissue specific immunity can also be observed in human VL, exemplified by nodular post Kala-Azar dermal leishmaniasis (PKDL), where parasites are controlled in the viscera after drug treatment, but continue replicating in the skin (65). This highlights the complexity of immune responses in different anatomical sites following resolution of visceral disease.
Furthermore, the chronic nature of VL, which affects organs such as the spleen, liver, and bone marrow, raises the possibility that prolonged exposure of the immune system to parasitic antigens may disrupt memory T cell development and maintenance, and the emergence of effector T cell populations, making it difficult to conclusively show what happens in the absence of infection.
Longevity of anti-parasitic immunity
The generation of anti-parasitic immunological memory in a given population occurs when individuals in endemic areas acquire immunity to subsequent infections after recovering from the disease. The cyclic nature of VL, referring to the periodic rise and fall of disease incidence in certain regions (66–68), is influenced by various factors, including the complex interactions between the parasite, the vector, the host population, population demographics, duration and intensity of the cycles and the environment. Importantly, this cyclic course of disease incidence has important implications for long-term immune maintenance. During periods of low disease transmission, asymptomatic individuals and those who have previously developed VL, been treated and recovered, contribute to the pool of immune individuals (64). This acquired immunity helps protect them from future infections. However, there is evidence to suggest that acquired immunity may gradually decline over time at least in CL (69). The duration and strength of immunity can vary among individuals, with some studies indicating that immunity may wane after a few years following the last infection in a given community (70, 71). This decline in immunity can lead to an increase in susceptible individuals in the population, providing opportunities for new infections and potential disease outbreaks during periods of increased transmission. However, repeated exposure to the parasite can lead to the maintenance of memory within a given population, as a significant portion of individuals will retain some level of immunity (69).
The maintenance of protective immunity in populations is also thought to be facilitated by the presence of asymptomatic carriers or individuals with subclinical infections. It is estimated that around 90% of Leishmania-infected individuals never present with any clinical symptoms and remain asymptomatic in India (72). However, diverse proportions of asymptomatic infections caused by L. (L.) donovani or L. (L.) infantum relative to symptomatic clinical cases occur in different part of the world. These ratios have been documented as 1:2.4 in Sudan, 4:1 in Kenya, 5.6:1 in Ethiopia, 6:1 to 18:1 in Brazil, 4:1 in Bangladesh, and 8.9:1 in India and Nepal (1, 73). Asymptomatic individuals, although not displaying clinical symptoms, still harbor parasites and therefore have the potential to contribute to disease transmission (68, 74). A recent xenodiagnoses study showed that asymptomatic individuals were not infectious to sand-fly vectors and may be considered an unimportant contributor to the maintenance of the L. (L.) donovani transmission cycle (75).
The treatment of VL is aimed at curing infected individuals and reducing the burden of the disease at the individual level rather than affecting the overall parasite population dynamics and maintenance of the parasite in populations. VL patients generally respond well to treatment, but treatment failure rates can range from 2-7% (76), and may reach up to 60% in HIV/VL co-infected individuals (77). Furthermore, incomplete treatment or treatment failure can lead to the persistence of parasites at a higher level than found in asymptomatic individuals. There is also an increasing concern that parasites will develop resistance to approved chemotherapeutic interventions, which may also promote disease relapse (78). Persisting splenomegaly after treatment may indicate incomplete parasites clearance and be another indicator of treatment failure (79). In addition, the immune status of a VL patient, particularly low CD4+ T cell count at the time of diagnosis and treatment, or during follow-ups may indicate a higher risk of relapse (80).
Multiple cases of reinfection in a community could indicate compromised immunity and/or diminished numbers of parasite-specific CD4+ memory T cells at the population level. To improve our current treatment and prevention approaches it is important to distinguish between relapsed disease and disease caused by reinfection, as relapse can indicate failure of treatment or persistence of large numbers of Leishmania parasites (81). A reinfection differs from a relapse in that new parasites are introduced. Reinfection signifies the lack or loss of infection-induced immunity. The impact of sterile cure following drug treatment (i.e., no parasites remaining) on the maintenance of immunity to VL is not known, and this may be an issue with the use of more effective anti-parasitic drugs such as Ambisome. However, evidence from studies in mice model of CL fuels the idea of concomitant immunity (82, 83)
In areas where the disease has been eliminated, the reintroduction of parasites can cause new disease outbreaks. Hence, monitoring immunity in regions where VL elimination programs have occurred or are ongoing could be informative in regard to population vulnerability to infection. Similar issues have been raised in malaria control programs, where continuous exposer to parasites may be important to maintain protective immunity in the population (84, 85).
Effect of HIV co-infections on T cell responses
The co-infection of Leishmania and HIV presents significant challenges for disease control and elimination efforts. Individuals with HIV have an increased risk of contracting VL, and VL can accelerate the progression of AIDS by promoting viral replication (6). HIV infection induces immune suppression primarily through the depletion of CD4+ T cells, which play a crucial role in controlling the Leishmania parasite. Additionally, the chronic immune activation caused by the Leishmania parasite may benefit HIV replication. This prolonged immune stimulation not only supports viral replication but also increases the expression of CCR5, a co-receptor crucial for HIV entry, on both CD4+ and CD8+ T cells (6, 86, 87). Various viral and parasitic factors contribute synergistically to promote each other’s persistence (88).
Patients co-infected with VL and HIV serve as critical reservoirs for Leishmania, with a higher potential for transmission compared to HIV-negative VL patients (89, 90). This heightened risk is due to the difficulty in fully eradicating the parasite in immunocompromised individuals (91), allowing Leishmania to persist and spread within populations. Furthermore, co-infected patients exhibit higher rates of relapse, as reported in multiple studies (92, 93).
Effect of nutritional status on T cell responses
The nutritional status of the host is a critical factor influencing immune responses and affecting the course and severity of VL. Malnutrition has been strongly linked to increased VL susceptibility, which is often associated with the socioeconomic conditions of endemic regions (94, 95). In a recent study, poly-nutrient-deficient L. infantum-infected mice exhibited higher parasite burdens in the spleen and liver, reduced T lymphocyte counts with lower IFN-γ, and elevated IL-10 production (96). Besides proteins, carbohydrates, and lipids, adequate vitamins and minerals (e.g., calcium, iron, iodine) are essential for adaptive and innate immunity, influencing treatment outcomes and relapse risk in leishmaniasis (97).
Factors affecting parasite persistence
As mentioned above, the continued exposure to parasite antigen (concomitant immunity), is likely important for maintaining effective anti-parasitic immunological memory. However, other disease interventions and control measures can impact on this exposure, and must be considered when managing the potential for disease outbreaks. Governments and organizations striving to eradicate VL have faced significant obstacles despite their substantial efforts, and the difficulties posed by the disease’s biology and its intricate socioeconomic association makes this task considerably more difficult. The disease has a cyclic nature in India as predicted before the epidemics of 1977 and 1991 by Napier (98, 99). The known cycle period of 10-15 years in India is not understood, but has been suggested to be associated with sand fly resistance to insecticides (100), which supports the implementation of vector control programs in conjunction with active case detection and treatment interventions (Figure 3). There have been times in the past when the disease appeared to vanish from highly endemic areas, as was the case in Assam (India), where an epidemic occurred between the late 19th and mid-20th century, killing thousands of people. However, the disease disappeared in the following years (31). At the time, credit for this was given to the use of DDT in the malaria elimination program. As a result of this effort, sand fly vectors were almost completely eliminated, and transmission ceased. However, there was an escalation in number of cases, nearly 60 years later, and epidemics reoccurred in 2004 and 2008, indicating a resurgence of vectors due to insecticide resistance or inefficient vector control strategies (101). Various environmental factors influence parasite life cycle and their persistence in the environment. For example, VL is associated with sub-standard housing and climatic conditions such as high humidity and temperature. A focus on the control of breeding habitats for sand flies can greatly reduce transmission rates (102, 103). The fact that there a range of reservoir hosts, which include rodents, wild dogs and even domestic dogs and cattle (104), indicate that the parasites are continually present in the immediate environment of humans, as long as sand flies are present to transmit them. Reports suggest that dogs serve as an important reservoir for VL-causing parasites in the Mediterranean and South America (105, 106).
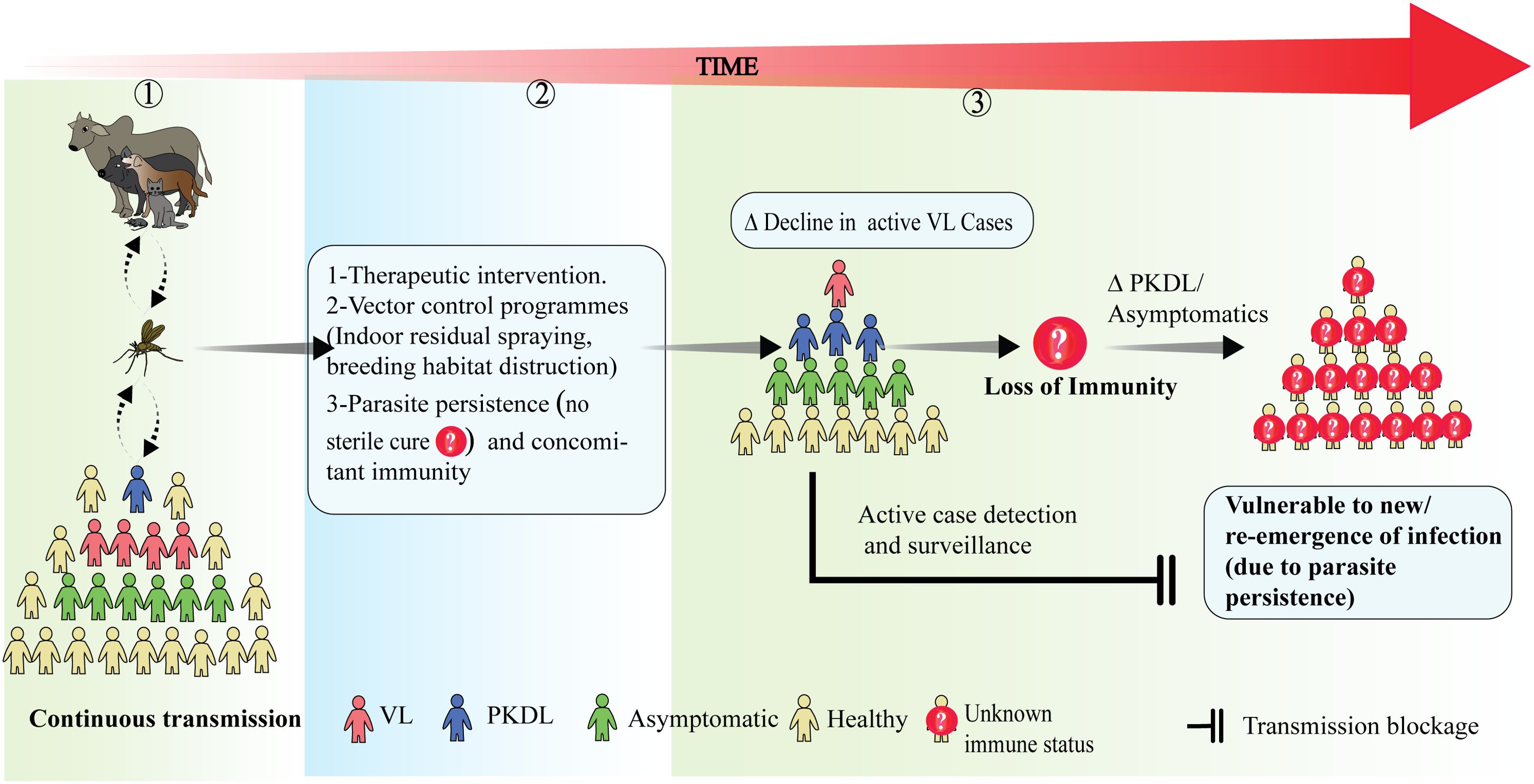
Figure 3. The effect of disease transmission on the maintenance of long-term population immunity and its implications for disease dynamics: (1) During continuous transmission in an endemic area there is a constant flow of leishmanial antigen among humans and other reservoir hosts and people with active VL, PKDL and asymptomatic infection co-exist. (2) Following disease & transmission control measures such as treatment interventions and vector control programs, parasite transmission may decline along with the number of active VL cases. However, parasites may still persist in treated VL cases, maintaining concomitant immunity. (3) An overall decline in transmission raises the possibility for impacts on long term population immunity. If this declines, it is important to understand the roles played by asymptomatic and PKDL individuals in the maintenance of population-level immunity and possible disease outbreaks. Factors effecting the vulnerability of populations to new/re-emergences of infection are still largely unknown. Thus, active case detection and surveillance still remains the best option to predict future outbreaks.
Human-made environmental changes like deforestation, settling near a forest or agriculture practices plays an important role in parasite transmission cycles by promoting closer proximity between humans and non-human reservoir hosts than would normally be the case (107). Even after efforts to limit the breeding or spread of sand fly vectors, active surveillance to identify human reservoirs such as asymptomatic individuals and PKDL patients is necessary (108–111). However, it should be acknowledged that the total eradication of the vector or the parasite may impair immunological memory developed during ongoing transmission and this may contribute to future outbreaks when infected individuals move into an area and/or sand fly vectors are reintroduced (112, 113). Furthermore, there have been suggestions that environmental factors may cause increased infectivity of parasites through genetic modifications resulting in parasite fitness gains (114). These findings highlight the need to understand the presence of parasites in environments and how this changes, as well as how immunity is maintained and the factors contributing to outbreaks (Figure 3).
PKDL and the challenge of persisting parasites
PKDL is a cutaneous manifestation of L. (L.) donovani infection that can arise after what appeared to be successful drug treatment. In PKDL patients, the immune system fails to control parasite growth in skin, while an effective systemic response protects the viscera. This suggests the presence of type 2 immune responses or other types of regulatory responses in the skin (115). Moreover, systemic protective immunity that persists is likely maintained by antigen-experienced memory cells, as evidenced by cytokine and proliferative CD4+ T cell responses during whole blood assay (56, 57). However, skin-specific immunological anomalies do exists, which could be attributed to a lack of organ-specific CD4+ T cell memory (116). PKDL presents with macules, papules or nodules on skin and rarely occurs in asymptomatic individuals having had no signs of VL. The disease is frequently seen before and after cure of African VL, while in India, PKDL is rare and the onset generally occurs years after VL cure. Notably, the African form is typically self-healing while the Indian form of disease is chronic. In nodular PKDL, the presence of heavily infected macrophages in skin can serve as reservoirs and facilitate transmission, posing a threat to the VL elimination programs (117). Pathogenesis of PKDL is considered to be immunologically mediated with increased IL-10 production by keratinocytes and other cells having been reported during active disease and has been suggested to promote the parasite persistence (118). The mRNA profiles from both blood and skin indicated that PKDL patients have a mixed Th1 and Th2 cell response, as indicated by high levels of expression of IFNγ, TNF, TGFβ, IL-10, IL-6, and IL-4 with decreased mRNA expression of IFN-γ receptor 1 (IFNγR1) (119). Accumulating evidence suggest a role for IL-10 mediated Treg cell differentiation and accumulation in the skin associated with disease severity (120). Understanding why some individuals develop PKDL is a major challenge in the context of VL elimination. Understanding how protection of visceral organs is maintained while disease manifests in the skin is an intriguing challenge, and understanding the immunological mechanisms responsible may shed important light about how immunity to VL is maintained and/or lost.
CD4+ T cell memory response and vaccine development
The idea that a vaccine to protect against VL would be feasible arose from the past success of “Leishmanization” where live parasites from cutaneous lesions were used to cause cutaneous disease in covered body locations to protect against disfiguring CL (28, 121–123). This form of deliberate infection typically conferred life-long protection against subsequent disease (124). It was relatively effective in terms of the protection it offered, but due to potential complications in some individuals, this vaccination technique was gradually abandoned (125). The creation of a vaccine against VL has still not been achieved and a major bottle neck is our incomplete understanding about how protective, anti-parasitic CD4+ T cell memory is generated and maintained in humans. However, the development of VL vaccines for dogs, like Leish-111f + MPL-SE (126), offers hope that vaccines for humans can be developed (127). Murine studies utilizing different vaccine candidates have also shown that a potent memory T cell response can be generated following immunization that can confer long term protection against VL (128, 129). The prospect of developing a successful VL vaccine is further strengthened by reports suggesting existence of leishmanial antigen reactive memory T cells long after clinical cure of human infection (49, 57).
CD4+ memory T cells are crucial for the development of an effective vaccine against VL as they are central to orchestrating the adaptive immune response needed to control and eliminate Leishmania parasites. These cells facilitate the production of cytokines, such as IFN-γ, which activate macrophages to effectively kill intracellular parasites (130). Moreover, CD4+ memory T cells enable a rapid and robust immune response upon re-exposure to the pathogen, thereby ensuring long-term immunity (131). Research has shown that polyclonal expansion of effector memory (Tem) cells occurs after treatment in human VL cases (132). Vaccine studies have also linked the generation of CD4+ T cell memory with protective immunity (133). The persistence of these memory T cells after initial infection or vaccination is vital for providing lasting protection, evidenced by the induction of tissue-resident memory (Trm) cells in mouse models of cutaneous leishmaniasis (CL) (134). Studies performed in both murine and canine models found that effective cell mediated immunity (CMI) along with Tcm (CD127high CD44high CD45low CD62Lhigh CD197high) and Tem (CD62Llow CD44high CD127high CD197low) cells not necessarily require live parasites and could be generated by a peptide cocktail from L. (L.) infantum, resulting in a decreased parasite load in splenic cell cultures of BALB/c mice when reinfected (135). A similar approach using an immunodominant fusion of multiple peptide epitopes from L. (L.) major, L. (L.) donovani and L. (V.) brazilinesis species was also able to generate potent CMI and CD4+ memory T cells, resulting in protection following challenge with L. (L.) infantum infection (136). CD4+ memory T cell generation against Leishmania parasites, just like other diseases, not only depends upon antigen stimulation, co-stimulation and DC cytokine production, but is also influenced by other immune cells. For example, marginal zone B cells (MZB) were reported to impair CD8+ and CD4+ Tem cell generation against L. (L.) donovani challenge in mice (137).
Interestingly, experimental models using L. (L.) infantum infection indicate that persistent parasite may negatively affect vaccine induced frequencies of effector and memory CD4+ T cells, as well as CD8+ T cells, in comparison to the non-infected mice. However when parasites where killed by drug treatment this resulted in release of antigens and the vaccine induced memory T cell development increased upon re-exposure to antigen (138).
The use of LACK (leishmania homologue for receptors of activated C kinase), an intracellular Leishmania protein as a vaccine has been shown to confer protection against CL in murine models (139) and against VL in dogs (140). The protection was reported to be mediated by a Th1 type immune responses. Another vaccination study identified the immune parameters involved in protective immunity against CL in BALB/c mice. The study reported that CD4+ and CD8+ effector memory T cells, along with TNF, IFNγ, and other type 1 cytokines, play crucial role in protection (141). Similarly, the polyprotein subunit vaccine KSAC produced by fusing the antigenic proteins KMP11, SMT, A2, and CPB has been shown to provide protection mediated by IFN-γ-producing Th1 cells (142).
The presence of leishmanial antigen-reactive CD4+ memory T cells long after clinical recovery highlights their role in immune surveillance and protection against reinfection. Leishmania infection can induce partial immunity, marked by memory T cells ready to rapidly respond upon re-exposure. Individuals recovered from symptomatic VL often display a more robust, IFN-γ-dominant cytokine response ex vivo, which may contribute to enhanced resistance to severe disease upon re-infection (143, 144). In mouse model of CL, persistent low-level parasites are believed to confer protection by maintaining immunological memory (18). However, evidence of this persistence in human cases of CL and VL is limited, as most studies focus on model organisms. Additionally, chronic exposure to leishmanial antigens can lead to T cell unresponsiveness, raising concerns about the effectiveness of these memory T cells over time. Chronic exposure to leishmanial antigens can also lead to regulatory T cell induction, which may compromise T cell responses (34, 145). These immune adaptations present challenges for vaccination efforts, as immune tolerance or exhaustion can reduce vaccine efficacy in VL endemic populations, similar to observations in populations with chronic Plasmodium exposure (146). Consequently, studying immune responses in asymptomatic individuals or VL patients that have been successfully drug treated may provide guidance on biomarkers that can be used to assess the effectiveness of vaccination and the duration of subsequent immunity.
Discussion and future prospective
Leishmaniasis is a neglected tropical disease and there is a critical need for an effective vaccine and more potent pharmacological therapies. Most of our knowledge regarding the immunological aspects of VL comes from experimental models and a lack of proper insight from infected humans has resulted in a major knowledge gap. To understand why those who have been treated gain protective immunity and why the majority of infected individuals do not develop disease, will require studies on human immune responses. Regarding VL, while it is evident that a Th1 cell immune response confers protection against the disease, we still don’t know whether the persistence of parasites is needed to maintain protective CD4+ T cell memory responses. Reports suggesting the persistence of low parasite numbers is needed for protective immunity has been reported in mouse models (reviewed in (20). Concomitant immunity, in which immunity to pathogens relies on viable pathogen persistence, may nonetheless, hold the key to delaying the onset of disease, but relapses might occur with lifelong persistence of parasites (138). There is an important need to establish the longevity of the CD4+ memory T cell pool following sterile cure, as this will help determine the degree of disease susceptibility in areas of potential disease outbreaks.
Finally, it is worth highlighting again that people who recover from VL following drug treatment are protected against future disease, thus demonstrating there is a natural mechanism of protective immunity that needs to be understood so we can design vaccines and treatments to eliminate this disease.
Author contributions
RT: Conceptualization, Writing – original draft, Writing – review & editing. AK: Software, Writing – review & editing. VS: Software, Writing – review & editing. SC: Software, Writing – review & editing. R: Software, Writing – review & editing. SS: Writing – review & editing. SN: Writing – review & editing. CE: Writing – review & editing, Validation. RK: Conceptualization, Funding acquisition, Supervision, Writing – original draft, Writing – review & editing.
Funding
The author(s) declare financial support was received for the research, authorship, and/or publication of this article. This work is supported through the funding from Science and Engineering Research Board (Grant number CRG/2022/007610). Research in the authors’ laboratory is supported through the funding from Indian Council of Medical Research (Grant number 2020-9898) and BHU-IoE (Seed Grant).
Acknowledgments
RT, VS, AK, R and SC would like to thank SERB, DBT, UGC, ICMR and BHU-IoE respectively for providing them Fellowship.
Conflict of interest
The authors declare that the research was conducted in the absence of any commercial or financial relationships that could be construed as a potential conflict of interest.
The author(s) declared that they were an editorial board member of Frontiers, at the time of submission. This had no impact on the peer review process and the final decision
Publisher’s note
All claims expressed in this article are solely those of the authors and do not necessarily represent those of their affiliated organizations, or those of the publisher, the editors and the reviewers. Any product that may be evaluated in this article, or claim that may be made by its manufacturer, is not guaranteed or endorsed by the publisher.
References
1. Burza S, Croft SL, Boelaert M. Leishmaniasis. Lancet (London England). (2018) 392:951–70. doi: 10.1016/s0140-6736(18)31204-2
2. Reithinger R, Dujardin JC, Louzir H, Pirmez C, Alexander B, Brooker S. Cutaneous leishmaniasis. Lancet Infect Dis. (2007) 7:581–96. doi: 10.1016/s1473-3099(07)70209-8
3. Espinosa OA, Serrano MG, Camargo EP, Teixeira MMG, Shaw JJ. An appraisal of the taxonomy and nomenclature of trypanosomatids presently classified as leishmania and endotrypanum. Parasitology. (2018) 145:430–42. doi: 10.1017/s0031182016002092
4. Sundar S, Rai M. Laboratory diagnosis of visceral leishmaniasis. Clin Vaccine Immunol. (2002) 9:951–8. doi: 10.1128/CDLI.9.5.951-958.2002
5. Chappuis F, Sundar S, Hailu A, Ghalib H, Rijal S, Peeling RW, et al. Visceral leishmaniasis: what are the needs for diagnosis, treatment and control? Nat Rev Microbiol. (2007) 5:873–82. doi: 10.1038/nrmicro1748
6. Lindoso JAL, Moreira CHV, Cunha MA, Queiroz IT. Visceral leishmaniasis and hiv coinfection: current perspectives. HIV/AIDS-research palliative care. (2018). Auckland, N.Z.: Dove Press, pp. 193–201. doi: 10.2147/HIV.S143929
7. Croft SL, Coombs GH. Leishmaniasis–current chemotherapy and recent advances in the search for novel drugs. Trends Parasitol. (2003) 19:502–8. doi: 10.1016/j.pt.2003.09.008
8. Classon C, Feng X, Eidsmo L, Nylén S. Intestinal nematode infection exacerbates experimental visceral leishmaniasis. Parasite Immunol. (2019) 41:e12618. doi: 10.1111/pim.2019.41.issue-4
9. Singh AK, de Gooyer T, Singh OP, Pandey S, Neyaz A, Cloots K, et al. Wuchereria bancrofti infection is associated with progression to clinical visceral leishmaniasis in vl- endemic areas in muzaffarpur, bihar, India. PLoS Negl Trop Dis. (2023) 17:e0011729. doi: 10.1371/journal.pntd.0011729
10. Singh N, Mishra J, Singh R, Singh S. Animal reservoirs of visceral leishmaniasis in India. J Parasitol. (2013) 99:64–7. doi: 10.1645/ge-3085.1
11. Kushwaha AK, Scorza BM, Singh OP, Rowton E, Lawyer P, Sundar S, et al. Domestic mammals as reservoirs for leishmania donovani on the Indian subcontinent: possibility and consequences on elimination. Transboundary emerging Dis. (2022) 69:268–77. doi: 10.1111/tbed.14061
12. Kushwaha AK, Shukla A, Scorza BM, Kumari Rai T, Chaubey R, Kumar Maurya D, et al. Livestock and rodents within an endemic focus of visceral leishmaniasis are not reservoir hosts for leishmania donovani. PLoS Negl Trop Dis. (2022) 16:e0010347. doi: 10.1371/journal.pntd.0010347
13. Cecílio P, Cordeiro-da-Silva A, Oliveira F. Sand flies: basic information on the vectors of leishmaniasis and their interactions with leishmania parasites. Commun Biol. (2022) 5:305. doi: 10.1038/s42003-022-03240-z
14. Kaye P, Scott P. Leishmaniasis: complexity at the host–pathogen interface. Nat Rev Microbiol. (2011) 9:604–15. doi: 10.1038/nrmicro2608
15. Peters NC, Egen JG, Secundino N, Debrabant A, Kimblin N, Kamhawi S, et al. In vivo imaging reveals an essential role for neutrophils in leishmaniasis transmitted by sand flies. Science. (2008) 321:970–4. doi: 10.1126/science.1159194
16. Bhor R, Rafati S, Pai K. Cytokine saga in visceral leishmaniasis. Cytokine. (2021) 147:155322. doi: 10.1016/j.cyto.2020.155322
17. Kolaczkowska E, Kubes P. Neutrophil recruitment and function in health and inflammation. Nat Rev Immunol. (2013) 13:159–75. doi: 10.1038/nri3399
18. Belkaid Y, Piccirillo CA, Mendez S, Shevach EM, Sacks DL. Cd4+Cd25+ Regulatory T cells control leishmania major persistence and immunity. Nature. (2002) 420:502–7. doi: 10.1038/nature01152
19. Uzonna JE, Wei G, Yurkowski D, Bretscher P. Immune elimination of leishmania major in mice: implications for immune memory, vaccination, and reactivation disease. J Immunol. (2001) 167:6967–74. doi: 10.4049/jimmunol.167.12.6967
20. Scott P. Long-lived skin-resident memory T cells contribute to concomitant immunity in cutaneous leishmaniasis. Cold Spring Harbor Perspect Biol. (2020) 12:a038059. doi: 10.1101/cshperspect.a038059
21. Sallusto F, Geginat J, Lanzavecchia A. Central memory and effector memory T cell subsets: function, generation, and maintenance. Annu Rev Immunol. (2004) 22:745–63. doi: 10.1146/annurev.immunol.22.012703.104702
22. Pennock ND, White JT, Cross EW, Cheney EE, Tamburini BA, Kedl RM. T cell responses: naive to memory and everything in between. Adv Physiol Educ. (2013) 37:273–83. doi: 10.1152/advan.00066.2013
23. Mempel TR, Henrickson SE, Von Andrian UH. T-cell priming by dendritic cells in lymph nodes occurs in three distinct phases. Nature. (2004) 427:154–9. doi: 10.1038/nature02238
24. Curtsinger JM, Schmidt CS, Mondino A, Lins DC, Kedl RM, Jenkins MK, et al. Inflammatory cytokines provide a third signal for activation of naive cd4+ and cd8+ T cells. J Immunol. (1999) m162:3256–62. doi: 10.4049/jimmunol.162.6.3256
25. Mondino A, Manzo T. To remember or to forget: the role of good and bad memories in adoptive T cell therapy for tumors. Front Immunol. (2020) 11):1915. doi: 10.3389/fimmu.2020.01915
26. Sanders ME, Makgoba M, Sharrow S, Stephany D, Springer T, Young H, et al. Human memory T lymphocytes express increased levels of three cell adhesion molecules (Lfa-3, cd2, and lfa-1) and three other molecules (Uchl1, cdw29, and pgp-1) and have enhanced ifn-gamma production. J Immunol. (1988) 140:1401–7. doi: 10.4049/jimmunol.140.5.1401
27. Mahnke YD, Brodie TM, Sallusto F, Roederer M, Lugli E. The who’s who of T-cell differentiation: human memory T-cell subsets. Eur J Immunol. (2013) 43:2797–809. doi: 10.1002/eji.201343751
28. Nylén S, Khamesipour A, Mohammadi A, Jafari-Shakib R, Eidsmo L, Noazin S, et al. Surrogate markers of immunity to leishmania major in leishmanin skin test negative individuals from an endemic area re-visited. Vaccine. (2006) 24:6944–54. doi: 10.1016/j.vaccine.2006.05.016
29. Glennie ND, Yeramilli VA, Beiting DP, Volk SW, Weaver CT, Scott P. Skin-resident memory cd4+ T cells enhance protection against leishmania major infection. J Exp Med. (2015) 212:1405–14. doi: 10.1084/jem.20142101
30. Alexander J, Brombacher F. T helper1/T helper2 cells and resistance/susceptibility to leishmania infection: is this paradigm still relevant? Front Immunol. (2012) 3. doi: 10.3389/fimmu.2012.00080
31. Rogers KA, DeKrey GK, Mbow ML, Gillespie RD, Brodskyn CI, Titus RG. Type 1 and type 2 responses to leishmania majoe. FEMS Microbiol Lett. (2002) 209:1–7. doi: 10.1111/j.1574-6968.2002.tb11101.x
32. Launois P, Tacchini-Cottier F, Parra-Lopez C, Louis JA. Cytokines in parasitic diseases: the example of cutaneous leishmaniasis. Int Rev Immunol. (1998) 17:157–80. doi: 10.3109/08830189809084491
33. Rostami MN, Khamesipour A. Potential biomarkers of immune protection in human leishmaniasis. Med Microbiol Immunol. (2021) 210:81–100. doi: 10.1007/s00430-021-00703-8
34. Nylén S, Maurya R, Eidsmo L, Manandhar KD, Sundar S, Sacks D. Splenic accumulation of il-10 mrna in T cells distinct from cd4+ Cd25+ (Foxp3) regulatory T cells in human visceral leishmaniasis. J Exp Med. (2007) 204:805–17. doi: 10.1084/jem.20061141
35. Nylén S, Sacks D. Interleukin-10 and the pathogenesis of human visceral leishmaniasis. Trends Immunol. (2007) 28:378–84. doi: 10.1016/j.it.2007.07.004
36. Maurya R, Kumar R, Prajapati VK, Manandhar KD, Sacks D, Sundar S, et al. Human visceral leishmaniasis is not associated with expansion or accumulation of foxp3+ Cd4 cells in blood or spleen. Parasite Immunol. (2010) 32:479–83. doi: 10.1111/j.1365-3024.2010.01219.x
37. Neumann C, Heinrich F, Neumann K, Junghans V, Mashreghi MF, Ahlers J, et al. Role of blimp-1 in programing th effector cells into il-10 producers. J Exp Med. (2014) 9):1807–19. doi: 10.1084/jem.20131548
38. Montes de Oca M, Kumar R, de Labastida Rivera F, Amante FH, Sheel M, Faleiro RJ, et al. Blimp-1-dependent il-10 production by tr1 cells regulates tnf-mediated tissue pathology. PLoS Pathog. (2016) 12:1005398. doi: 10.1371/journal.ppat.1005398
39. Swaminathan S, Mai LT, Meli AP, Carmona-Pérez L, Charpentier T, Lamarre A, et al. Lag-3- and cxcr5-expressing cd4 T cells display progenitor-like properties during chronic visceral leishmaniasis. Cell Rep. (2024) 43:113879. doi: 10.1016/j.celrep.2024.113879
40. Stäger S, Rafati S. Cd8(+) T cells in leishmania infections: friends or foes? Front Immunol. (2012) 3:5. doi: 10.3389/fimmu.2012.00005
41. Gautam S, Kumar R, Maurya R, Nylén S, Ansari N, Rai M, et al. Il-10 neutralization promotes parasite clearance in splenic aspirate cells from patients with visceral leishmaniasis. J Infect Dis. (2011) 204:1134–7. doi: 10.1093/infdis/jir461
42. Gautam S, Kumar R, Singh N, Singh AK, Rai M, Sacks D, et al. Cd8 T cell exhaustion in human visceral leishmaniasis. J Infect Dis. (2014) 209:290–9. doi: 10.1093/infdis/jit401
43. Singh B, Bhushan Chauhan S, Kumar R, Singh SS, Ng S, Amante F, et al. A molecular signature for cd8+ T cells from visceral leishmaniasis patients. Parasite Immunol. (2019) 41:e12669. doi: 10.1111/pim.v41.11
44. Joshi T, Rodriguez S, Perovic V, Cockburn IA, Stäger S. B7-H1 blockade increases survival of dysfunctional cd8(+) T cells and confers protection against leishmania donovani infections. PLoS Pathog. (2009) 5:e1000431. doi: 10.1371/journal.ppat.1000431
45. AMdC F, Silva-Freitas ML, AdQ S, Santos-Oliveira JR, Da-Cruz AM. Lower levels of leptin are associated with severity parameters in visceral leishmaniasis patients. PLoS One. (2019) 14:e0214413. doi: 10.1371/journal.pone.0214413
46. Dayakar A, Chandrasekaran S, Veronica J, Bharadwaja V, Maurya R. Leptin regulates granzyme-a, pd-1 and ctla-4 expression in T cell to control visceral leishmaniasis in balb/C mice. Sci Rep. (2017) 7:14664. doi: 10.1038/s41598-017-15288-7
47. Murray HW. Prevention of relapse after chemotherapy in a chronic intracellular infection: mechanisms in experimental visceral leishmaniasis. J Immunol. (2005) 174:4916–23. doi: 10.4049/jimmunol.174.8.4916
48. Majumder S, Bhattacharjee S, Paul Chowdhury B, Majumdar S. Cxcl10 is critical for the generation of protective cd8 T cell response induced by antigen pulsed cpg-odn activated dendritic cells. PLoS One. (2012) 7:e48727. doi: 10.1371/journal.pone.0048727
49. Kaushal H, Bras-Gonçalves R, Negi NS, Lemesre JL, Papierok G, Salotra P. Role of cd8(+) T cells in protection against leishmania donovani infection in healed visceral leishmaniasis individuals. BMC Infect Dis. (2014) 14:653. doi: 10.1186/s12879-014-0653-6
50. Stern JJ, Oca MJ, Rubin BY, Anderson SL, Murray HW. Role of L3t4+ and lyt-2+ Cells in experimental visceral leishmaniasis. J Immunol. (1988) 140:3971–7. doi: 10.4049/jimmunol.140.11.3971
51. Pitta MG, Romano A, Cabantous S, Henri S, Hammad A, Kouriba B, et al. Il-17 and il-22 are associated with protection against human kala azar caused by leishmania donovani. J Clin Invest. (2009) 119:2379–87. doi: 10.1172/JCI38813
52. Banerjee A, Bhattacharya P, Dagur PK, Karmakar S, Ismail N, Joshi AB, et al. Live attenuated leishmania donovani centrin gene-deleted parasites induce il-23-dependent il-17-protective immune response against visceral leishmaniasis in a murine model. J Immunol. (2018) 200:163–76. doi: 10.4049/jimmunol.1700674
53. Tanchot C, Lemonnier FA, Pérarnau B, Freitas AA, Rocha B. Differential requirements for survival and proliferation of cd8 naive or memory T cells. Science. (1997) 276:2057–62. doi: 10.1126/science.276.5321.2057
54. Polley R, Zubairi S, Kaye PM. The fate of heterologous cd4+ T cells during leishmania donovani infection. Eur J Immunol. (2005) 35:498–504. doi: 10.1002/eji.200425436
55. Garcia S, DiSanto J, Stockinger B. Following the development of a cd4 T cell response in vivo: from activation to memory formation. Immunity. (1999) 11:163–71. doi: 10.1016/S1074-7613(00)80091-6
56. Rodrigues LS, Barreto AS, Bomfim LGS, Gomes MC, Ferreira NLC, da Cruz GS, et al. Multifunctional, tnf-Α and ifn-Γ-secreting cd4 and cd8 T cells and cd8(High) T cells are associated with the cure of human visceral leishmaniasis. Front Immunol. (2021) 12:773983. doi: 10.3389/fimmu.2021.773983
57. Rodrigues-Neto JF, Monteiro GR, Keesen TSL, Lacerda HG, Carvalho EM, Jeronimo SMB. Cd45ro+ T cells and T cell activation in the long-lasting immunity after leishmania infantum infection. Am J Trop Med hygiene. (2018) 98:875–82. doi: 10.4269/ajtmh.16-0747
58. Hale JS, Youngblood B, Latner DR, Mohammed AUR, Ye L, Akondy RS, et al. Distinct memory cd4+ T cells with commitment to T follicular helper-and T helper 1-cell lineages are generated after acute viral infection. Immunity. (2013) 38:805–17. doi: 10.1016/j.immuni.2013.02.020
59. Liu D, Uzonna JE. The P110δ Isoform of phosphatidylinositol 3-kinase controls the quality of secondary anti-leishmania immunity by regulating expansion and effector function of memory T cell subsets. J Immunol. (2010) 184:3098–105. doi: 10.4049/jimmunol.0903177
60. Jameson SC, Masopust D. Diversity in T cell memory: an embarrassment of riches. Immunity. (2009) 31:859–71. doi: 10.1016/j.immuni.2009.11.007
61. Belz GT, Masson F. Interleukin-2 tickles T cell memory. Immunity. (2010) 32:7–9. doi: 10.1016/j.immuni.2010.01.009
62. Murray HW, Stern JJ, Welte K, Rubin B, Carriero S, Nathan C. Experimental visceral leishmaniasis: production of interleukin 2 and interferon-gamma, tissue immune reaction, and response to treatment with interleukin 2 and interferon-gamma. J Immunol. (1987) 138:2290–7. doi: 10.4049/jimmunol.138.7.2290
63. Murray HW. Tissue granuloma structure-function in experimental visceral leishmaniasis. Int J Exp Pathol. (2001) 82:249–67. doi: 10.1046/j.1365-2613.2001.00199.x
64. Gidwani K, Picado A, Ostyn B, Singh SP, Kumar R, Khanal B, et al. Persistence of leishmania donovani antibodies in past visceral leishmaniasis cases in India. Clin Vaccine Immunol. (2011) 18:346–8. doi: 10.1128/CVI.00473-10
65. Kumar A, Singh VK, Tiwari R, Madhukar P, Rajneesh, Kumar S, et al. Post kala-azar dermal leishmaniasis in the Indian sub-continent: challenges and strategies for elimination. Front Immunol. (2023) 14:1236952. doi: 10.3389/fimmu.2023.1236952
66. Deb RM, Stanton MC, Foster GM, Gupta RKD, Roy N, Das P, et al. Visceral leishmaniasis cyclical trends in bihar, India–implications for the elimination programme. Gates Open Res. (2018) 2. doi: 10.12688/gatesopenres
67. Ceballos LA, Piccinali RV, Marcet PL, Vazquez-Prokopec GM, Cardinal MV, Schachter-Broide J, et al. Hidden sylvatic foci of the main vector of chagas disease triatoma infestans: threats to the vector elimination campaign? PLoS Negl Trop Dis. (2011) 5:e1365. doi: 10.1371/journal.pntd.0001365
68. Stauch A, Sarkar RR, Picado A, Ostyn B, Sundar S, Rijal S, et al. Visceral leishmaniasis in the Indian subcontinent: modelling epidemiology and control. PLoS Negl Trop Dis. (2011) 5:e1405. doi: 10.1371/journal.pntd.0001405
69. Pereira-Carvalho R, Mendes-Aguiar CO, Oliveira-Neto MP, Covas CJ, Bertho AL, Da-Cruz AM, et al. Leishmania Braziliensis-reactive T cells are down-regulated in long-term cured cutaneous leishmaniasis, but the renewal capacity of T effector memory compartments is preserved. PLoS One. (2013) 8:e81529. doi: 10.1371/journal.pone.0081529
70. Marlet MVL, Sang DK, Ritmeijer K, Muga RO, Onsongo J, Davidson RN. Emergence or re-emergence of visceral leishmaniasis in areas of Somalia, northeastern Kenya, and south-eastern Ethiopia in 2000–2001. Trans R Soc Trop Med Hygiene. (2003) 97:515–8. doi: 10.1016/S0035-9203(03)80012-3
71. Bulstra CA, Le Rutte EA, Malaviya P, Hasker EC, Coffeng LE, Picado A, et al. Visceral leishmaniasis: spatiotemporal heterogeneity and drivers underlying the hotspots in muzaffarpur, bihar, India. PLoS Negl Trop Dis. (2018) 12:e0006888. doi: 10.1371/journal.pntd.0006888
72. Dos Santos PL, de Oliveira FA, Santos MLB, Cunha LCS, Lino MT, de Oliveira MF, et al. The severity of visceral leishmaniasis correlates with elevated levels of serum il-6, il-27 and scd14. PLoS Negl Trop Dis. (2016) 10:e0004375. doi: 10.1371/journal.pntd.0004375
73. Hasker E, Malaviya P, Gidwani K, Picado A, Ostyn B, Kansal S, et al. Strong association between serological status and probability of progression to clinical visceral leishmaniasis in prospective cohort studies in India and Nepal. PLoS Negl Trop Dis. (2014) 8:e2657. doi: 10.1371/journal.pntd.0002657
74. Le Rutte EA, Coffeng LE, Bontje DM, Hasker EC, Ruiz Postigo JA, Argaw D, et al. Feasibility of eliminating visceral leishmaniasis from the Indian subcontinent: explorations with a set of deterministic age-structured transmission models. Parasites Vectors. (2016) 9:1–14. doi: 10.1186/s13071-016-1292-0
75. Singh OP, Tiwary P, Kushwaha AK, Singh SK, Singh DK, Lawyer P, et al. Xenodiagnosis to evaluate the infectiousness of humans to sandflies in an area endemic for visceral leishmaniasis in bihar, India: A transmission-dynamics study. Lancet Microbe. (2021) 2:e23–31. doi: 10.1016/S2666-5247(20)30166-X
76. Mondal D, Alvar J, Hasnain MG, Hossain MS, Ghosh D, Huda MM, et al. Efficacy and safety of single-dose liposomal amphotericin B for visceral leishmaniasis in a rural public hospital in Bangladesh: A feasibility study. Lancet Global Health. (2014) 2:e51–e7. doi: 10.1016/S2214-109X(13)70118-9
77. Laguna F, López-Vélez R, Pulido F, Salas A, Torre-Cisneros J, Torres E, et al. Treatment of visceral leishmaniasis in hiv-infected patients: A randomized trial comparing meglumine antimoniate with amphotericin B. Aids. (1999) 13:1063–9. doi: 10.1097/00002030-199906180-00009
78. Rijal S, Yardley V, Chappuis F, Decuypere S, Khanal B, Singh R, et al. Antimonial treatment of visceral leishmaniasis: are current in vitro susceptibility assays adequate for prognosis of in vivo therapy outcome? Microbes. (2007) 9:529–35. doi: 10.1016/j.micinf.2007.01.009
79. Gorski S, Collin SM, Ritmeijer K, Keus K, Gatluak F, Mueller M, et al. Visceral leishmaniasis relapse in southern Sudan (1999–2007): A retrospective study of risk factors and trends. PLoS Negl Trop Dis. (2010) 4:e705. doi: 10.1371/journal.pntd.0000705
80. Cota GF, De Sousa MR, Rabello A. Predictors of visceral leishmaniasis relapse in hiv-infected patients: A systematic review. PLoS Negl Trop Dis. (2011) 5:e1153. doi: 10.1371/journal.pntd.0001153
81. Taylor AR, Watson JA, Chu CS, Puaprasert K, Duanguppama J, Day NP, et al. Resolving the cause of recurrent plasmodium vivax malaria probabilistically. Nat Commun. (2019) 10:1–11. doi: 10.1038/s41467-019-13412-x
82. Mandell MA, Beverley SM. Concomitant immunity induced by persistent leishmania major does not preclude secondary re-infection: implications for genetic exchange, diversity and vaccination. PLoS Negl Trop Dis. (2016) 10:e0004811. doi: 10.1371/journal.pntd.0004811
83. Mandell MA, Beverley SMJ. Continual renewal and replication of persistent leishmania major parasites in concomitantly immune hosts. Proc Natl Acad Sci. (2017) 114:E801–E10. doi: 10.1073/pnas.1619265114
84. Carter R, Mendis KN. Evolutionary and historical aspects of the burden of malaria. Clin Microbiol Rev. (2002) 15:564–94. doi: 10.1128/CMR.15.4.564-594.2002
85. Doolan DL, Dobaño C, Baird JK. Acquired immunity to malaria. Clin Microbiol Rev. (2009) 22:13–36. doi: 10.1128/CMR.00025-08
86. Casado JL, Abad-Fernández M, Moreno S, Pérez-Elías MJ, Moreno A, Bernardino JI, et al. Visceral leishmaniasis as an independent cause of high immune activation, T-cell senescence, and lack of immune recovery in virologically suppressed hiv-1-coinfected patients. HIV Med. (2015) 16:240–8. doi: 10.1111/hiv.12206
87. Vallejo A, Abad-Fernández M, Moreno S, Moreno A, Pérez-Elías MJ, Dronda F, et al. High levels of cd4⁺ Ctla-4⁺ Treg cells and ccr5 density in hiv-1-infected patients with visceral leishmaniasis. Eur J Clin Microbiol Infect diseases: Off Publ Eur Soc Clin Microbiol. (2015) 34:267–75. doi: 10.1007/s10096-014-2229-1
88. Okwor I, Uzonna JE. The immunology of leishmania/hiv co-infection. Immunologic Res. (2013) 56:163–71. doi: 10.1007/s12026-013-8389-8
89. Singh OP, Chaubey R, Kushwaha AK, Fay MP, Sacks D, Sundar S. Visceral leishmaniasis-human immunodeficiency virus-coinfected patients are highly infectious to sandflies in an endemic area in India. J Infect Dis. (2024) 229:1909–12. doi: 10.1093/infdis/jiae156
90. Cloots K, Marino P, Burza S, Gill N, Boelaert M, Hasker E. Visceral leishmaniasis-hiv coinfection as a predictor of increased leishmania transmission at the village level in bihar, India. Front Cell infection Microbiol. (2021) 11:604117. doi: 10.3389/fcimb.2021.604117
91. Pinto AI, Caldas C, Santarém N, Luelmo S, Costa I, Martins C, et al. Leishmania and hiv co-infection: first naturally leishmania strain presenting decreased susceptibility to miltefosine, recovered from a patient in Portugal. J infection Public Health. (2024) 17:810–8. doi: 10.1016/j.jiph.2024.03.008
92. Lindoso JA, Cota GF, da Cruz AM, Goto H, Maia-Elkhoury AN, Romero GA, et al. Visceral leishmaniasis and hiv coinfection in latin america. PLoS Negl Trop Dis. (2014) 8:e3136. doi: 10.1371/journal.pntd.0003136
93. Cota GF, de Sousa MR, de Mendonça AL, Patrocinio A, Assunção LS, de Faria SR, et al. Leishmania-hiv co-infection: clinical presentation and outcomes in an urban area in Brazil. PLoS Negl Trop Dis. (2014) 8:e2816. doi: 10.1371/journal.pntd.0002816
94. Malafaia G. Protein-energy malnutrition as a risk factor for visceral leishmaniasis: A review. Parasite Immunol. (2009) 31:587–96. doi: 10.1111/j.1365-3024.2009.01117.x
95. Feleke BE. Nutritional status of visceral leishmaniasis patients: A comparative cross-sectional study. Clin Nutr ESPEN. (2019) 33:139–42. doi: 10.1016/j.clnesp.2019.06.005
96. Sacramento LA, Lombana C, Scott P. Malnutrition disrupts adaptive immunity during visceral leishmaniasis by enhancing il-10 production. bioRxiv: preprint server Biol. (2024). doi: 10.1101/2024.06.06.597776
97. Kumar VU, Kt MF, Sharma A, Bisht P, Dhingra S, Ravichandiran V, et al. The possible role of selected vitamins and minerals in the therapeutic outcomes of leishmaniasis. Biol Trace element Res. (2023) 201:1672–88. doi: 10.1007/s12011-022-03311-6
98. Addy M, Nandy A. Ten years of kala-azar in west bengal, part I. Did post-kala-azar dermal leishmaniasis initiate the outbreak in 24-parganas? Bull World Health Organ. (1992) 70:341–6.
99. Deb RM, Stanton MC, Foster GM, Das Gupta RK, Roy N, Das P, et al. Visceral leishmaniasis cyclical trends in bihar, India - implications for the elimination programme. Gates Open Res. (2018) 2:10. doi: 10.12688/gatesopenres.12793.1
100. Malaviya P, Picado A, Singh SP, Hasker E, Singh RP, Boelaert M, et al. Visceral leishmaniasis in muzaffarpur district, bihar, India from 1990 to 2008. PLoS One. (2011) 6:e14751. doi: 10.1371/journal.pone.0014751
101. Khan AM, Dutta P, Khan SA, Baruah SK, Raja D, Khound K, et al. Kala-azar and post-kala-azar dermal leishmaniasis, assam, India. Emerging Infect Dis. (2014) 20:487–9. doi: 10.3201/eid2003.130260
102. Kishore K, Kumar V, Kesari S, Dinesh D, Kumar A, Das P, et al. Vector control in leishmaniasis. Indian J Med Res. (2006) 123:467.
103. Sharma U, Singh S. Insect vectors of leishmania: distribution, physiology and their control. J Vector Borne Dis. (2008) 45:255–72.
104. Aversi-Ferreira RA, Galvão JD, da Silva SF, Cavalcante GF, da Silva EV, Bhatia-Dey N, et al. Geographical and environmental variables of leishmaniasis transmission. Leishmaniasis-trends epidemiology diagnosis Treat InTech. (2014), 105–24. doi: 10.5772/57067
105. Matsumoto PSS, Hiramoto RM, Pereira VBR, Camprigher VM, Taniguchi HH, de Raeffray Barbosa JE, et al. Impact of the household environment risk for maintenance of natural foci of leishmania infantum transmission to human and animal hosts in endemic areas for visceral leishmaniasis in sao paulo state, Brazil. medRxiv. (2021) 16:e0256534. doi: 10.1101/2021.05.18.21257380
106. Medkour H, Davoust B, Dulieu F, Maurizi L, Lamour T, Marié JL, et al. Potential animal reservoirs (Dogs and bats) of human visceral leishmaniasis due to leishmania infantum in French Guiana. PLoS Negl Trop Dis. (2019) 13:e0007456. doi: 10.1371/journal.pntd.0007456
107. Tsegaw T, Gadisa E, Seid A, Abera A, Teshome A, Mulugeta A, et al. Identification of environmental parameters and risk mapping of visceral leishmaniasis in Ethiopia by using geographical information systems and a statistical approach. Geospatial Health. (2013) 7:299–308. doi: 10.4081/gh.2013.88
108. Topno RK, Das VN, Ranjan A, Pandey K, Singh D, Kumar N, et al. Asymptomatic infection with visceral leishmaniasis in a disease-endemic area in bihar, India. Am J Trop Med hygiene. (2010) 83:502–6. doi: 10.4269/ajtmh.2010.09-0345
109. Le Rutte EA, Zijlstra EE, de Vlas SJ. Post-kala-azar dermal leishmaniasis as a reservoir for visceral leishmaniasis transmission. Trends Parasitol. (2019) 35:590–2. doi: 10.1016/j.pt.2019.06.007
110. Mondal D, Bern C, Ghosh D, Rashid M, Molina R, Chowdhury R, et al. Quantifying the infectiousness of post-kala-azar dermal leishmaniasis toward sand flies. Clin Infect diseases: an Off Publ Infect Dis Soc America. (2019) 69:251–8. doi: 10.1093/cid/ciy891
111. Arce A, Estirado A, Ordobas M, Sevilla S, García N, Moratilla L, et al. Re-Emergence of leishmaniasis in Spain: community outbreak in madrid, Spain, 2009 to 2012. Eurosurveillance. (2013) 18:20546. doi: 10.2807/1560-7917.ES2013.18.30.20546
112. Carrillo E, Moreno J, Cruz I. What is responsible for a large and unusual outbreak of leishmaniasis in madrid? Trends Parasitol. (2013) 29:579–80. doi: 10.1016/j.pt.2013.10.007
113. Zijlstra E, El-Hassan A. Leishmaniasis in Sudan. 3. Visceral leishmaniasis. Trans R Soc Trop Med Hygiene. (2001) 95:S27–58. doi: 10.1016/S0035-9203(01)90218-4
114. Bussotti G, Gouzelou E, Côrtes Boité M, Kherachi I, Harrat Z, Eddaikra N, et al. Leishmania genome dynamics during environmental adaptation reveal strain-specific differences in gene copy number variation, karyotype instability, and telomeric amplification. MBio. (2018) 9:e01399–18. doi: 10.1128/mBio.01399-18
115. Gedda MR, Singh B, Kumar D, Singh AK, Madhukar P, Upadhyay S, et al. Post kala-azar dermal leishmaniasis: A threat to elimination program. PLoS Negl Trop Dis. (2020) 14:e0008221. doi: 10.1371/journal.pntd.0008221
116. Mukhopadhyay D, Dalton JE, Kaye PM, Chatterjee M. Post kala-azar dermal leishmaniasis: an unresolved mystery. Trends Parasitol. (2014) 30:65–74. doi: 10.1016/j.pt.2013.12.004
117. Zijlstra EE. The immunology of post-kala-azar dermal leishmaniasis (Pkdl). Parasites Vectors. (2016) 9:1–9. doi: 10.1186/s13071-016-1721-0
118. Gasim S, Elhassan AM, Kharazmi A, Khalil EAG, Ismail A, Theander T. The development of post-kala-azar dermal leishmaniasis (Pkdl) is associated with acquisition of leishmania reactivity by peripheral blood mononuclear cells (Pbmc). Clin Exp Immunol. (2000) 119:523–9. doi: 10.1046/j.1365-2249.2000.01163.x
119. Ansari NA, Ramesh V, Salotra P. Interferon (Ifn)–Γ, tumor necrosis factor–Α, interleukin-6, and ifn-Γ Receptor 1 are the major immunological determinants associated with post–kala azar dermal leishmaniasis. J Infect Dis. (2006) 194:958–65. doi: 10.1086/jid.2006.194.issue-7
120. Katara GK, Ansari NA, Verma S, Ramesh V, Salotra P. Foxp3 and il-10 expression correlates with parasite burden in lesional tissues of post kala azar dermal leishmaniasis (Pkdl) patients. PLoS Negl Trop Dis. (2011) 5:e1171. doi: 10.1371/journal.pntd.0001171
121. Momeni AZ, Jalayer T, Emamjomeh M, Khamesipour A, Zicker F, Ghassemi RL, et al. A randomised, double-blind, controlled trial of a killed L. Major vaccine plus bcg against zoonotic cutaneous leishmaniasis in Iran. Vaccine. (1999) 17:466–72. doi: 10.1016/S0264-410X(98)00220-5
122. Khamesipour A, Dowlati Y, Asilian A, Hashemi-Fesharki R, Javadi A, Noazin S, et al. Leishmanization: use of an old method for evaluation of candidate vaccines against leishmaniasis. Vaccine. (2005) 23:3642–8. doi: 10.1016/j.vaccine.2005.02.015
123. Koh CC, Wardini AB, Vieira M, Passos LSA, Martinelli PM, Neves EGA, et al. Human cd8+ T cells release extracellular traps co-localized with cytotoxic vesicles that are associated with lesion progression and severity in human leishmaniasis. Front Immunol. (2020) 8:594581. doi: 10.3389/fimmu.2020.594581
124. Pacheco-Fernandez T, Volpedo G, Gannavaram S, Bhattacharya P, Dey R, Satoskar A, et al. Revival of leishmanization and leishmanin. Front Cell Infection Microbiol. (2021) 11:639801. doi: 10.3389/fcimb.2021.639801
125. Seyed N, Peters NC, Rafati S. Translating Observations from Leishmanization into Non-Living Vaccines: The Potential of Dendritic Cell-Based Vaccination Strategies against Leishmania. Front Immunol. (2018) 9:1227. doi: 10.3389/fimmu.2018.01227
126. Trigo J, Abbehusen M, Netto EM, Nakatani M, Pedral-Sampaio G, de Jesus RS, et al. Treatment of canine visceral leishmaniasis by the vaccine leish-111f+ Mpl-se. Vaccine. (2010) 28:3333–40. doi: 10.1016/j.vaccine.2010.02.089
127. Le Rutte EA, Coffeng LE, Malvolti S, Kaye PM, De Vlas SJ. The potential impact of human visceral leishmaniasis vaccines on population incidence. PLoS Negl Trop Dis. (2020) 14:e0008468. doi: 10.1101/2020.05.05.20090480
128. Dias DS, Ribeiro PAF, Martins VT, Lage DP, Costa LE, Chávez-Fumagalli MA, et al. Vaccination with a cd4(+) and cd8(+) T-cell epitopes-based recombinant chimeric protein derived from leishmania infantum proteins confers protective immunity against visceral leishmaniasis. Trans research: J Lab Clin Med. (2018) 200:18–34. doi: 10.1016/j.trsl.2018.05.001
129. Sabur A, Bhowmick S, Chhajer R, Ejazi SA, Didwania N, Asad M, et al. Liposomal elongation factor-1Α Triggers effector cd4 and cd8 T cells for induction of long-lasting protective immunity against visceral leishmaniasis. Front Immunol. (2018) 9:18. doi: 10.3389/fimmu.2018.00018
130. Duthie MS, Pereira L, Favila M, Hofmeyer KA, Reed SJ, Metangmo S, et al. A defined subunit vaccine that protects against vector-borne visceral leishmaniasis. NPJ Vaccines. (2017) 2:23. doi: 10.1038/s41541-017-0025-5
131. de Carvalho Clímaco M, Kraemer L, Fujiwara RT. Vaccine development for human leishmaniasis. In: Vaccines for neglected pathogens: strategies, achievements and challenges: focus on leprosy, leishmaniasis, melioidosis and tuberculosis. Springer International Publishing, Cham (2023). p. 307–26. doi: 10.1007/978-3-031-24355-4
132. de Vrij N, Pollmann J, Rezende AM, Ibarra-Meneses AV, Pham TT, Hailemichael W, et al. Persistent T cell unresponsiveness associated with chronic visceral leishmaniasis in hiv-coinfected patients. Commun Biol. (2024) 7:524. doi: 10.1038/s42003-024-06225-2
133. Helou DG, Mauras A, Fasquelle F, Lanza JS, Loiseau PM, Betbeder D, et al. Intranasal vaccine from whole leishmania donovani antigens provides protection and induces specific immune response against visceral leishmaniasis. PLoS Negl Trop Dis. (2021) 15:e0009627. doi: 10.1371/journal.pntd.0009627
134. Nateghi-Rostami M, Sohrabi Y. Memory T cells: promising biomarkers for evaluating protection and vaccine efficacy against leishmaniasis. Front Immunol. (2024) 15:1304696. doi: 10.3389/fimmu.2024.1304696
135. De Brito RCF, JMdO C, Reis LES, Mathias FAS, Aguiar-Soares RD, Teixeira-Carvalho A, et al. Synthetic peptides elicit strong cellular immunity in visceral leishmaniasis natural reservoir and contribute to long-lasting polyfunctional T-cells in balb/C mice. Vaccines. (2019). doi: 10.3390/vaccines7040162
136. Brito RCFD, Ruiz JC, JMdO C, Ostolin TLVDP, Reis LES, Mathias FAS, et al. Chimeric vaccines designed by immunoinformatics-activated polyfunctional and memory T cells that trigger protection against experimental visceral leishmaniasis. Vaccines. (2020) 8:252. doi: 10.3390/vaccines8020252
137. Bankoti R, Gupta K, Levchenko A, Stäger S. Marginal zone B cells regulate antigen-specific T cell responses during infection. J Immunol. (2012) 188:3961–71. doi: 10.4049/jimmunol.1102880
138. Rodrigues A, Claro M, Alexandre-Pires G, Santos-Mateus D, Martins C, Valério-Bolas A, et al. Leishmania infantum antigens modulate memory cell subsets of liver resident T lymphocyte. Immunobiology. (2017) 222:409–22. doi: 10.1016/j.imbio.2016.08.009
139. Pérez-Jiménez E, Kochan G, Gherardi MM, Esteban M. infection. Mva-lack as a safe and efficient vector for vaccination against leishmaniasis. Microbes infection. (2006) 8:810–22. doi: 10.1016/j.micinf.2005.10.004
140. Ramos I, Alonso A, Marcen JM, Peris A, Castillo J, Colmenares M, et al. Heterologous prime-boost vaccination with a non-replicative vaccinia recombinant vector expressing lack confers protection against canine visceral leishmaniasis with a predominant th1-specific immune response. Vaccine. (2008) 26:333–44. doi: 10.1016/j.vaccine.2007.11.021
141. Sánchez-Sampedro L, Gómez CE, Mejías-Pérez E, S. Sorzano CO, Esteban M. High quality long-term cd4+ and cd8+ Effector memory populations stimulated by DNA-lack/mva-lack regimen in leishmania major balb/C model of infection. PLoS One. (2012) 7:e38859. doi: 10.1371/journal.pone.0038859
142. Goto Y, Bhatia A, Raman VS, Liang H, Mohamath R, Picone AF, et al. Ksac, the first defined polyprotein vaccine candidate for visceral leishmaniasis. Clin Vaccine Immunol. (2011) 18:1118–24. doi: 10.1128/CVI.05024-11
143. Hailu A, van Baarle D, Knol GJ, Berhe N, Miedema F, Kager PA. T cell subset and cytokine profiles in human visceral leishmaniasis during active and asymptomatic or sub-clinical infection with leishmania donovani. Clin Immunol (Orlando Fla). (2005) 117:182–91. doi: 10.1016/j.clim.2005.06.015
144. Adem E, Tajebe F, Getahun M, Kiflie A, Diro E, Hailu A, et al. Successful treatment of human visceral leishmaniasis restores antigen-specific ifn-Γ, but not il-10 production. PLoS Negl Trop Dis. (2016) 10:e0004468. doi: 10.1371/journal.pntd.0004468
145. Bhattacharya P, Ghosh S, Ejazi SA, Rahaman M, Pandey K, Ravi Das VN, et al. Induction of il-10 and tgfβ from cd4+Cd25+Foxp3+ T cells correlates with parasite load in Indian kala-azar patients infected with leishmania donovani. PLoS Negl Trop Dis. (2016) 10:e0004422. doi: 10.1371/journal.pntd.0004422
Keywords: leishmaniasis, immunological memory, CD4 + T cell responses, effector T cells, tissue resident memory T cells, vaccines
Citation: Tiwari R, Kumar A, Singh VK, Rajneesh, Chauhan SB, Sundar S, Nylén S, Engwerda C and Kumar R (2024) The development and maintenance of immunity against visceral leishmaniasis. Front. Immunol. 15:1486407. doi: 10.3389/fimmu.2024.1486407
Received: 26 August 2024; Accepted: 20 November 2024;
Published: 09 December 2024.
Edited by:
Thiago Almeida Pereira, Stanford University, United StatesReviewed by:
Paula Mello De Luca, Oswaldo Cruz Foundation (Fiocruz), BrazilRahul Shivahare, The Ohio State University, United States
Dirlei Nico, Federal University of Rio de Janeiro, Brazil
Copyright © 2024 Tiwari, Kumar, Singh, Rajneesh, Chauhan, Sundar, Nylén, Engwerda and Kumar. This is an open-access article distributed under the terms of the Creative Commons Attribution License (CC BY). The use, distribution or reproduction in other forums is permitted, provided the original author(s) and the copyright owner(s) are credited and that the original publication in this journal is cited, in accordance with accepted academic practice. No use, distribution or reproduction is permitted which does not comply with these terms.
*Correspondence: Rajiv Kumar, cmFqaXYua3VtYXJAYmh1LmFjLmlu; Christian Engwerda, Y2hyaXN0aWFuLmVuZ3dlcmRhQHFpbXJiZXJnaG9mZXIuZWR1LmF1