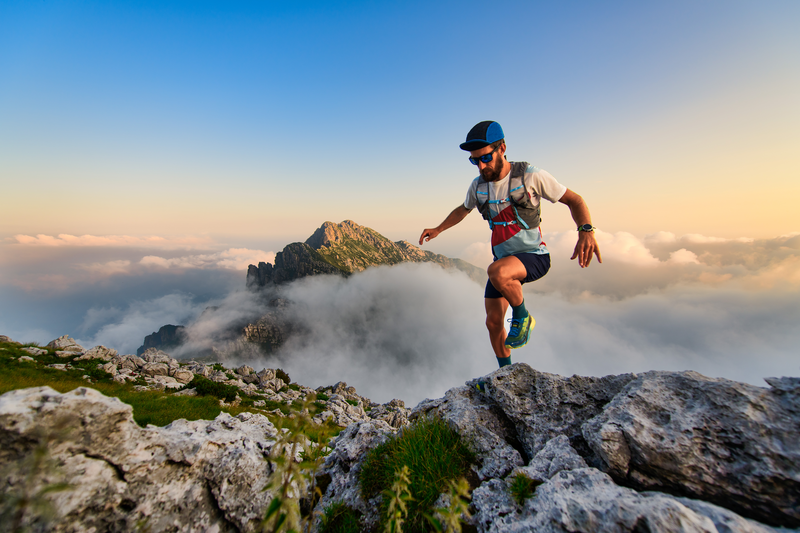
94% of researchers rate our articles as excellent or good
Learn more about the work of our research integrity team to safeguard the quality of each article we publish.
Find out more
ORIGINAL RESEARCH article
Front. Immunol. , 27 January 2025
Sec. Inflammation
Volume 15 - 2024 | https://doi.org/10.3389/fimmu.2024.1485009
This article is part of the Research Topic The Role of Inflammation in Organ Injury View all 11 articles
Background: Multisystem inflammatory syndrome in children (MIS-C) is a rare and severe post-COVID-19 complication with multiple phenotypes.
Objectives: The aim of this study is to study inflammatory biomarkers (cytokines and oxidative stress) in critical MIS-C patients and to observe if there is association between these biomarkers and mortality.
Methods: A single-center prospective study enrolled patients with MIS-C (with positive molecular test), aged between 1 month and 18 years of age. Data was collected from 20 pediatric intensive care unit (PICU)’s bed. Inflammatory biomarkers (cytokines and oxidative stress markers) were performed on day 1 and 3 after hospitalization. Survival rate was calculated, and Kaplan-Meier curves were plotted. Univariate and multivariate Cox regression analyses were conducted. The ROC (Receiver Operating Characteristic) curve analysis was performed.
Results and conclusions: A total of 41 patients out of 109 patients admitted at PICU with suspected MIS-C during the study period were included, of which 33 (80.5%) were male, 9 (22%) were under one year old, and 30 (73.2%) presented comorbidities. Among them, 16 (39%) did not survive. The mean survival time was shorter in patients with higher levels of IL-17A (≥ 19.71 pg/mL) on day 1 (115 vs 323 days, p = 0.004). Higher levels of IL-17A on day 1 were associated with mortality in both the crude model (HR 1.03, CI95% 1.004-1.057, p = 0.022) and the adjusted model (HR 1.043, CI95% 1.013-1.075, p = 0.012). ROC analysis revealed a cut-off value for the IL-17A of 14.32 pg/ml. The other immunological and inflammatory markers did not demonstrate an association with survival (p>0.05). Our findings suggest that patients with high levels of IL-17A are at greater risk for death.
At the onset of the coronavirus disease 2019 (COVID-19) pandemic, a severe multisystem inflammatory syndrome in children (MIS-C) emerged in various countries across Europe and North America (1–4). MIS-C is typified by systemic inflammation, with clinical, epidemiological, or microbiological indicators of exposure to severe acute respiratory syndrome coronavirus 2 (SARS-CoV-2). Presently, it is considered a rare yet severe complication post-COVID-19, presenting with multiple clinical phenotypes (4). Consequently, early recognition, intervention, and admission to a pediatric intensive care unit (PICU) are typically necessary (1–5).
In both COVID-19 and MIS-C, as seen in other chronic infections, there is a notable dysregulation involving hyperactivation of CD4+ T lymphocytes, potentially leading to the exhaustion of CD8+ T lymphocytes. This dysregulation suggests a reduction in antiviral immunity, which may exacerbate the clinical severity of MIS-C, particularly in children under the age of two (6). A distinct immune response is noted between COVID-19 and MIS-C, characterized by a persistent elevation in pro-inflammatory cytokines such as IL-1β, IL-2, IL-6, IFN-α, IL-10, IL-17, granulocyte-macrophage colony-stimulating factor (GM-CSF), and high-mobility group box 1 (HMGB1) among MIS-C patients (6–12).
Respiratory viral infections, including SARS-CoV-2, can instigate genomic damage by provoking excessive cytokine release, elevating inflammatory levels that destabilize immune response (6, 7). This inflammatory cascade often coincides with increased intracellular levels of reactive oxygen species (ROS), causing oxidative damage to lipids, proteins, and DNA—a condition termed oxidative stress (OS) (13, 14). Prior research has shown that such cytokine release is amplified during COVID-19, potentially resulting in acute lung injury and, in severe cases, mortality (6–12). Elevated OS has also been suggested as a contributory factor in the disease’s pathogenesis (13–18).
Emerging research underscores the significant role of OS and cytokine dysregulation in the pathophysiology of MIS-C associated with COVID-19. Elevated ROS levels have been associated with cellular damage in COVID-19 cases, with oxidative damage to lipids, proteins, and DNA potentially leading to organ dysfunction and severe outcomes (12, 13, 17). In MIS-C, specific oxidative stress biomarkers—such as catalase (CAT), malondialdehyde (MDA), and trolox equivalent antioxidant capacity (TEAC)—have shown variable levels, some correlating with disease severity and mortality (15, 16, 18). These biomarkers reflect oxidative damage as well as the body’s compensatory antioxidant mechanisms, offering valuable insights into the severity of MIS-C (12–18).
However, current studies on ROS and cytokine profiles in MIS-C remain limited. Most available studies explore antioxidant therapies in non-pediatric populations (13, 14), focus on mixed pediatric populations (15–20), or employ experimental murine models (21, 22), while few concentrate specifically on critical MIS-C cases and their association with mortality.
The primary objective of this study is to evaluate inflammatory biomarkers in MIS-C patients admitted to the pediatric intensive care unit (PICU) and to determine their potential as predictors of in-hospital mortality. A secondary objective is to assess the relationship between these biomarkers and the occurrence of cardiogenic shock, as well as PICU length of stay. Additionally, we aim to compare laboratory parameters between days 1 and 3 of hospitalization and to evaluate the accuracy of IL-17A levels in predicting mortality among this patient population.
This was a single center observational prospective study with a convenience sample consisting of patients diagnosed with MIS-C between one month and 18 years of age, with confirmed SARS-CoV-2 infection (molecular test), and who required hospitalization in PICU. Patients were recruited from a tertiary Service in Belém, state of Pará, Brazil, between May 1, 2020, and December 31, 2023. All patients included in this study fulfilled the MIS-C case definition of World Health Organization (WHO) (4). Patients with similar presentation but with positive microbiology other than SARS-CoV-2 and those with immunosuppression status were excluded. Ethics approvals were obtained from the institutional review board of the Institution. We obtained a waiver of written informed consent to conduct this observational study.
Recognizing the unprecedented challenges posed by MIS-C and the need for comprehensive biomarker analysis, a task force was organized to support both the clinical and research aspects of this study. In practical terms, despite the designation as a single-center study, an informal network of pediatric intensivists in the region collaborated to facilitate active case identification. As MIS-C cases were identified in other local PICUs, the attending physicians promptly contacted the lead investigator to expedite the transfer of patients meeting MIS-C criteria to the primary institution for consistent clinical and laboratory management. This approach ensured a streamlined and uniform protocol for patient assessment and sample collection. The biomarker analyses were conducted within this centralized framework, though specific aspects required external partnerships for optimized processing. The oxidative stress measurements were conducted in collaboration with the Laboratory of In Vitro and Microbiology Assays at the Federal University of Pará, and cytokine level assessments were performed at the Evandro Chagas Institute’s Immunology Laboratory. These collaborations aimed to enhance the diagnostic and prognostic understanding of MIS-C, especially considering the notably higher mortality rates observed in our region compared to global data. As for the ethics approval process, the institutional review board approval was obtained solely from the primary hospital, as this institution served as the formal site of data collection, patient care, and biomarker analyses.
The PICU fulfil international criteria (23) for quaternary care levels, characterized by having a high level of resources and access to Mechanical Ventilation (MV), Renal Replacement Therapy (RRT), vasoactive/inotropic medications, and treatments targeted to MIS-C (Intravenous Immunoglobulin [IVIG] and steroids). They also serve as training centers for medical and health professionals.
Patients were splitted into two groups based on the main outcome, i.e., in-hospital mortality up to 28th day: survivors and non-survivors. Presence of cardiogenic shock, critical disease, and length of stay at the Hospital were evaluated. The 28-day mortality endpoint was assessed according to current pattern of outcome measures of clinical studies in order not to achieve an ambiguous measure (24). All outcomes and the worst features were recorded and compared between the 2 groups at admission and on the third day.
Clinical and laboratory data as well as demographic and epidemiological characteristics were collected from electronic records of the patients in PICU. Intensive support characteristics were also assessed sequentially until the end of hospitalization. The patients presented critical or non-critical disease according to the multiple organ dysfunction syndrome (MODS) but at least two organic dysfunctions should be present (25–28).
The presence of critical illness was determined by the finding of MODS which was defined as the simultaneous occurrence of three or more organ dysfunctions according to previously published criteria (25–28). Some patients in our study were hospitalized in the PICU only for monitoring, and they were not considered for classification in critical illness. The definition and categorization of comorbidities were based on the classification by Feudtner et al., named Pediatric Complex Chronic Conditions (PCCC) (29).
In the current study, circulatory shock was defined by altered levels of consciousness, decreased urine output (< 1 mL/kg/h or 12 mL/m²/h), and lactic acidosis, with arterial hypotension being a late sign of shock in children. Meanwhile, cardiogenic shock was defined by the presence of an ejection fraction less than 55%, as assessed by a pediatric echocardiographer using the Teichholz method, troponin elevation to twice the reference value, and the presence of clinical signs of circulatory shock (30, 31). Severely immunocompromised patients: HIV carriers; patients on immunosuppressive therapy post-solid organ or bone marrow transplant; neutropenic patients (below 500 neutrophils/mm3 or below 1000/mm3 with a downward trend); patients undergoing chemotherapy in the last 28 days or other immunosuppressive therapies; patients on immunosuppressive dose of corticosteroid (equivalent to 2mg/kg/day of prednisone for more than 2 weeks in those under 10 kg or 20mg/day for more than 2 weeks or 40mg of prednisone for ≥ 10 days in those over 10 kg) (32).
Blood samples were drawn by venipuncture from all patients on day 1 and 3 of the admission, before the initiation of immunomodulatory therapy. For complete blood count parameters, 2 mL of blood was taken into in tubes containing ethylenediaminetetraacetic acid (EDTA). The plasma obtained was stored at − 80°C. Next, cytokines and oxidative stress biomarkers were measured.
Plasma levels of seven cytokines (IL-2, IL-4, IL-6, IL-10, TNF, IFN-γ and IL-17A) were analyzed after filtering proteins with measurements > 30% below the detection threshold, by CBA according to the instruction of BD™ Cytometric Bead Array (CBA) Human Th1/Th2/Th17 Cytokine Kit (Becton & Dickinson, CA, USA). Measurement by flow cytometry was performed using a FACSCanto™ II cytometer (Becton & Dickinson, CA, USA). Plasma levels of the respective cytokines were expressed in pg/ml.
The nitrate (NO3 −) present in the serum samples was converted to nitrite (NO2 −) with nitrate reductase, and the nitrite concentration was determined using the Griess method (33). In brief, 100 μL of the supernatant samples were incubated with an equal volume of Griess reagent for 10 min at room temperature. The absorbance was measured on a plate scanner (Spectra Max 250; Molecular Devices, Menlo Park, CA, USA) at 550 nm. The nitrite concentration was determined using a standard curve generated using sodium nitrite (NaNO2). Nitrite production was expressed per μM.
The MDA was used for the reaction of thiobarbituric acid reactive substances (TBARS) performed according to the adapted form (34) of a previously proposed method (35). An aliquot of 1 mL of the reagent (TBA 10 nM) and 0.5 mL of the sample was added to each test tube. Then, the tubes were placed in a water bath at 94°C for 1 h. After this procedure, the samples were cooled under running water for about 15 minutes, and then 4 mL of butyl alcohol was added to each sample. Subsequently, the samples were mixed on a vortex shaker, in order to obtain the maximum extraction of MDA into the organic phase. Finally, the tubes were centrifuged at 2,500 rpm for 10 minutes. A volume of 3 mL of supernatant was pipetted to carry out spectrophotometric reading at 535 mm. Results were expressed in nmol/mL-1.
The trolox equivalent antioxidant capacity (TEAC) is a sensitive and reliable marker for detecting in vivo oxidative stress markers that may not be detectable through the measurement of a single specific antioxidant (36). TEAC level of the plasma serum samples was measured using a previously developed method (37). In this assay, 7 mM of 2,2-azinobis, 3-ethylbenzothiazoline, 6-sulfonate (ABTS) were incubated with 2.45 mM of potassium persulfate and ABTS-potassium persulfate (1: 0.5, v/v), and the mixture was allowed to stand in the dark at room temperature for 12–16 h before use. For the study, the blue green ABTS+ solution was diluted with ethanol 95% (v/v) until the absorbance reached 0.70 ± 0.02 at 734 nm. Then, 10 μL of the plasma or trolox standard was mixed with 1 mL of ABTS+ solution, and a decrease in absorbance at 734 nm was recorded after 4 min for all samples. The absorbance of the mixture was monitored at 734 nm after 6 min. For the blank, 10 μL of water instead of the sample was used and each sample was measured in triplicate. Total antioxidant potential of plasma serum was expressed as μmol/mL of TEAC and was calculated through a calibration curve plotted with different amounts of trolox (38).
Catalase (CAT) activity was determined according to a previously validated method (39). Blood samples were hemolyzed into ice water (1: 3) and then diluted in a Tris based buffer (Tris 1 M/EDTA 5 mM, pH 8.0). To verify the decay of hydrogen peroxide (H2O2), aliquots of the diluted samples were added to 900 μL of reaction solution (Tris base, H2O2 30% and ultrapure water, pH 8) (40). The decrease of H2O2 concentration was established at λ = 240 nm at 25°C for 60 seconds. CAT activity was defined as the activity required to degrade 1 mol of H2O2 for 60 seconds (pH 8 and 25°C) and was expressed as U/mg protein. The molar extinction coefficient of H2O2 used for the calculation was 39.4 cm (2)/mol. The enzymatic activity data obtained in CAT assays were normalized by the total protein concentrations, using the commercial kit (Doles, Brazil).
Determination of intracellular GSH levels was based on the ability of GSH to reduce 5,5-dithiobis-2-nitrobenzoic acid (DTNB) to nitrobenzoic acid (TNB), which was quantified by spectrophotometry at 412 nm. The methodology described by Ellman (41) as adapted for this determination, and GSH concentrations, were expressed in µmol/mL. This assay was adapted (42) for use in a microtitre plate using a microplate spectrophotometer system, Spectra MAX 250 (Molecular Devices, Union City, CA, USA).
The cohort characteristics were summarized using median (interquartile range) for continuous variables, and absolute numbers and frequencies (%) for categorical variables. Comparisons between groups were made using χ2 or Fisher exact tests for categorical variables, and Mann–Whitney U was used for continuous data. For comparison between day 1 and day 3 the Wilcoxon signed-ranks test was used. Holm-Bonferroni correction for multiple comparisons was employed adjusting the significance level when patients were compared between two groups (survivors and non-survivors).
We used the ROC (Receiver Operating Characteristic) analysis to choose the optimal cut-point associated with mortality outcome. Survival rate in days was calculated, and Kaplan-Meier curves were plotted. We performed a univariate and multivariate Cox regression “time - to first - event” analysis to determine risk factors for survival. In the regression model we adjusted for a risk of mortality using age under one year of age and presence of comorbidity simultaneously. For these analyses the adopted significance level was set at two-sided p <0.05. The ROC curve analysis was performed to determine the effectiveness of IL-17A as a biomarker to predict the likelihood of death in patients. Data analyses were conducted using SPSS (Statistical Package for the Social Sciences, Chicago, IL) version 28.0.
There were 109 patients admitted at PICU with suspected MIS-C during the study period. A total of 41 patients (median age of 55 months) were included (Figure 1). Thirty-three (80.5%) were male, nine (22%) were up to one year of age, and 30 (73.2%) presented PCCC. The median time from SARS-CoV-2 exposure to symptom onset was 23 days (IQR: 15-34, days). Critical disease and presence of more than three organ dysfunctions occurred in 21 (52.1%) and 26 (63.4%) patients, respectively. Invasive mechanical ventilation was required in 22 (53.7%), and deaths occurred in 16 (39%) patients (Table 1).
The assessment of non-specific laboratory tests between day 1 and day 3 revealed significant improvement in lymphocytes and platelets as well as in inflammatory markers, such as CRP, ESR and ferritin (Table 2). The analysis of oxidative stress markers demonstrated significant differences between Day 1 and Day 3. Levels of TEAC and GSH exhibited a notable upward trend, with TEAC (mmol/L) increasing significantly from 1.67 to 2.06 (p < 0.001) and GSH (µmol/mL) rising from 34 to 37 (p = 0.02). These changes are indicative of enhanced oxidative defense mechanisms and potentially reflect increased metabolic activity during the hospitalization period. Conversely, MDA (mmol/mL) levels decreased significantly from 3.62 to 1.96 (p = 0.002), catalase (U/mg protein) levels declined from 0.042 to 0.035 (p < 0.001), and nitrite (µM/mg protein) levels were markedly reduced from 80.5 to 54.3 (p < 0.001). These reductions suggest a diminishing inflammatory and oxidative state, likely corresponding to clinical improvement and a positive therapeutic response. In contrast, cytokine levels did not exhibit significant differences between Day 1 and Day 3, as demonstrated in Table 2.
There were 25 survivors and 16 non-survivors. Regarding cytokines, non-survivors presented higher levels of IL-17A, IL-6 and IL-4 compared to survivors on day 1 (p<0.001, p=0.001, and p=0.001, respectively) and day 3 (p<0.001, p=0.002 and p<0.001, respectively). Regarding oxidative stress markers, non-survivors presented higher levels of plasma nitrite in both days (p<0.001), and lower levels of glutathione reductase on day 3 (p<0.001) compared with survivors, as shown in Table 3. The elapsed time between admission and the initiation of immunomodulatory therapy did not reveal difference (p=0.575) between non-survivors (median 3.5 days, IQR 3.2-4.5) and survivors (median 3.5 days, IQR 3.6-5.2).
Cardiogenic shock was present in 17 (41%) patients; levels of IL-4 were significantly higher on day 3 in the group with cardiogenic shock compared to the group without cardiogenic shock (p=0.001), with no other differences among the other inflammatory biomarkers (Table 4).
In relation to the length of stay in PICU, 23 (56%) stayed longer than 7 days; none of the inflammatory biomarkers stood out in relation to this outcome (Table 4). We also carried out a comparison between critical and non-critical patients, however the results were similar to the mortality outcome.
Patients with higher levels of IL-17A (≥ 19.71 pg/mL) on day 1, higher levels of IL-4 (≥ 11.89 pg/mL) on day 1, higher levels of IL-4 (≥14.96 pg/mL) on day 3 and higher levels of NO (≥75.36 µM/mg protein) on day 1 presented shorter survival time (p=0.004, p=0.002, p=0.001, p=0.009, respectively). On the other hand, patients with lower levels of GSH (< 0.036 µmol/mL) on day 3 (p=0.009), presented shorter survival time, as showed in Figures 2–6.
After multivariate analysis, IL-17A was the only factor associated with shorter survival time on day 1 either in crude (HR 1.03, CI95%1.004-1.057, p=0.022) or adjusted models (HR 1.043, CI95%1.013-1.075, p=0.012) as shown in Table 5.
As cut-point values of inflammatory biomarkers for mortality have not yet been described in MIS-C patients, we chose to perform ROC analysis to identify the best possible cut-point associated with mortality outcome after we plotted the Kaplan-Meyer curves and performed univariate and multivariate Cox regression. We observed that the cut-off value for the IL-17A was 14.32 pg/ml with sensitivity of 99% and specificity of 80%, as showed in Figure 7.
We conducted a study with a substantial cohort of MIS-C patients requiring PICU hospitalization, focusing on clinical and laboratory characteristics, especially inflammatory biomarkers, including cytokines and oxidative stress markers. The findings revealed a high rate of in-hospital mortality, and we examined factors associated with this outcome, such as critical disease, cardiogenic shock, and PICU length of stay. While various inflammatory biomarkers showed associations with mortality, IL-17A was the only factor significantly linked with shorter survival after multivariate analysis, both in crude and adjusted models.
Previous studies have evaluated circulating cytokine profiles in MIS-C patients, but their comparability to our cohort is limited due to differences in sample size, age range, and definitions of COVID-19 and MIS-C, including coinfection presence and immunosuppressant use (6, 11, 43–49). These studies generally reported lower rates of comorbidities, reduced need for intensive care, and lower mortality than observed in our cohort. Despite these differences, prior research has consistently shown elevated inflammatory cytokines, such as IFN-γ, IL-10, IL-6, IL-8, CXCL10, MIP-1α, MIP-1β, TNF-α, and IL-17 in MIS-C patients compared to healthy controls and other pediatric COVID-19 cases (6, 11, 43–49). Nonetheless, a definitive cytokine signature for MIS-C has yet to emerge, and these studies did not examine mortality predictors.
Our findings align with studies on pediatric inflammatory biomarkers (43–49) and those from adult cohorts (50), which suggest that patients requiring PICU admission show significantly elevated IL-6, IL-10, and TNF-α levels and reduced lymphocyte counts. Additionally, elevated CRP, ESR, troponin, D-dimer, ferritin, and a tendency towards thrombocytopenia indicate acute inflammation and potential for organ dysfunction. Troponin elevation suggests myocardial injury, while high fibrinogen, elevated D-dimer, and low platelet counts in the acute phase indicate a procoagulant state and heightened risk of organ dysfunction (6, 20).
This study documented hyperinflammation marked by moderate hyperferritinemia and elevated CRP levels. Previous studies have reported even higher hyperferritinemia levels, which may differ due to our cohort’s high proportion of malnutrition, potentially affecting ferritin expression (6–11, 51, 52). Non-survivors in our cohort exhibited elevated cytokine levels (IL-2, IFN-γ, TNF-α, IL-10) at admission and increased IL-17A, IL-6, and IL-4 levels on days 1 and 3. Notably, IL-17A levels were associated with mortality when adjusted for comorbidity and age under one year. Persistently elevated IFN-γ, IL-6, and IL-10 in non-survivors also raised the possibility of an association with hemophagocytic lymphohistiocytosis (HLH) (53–60). We observed that elevated plasma IL-17A levels in MIS-C patients correlated with reduced survival time, supporting previous reports of high IL-17A in MIS-C (43–50, 53). However, few studies (19, 60–62) have examined mortality risk factors, and none investigated the relationship between cytokine levels, oxidative stress, and mortality. Our findings suggest that IL-17A may play a role in the pathogenesis of severe MIS-C.
Th17 cells, responsible for producing the IL-17 cytokine family (IL-17A to IL-17F), also include other cells such as γδ T cells, NKT cells, NK cells, neutrophils, and eosinophils in IL-17 production (63). IL-17A and IL-17F levels have been linked to chronic inflammatory diseases, such as psoriasis, multiple sclerosis, systemic lupus erythematosus, rheumatoid arthritis, and juvenile idiopathic arthritis (64–67). Th17 cells are known to recruit neutrophils to sites of inflammation, which may contribute to inflammation and tissue damage in MIS-C, similar to mechanisms observed in acute myocardial infarction and autoimmune diseases (64, 66, 68, 69).
For oxidative stress biomarkers, only NO (on both days) and GSH (on day 3) were statistically significant. High NO levels, associated with increased mortality, may result from heightened nitric oxide production, leading to cardiovascular dysfunction, bioenergetic failure, and cellular toxicity. This association is supported by the frequent cardiogenic shock, high VIS levels, and impaired oxygenation in our cohort. Similar associations have been reported in adult COVID-19 and sepsis cases (69–71). The GSH levels in MIS-C non-survivors in our study matched those reported in severe clinical cases, such as septic shock and severe COVID-19, with reduced levels indicating severe oxidative stress (61, 62, 70–74).
Catalase, crucial for decomposing hydrogen peroxide, is a vital defense against ROS-induced cellular damage. Its low levels in non-survivors suggest a weakened antioxidant defense, potentially increasing susceptibility to oxidative stress and contributing to multiorgan damage in MIS-C (12, 13, 17). Additionally, alterations in TEAC and MDA levels indicate oxidative imbalance, with MDA signifying lipid peroxidation and TEAC reflecting total antioxidant capacity, both of which may be insufficient in critical MIS-C cases (14, 18).
In summary, the interplay between cytokine-induced ROS production and oxidative stress may amplify disease severity. This feedback loop could drive further immune activation and oxidative damage, emphasizing the therapeutic potential of antioxidant or anti-cytokine treatments in MIS-C. These findings support that evaluating both cytokine and oxidative stress biomarkers may improve prognostic accuracy and inform future therapeutic approaches for MIS-C (12–18).
This study highlights the potential pathogenic mechanism in critical MIS-C involving an exaggerated immune response with self-perpetuating inflammation, paralleling autoimmune disease processes. However, further research is needed to confirm this association in MIS-C (11, 45, 47, 69). Although biomarker development faces practical limitations, it remains crucial for predicting diagnosis and mortality in critical patients. Thus, biomarkers with high diagnostic and prognostic value, especially those linked to actionable therapeutic options, are a primary focus in critical care (75).
The COVID-19 pandemic presented a profound health challenge. While MIS-C prevalence and mortality remain low, particularly in developed nations, evidence suggests that MIS-C disproportionately affects Black and Hispanic children, those under one year, and those with comorbidities. Our study corroborates this, identifying increased disease severity and mortality among Brazilian “Pardo” children, possibly due to higher SARS-CoV-2 exposure in socioeconomically disadvantaged regions and potential genetic factors (75–78).
In addressing the diagnostic limitations of this study, several constraints should be acknowledged that may impact the generalizability and depth of our findings. Firstly, as a single-center study, data collection was restricted to one institution, limiting the variability of the population sample and potentially introducing bias specific to regional patient demographics and care practices. The single-center design underscores the need for multicentric studies to validate our results across diverse populations and healthcare settings, which would improve the robustness and applicability of our findings.
Secondly, the absence of immunophenotyping represents a significant limitation, as it restricts our ability to analyze detailed immune cell subpopulations in MIS-C patients. Immunophenotyping would allow for a more nuanced understanding of the specific immune cell profiles and dysfunctions involved in MIS-C, particularly in elucidating roles of T-cell subsets and other critical immune components, which are likely central to MIS-C pathogenesis.
Additionally, methodological variations, particularly in the expression and analysis of cytokine and oxidative stress biomarkers, may influence outcome interpretation. Standardizing biomarker assessment across studies is essential to reduce variability and enhance comparability between findings. Finally, the relatively small patient sample size, a common limitation in MIS-C research, may limit the statistical power of our analyses, reducing the reliability of outcome associations and constraining subgroup analyses.
Together, these limitations highlight the need for future multicentric studies with larger sample sizes, standardized methodologies, and comprehensive immunophenotyping. Such studies are essential to validate the biomarkers identified here and to provide a more detailed understanding of immune and inflammatory processes in MIS-C, ultimately guiding improved diagnostic and therapeutic strategies.
Our study’s strengths include focusing on critically ill MIS-C patients, serial biomarker measurements prior to immunomodulatory therapy, prospective SARS-CoV-2 confirmation, and elimination of epidemiological biases. This study is pioneering within a region marked by healthcare disparities, highlighting a vulnerable subgroup and underscoring the need for targeted interventions. Although overall MIS-C mortality is low, our study shows that younger children with comorbidities face greater mortality risks. Further research should explore the pathophysiological aspects of cytokine and oxidative stress responses in MIS-C, especially the role of IL-17 levels in disease progression. Internationally standardized definitions for MIS-C and neonatal MIS associated with COVID-19 and globally compiled datasets are essential to improve management and guide future trials.
In conclusion, our findings underscore the need to include infants under 12 months in MIS-C research due to their representation in our cohort, contributing valuable insights for all pediatric age groups. This study reinforces the importance of investigating cytokine and oxidative stress biomarkers in MIS-C, as understanding these pathways could facilitate the development of targeted therapies to mitigate severe inflammation and improve patient outcomes. Our research advances understanding of MIS-C by highlighting novel associations between inflammatory biomarkers and mortality. These insights may inform future clinical management strategies, although further studies are essential to validate these findings and investigate therapeutic interventions based on these biomarkers.
The original contributions presented in the study are included in the article/supplementary material. Further inquiries can be directed to the corresponding author.
The studies involving humans were approved by COMITE DE ÉTICA EM PESQUISA/FUNDAÇÃO SANTA CASA DE MISERICÓRDIA DO PARÁ. The studies were conducted in accordance with the local legislation and institutional requirements. Written informed consent for participation in this study was provided by the participants’ legal guardians/next of kin.
EF: Conceptualization, Data curation, Formal analysis, Funding acquisition, Investigation, Methodology, Project administration, Resources, Software, Supervision, Validation, Visualization, Writing – original draft, Writing – review & editing. LN: Investigation, Methodology, Supervision, Validation, Visualization, Writing – review & editing. MPJ: Investigation, Methodology, Supervision, Validation, Visualization, Writing – review & editing. DP: Formal analysis, Investigation, Methodology, Supervision, Validation, Visualization, Writing – review & editing. APSP: Formal analysis, Investigation, Methodology, Supervision, Validation, Visualization, Writing – review & editing. AHOP: Formal analysis, Investigation, Methodology, Supervision, Validation, Visualization, Writing – review & editing. MA: Formal analysis, Investigation, Methodology, Supervision, Validation, Visualization, Writing – review & editing. KF: Formal analysis, Investigation, Methodology, Supervision, Validation, Visualization, Writing – review & editing. LA: Formal analysis, Investigation, Methodology, Supervision, Validation, Visualization, Writing – review & editing. LD: Investigation, Methodology, Supervision, Validation, Visualization, Writing – review & editing. MMMM: Conceptualization, Investigation, Methodology, Resources, Software, Supervision, Validation, Visualization, Writing – review & editing. MS: Investigation, Methodology, Software, Supervision, Validation, Visualization, Writing – review & editing. RG: Investigation, Methodology, Supervision, Validation, Visualization, Writing – review & editing. SM: Investigation, Methodology, Supervision, Validation, Visualization, Writing – review & editing. GP: Investigation, Methodology, Resources, Supervision, Validation, Visualization, Writing – review & editing. RC: Investigation, Methodology, Supervision, Validation, Visualization, Writing – review & editing. CS: Investigation, Methodology, Supervision, Validation, Visualization, Writing – review & editing. CN: Investigation, Methodology, Supervision, Validation, Visualization, Writing – review & editing. JS: Investigation, Methodology, Supervision, Validation, Visualization, Writing – review & editing. AS: Investigation, Methodology, Supervision, Validation, Visualization, Writing – review & editing. LS: Investigation, Methodology, Supervision, Validation, Visualization, Writing – review & editing. MLFMFM: Investigation, Methodology, Supervision, Validation, Visualization, Writing – review & editing. PC: Investigation, Methodology, Supervision, Validation, Visualization, Writing – review & editing. RB: Investigation, Methodology, Supervision, Validation, Visualization, Writing – review & editing. KH: Investigation, Methodology, Supervision, Validation, Visualization, Writing – review & editing. MJ: Data curation, Formal analysis, Funding acquisition, Investigation, Methodology, Project administration, Resources, Software, Supervision, Validation, Visualization, Writing – review & editing. IC: Investigation, Methodology, Supervision, Validation, Visualization, Writing – review & editing. IB: Conceptualization, Data curation, Formal analysis, Funding acquisition, Investigation, Methodology, Project administration, Software, Resources, Supervision, Validation, Visualization, Writing – original draft, Writing – review & editing. MCM: Writing – original draft, Writing – review & editing. GC: Conceptualization, Data curation, Formal analysis, Funding acquisition, Investigation, Methodology, Project administration, Resources, Software, Supervision, Validation, Visualization, Writing – original draft, Writing – review & editing. MT: Conceptualization, Data curation, Formal analysis, Funding acquisition, Investigation, Methodology, Project administration, Resources, Software, Supervision, Validation, Visualization, Writing – original draft, Writing – review & editing.
The author(s) declare that no financial support was received for the research, authorship, and/or publication of this article.
We are glad to thank Fundação Santa Casa de Misericórdia do Pará for giving us the chance to conduct this research. We would also like to extend our gratitude to the Virology Laboratory of the Evandro Chagas Institute, the Graduate Program in Neurosciences and Molecular Biology, and the Institute of Biological Sciences of the Federal University of Pará for their technical support in the determination of biomarkers. Our special thanks go to all the children, parents, and participating PICU.
The authors declare that the research was conducted in the absence of any commercial or financial relationships that could be construed as a potential conflict of interest.
The author(s) declared that they were an editorial board member of Frontiers, at the time of submission. This had no impact on the peer review process and the final decision.
All claims expressed in this article are solely those of the authors and do not necessarily represent those of their affiliated organizations, or those of the publisher, the editors and the reviewers. Any product that may be evaluated in this article, or claim that may be made by its manufacturer, is not guaranteed or endorsed by the publisher.
ABTS, 2,2-azinobis, 3-ethylbenzothiazoline, 6-sulfonate; DTNB, 5,5-dithiobis-2-nitrobenzoic acid; CAT, Catalase activity; COVID-19, coronavirus disease 2019; CBA, Cytometric Bead Array; EDTA, Ethylenediaminetetraacetic acid; GSH, Glutathione reductase; GM –CSF, Granulocyte-macrophage colony-stimulating factor; HLH, Hemophagocytic lymphohistiocytosis; HMGB1, High mobility group box 1; IVIG, Intravenous Immunoglobulin; MV, Invasive mechanical Ventilation; MODS, Multiple organ dysfunction syndrome; TNB, Nitrobenzoic acid; OS, Oxidate Stress; PCCC, Pediatric Complex Chronic Conditions; PICU, Pediatric intensive care unit; MDA, Plasma Malondialdehyde; NO, Plasma Nitrite; ROS, Reactive oxygen species; ROC, Receiver Operating Characteristic; RRT, Renal Replacement Therapy; SARS-cov-2, Severe acute respiratory syndrome coronavirus 2; MIS-C, Severe inflammatory syndrome in children; TBARS, Thiobarbituric acid reactive substances; TEAC, Trolox equivalent antioxidant capacity.
1. WHO. World Health Organization. Coronavirus disease 2019 (COVID-19). Geneva: WHO (2020). Available at: https://www.who.int/docs/default-source/coronaviruse/situation-reports/20200317-sitrep-57-covid-19.pdf?sfvrsn=a26922f2_4 (Accessed August 22, 2024).
2. Riphagen S, Gomez X, Gonzalez-Martinez C, Wilkinson N, Theocharis P. Hyperinflammatory shock in children during COVID-19 pandemic. Lancet. (2020) 395:1607–8. doi: 10.1016/S0140-6736(20)31094-1
3. Royal College of Paediatrics and Child Health. Guidance: Paediatric multisystem inflammatory syndrome temporally associated with COVID-19. London: RCPCH (2020). Available at: https://www.rcpch.ac.uk/sites/default/files/2020-05/COVID-19-Paediatric-multisystem-%20inflammatory%20syndrome-20200501.pdf (Accessed August 22, 2024).
4. Freedman S, Godfred-Cato S, Gorman R, Lodha R, Mofenson L, Murthy S, et al. BRIEF, Scientific. Multisystem inflammatory syndrome in children and adolescents temporally related to COVID-19. (2020). Available at: WHO/2019-NCoV/Sci_Brief/Multisystem_Syndrome_Children/20201 (Accessed August 22, 2024).
5. Cavalcanti A, Islabão A, Magalhães C, Veloso S, Lopes M, Prado R, et al. Paediatric inflammatory multisystem syndrome temporally associated with SARS-CoV-2 (PIMS-TS): a Brazilian cohort. Adv Rheumatol. (2022) 62:6. doi: 10.1186/s42358-022-00237-4
6. DeBiasi RL, Harahsheh AS, Srinivasalu H, Krishnan A, Sharron MP, Parikh K, et al. Multisystem inflammatory syndrome of children: subphenotypes, risk factors, biomarkers, cytokine profiles, and viral sequencing. J Pediatr. (2021) 237:125–135.e18. doi: 10.1016/j.jpeds.2021.06.002
7. Akindele NP, Kouo T, Karaba AH, Gordon O, Fenstermacher KZJ, Beaudry J, et al. Distinct cytokine and chemokine dysregulation in hospitalized children with acute coronavirus disease 2019 and multisystem inflammatory syndrome with similar levels of nasopharyngeal severe acute respiratory syndrome coronavirus 2 shedding. J Infect Dis. (2021) 224:606–15. doi: 10.1093/infdis/jiab285
8. Abo-Haded HM, Alshengeti AM, Alawfi AD, Khoshhal SQ, Al-Harbi KM, Allugmani MD, et al. Cytokine Profiling among Children with Multisystem Inflammatory Syndrome versus Simple COVID-19 Infection: A Study from Northwest Saudi Arabia. Biol (Basel). (2022) 11:946. doi: 10.3390/biology11070946
9. Rodríguez-Rubio M, Menéndez-Suso JJ, Cámara-Hijón C, Río-García M, Laplaza-González M, Amores-Hernández I, et al. Cytokine profile in children with severe multisystem inflammatory syndrome related to the coronavirus disease 2019. J Pediatr Intensive Care. (2021) 11:259–64. doi: 10.1055/s-0041-1724101
10. Lapp SA, Abrams J, Lu AT, Hussaini L, Kao CM, Hunstad DA, et al. Serologic and cytokine signatures in children with multisystem inflammatory syndrome and coronavirus disease 2019. Open Forum Infect Dis. (2022) 9(3):ofac070. doi: 10.1093/ofid/ofac070
11. Consiglio CR, Cotugno N, Sardh F, Pou C, Amodio D, Rodriguez L, et al. The immunology of multisystem inflammatory syndrome in children with COVID-19. Cell. (2020) 183:968–981.e7. doi: 10.1016/j.cell.2020.09.016
12. Rowley AH. Understanding SARS-CoV-2-related multisystem inflammatory syndrome in children. Nat Rev Immunol. (2020) 20:453–4. doi: 10.1038/s41577-020-0367-5
13. Delgado-Roche L, Mesta F. Oxidative stress as key player in severe acute respiratory syndrome coronavirus (SARS-CoV) infection. Arch Med Res. (2020) 51:384–7. doi: 10.1016/j.arcmed.2020.04.019
14. Lorente L, Martín MM, González-Rivero AF, Pérez-Cejas A, Cáceres JJ, Perez A, et al. DNA and RNA oxidative damage and mortality of patients with COVID-19. Am J Med Sci. (2021) 361:585–90. doi: 10.1016/j.amjms.2021.02.012
15. Perrone S, Cannavò L, Manti S, Rullo I, Buonocore G, Esposito SMR, et al. Pediatric multisystem syndrome associated with SARS-coV-2 (MIS-C): the interplay of oxidative stress and inflammation. Int J Mol Sci. (2022) 23:12836. doi: 10.3390/ijms232112836
16. Graciano-Machuca O, Villegas-Rivera G, López-Pérez I, Macías-Barragán J, Sifuentes-Franco S. Multisystem inflammatory syndrome in children (MIS-C) following SARS-coV-2 infection: role of oxidative stress. Front Immunol. (2021) 12:723654. doi: 10.3389/fimmu.2021.723654
17. Rivas MN, Porritt RA, Cheng MH, Bahar I, Arditi M. Multisystem inflammatory syndrome in children and long COVID: the SARS-coV-2 viral superantigen hypothesis. Front Immunol. (2022) 13:941009. doi: 10.3389/fimmu.2022.941009
18. Tsermpini EE, Glamočlija U, Ulucan-Karnak F, Redenšek Trampuž S, Dolžan V. Molecular mechanisms related to responses to oxidative stress and antioxidative therapies in COVID-19: A systematic review. Antioxidants (Basel). (2022) 11:1609. doi: 10.3390/antiox11081609
19. Gümüş H, Erat T, Öztürk İ, Demir A, Koyuncu I. Oxidative stress and decreased Nrf2 level in pediatric patients with COVID-19. J Med Virol. (2022) 94:2259–64. doi: 10.1002/jmv.27640
20. Henry BM, de Oliveira MHS, Benoit S, Plebani M, Lippi G. Hematologic, biochemical and immune biomarker abnormalities associated with severe illness and mortality in coronavirus disease 2019 (COVID-19): a meta-analysis. Clin Chem Lab Med. (2020) 58:1021–8. doi: 10.1515/cclm-2020-0369
21. Yee M, David Cohen E, Haak J, Dylag AM, O'Reilly MA. Neonatal hyperoxia enhances age-dependent expression of SARS-CoV-2 receptors in mice. Sci Rep. (2020) 10:22401. doi: 10.1038/s41598-020-79595-2
22. Keles ES. Mild SARS-CoV-2 infections in children might be based on evolutionary biology and linked with host reactive oxidative stress and antioxidant capabilities. New Microbes New Infect. (2020) 36:100723. doi: 10.1016/j.nmni.2020.100723
23. Hsu BS, Hill V, Frankel LR, Yeh TS, Simone S, Arca MJ, et al. Executive summary: criteria for critical care of infants and children: PICU admission, discharge, and triage practice statement and levels of care guidance. Pediatrics. (2019) 144(1 Suppl 1):S1–S12. doi: 10.1542/peds.2019-2433
24. Vincent JL. Endpoints in sepsis trials: More than just 28-day mortality? Crit Care Med. (2004) 32:S209–13. doi: 10.1097/01.CCM.0000126124.41743.86
25. Bembea MM, Agus M, Akcan-Arikan A, Alexander P, Basu R, Bennett TD, et al. Pediatric organ dysfunction information update (PODIUM) contemporary organ dysfunction criteria: executive summary. Pediatrics. (2022) 149:S1–S12. doi: 10.1542/peds.2021-052888B
26. Yehya N, Smith L, Thomas NJ, Steffen KM, Zimmerman J, Lee JH, et al. Definition, incidence, and epidemiology of pediatric acute respiratory distress syndrome: from the second pediatric acute lung injury consensus conference. Pediatr Crit Care Med. (2023) 24:S87–98. doi: 10.1097/PCC.0000000000003161
27. Weis SL, Peters MJ, Alhazzani W, Agus MSD, Flori HR, Inwald DP, et al. Surviving sepsis campaign international guidelines for the management of septic shock and sepsis-associated organ dysfunction in children. Pediatr Crit Care Med. (2020) 21:e52–e106. doi: 10.1097/PCC.0000000000002198
28. Goldstein B, Giroir B, Randolph A. International Consensus Conference on Pediatric Sepsis. International pediatric sepsis consensus conference: definitions for sepsis and organ dysfunction in pediatrics. Pediatr Crit Care Med. (2005) 6:2–8. doi: 10.1097/01.PCC.0000149131.72248.E6
29. Feudtner C, Feinstein JA, Zhong W, Hall M, Dai D. Pediatric complex chronic conditions classification system version 2: updated for ICD-10 and complex medical technology dependence and transplantation. BMC Pediatr. (2014) 14:199. doi: 10.1186/1471-2431-14-199
30. Lai WW, Geva T, Shirali GS, Frommelt PC, Humes RA, Brook MM, et al. Guidelines and standards for performance of a pediatric echocardiogram: A report from the task force of the pediatric council of the american society of echocardiography. J Am Soc Echocardiogr. (2006) 19:1413–30. doi: 10.1016/j.echo.2006.09.001
31. Topjian AA, Raymond TT, Atkins D, Chan M, Duff JP, Joyner BL Jr, et al. Pediatric basic and advanced life support collaborators. Part 4: pediatric basic and advanced life support: 2020 american heart association guidelines for cardiopulmonary resuscitation and emergency cardiovascular care. Circulation. (2020) 142:S469–523. doi: 10.1161/CIR.0000000000000901
32. Schnepp E, Kaminsk D. Chronic immunosuppression. Pediatr Review. (2012) 33:481–2. doi: 10.1542/pir.33.10.481
33. Granger DL, Anstey NM, Miller WC, Weinberg JB. Measuring nitric oxide production in human clinical studies. Methods Enzymol. (1999) 301:49–61. doi: 10.1016/s0076-6879(99)01068-x
34. Percário S. Dosagem das LDLs modificadas através da peroxidação lipídica: correlação com o risco aterogênico (Measurement of LDLs modified through lipid peroxidation: correlation with atherogenic risk). Anais Médicos dos Hospitais e da Faculdade Ciências Médicas da Santa Casa São Paulo. (1994) 13:7–9.
35. Kohn HI, Liversedge M. On a new aerobic metabolite whose production by brain is inhibited by apomorphine, emetine, ergotamine, epinephrine, and menadione. J Pharmacol Exp Ther. (1944) 82:292–300. Available online at: https://api.semanticscholar.org/CorpusID:84090831.
36. Cohen AA, McGraw KJ, Robinson WD. Serum antioxidant levels in wild birds vary in relation to diet, season, life history strategy, and species. Oecologia. (2009) 161:673–83. doi: 10.1007/s00442-009-1423-9
37. Rufino MSM, Alves RE, Brito ES, de Morais SM, Sampaio CG, Pérez-Jiménez J, et al. Determinação da atividade antioxidante total em frutas pela captura do radical livre DPPH (Determination of Total Antioxidant Activity in Fruits by Capture of the Free Radical DPPH). Comunicado Técnico Embrapa. (2007) 127:1–4. Available at: https://www.researchgate.net/publication/281323118_Metodologia_Cientifica_Determinaao_da_atividade_antioxidante_total_em_frutas_pela_captura_do_radical_ABTS.
38. Re R, Pellegrini N, Proteggente A, Pannala A, Yang M, Rice-Evans C. Antioxidant activity applying an improved ABTS radical cation decolorization assay. Free Radic Biol Med. (1999) 26:1231–7. doi: 10.1016/s0891-5849(98)00315-3
39. Góth L. A simple method for determination of serum catalase activity and revision of reference range. Clin Chim Acta. (1991) 196:143–51. doi: 10.1016/0009-8981(91)90067-m
40. Bukowska B, Kowalska S. Phenol and catechol induce prehemolytic and hemolytic changes in human erythrocytes. Toxicol Lett. (2004) 152:73–84. doi: 10.1016/j.toxlet.2004.03.025
41. Ellman GL. Tissue sulfhydryl groups. Arch Biochem Biophys. (1959) 82:70–7. doi: 10.1016/0003-9861(59)90090-6
42. Romão PR, Tovar J, Fonseca SG, Moraes RH, Cruz AK, Hothersall JS, et al. Glutathione and the redox control system trypanothione/trypanothione reductase are involved in the protection of Leishmania spp. against nitrosothiol-induced cytotoxicity. Braz J Med Biol Res. (2006) 39:355–63. doi: 10.1590/S0100-879X2006000300006
43. Carter MJ, Fish M, Jennings A, Doores KJ, Wellman P, Seow J, et al. Peripheral immunophenotypes in children with multisystem inflammatory syndrome associated with SARS-CoV-2 infection. Nat Med. (2020) 26:1701–7. doi: 10.1038/s41591-020-1054-6
44. Lee PY, Day-Lewis M, Henderson LA, Friedman KG, Lo J, Roberts JE, et al. Distinct clinical and immunological features of SARS-CoV-2–induced multisystem inflammatory syndrome in children. J Clin Invest. (2020) 130:5942–50. doi: 10.1172/JCI141113
45. Ramaswamy A, Brodsky NN, Sumida TS, Comi M, Asashima H, Hoehn KB, et al. Immune dysregulation and autoreactivity correlate with disease severity in SARS-CoV-2-associated multisystem inflammatory syndrome in children. Immunity. (2021) 54:1083–1095.e7. doi: 10.1016/j.immuni.2021.04.003
46. de Cevins C, Luka M, Smith N, Meynier S, Magérus A, Carbone F, et al. A monocyte/dendritic cell molecular signature of SARS-CoV-2-related multisystem inflammatory syndrome in children with severe myocarditis. Med. (2021) 2:1072–1092.e7. doi: 10.1016/j.medj.2021.08.002
47. Diorio C, Henrickson SE, Vella LA, McNerney KO, Chase JM, Burudpakdee C, et al. Multisystem inflammatory syndrome in children and COVID-19 are distinct presentations of SARS-CoV-2. J Clin Invest. (2020) 130:5967–75. doi: 10.1172/JCI140970
48. Gruber CN, Patel RS, Trachtman R, Lepow L, Amanat F, Krammer FW, et al. Mapping systemic inflammation and antibody responses in multisystem inflammatory syndrome in children (MIS-C). Cell. (2020) 183:982–995.e14. doi: 10.1016/j.cell.2020.09.034
49. Haslak F, Barut K, Durak C, Aliyeva A, Yildiz M, Guliyeva V, et al. Clinical features and outcomes of 76 patients with COVID-19-related multi-system inflammatory syndrome in children. Clin Rheumatol. (2021) 40:4167–78. doi: 10.1007/s10067-021-05780-x
50. Chen CJ, Kao HY, Huang CH, Li CJ, Hung CH, Yong SB. New insight into the intravenous immunoglobulin treatment in Multisystem Inflammatory Syndrome in children and adults. Ital J Pediatr. (2024) 50:18. doi: 10.1186/s13052-024-01585-1
51. Carcillo JA, Kernan KK, Horvat CM, Simon DW, Aneja RK. Why and how is hyperferritinemic sepsis different from sepsis without hyperferritinemia? Pediatr Crit Care Med. (2020) 21:509–12. doi: 10.1097/PCC.0000000000002285
52. World Health Organization. Serum Ferritin Concentrations for the Assessment of Iron Status and Iron Deficiency in Populations. Vitamin and Mineral Nutrition Information System (2011). Available online at: https://who.int/vmnis/indicators/serum_ferritin.pdf (Accessed August 22, 2024).
53. Molloy EJ, Nakra N, Gale C, Dimitriades VR, Lakshminrusimha S. Multisystem inflammatory syndrome in children (MIS-C) and neonates (MIS-N) associated with COVID-19: optimizing definition and management. Pediatr Res. (2023) 93:1499–508. doi: 10.1038/s41390-022-02263-w
54. Fieschi C, Dupuis S, Picard C, Smith CI, Holland SM, Casanova JL. High levels of interferon gamma in the plasma of children with complete interferon gamma receptor deficiency. Pediatrics. (2001) 107:E48. doi: 10.1542/peds.107.4.e48
55. Huang KJ, Su IJ, Theron M, Wu YC, Lai SK, Liu CC, et al. An interferon-gamma-related cytokine storm in SARS patients. J Med Virol. (2005) 75:185–94. doi: 10.1002/jmv.20255
56. Lee WI, Huang JL, Yeh KW, Jaing TH, Lin TY, Huang YC, et al. Immune defects in active mycobacterial diseases in patients with primary immunodeficiency diseases (PIDs). J Formos Med Assoc. (2011) 110:750–8. doi: 10.1016/j.jfma.2011.11.004
57. Henter JI, Horne A, Aricó M, Egeler RM, Filipovich AH, Imashuku S, et al. HLH-2004: diagnostic and therapeutic guidelines for hemophagocytic lymphohistiocytosis. Pediatr Blood Cancer. (2007) 48:124–31. doi: 10.1002/pbc.21039
58. Chen Y, Wang Z, Luo Z, Zhao N, Yang S, Tang Y. Comparison of Th1/Th2 cytokine profiles between primary and secondary haemophagocytic lymphohistiocytosis. Ital J Pediatr. (2016) 42:50. doi: 10.1186/s13052-016-0262-7
59. Tang Y, Xu X, Song H, Yang S, Shi S, Wei J, et al. Early diagnostic and prognostic significance of a specific Th1/Th2 cytokine pattern in children with haemophagocytic syndrome. Br J Haematol. (2008) 143:84–91. doi: 10.1111/j.1365-2141.2008.07298.x
60. Bracaglia C, de Graaf K, Marafon DP, Guilhot F, Ferlin W, Prencipe G, et al. Elevated circulating levels of interferon-γ and interferon-γ-induced chemokines characterize patients with macrophage activation syndrome complicating systemic juvenile idiopathic arthritis. Ann Rheum Dis. (2017) 76:166–72. doi: 10.1136/annrheumdis-2015-209020
61. Lorente L, Gómez-Bernal F, Martín MM, Navarro-Gonzálvez JA, Argueso M, Perez A, et al. High serum nitrates levels in non-survivor COVID-19 patients. Med Intensiva (Engl Ed). (2022) 46:132–9. doi: 10.1016/j.medine.2020.10.007
62. Çakırca G, Damar Çakırca T, Üstünel M, Torun A, Koyuncu İ. Thiol level and total oxidant/antioxidant status in patients with COVID-19 infection. Ir J Med Sci. (2022) 191:1925–30. doi: 10.1007/s11845-021-02743-8
63. Krasic S, Vukomanovic V, Ninic S, Pasic S, Samardzija G, Mitrovic N, et al. Mechanisms of redox balance and inflammatory response after the use of methylprednisolone in children with multisystem inflammatory syndrome associated with COVID-19. Front Immunol. (2023) 14:1249582. doi: 10.3389/fimmu.2023.1249582
64. Korn T, Bettelli E, Oukka M, Kuchroo VK. IL-17 and th17 cells. Annu Rev Immunol. (2009) 27:485–517. doi: 10.1146/annurev.immunol.021908.132710
65. Eskandari-Nasab E, Moghadampour M, Tahmasebi A. Meta-analysis of risk association between interleukin-17A and F gene polymorphisms and inflammatory diseases. J Interferon Cytokine Res. (2017) 37:165–74. doi: 10.1089/jir.2016.0088
66. Ponce-Gallegos M, Ramírez-Venegas A, Falfán-Valencia R. Th17 profile in COPD exacerbations. Int J Chron Obstruct Pulmon Dis. (2017) 12:1857–65. doi: 10.2147/COPD.S136592
67. Long X, Xie J, Ren L, Yu G, Liu E, Deng Y, et al. IL-17A plays a critical role in RSV infection in children and mice. Virol J. (2023) 20:30. doi: 10.1186/s12985-023-01990-8
68. Mora-Ruíz MD, Blanco-Favela F, Chávez RAK, Legorreta-Haquet MV, Chávez-Sánchez L. Role of interleukin-17 in acute myocardial infarction. Mol Immunol. (2019) 107:71–8. doi: 10.1016/j.molimm.2019.01.008
69. Filippatos F, Tatsi EB, Michos A. Immunology of multisystem inflammatory syndrome after COVID-19 in children: A review of the current evidence. Int J Mol Sci. (2023) 24:5711. doi: 10.3390/ijms24065711
70. Ho JT, Chapman MJ, O’Connor S, Lam S, Edwards J, Ludbrook G, et al. Characteristics of plasma NOx levels in severe sepsis: high interindividual variability and correlation with illness severity, but lack of correlation with cortisol levels. Clin Endocrinol (Oxf). (2010) 73:413–20. doi: 10.1111/j.1365-2265.2010.03817.x
71. Neves FF, Pott-Junior H, Yamashita KMC, Santos SS, Cominetti MR, Freire CCM, et al. Do the oxidative stress biomarkers predict COVID-19 outcome? An in-hospital cohort study. Free Radic Biol Med. (2023) 207:194–9. doi: 10.1016/j.freeradbiomed.2023.06.026
72. Žarković N, Jastrząb A, Jarocka-Karpowicz I, Orehovec B, Baršić B, Tarle M, et al. The impact of severe COVID-19 on plasma antioxidants. Molecules (Basel). (2022) 27:5323. doi: 10.3390/molecules27165323
73. Silvagno F, Vernone A, Pescarmona GP. The role of glutathione in protecting against the severe inflammatory response triggered by COVID-19. Antioxidants (Basel). (2020) 9:624. doi: 10.3390/antiox9070624
74. Torres A, Artigas A, Ferrer R. Biomarkers in the ICU: less is more? No. Intensive Care Med. (2021) 47:97–100. doi: 10.1007/s00134-020-06271-4
75. Kendir-Demirkol Y, Jenny LA, Demirkol A, Özen M, Ayata A, Canatan D. The protective effects of pyridoxine on linezolid-induced hematological toxicity, hepatotoxicity, and oxidative stress in rats. Turk Arch Pediatr. (2023) 58:298–301. doi: 10.5152/TurkArchPediatr.2023.21363
76. CDC COVID Data Tracker: Multisystem Inflammatory Syndrome in Children (MIS-C). Available online at: https://covid.cdc.gov/covid-data-tracker/mis-national-surveillance (Accessed August 22, 2024).
77. CDC COVID Data Tracker: Total Cases and Deaths by Race/Ethnicity, Age, and Sex. Available online at: https://covid.cdc.gov/covid-data-tracker/demographics (Accessed August 22, 2024).
Keywords: COVID-19, cytokines, mortality, oxidative stress, pediatric intensive care unit, post-acute COVID-19 syndrome
Citation: de Farias ECF, Nascimento LMPPd, Pavão Junior MJC, Pavão DCA, Pinheiro APS, Pinheiro AHO, Alves MCB, Ferraro KMMM, Aires LFQ, Dias LG, Machado MMM, Serrão MJD, Gomes RR, de Moraes SMP, Pontes GCL, Carvalho RDFP, Silva CTC, Neves CMAd, Santos JCLd, de Sousa AMB, da Silva LL, de Mello MLFMF, Carvalho PB, Braga RdB, Harada KdO, Justino MCA, Costa IB, Brasil-Costa I, Monteiro MC, Clemente G and Terreri MT (2025) Plasma IL-17A is increased in patients with critical MIS-C and associated to in-hospital mortality. Front. Immunol. 15:1485009. doi: 10.3389/fimmu.2024.1485009
Received: 23 August 2024; Accepted: 23 December 2024;
Published: 27 January 2025.
Edited by:
Paolo Biban, Integrated University Hospital Verona, ItalyReviewed by:
Ozgur Kasapcopur, Istanbul University-Cerrahpasa, TürkiyeCopyright © 2025 de Farias, Nascimento, Pavão Junior, Pavão, Pinheiro, Pinheiro, Alves, Ferraro, Aires, Dias, Machado, Serrão, Gomes, de Moraes, Pontes, Carvalho, Silva, Neves, Santos, de Sousa, da Silva, de Mello, Carvalho, Braga, Harada, Justino, Costa, Brasil-Costa, Monteiro, Clemente and Terreri. This is an open-access article distributed under the terms of the Creative Commons Attribution License (CC BY). The use, distribution or reproduction in other forums is permitted, provided the original author(s) and the copyright owner(s) are credited and that the original publication in this journal is cited, in accordance with accepted academic practice. No use, distribution or reproduction is permitted which does not comply with these terms.
*Correspondence: Emmerson C. F. de Farias, ZW1tZXJzb24uZmFyaWFzQHNhbnRhY2FzYS5wYS5nb3YuYnI=
†These authors contributed equally to this work and share senior authorship
Disclaimer: All claims expressed in this article are solely those of the authors and do not necessarily represent those of their affiliated organizations, or those of the publisher, the editors and the reviewers. Any product that may be evaluated in this article or claim that may be made by its manufacturer is not guaranteed or endorsed by the publisher.
Research integrity at Frontiers
Learn more about the work of our research integrity team to safeguard the quality of each article we publish.